Introduction
The demand for organic produce in the United States is continuously rising due to increased health concerns and an increased number of health-conscious consumers (Saini and Singh Reference Saini and Singh2019). Moreover, there are increasing concerns about the harmful environmental impacts of intensive use of chemicals in conventional vegetable production (Saini and Singh Reference Saini and Singh2019). From 2015 to 2016, the number of certified organic hectares increased by 15% and the total area under certified organic production was 2 million hectares (USDA-NASS 2017). Of the 2 million hectares, 75,000 hectares are used to grow vegetable crops (USDA-NASS 2017). Although organic vegetable production still needs to increase in order to meet consumer demand, various factors constrain conventional growers from converting to organic production. Weeds are one of the major biotic factors that pose a challenge to efficient and profitable organic crop production (Saini and Singh Reference Saini and Singh2019; Webber et al. Reference Webber, Shrefler and Brandenberger2012). Surveys of organic crop producers and studies on organic crop production in many countries have revealed that weeds are a major constraint to successful organic crop production (Brainard et al. Reference Brainard, Curran, Bellinder, Ngouajio, VanGessel, Haar, Lanini and Masiunas2013; Johnson and Luo Reference Johnson and Luo2018; Kristiansen Reference Kristiansen2003; Tworkoski Reference Tworkoski2002). Many vegetable crops are weak competitors with weeds due to their shallow root systems and slow growth rates, especially during the early growth stages (Mennan et al. Reference Mennan, Jabran, Zandstra and Pala2020). Uncontrolled weeds in vegetable crop production can reduce a crop’s yield by 45% to 95% depending upon the growing conditions (Al-Khatib et al. Reference Al-Khatib, Kadir and Libbey1995; Aliyu and Lagoke Reference Aliyu and Lagoke1995; Mennan et al. Reference Mennan, Jabran, Zandstra and Pala2020; Shrefler et al. Reference Shrefler, Brandenberger, Webber, Roberts, Payton and Wells2007; Walters and Young Reference Walters and Young2010). Weeds cause economic losses in vegetable production not only due to reduced yield but also due to the reduced quality and market value of the produce (Brown et al. Reference Brown, Hoshide and Gallandt2019).
Weeds compete with the crops for nutrients, water, light, and space. Competition leads to decreased water uptake by crop, which leads to decreased growth and yield, which ultimately results in lower water use efficiency (WUE; Massinga et al. Reference Massinga, Currie and Trooien2003). Efficient water use is of prime importance particularly in water-limited arid and semiarid regions such as Southern High Plains (SHP) of the United States. In most parts of the SHP, the water table has substantially decreased (by 12 m from 1935 to 2012) due to high water extraction for agricultural production (Haacker et al. Reference Haacker, Kendall and Hyndman2016). Therefore, improvement in WUE of crops through effective weed control practices is imperative to efficiently utilize limited water resources in the SHP.
Weed control practices includes 1) mulching with biodegradable (crop residues), plastic, or synthetic materials; 2) mowing; 3) livestock grazing; 4) hand weeding; 5) mechanical cultivation; and 6) cover crops (Penn State Entension 2004; Webber et al. Reference Webber, Shrefler and Brandenberger2012). Hand-hoeing is an expensive and time-consuming method. Moreover, labor shortages also pose a challenge to the adoption of the hand-hoeing for weed control (Kristiansen Reference Kristiansen2003). Until now, the adoption of cover crops for weed control in vegetable crop production has been limited by biological, farm operational, and economic factors (Saini and Singh Reference Saini and Singh2019). Excessive tillage can reduce the soil organic matter and water holding capacity of the soil, and increase nutrient loss and soil erosion (Webber et al. Reference Webber, Shrefler and Brandenberger2012). However, herbicides with biological or botanical substances or soap-based herbicides can be a viable option for organic weed control and could be used when cultural practices fail to provide adequate weed control in organic vegetable production.
Agricultural by-products such as corn gluten meal (CGM), mustard seed meal (MSM), and soybean meal have proved to be effective preemergence weed control options (Saini and Singh Reference Saini and Singh2018; Webber et al. Reference Webber, Shrefler, Brandenberger, Taylor, Carrier and Shannon2010b). These by-products release allelochemicals that suppress weeds (Saini et al. Reference Saini, Singh and Deb2020), but on other hand, they also enhance the growth of escaped weeds by providing nitrogen contained in them. However, postemergence weed control measures are also necessary to control escaped weeds, established perennial weeds, or weeds emerging when the effectiveness of agricultural by-products (CGM and MSM) declines (Webber et al. Reference Webber, Shrefler, Brandenberger, Taylor, Carrier and Shannon2010b). Therefore, more research is needed to identify essential oil and/or soap based postemergence weed control measures for better overall weed control.
