Like many other species, humans are able to adapt to a wide range of environmental conditions and exposures (Bateson et al., Reference Bateson, Barker, Clutton-Brock, Deb, D'Udine, Foley and Sultan2004). Such adaptation is crucial for successful development given that different contexts may require different behavioral strategies (e.g., aggression in hostile conditions vs. cooperation in supportive contexts) in order to achieve survival and reproduction (Belsky & Pluess, Reference Belsky and Pluess2009a). However, effective adaptation is conditional on the accurate perception and interpretation of environmental cues. The general ability to register and process external stimuli has been defined as “environmental sensitivity” (Pluess, Reference Pluess2015). Empirical studies report substantial inter-individual differences in the degree of environmental sensitivity as a function of genetic, physiological, and psychological factors (Belsky & Pluess, Reference Belsky and Pluess2009b, Reference Belsky, Pluess, Cicchetti and Roisman2011, Reference Belsky and Pluess2013). These sensitivity factors are hypothesized to be markers of a more responsive central nervous system (CNS) (Pluess, Stevens, & Belsky, Reference Pluess, Stevens, Belsky, Legerstee, Haley and Bornstein2013). In the current study, this neurosensitivity hypothesis was tested in an exploratory analysis by investigating whether individual differences in brain structure of the amygdala and hippocampus, assessed in early adolescence, moderate the association between broad environmental quality across childhood and behavior problems in early adolescence (accounting for potential correlations between childhood environment and brain structure).
It can be widely observed that humans, like many other species (Wolf, van Doorn, & Weissing, Reference Wolf, van Doorn and Weissing2008), differ substantially in their response to various environmental exposures and experiences. Such differences have traditionally been interpreted from a perspective of diathesis–stress, with a focus on individual differences in vulnerability to adversity (Zuckerman, Reference Zuckerman1999). The diathesis–stress perspective suggests that certain people, those characterized by some kind of vulnerability (e.g., genetic or psychological traits), are more likely to develop psychological problems when experiencing adversity. However, the model does not make any predictions about differences in the response to positive experiences. Over the last decade, several theories have been put forward suggesting that people differ in their sensitivity to environmental quality, with some being generally more and some generally less sensitive (Belsky & Pluess, Reference Belsky and Pluess2009b; Boyce & Ellis, Reference Boyce and Ellis2005; Ellis, Boyce, Belsky, Bakermans-Kranenburg, & Van IJzendoorn, Reference Ellis, Boyce, Belsky, Bakermans-Kranenburg and Van IJzendoorn2011). For example, the frameworks of differential susceptibility (Belsky & Pluess, Reference Belsky and Pluess2009b), biological sensitivity to context (Boyce & Ellis, Reference Boyce and Ellis2005), and sensory processing sensitivity (Aron & Aron, Reference Aron and Aron1997) all propose that individuals with higher sensitivity are not just more reactive to negative experiences but are also more sensitive to positive environmental influences, when compared to less sensitive people. The notion that people differ in their response to positive experiences, with some benefitting disproportionately more than others, has recently been formalized more specifically in the framework of vantage sensitivity and is supported by a growing number of empirical studies (Pluess & Belsky, Reference Pluess and Belsky2013) (see Figure 1 for a graphical illustration of diathesis–stress, vantage sensitivity, and differential susceptibility).