Clove oil is an essential oil that has been used as a nonselective contact organic herbicide (Boyd and Brennan Reference Boyd and Brennan2006). Several studies have been conducted to evaluate the efficacy of clove oil for controlling weeds (Abouziena et al. Reference Abouziena, Omar, Sharma and Singh2009; Boyd and Brennan Reference Boyd and Brennan2006; Brainard et al. Reference Brainard, Curran, Bellinder, Ngouajio, VanGessel, Haar, Lanini and Masiunas2013; Evans et al. Reference Evans, Bellinder and Goffinet2009; Tworkoski Reference Tworkoski2002) but results have been variable. Brainard et al. (Reference Brainard, Curran, Bellinder, Ngouajio, VanGessel, Haar, Lanini and Masiunas2013) reported that the weed control efficacy of clove oil was affected by the application rate and environmental conditions. Cinnamon oil is another essential oil that has promising herbicidal activity because it provides nearly complete weed control at concentrations of 5% to 10% (Campiglia et al. Reference Campiglia, Mancinelli, Cavalieri and Caporali2007; Cavalieri and Caporali Reference Cavalieri and Caporali2010; Tworkoski Reference Tworkoski2002). Cinnamon oil has a strong inhibitory effect on seed germination. A study by Cavalieri and Caporali (Reference Cavalieri and Caporali2010) reported that in a germination chamber, total inhibition of weed seed germination was achieved by using 1.8 and 5.4 mg/L of cinnamon oil, whereas in a greenhouse study, total inhibition of weed seed germination was achieved by using 345.6 mg/L of cinnamon oil. The effectiveness of cinnamon oil varied with different environmental conditions.
Ammonium nonanoate is a synthetic and nonselective contact herbicide used for weed control. Products with this active ingredient are currently labeled for weed control in nonfood crops and it is under evaluation for registration as organic herbicide for controlling weeds in food crops (Webber et al. Reference Webber, Shrefler, Brandenberger, Taylor, Carrier and Shannon2010b). Chemically, ammonium nonanoate consists of a form of ammoniated pelargonic salts (Johnson and Luo Reference Johnson and Luo2018). Ammonium nonanoate is a naturally occurring soap and has short persistence (less than 24 h) in the environment (Webber et al. Reference Webber, Shrefler, Brandenberger, Taylor, Carrier and Shannon2010b). In a study by Webber et al. (Reference Webber, Shrefler, Brandenberger, Taylor, Carrier and Shannon2010b), ammonium nonanoate provided effective control of a number of warm-season weed species, though the results varied with application rate and volume. Weed control was higher with an application rate of 10.8 kg ha−1 compared with a rate of 7.2 kg ha−1 for most of the weed species in the study. Weed control among the weed species was not consistent with the various spray volumes (164, 327, 654, and 981 L ha−1) of ammonium nonanoate used in the study. Control of carpetweed (Mollugo verticillata L.), goosegrass (Eleusine indica L.), and crabgrass (Digitaria spp.) was not affected by the spray volume of ammonium nonanoate, in contrast to tumble pigweed (Amaranthus albus L.) and spiny pigweed (Amaranthus spinosus L.).
Weed control efficacy of essential oil and/or ammonium nonanoate herbicides may be affected by various factors such as weed species, stage of development of target weed species, application rate, spray volume, and environmental conditions before, during, and after herbicide application (Brainard et al. Reference Brainard, Curran, Bellinder, Ngouajio, VanGessel, Haar, Lanini and Masiunas2013; Cavalieri and Caporali Reference Cavalieri and Caporali2010; Webber et al. Reference Webber, Shrefler, Brandenberger, Taylor, Carrier and Shannon2010b). Crop injury is another concern for using these nonselective essential oil and/or ammonium nonanoate herbicides. The extent of crop injury varies with the size of the crop at the time of herbicide application. Crop injury can be minimized by adopting lower application rates for the herbicides and/or by directed application of the herbicide to reduce its contact with the crop (Evans and Bellinder Reference Evans and Bellinder2009). It is important to optimize the application rate, spray volume, application method, and time of application of nonselective essential oil and/or soap based herbicides in order to maximize the weed control efficacy and to minimize crop injury especially in high value crops such as vegetables.