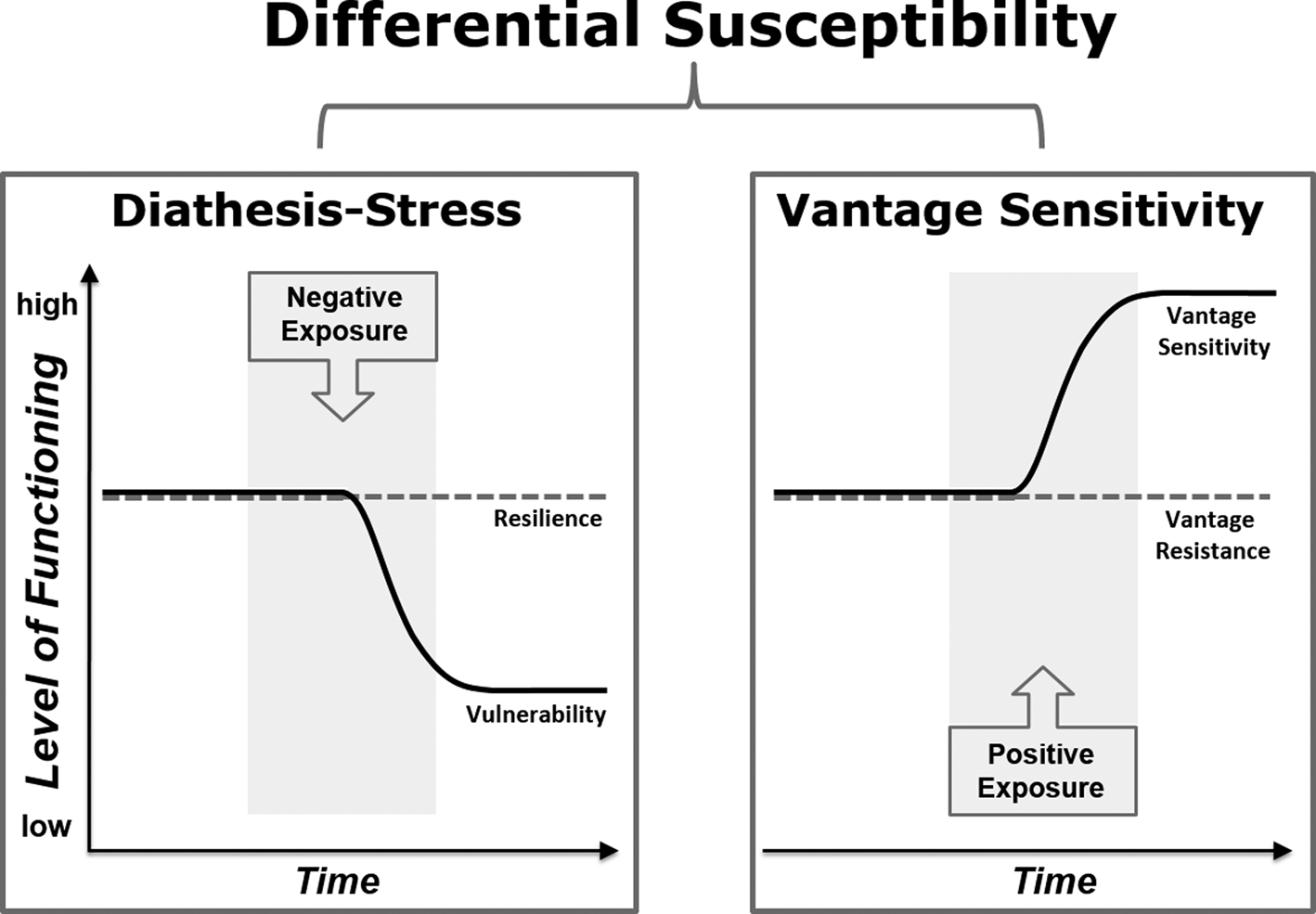
Figure 1. Illustration of three models of environmental sensitivity. Diathesis–stress describes individual differences in response to exclusively negative influences whereas vantage sensitivity refers to variability regarding positive influences only. Differential susceptibility represents the combination of diathesis–stress and vantage sensitivity with heightened sensitivity to both negative and positive experiences (based on Figure 1 in Pluess (Reference Pluess2015)).
Drawing on evolutionary considerations, both differential susceptibility (Belsky & Pluess, Reference Belsky and Pluess2009b) and biological sensitivity to context (Boyce & Ellis, Reference Boyce and Ellis2005) propose that natural selection shapes individual differences in the propensity for conditional adaptation to the environment because both low- and high- sensitive phenotypes have particular evolutionary benefits, depending on the specific quality of the developmental context (Ellis et al., Reference Ellis, Boyce, Belsky, Bakermans-Kranenburg and Van IJzendoorn2011). For example, while low-sensitive individuals tend to be more resilient in the face of adversity, they also suffer the disadvantage of being less responsive to supportive aspects of the environment. Highly sensitive individuals, by contrast, are more likely to benefit from positive environmental exposures whilst also being more vulnerable to the negative effects of adverse experiences (Pluess, Reference Pluess2015). As a consequence, the general population is made up of individuals that differ in their general environmental sensitivity, with a significant minority of about 30% characterized by particularly high sensitivity (Lionetti et al., Reference Lionetti, Aron, Aron, Burns, Jagiellowicz and Pluess2018; Pluess et al., Reference Pluess, Assary, Lionetti, Lester, Krapohl, Aron and Aron2018).
A large number of studies provide empirical evidence that some people are more sensitive than others to both negative and positive environmental influences (Belsky & Pluess, Reference Belsky, Pluess and Cicchetti2016; Slagt, Dubas, Dekovic, & van Aken, Reference Slagt, Dubas, Dekovic and van Aken2016). For example, sensitive children (i.e., those with a highly reactive temperament in infancy) have been shown to develop more behavioral problems than less sensitive children when experiencing harsh parenting but also as having fewer problems when parenting was especially sensitive (Slagt et al., Reference Slagt, Dubas, Dekovic and van Aken2016). Similarly, children carrying more sensitivity genes (based on a recently developed genome-wide polygenic sensitivity score) have been found to present with more emotional problems than genetically less sensitive children when experiencing negative parenting but also significantly fewer problems than other children when exposed to positive parenting (Keers et al., Reference Keers, Coleman, Lester, Roberts, Breen, Thastum and Meiser-Stedman2016).