Pumpkin is a high value vegetable crop that has high nutritional and health benefits (El-Hamed and Elwan Reference El-Hamed and Elwan2011), and is a rich source of β-carotene, an important carotenoid and an antioxidant (Stevenson et al. Reference Stevenson, Eller, Wang, Jane, Wang and Inglett2007). In the human body, β-carotene is converted into vitamin A, which is essential for healthy skin and mucus membranes, the immune system, as well as eye health and vision (Gonçalves et al. Reference Gonçalves, Pinheiro, Abreu, Brandão and Silva2007). Pumpkin is one of the most widely cultivated vegetable crops in Texas, with 2,023 to 3,238 ha planted every year, primarily in West Texas. This makes Texas the fourth largest pumpkin producing state in the United states (TAMU 2000). Therefore, pumpkin growers in West Texas are always looking for research-based weed control methods, preferably essential oil–based and/or soap-based herbicides, that could improve pumpkin yield and WUE, and are also environmentally safe. However, very few studies have investigated the use of essential oils or soap-based weed control practices and their effects on yield and water productivity, particularly in West Texas. Therefore, the objective of this study was to determine the effect of ammonium nonanoate and an essential oil herbicide on physiology, plant growth, yield, soil water depletion pattern, and WUE of pumpkin.
Materials and Methods
Experimental Conditions
A field study was conducted for two seasons from June 24th to September 25th in 2019 and from June 8th to September 3rd in 2020 on land used for conventional crop production at the Quaker Research Farm of Texas Tech University, in Lubbock, TX (33.6754°N, 101.7980°W; 990 m above sea level). The experimental site has semiarid climatic conditions with average annual high and low temperatures of 23.83 C and 7.83 C, respectively, and an average annual rainfall of 469 mm (Parkash et al. Reference Parkash, Singh, Deb, Ritchie and Wallace2021; TAMU 2020). The study site has a combination of Amarillo sandy clay loam (fine-loamy, mixed, superactive, thermic Aridic Paleustoll) and Olton clay loam (fine, mixed, superactive, thermic Aridic Paleustolls).
Crop Management Practices and Experimental Design
Untreated seeds of Bayhorse Gold hybrid of pumpkin were procured from Rupp seeds Inc. (Wauseon, OH). The field was disked and plowed, and 1-m-wide raised beds were prepared. Seed was planted using a four-row planter with 1-m row-to-row spacing but seed was put only in the first and third box of the planter to achieve 2-m row-to-row spacing of pumpkin. Plant-to-plant spacing within rows was maintained at 60 cm. The URAN 32 (32–0–0, Nitrogen Fertilizer Solution; Nutrien Ag Solution, Loveland, CC) was used to apply 55 kg of nitrogen per hectare in both years. The crop was irrigated using a subsurface drip irrigation system. Irrigation was applied based on daily crop evapotranspiration (ETc) losses calculated as a product of the reference evapotranspiration (ET0) and crop coefficient (Kc). The Penman-Monteith equation was used to calculate ET0 from weather data (Allen et al. Reference Allen, Pereira, Raes and Smith1998). Weather data were obtained from a weather station installed at the experimental location. Predetermined stage-specific crop coefficients for pumpkin were used; Kc initial = 0.5 (0–20 DAP), Kc development = 0.70 (21–50 DAP), Kc mid-season = 1.0 (51–80 DAP), and Kc late-season = 0.8 (81–100 DAP; Allen et al. Reference Allen, Pereira, Raes and Smith1998). To calculate actual irrigation requirements, rainfall was subtracted from crop water requirements (ETc). If ETc requirements for a week were higher than rainfall received in that week then at the end of the week, the irrigation amount applied was equal to the amount of ETc minus rainfall. Irrigation was applied once per week and on the same day to replace the amount of water used for ETc in the previous week (Parkash et al. Reference Parkash, Singh, Deb, Ritchie and Wallace2021).
The experiment was conducted using a randomized complete block design with four replications for each treatment. Each plot was 9 m long and 10 m wide. The following two herbicides were evaluated: 1) ammonium nonanoate (Axxe®, 40% ammonium nonanoate; BioSafe Systems, East Hartford, CT) and 2) clove oil + cinnamon oil (Weed Zap®, 45% clove oil, and 45% Cinnamon oil; JHBiotech Inc., Ventura, CA). Each herbicide was evaluated at two application rates. Overall, there were five treatments: 1) ammonium nonanoate 5% ai, 2) ammonium nonanoate 6% ai, 3) clove oil + cinnamon oil 4.5% ai, 4) clove oil + cinnamon oil 9% ai, and 5) untreated control. These two concentrations (highest and second highest recommended concentrations) for each herbicide were chosen based on the recommendations on herbicide labels to evaluate their weed control efficacy and their potential for crop injury. Adjuvant (Nufilm-P; Miller chemical and fertilizer, LLC, Hanover, PA) was added at a rate of 0.125% vol/vol to spray solution of each herbicide treatment to increase the contact and adhesion of the herbicide onto the plant surface. For each herbicide treatment, an equal volume (600 L ha−1) of herbicide solution was sprayed. Herbicides were sprayed using a single boom battery-powered spray pump (EWING 4 Gallon Pro Backpack Sprayer). A directed spray hood was used to prevent any herbicide drift onto the pumpkin. In both years of the study, each plot was sprayed twice (20 and 30 d after planting [DAP]) with the same herbicide treatment.