Although sensitivity markers have been identified across different levels of analysis, including the genetic, physiological, and behavioral level, the exact mechanism(s) underlying individual differences in environmental sensitivity is not yet well understood. However, all leading theories in the field converge on the hypothesis that features of the CNS play a central role (Acevedo et al., Reference Acevedo, Aron, Aron, Sangster, Collins and Brown2014; Aron, Aron, & Jagiellowicz, Reference Aron, Aron and Jagiellowicz2012; Ellis et al., Reference Ellis, Boyce, Belsky, Bakermans-Kranenburg and Van IJzendoorn2011). This view is supported by the notion that environmental sensitivity requires a vast array of highly complex cognitive functions, including sensory perception, processing of sensory intake, and emotional reactivity, as well as higher order cognitive functions such as attention, memory, and executive function. Hence, the various sensitivity markers are understood to contribute to – or manifest – features of a more sensitive CNS (Aron et al., Reference Aron, Aron and Jagiellowicz2012; Ellis et al., Reference Ellis, Boyce, Belsky, Bakermans-Kranenburg and Van IJzendoorn2011; Pluess, Reference Pluess2015). While this neurosensitivity hypothesis (Pluess & Belsky, Reference Pluess and Belsky2013; Pluess et al., Reference Pluess, Stevens, Belsky, Legerstee, Haley and Bornstein2013) is rather broad and unspecific, studies reporting associations between established sensitivity markers and both structural (e.g., Holmes et al., Reference Holmes, Lee, Hollinshead, Bakst, Roffman, Smoller and Buckner2012) and functional measures of the brain (e.g., Munafo, Brown, & Hariri, Reference Munafo, Brown and Hariri2008; Schwartz, Wright, Shin, Kagan, & Rauch, Reference Schwartz, Wright, Shin, Kagan and Rauch2003) point to the amygdala and hippocampus as key regions of interest (although other brain regions and networks likely also play a role). For example, several candidate genes that have been associated with heightened sensitivity to the environment (Belsky et al., Reference Belsky, Jonassaint, Pluess, Stanton, Brummett and Williams2009; Belsky & Pluess, Reference Belsky and Pluess2009b, Reference Belsky and Pluess2013) have been linked to amygdala or hippocampus function or structure, such as genetic variation in the serotonin transporter gene (5-HTTLPR; e.g., Hariri et al., Reference Hariri, Mattay, Tessitore, Kolachana, Fera, Goldman and Weinberger2002), catechol-O-methyltransferase (COMT; e.g., Rasch et al., Reference Rasch, Spalek, Buholzer, Luechinger, Boesiger, de Quervain and Papassotiropoulos2010), and monoamine oxidase A (MAOA; e.g., Meyer-Lindenberg et al., Reference Meyer-Lindenberg, Buckholtz, Kolachana, Hariri, Pezawas, Blasi and Weinberger2006). Similarly, behavioral markers of sensitivity, including high reactivity in infancy (Schwartz et al., Reference Schwartz, Kunwar, Greve, Kagan, Snidman and Bloch2012), inhibited temperament at age 5 years (Hill, Tessner, Wang, Carter, & McDermott, Reference Hill, Tessner, Wang, Carter and McDermott2010), and behavioral inhibition in adults (Barros-Loscertales et al., Reference Barros-Loscertales, Meseguer, Sanjuan, Belloch, Parcet, Torrubia and Avila2006), have been associated with amygdala function and structure.
In the current study we focused on structural (rather than functional) differences in these two regions, given that a small number of studies have provided first evidence that structural differences in these brain regions moderate the effects of environmental influences consistent with the predictions of environmental sensitivity frameworks. For example, one cross-sectional study featuring a sample of 106 11- to 14-year-old adolescents and their mothers was aimed at investigating whether structural differences in the amygdala, hippocampus, and anterior cingulate cortex moderated the relationship between observationally assessed maternal aggression and adolescents' depression symptoms (Yap et al., Reference Yap, Whittle, Yucel, Sheeber, Pantelis, Simmons and Allen2008). Several statistically significant three-way interactions between maternal aggression, brain structure, and gender emerged. Most relevant for the current study, girls with smaller (bilateral) amygdala volumes were more sensitive to maternal aggression, for better and for worse. Compared with girls with larger amygdala, they had the lowest depression scores when maternal aggression was low and the highest depression scores when mothers were more aggressive. However, in boys, a larger right amygdala was associated with reduced depression symptoms when maternal aggression was low (i.e., reflecting vantage sensitivity), with no evidence for heightened vulnerability to high maternal aggression. In a follow-up study of the same sample, the authors tested whether individual differences in hippocampal volume moderated change in depression scores over time (Whittle et al., Reference Whittle, Yap, Sheeber, Dudgeon, Yuecel, Pantelis and Allen2011). Again, a three-way interaction emerged, but this time suggesting that larger (bilateral) hippocampal volume predicted sensitivity to maternal aggression in the prediction of depression – but only in girls. Those with larger hippocampal volumes had the lowest depression scores when maternal aggressive behavior was low, and the highest depression scores when maternal aggressive behavior was high. Conversely, depression symptoms in girls with smaller hippocampal volumes were less affected by maternal aggression. More recently, a longitudinal study involving 209 17-year-old adolescents tested whether hippocampal volume moderated the effects of family connectedness and community crime on depression, anxiety, and externalizing symptoms (Schriber et al., Reference Schriber, Anbari, Robins, Conger, Hastings and Guyer2017). The effects of both environmental measures on depression were moderated by the volume of the left hippocampus across both genders. According to follow-up analyses, adolescents with a larger left hippocampus had higher depression scores when family connectedness was low and when community crime was high, consistent with diathesis–stress.
In summary, these three studies (Schriber et al., Reference Schriber, Anbari, Robins, Conger, Hastings and Guyer2017; Whittle et al., Reference Whittle, Yap, Sheeber, Dudgeon, Yuecel, Pantelis and Allen2011; Yap et al., Reference Yap, Whittle, Yucel, Sheeber, Pantelis, Simmons and Allen2008) provide important first evidence that individual differences in environmental sensitivity are associated with differences in amygdalar and hippocampal volumes. However, this pioneering research was primarily informed by a pathology framework focused on vulnerability to the negative effects of specific adverse experiences. Consequently, most of the studies did not consider whether brain structure also predicted individual differences at the positive end of the environmental quality spectrum. Furthermore, outcome measures were exclusively based on self-reports and significant interactions were not followed up with the statistical procedures required to explore whether detected interaction patterns are more consistent with diathesis–stress, differential susceptibility, or vantage sensitivity (Roisman et al., Reference Roisman, Newman, Fraley, Haltigan, Groh and Haydon2012).
The current analysis builds on the above-mentioned studies, but rather than focusing on specific childhood experiences it features a more general and cumulative measure of broad environmental quality across childhood, ranging from low (i.e., negative) to high (i.e., positive). Such a broad and inclusive measure of environmental quality may be more suitable when investigating individual differences in general environmental sensitivity, as was the case in the study reported here. In addition, the study examined the pattern of significant interactions to test whether interactions are more supportive of diathesis–stress, differential susceptibility, or vantage sensitivity. Importantly, the study was exploratory in nature given that it was based on data from a relatively small number of children, initially recruited for a study with different aims, and including only boys. The main objective of this proof of concept study was to investigate whether differences in amygdalar and hippocampal volumes, assessed in early adolescence, moderate the effects of a cumulative score of broad early environmental quality on teacher-reported child behavior (i.e., an index of emotional and behavioral problems as well as prosocial skills) in early adolescence.
Method
Participants
Rather than recruiting new participants, we relied on a data set that was already available to us. It is important to note that the available sample did not include any girls and was made up of male twins. Data were obtained from 62 boys originally included in the Twins Early Development Study (TEDS), a large longitudinal study of over 16,000 twin pairs born in England and Wales in 1994, 1995, and 1996. TEDS includes extensive data on various aspects of development, collected at regular intervals from a sample that is representative of the UK population (Kovas et al., Reference Kovas, Haworth, Dale, Plomin, Weinberg, Thomson and Fischer2007). General data and recruitment procedures for TEDS are reported in detail elsewhere (Haworth, Davis, & Plomin, Reference Haworth, Davis and Plomin2013). The participants of the current study were a subset of boys that were initially recruited from the TEDS database for an associated twin neuroimaging project on precursors of psychopathic traits led by some of the authors of the current study (Rijsdijk et al., Reference Rijsdijk, Viding, De Brito, Forgiarini, Mechelli, Jones and McCrory2010).
Parents completed questionnaires about magnetic resonance imaging (MRI) contraindicators and provided consent to be contacted regarding study participation. After describing the study to the children and their parents, written informed consent was obtained from the parents and oral assent from the boys. The study and recruitment procedure were approved by the Institute of Psychiatry and the South London and Maudsley NHS Foundation Trust Research Ethics Committee. For the current analysis, only boys with complete data on all measures and very good quality imaging data (i.e., no blurring by motion) were included, resulting in a total sample of N = 62 boys with a mean age of 11.61 years (SD = 0.81) at the time of imaging (see Table 1 for a detailed description of the sample). The participating boys were aged 10–13 years, had no psychiatric, neurological, or medical problems, and a full-scale IQ of at least 80. Premature and low-birthweight children were excluded from analyses. All boys included in the current analysis were from same-sex twin pairs (20 monozygotic (MZ) and 42 dizygotic (DZ) twin siblings). Relatedness between boys from the same twin pair (the sample included two complete MZ twin pairs and 16 complete DZ twin pairs) was statistically controlled for by nesting related siblings in hierarchical linear models. Compared with the full TEDS sample, the boys included in the current study were significantly older when behavioral problems were rated by teachers (M = 12.09 and SD = 0.29 for included cases vs. M = 11.53 and SD = 0.66 for the remaining TEDS sample, with t = −14.78, p < .01). Furthermore, total problem scores were slightly higher in this subsample of boys compared with the complete TEDS sample (M = 6.50 and SD = 5.57 for included cases vs. M = 5.13 and SD = 5.16 for other TEDS cases, with t = −2.08, p = .04). This can be explained by the fact that a proportion of the sample recruited for the original neuroimaging project on psychopathic traits was selected based on elevated conduct problems and callous–unemotional scores (i.e., those scoring in the top 10% on these measures) when they were 9 years old. Importantly, 42 of the boys included in the current analysis represented control cases with normal levels of behavioral problems (i.e., the sample did not reflect high risk for psychopathology). The control cases had significantly lower behavioral problems (M = 5.21 and SD = 4.9) than those selected for elevated problems (M = 9.04 and SD = 6.04, with t = 2.69, p = .01). However, the boys with elevated problems did not differ from the controls regarding amygdala or hippocampus volumes or any other variable included in the analysis. Furthermore, the cumulative environmental quality score of the subsample did not differ from the total sample (M = 13.88 and SD = 1.67 for included cases vs. M = 13.69 and SD = 1.89 for other TEDS participants, with t = −0.80, p = .42). According to chi-square tests, there were no significant differences in ethnicity and income between the current sample and the remaining TEDS sample.
Table 1. Demographic characteristics of sample (N = 62)

Note: 1Higher scores reflect higher quality of the environment. The environmental quality score ranged from 8 = lowest quality to 18 = highest quality.
MRI acquisition
Structural brain images were acquired using a General Electric Signa 3.0 Telsa Excite II MRI scanner (GE Medical Systems, Milwaukee, WI, USA) at the Centre for Neuroimaging Science, Institute of Psychiatry, London, UK. A high-resolution, three-dimensional T1-weighted data set was acquired using an inversion recovery prepared spoiled gradient echo (IR-SPGR) sequence. The imaging parameters were TR (repetition time) = 8 ms, TE (echo time) = 2.9 ms, TI (inversion time) = 450 ms, and excitation flip angle = 20°. The in-plane matrix size was 256 × 192 over a 280 × 210 mm field of view, reconstructed to 256 × 256 over 280 × 280 mm. The in-plane pixel size was thus 1.094 × 1.094 mm. 200 through-plane partitions (each 1.1 mm thick) were collected, with two partitions discarded at each end of the imaging volume to minimize wrap-round artefacts. Partial k-space coverage (“0.75 NEX”) was used. The scanning time was 6 min.
MRI data preprocessing
To quantify and extract the gray matter volume from the amygdala and the hippocampus, we used voxel-based morphometry (VBM), which is a widely employed automated MRI analysis technique (Whitwell, Reference Whitwell2009). Specifically, the data were preprocessed using the VBM8 toolbox and SPM8, which provide improved segmentation and registration procedures such as the Diffeomorphic Anatomical Registration Through Exponentiated Lie Algebra (DARTEL) toolbox (Ashburner, Reference Ashburner2007). Given that our sample included children aged 10–13 years, customized tissue probability maps were created at the Montreal Neurological Institute (MNI) space for use with the VBM8 toolbox. These were produced using the matched template approach of the Template-O-Matic toolbox for SPM8 with each participant's age and sex as defining variables (Wilke, Holland, Altaye, & Gaser, Reference Wilke, Holland, Altaye and Gaser2008). The preprocessing included the following steps. The anterior commissure was first manually indicated on all structural images as the [0, 0, 0 mm] origin in the MNI spatial coordinate system. Individual images were then corrected for bias-field inhomogeneities, segmented, and spatially normalized (affine-only transformation) with reference to customized tissue probability maps. Segmentation accuracy was visually checked for each participant. Based on individual registered gray matter and white matter segmentations, an average DARTEL template of all participants was created in MNI space (Ashburner, Reference Ashburner2007). The affine-registered gray matter and white matter segments were then warped to this average template using the high-dimensional DARTEL approach. Importantly, in order to account for individual differences in the brain size modulated data produced, gray matter volume and the voxel values in the gray matter segments were only multiplied by the nonlinear component of the registration. Given that the two structures we focused on are well defined and adjacent to each other, we did not smooth the data. For each participant, the MarsBaR region of interest toolbox (Brett et al., Reference Brett, Anton, Valabregue and Poline2002; Tzourio-Mazoyer et al., Reference Tzourio-Mazoyer, Landeau, Papathanassiou, Crivello, Etard, Delcroix and Joliot2002), as implemented in SPM8, was used to extract the mean gray matter volume value for the amygdala and the hippocampus, bilaterally, using anatomical masks from the automated anatomical labeling (AAL) atlas (Figure 2).

Figure 2. Graphic illustration of the selected brain regions used to generate the amygdala and hippocampus volumes. Left and right amygdala are colored red whereas left and right hippocampus are colored blue.
Measures
Environmental quality
In order to obtain a measure of general and broad environmental quality across childhood, a new scale for cumulative environmental quality across years 1–9 was created by recoding and summing up existing parent-reported variables and scales reflecting both negative and positive aspects of the social and material developmental context. All the included variables reflect normative aspects of children's environments rather than unusual experiences such as severe adversity. The subscale “social environment” covered six parent-reported psychosocial aspects (i.e., parental feelings, parental discipline, family order and chaos, partner presence, parental reading to child, and parent outings with child – all assessed at age 3 years and then again at 9 years). The subscale “material environment” was based on six parent-reported socioeconomic characteristics of the environment (i.e., socioeconomic status composite scores at first contact and at age 7 years, number of books at age 3 years, financial changes, parental unemployment, and household income at age 9 years). After recoding all individual variables to fit a categorical scale ( 1 = low quality, 2 = medium quality, and 3 = high quality) items were summed up separately for each of the subscales (see Supplementary Material for details). Importantly, according to preliminary analyses using the complete TEDS sample, the recoded items were all significantly and negatively associated with child total problems (following the Strengths and Difficulties Questionnaire (SDQ)) rated by parents (i.e., the higher the quality, the lower the problems). The separate sum scores for the social environment at ages 3 and 9 years were averaged to compute a social environment subscale that reflects environmental quality across childhood. In order to create the final environmental quality score, the social environment subscale score was then averaged with the material environment subscale score. This resulted in a total score with a theoretical range of 8–18, with 8 = lowest quality and 18 = highest quality.
Child behavior
The SDQ, a well-established 25-item questionnaire with five subscales (Goodman, Reference Goodman1997), was completed by teachers when the children were 12–13 years old. Items were rated on a Likert scale (0 = not true, 1 = somewhat true, 2 = certainly true). The five subscales (i.e., emotional symptoms, conduct problems, hyperactivity/inattention, peer relationship problems, and prosocial behavior) can be used individually (each ranging from 0 to 10) but the four problem subscales can also be combined to yield a total problem score (with a range of 0–40). For the current analysis we focused on the total problem score, which included the subscales emotional symptoms, conduct problems, hyperactivity/inattention, and peer relationship problems. In a second step we also conducted a set of post-hoc analyses for each individual subscale of the SDQ (see Supplementary Material).
Statistical analysis
Interactions between brain volumes and environmental quality predicting total behavior problems were tested with hierarchical linear models. Relatedness between siblings was controlled for by including the family level as a random effect (i.e., data of related siblings were nested in families). Models were kept as parsimonious as possible given the small sample size and tested for the main effects of the environmental quality score and continuous brain volumes as well as the interaction between both. Given we had no hypothesis to assume sensitivity would be different as a function of zygosity (according to bivariate correlations zygosity was unrelated to all included variables, p > .05), we did not control for MZ or DZ status. Importantly, all brain volume measures were corrected for total brain size (modulation with nonlinear registration; see the section on MRI data preprocessing) and age at the time of imaging was not significantly correlated with any of the volumes (r = −.10 to .12 with p = .25 to .90). Separate models were run for the four different brain regions (i.e., left and right amygdala, left and right hippocampus). In order to follow up significant interactions, the continuous brain volume variable was divided into low and high volume at the median to create and compare simple slopes. Finally, regions of significance analyses were conducted in order to examine whether detected interactions were more supportive of diathesis–stress, vantage sensitivity, or differential susceptibility patterns (Preacher, Curran, & Bauer, Reference Preacher, Curran and Bauer2006). In addition, significant interactions were further investigated in post-hoc analyses that considered the five different subscales of the SDQ separately in relation to environmental quality, but also the subscales of social environment and material environment. The level of significance was set at α = .05 for all tests but Bonferroni correction that accounts for the correlation between the brain volumes (on average r = .58) was applied to correct for multiple testing in relation to the four tested multilevel models. All statistical analyses were conducted in SPSS (version 22).
Results
Bivariate correlations
According to bivariate correlations, all four brain volumes were significantly associated with each other (see Table 2). Importantly, brain volumes were not associated with the environmental quality score (r = −.13 to .08, p > .05) or the total problems outcome variable (r = −.21 to .12, p > .05). Although higher environmental quality was associated with fewer problems, this association did not reach statistical significance (r = −.14, p > .05).
Table 2. Unadjusted associations between variables (N = 62)

Note: 1Higher scores reflect higher quality of the environment. *p < .05. **p < .01.
Hierarchical linear models
Interactions between continuous brain volumes and the environmental score were tested with separate hierarchical linear models with biologically related boys nested in families to account for relatedness (i.e., level 1) and controlling for main effects of the cumulative environmental quality score and brain volumes. A significant interaction emerged between left amygdala volume and environmental quality in the prediction of total problems (B = −16.10, p = .01). None of the other brain volumes significantly moderated effects of the cumulative environmental score (i.e., interaction terms were B = −12.32, p = .24 for right amygdala, B = 3.48, p = .11 for left hippocampus, and B = −11.73, p = .36 for right hippocampus volumes). Importantly, the significant interaction between left amygdala volume and environmental quality survived Bonferroni correction for multiple testing (corrected α = .028 taking correlations between the four tested brain volumes into account). The hierarchical linear model results are displayed in Table 3.
Table 3. Summary of hierarchical linear models predicting total problems (N = 62)

Note: The displayed coefficients of variables at step 1 represent the values before inclusion of interaction term at step 2. AL = Amygdala left; AR = Amygdala right; HL = Hippocampus left; HR = Hippocampus right; *p < .05. **p < .01.
Follow-up analyses
The significant interaction was followed up in order to interpret the nature of the interaction through simple slopes by dividing the sample by median split into two groups – low and high left amygdala volume. In addition to testing simple slopes between environmental score and outcomes for the low and high left amygdala volume groups, we also conducted regions of significance analyses in order to examine the specific pattern of the interaction (Preacher et al., Reference Preacher, Curran and Bauer2006; Roisman et al., Reference Roisman, Newman, Fraley, Haltigan, Groh and Haydon2012). According to simple slopes and regions of significance analysis, larger left amygdala volume was associated with higher sensitivity to higher quality of the environment. While the lower bound of the regions of significance analysis was outside of the observed data, the upper bound was within the upper range, suggesting that the association between larger left amygdala volume and fewer behavioral outcomes reached statistical significance only toward the higher end of environmental quality. More clearly, whereas environmental quality was not associated with total problems in boys with a small amygdala (β = .16, p = .39), higher environmental quality was associated with fewer problems in those with a large amygdala (β = −.44, p = .01), with differences between groups being significant above a threshold of 13.79 on the cumulative environmental quality scale (see Figure 3).

Figure 3. Simple slopes and scatter plot of the significant interaction between left amygdala and environmental quality predicting total behavior problems. The hierarchical linear models were run with continuous brain volumes; for the follow-up analysis the sample was divided by medium split into small amygdala (triangles in scatter plot) and large amygdala (dots in scatter plot) (some of the triangles and dots are overlapping). Shaded areas reflect regions of significance. In these regions the association between amygdala left volume and behavior problems is significant.
Post-hoc analyses
In a series of exploratory post-hoc analyses (not controlling for multiple testing), the hierarchical linear models involving amygdala left volume were rerun separately for the environmental quality score as well as the social environment and material environment subscales predicting all five subscales of the SDQ. Six additional significant interactions emerged: amygdala left volume moderated the effects of the cumulative environmental score on peer problems (B = −6.05, p = .01) and conduct problems (B = −3.73, p = .02), the effects of the social environment subscale on emotional symptoms (B = −3.65, p = .03) and peer problems (B = −4.41, p = .02), and the effects of the material environment subscale on prosocial behavior (B = 3.18, p = .05) and conduct problems (B = −2.43, p = .02). The majority of these interactions yielded similar simple slope patterns, with larger left amygdala volume reflecting higher sensitivity to higher quality of the early environment. Importantly, interaction effects were not limited to problem behaviors but left amygdala volume also moderated the positive effect of environmental quality on prosocial behavior, a positive outcome, with larger left amygdala being associated with greater prosocial behavior when material environment was particularly high (see Supplementary Material for more information).
Discussion
This exploratory proof of concept study aimed to test whether differences in amygdala and hippocampus volumes moderate the relationship between broad cumulative environmental quality, ranging from low to high, across early childhood and teacher-reported behavioral problems as well as prosocial behavior in early adolescence. Significant interactions were followed up in order to examine whether the detected sensitivity patterns were more consistent with diathesis–stress (Zuckerman, Reference Zuckerman1999), differential susceptibility (Belsky & Pluess, Reference Belsky and Pluess2009b), or vantage sensitivity (Pluess & Belsky, Reference Pluess and Belsky2013).
In general, the results support a vantage sensitivity pattern for boys with larger amygdala volumes. In more detail, our findings indicate that left amygdala volume moderates associations between early environmental quality and teacher-reported total problems in early adolescence. In boys with a larger left amygdala, there was a significant association between higher quality of the environment and lower total problems. This association was not statistically significant in boys with smaller amygdala volumes. The finding that larger left amygdala size in boys reflects higher sensitivity to higher environmental quality is consistent with a previous study with different predictor and outcome measures, which reported that boys with larger amygdala had fewer depression symptoms when maternal aggression was low (Yap et al., Reference Yap, Whittle, Yucel, Sheeber, Pantelis, Simmons and Allen2008). Interestingly, several studies investigating the moderating effects of hippocampal volumes (Whittle et al., Reference Whittle, Yap, Sheeber, Dudgeon, Yuecel, Pantelis and Allen2011; Yap et al., Reference Yap, Whittle, Yucel, Sheeber, Pantelis, Simmons and Allen2008) detected such effects in girls but failed to do so in boys. This appears to be somewhat consistent with observations of the current study that hippocampal volumes did not moderate associations between child behavior and the environment in boys (but one study found that hippocampal volume moderated the effects of the social environment across both genders (Schriber et al., Reference Schriber, Anbari, Robins, Conger, Hastings and Guyer2017)). Further studies are required to investigate the potential existence of sex and gender differences regarding associations between brain structure and environmental sensitivity. This is important given well-known sex-specific differences in brain structure, including the amygdala, which tends to be generally larger in males (Ruigrok et al., Reference Ruigrok, Salimi-Khorshidi, Lai, Baron-Cohen, Lombardo, Tait and Suckling2014).
Furthermore, according to post-hoc analyses, the moderating effect of left amygdala volume was not only restricted to associations between higher environmental quality and lower maladaptive outcomes (such as peer problems and emotional problems), but also to higher levels of an adaptive outcome (prosocial behavior). This further corroborates the notion that individuals may differ in their general sensitivity to environmental influences rather than exclusively in their vulnerability to develop problem behaviors when exposed to harsh environments (Belsky & Pluess, Reference Belsky and Pluess2009b). The finding that larger amygdala volume in boys growing up in supportive environments was not only associated with fewer problem behaviors but also with more prosocial behavior is consistent with human and animal studies pointing to associations between amygdala volume and social behavior (Bickart, Wright, Dautoff, Dickerson, & Barrett, Reference Bickart, Wright, Dautoff, Dickerson and Barrett2011; Dunbar, Reference Dunbar2012). Interestingly, the follow-up analyses suggest that the significant interaction between amygdala left volume and environmental quality reflects a vantage sensitivity (Pluess & Belsky, Reference Pluess and Belsky2013) rather than a diathesis–stress or differential susceptibility pattern. In other words, boys with larger amygdala volumes were particularly sensitive to higher environmental quality in that they presented with fewer problems (and also with more prosocial behaviors) than boys with smaller amygdala volumes when raised in more supportive and more affluent families. In lower quality environments, left amygdala volume was not associated with behavior problems (or prosocial behavior). This is similar to a previous study in which larger amygdala volume of boys predicted vantage sensitivity to low maternal aggression but not vulnerability to high maternal aggression (Yap et al., Reference Yap, Whittle, Yucel, Sheeber, Pantelis, Simmons and Allen2008). Consequently, this suggests that larger amygdala volume may be an advantage in more supportive and less threatening environments while not necessarily increasing vulnerability to adversity – at least in boys that grow up in relatively mild adversity. Future research will have to investigate whether the current findings replicate in samples of more deprived backgrounds than the one included in the current study.
Our findings further suggest that structural differences in the amygdala of boys predict the sensitivity to broad and cumulative environmental quality in childhood. Although it is important to identify the specific environmental factors that shape the development of more sensitive children at specific times, combining a large number of environmental aspects across childhood into general and cumulative scores that range from low to high quality and cover various aspects of the normative developmental context appears to be a promising approach when investigating individual differences in more general environmental sensitivity. Future research studies may want to adopt this novel approach in order consider sensitivity across a broader spectrum of environmental quality.
The amygdala has been hypothesized as a key region of interest for environmental sensitivity (Pluess, Reference Pluess2015; Pluess & Belsky, Reference Pluess and Belsky2013; Pluess et al., Reference Pluess, Stevens, Belsky, Legerstee, Haley and Bornstein2013) due to associations with various established sensitivity markers. According to empirical studies, larger amygdala volume predicts heightened sensitivity to punishment (Barros-Loscertales et al., Reference Barros-Loscertales, Meseguer, Sanjuan, Belloch, Parcet, Torrubia and Avila2006) as well as negative affect (Holmes et al., Reference Holmes, Lee, Hollinshead, Bakst, Roffman, Smoller and Buckner2012). Importantly, meta-analytic evidence provides strong support for the notion that the amygdala responds to both negative and positive stimuli (Sergerie, Chochol, & Armony, Reference Sergerie, Chochol and Armony2008). Our research provides further evidence for the amygdala as a brain region of particular relevance for environmental sensitivity. Although our findings need to be replicated before investigating the specific biological mechanisms underlying the detected associations between amygdala structure and sensitivity in more detail (including potential laterality effects), we suggest that there are good reasons beyond the ones already mentioned why the amygdala might be relevant for sensitivity to the environment. For example, the amygdala's general role in emotional reactivity and response to both negative and positive stimuli (Sergerie et al., Reference Sergerie, Chochol and Armony2008) may explain why boys with a larger amygdala are more positively affected by higher environmental quality. Furthermore, one of the primary functions of amygdala processing is to signal what is important in any particular situation (i.e., salience processing) and then modulate the appropriate perceptual, attentional, autonomic, and cognitive/conceptual processes to deal with the challenges or opportunities that are present (Cunningham, Arbuckle, Jahn, Mowrer, & Abduljalil, Reference Cunningham, Arbuckle, Jahn, Mowrer and Abduljalil2010). Children whose brains are more able to do so (e.g., by having a larger amygdala) might adjust better to the conditions of their specific developmental context, such as showing fewer behavior problems and being more prosocial in a supportive environment. Importantly, amygdala volume itself has been shown to be associated with environmental conditions in early development such as maternal cortisol during pregnancy (Buss et al., Reference Buss, Davis, Shahbaba, Pruessner, Head and Sandman2012). In light of studies reporting that prenatal and early postnatal factors influence the development of environmental sensitivity (Hartman, Freeman, Bales, & Belsky, Reference Hartman, Freeman, Bales and Belsky2018; Pluess & Belsky, Reference Pluess and Belsky2011), future studies should also investigate how conditions of the early environment shape characteristics of the brain that are associated with sensitivity to the environment. Moreover, future research should also investigate the role of amygdala function (e.g., Gard, Shaw, Forbes, & Hyde, Reference Gard, Shaw, Forbes and Hyde2018), taking into account recently identified methodological challenges for the use of functional data when researching individual differences (Elliott et al., Reference Elliott, Knodt, Ireland, Morris, Poulton, Ramrakha and Hariri2020).
The current study has several important strengths, including prospective longitudinal data, the use of an innovative cumulative environmental score based on objective or parent-reported measures that range across a broad spectrum of environmental quality, as well as teacher-reported outcomes. However, the findings have to be considered in the light of several limitations.
First, the available sample was small and included only twin boys, a third of which had elevated conduct problems and/or callous–unemotional behavior scores at age 9 years. Future studies should feature larger samples with equal numbers of (non-twin) boys and girls from a general nonclinical population that represents individuals from both low and high socioeconomic backgrounds (in order to test whether findings are similar in both low- and high-risk contexts). In addition, future studies should also investigate whether the findings reported here in early adolescence extend to other developmental periods across the life span.
Second, the sample included some siblings from the same twin pairs. Such siblings tend to differ less from each other due to shared genetic and environmental factors (however, we statistically controlled for relatedness by testing hypotheses in a multilevel model and including family as one level within which related individuals were nested).
Third, brain structure was assessed after the environmental exposure occurred and only shortly before teachers rated children's behavior. Ideally, the imaging data would have been obtained before the exposure to exclude the possibility that the brain structure itself had been influenced by environmental quality (Evans et al., Reference Evans, Swain, King, Wang, Javanbakht, Ho and Liberzon2016). Furthermore, although environmental quality was not associated with any of the brain variables according to bivariate correlations, and we also statistically accounted for main effects of brain structure in the model, we cannot exclude the possibility that other environmental factors in early childhood, not assessed with the current measure, contributed to differences in amygdala and hippocampus volumes.
Fourth, while we used an innovative cumulative environmental quality score in order to capture the general quality of early childhood, this particular score or approach has not yet been validated through replication in other samples. In light of our findings, future studies may want to focus more specifically on measures of environmental enrichment (e.g., frequency of attending museums, shows, exhibitions, after-school activities etc.).
Fifth, the study did not include important covariates such as gestational age at birth and other pre- and perinatal factors that might influence brain structure (e.g., maternal smoking). Future studies should consider such covariates.
Sixth, the current study did not include any explicit measures of sensitivity, such as the Highly Sensitive Child Scale (Pluess et al., Reference Pluess, Assary, Lionetti, Lester, Krapohl, Aron and Aron2018). Future studies should investigate whether validated psychological measures of sensitivity correlate with brain structures found to moderate environmental quality.
Finally, the current study only considered amygdala and hippocampus, but it is very likely that other brain regions and networks are also relevant (Acevedo et al., Reference Acevedo, Aron, Aron, Sangster, Collins and Brown2014; Moore & Depue, Reference Moore and Depue2016), which should be considered in future studies on sensitivity.
In conclusion, this exploratory study provides novel but preliminary empirical evidence for heightened sensitivity to positive environmental influences for boys in early childhood as a function of structural brain differences in the left amygdala (but not hippocampus) and supports the hypothesis that individual differences in environmental sensitivity are associated with neurological factors. However, we recommend that our findings be replicated in larger samples of both boys and girls (from both high and low socioeconomic backgrounds), considering a broader range of behavioral outcomes, and with brain structure measured before the environmental exposure.
Supplementary Material
The Supplementary Material for this article can be found at https://doi.org/10.1017/S0954579420001698.
Acknowledgement
We gratefully acknowledge the ongoing contribution of the participants in the Twins Early Development Study (TEDS) and their families.
Financial Statement
TEDS is supported by a program grant to Robert Plomin from the UK Medical Research Council (MR/M021475/1 and previously G0901245), with additional support from the US National Institutes of Health (HD044454, HD059215 and AG046903) and the European Commission (602768). Collection of the neuroimaging data reported here was supported by grant G0401170 from the Medical Research Council and grant MRD 12-73 from the UK Department of Health Forensic Mental Health Programme awarded to Essi Viding. Stephane De Brito was supported by an advanced research fellowship from the Swiss National Science Foundation (FNS PA00P1_139586).
Conflicts of Interest
None.