Weed Control Measurements
Weed density in each experimental unit was determined at 23 (3 d after the first application of herbicide treatments) and 33 (3 d after the second application of herbicide treatments) DAP in both years. Weeds with injury but not dead were counted in three 0.25-m2 (0.5 m × 0.5 m) quadrats randomly placed in each plot. The average of the number of weeds present in three quadrats were taken to convert the number of weeds per quadrat to the number of weeds per square meter for each plot. Weed control rate was calculated as follows: weed control = 100 – ([weed density in a treatment/weed density in untreated control] × 100). Weed biomass per square meter was determined at 33 DAP in both years. After counting the weeds present in the three 0.25-m2 quadrats, weeds were cut at the soil surface and all weeds from three quadrats were put in one bag for each plot. Weed samples were dried in an oven at 70 C for 48 h to determine the weed biomass per square meter in each plot.
Gas Exchange Measurements
To determine the effect of herbicide treatments on the gas exchange parameters of the pumpkin, leaf photosynthesis rate (Pn) and stomatal conductance (gs) were measured using a portable photosynthesis system (model Li-6800; LI-COR, Lincoln, NE). Gas exchange measurements were taken between 1100 and 1400 hours from the newest, fully expanded, and well-illuminated leaf. For all measurements in both years, the portable photosynthesis system was kept at a steady state by maintaining airflow rate at 700 μmol s–1, reference CO2 concentration at 400 µmol mol−1, photosynthetically active radiation at 1,500 µmol m2− s−1, and relative humidity at 65% inside a 6-cm2 leaf chamber. However, the temperature control of the chamber was kept off. Gas exchange measurements were taken from two different plants in each plot. Gas exchange measurements were taken twice at 37 and 51 DAP in 2019, and 38 and 55 DAP in 2020.
Plant Biomass and Fruit Yield
To assess the effects of herbicide treatments on shoot dry biomass (stems and leaves) accumulation in pumpkin, plants were cut at the base in a 4-m2 area (around three plants per plot), put in one brown paper bag for each plot, and oven-dried to a constant weight at 70 C. Plants were sampled for shoot dry biomass at 37 and 73 DAP in 2019, and 38 and 52 DAP in 2020. To determine pumpkin yield, fruits were harvested only one time from a 10-m2 area in each plot at 93 and 87 DAP in 2019 and 2020, respectively. Average fruit weight was determined by dividing the total weight of fruits harvested by the total number of fruits harvested.
Soil Water Depletion, Evapotranspiration, and WUE
Volumetric water (VWC, m3 m–3) was determined at the start of the growing season and at harvest using a capacitance probe (model PR2/6 Profile Probe; Delta-T Devices Ltd., UK). A 100-cm-long access tube for the capacitance probe was installed manually in the center of each plot next to a pumpkin plant (5 cm away from the base of the plant). The PR2 probe measures the VWC at 10-, 20-, 30-, 40-, 60-, and 100-cm depths of the soil profile. At each soil depth, soil water content (in millimeters) was calculated by multiplying the VWC at each depth with the depth increment (also in millimeters; Bhattarai et al. Reference Bhattarai, Singh, West, Ritchie and Trostle2020b). Change in soil water storage (ΔS) during the growing season was calculated by subtracting the soil water content at harvest from the initial soil water content. The water balance equation was used to calculate the evapotranspiration (ET) as follows: ET= I + P ± ΔS – R – D, where I is irrigation (mm), P is precipitation (mm), ΔS is the seasonal change in the amount of stored soil water in the 100-cm soil profile (mm), R is surface runoff (mm), and D is the drainage of water vertically below the root zone (mm; Singh et al. Reference Singh, Angadi, Grover, Hilaire and Begna2016; Wang et al. Reference Wang, Gan, Hamel and Lemke2012). Since the crop was irrigated using a subsurface drip irrigation system, the experimental site was leveled, and there were no heavy precipitation events during the growing season; therefore, drainage (D) and surface runoff (R) were considered negligible when calculating evapotranspiration (Bhattarai et al. Reference Bhattarai, Singh, West, Ritchie and Trostle2020b; Hao et al. Reference Hao, Xue, Marek, Jessup, Hou, Xu, Bynum and Bean2015; Singh et al. Reference Singh, Angadi, Grover, Hilaire and Begna2016). The WUE (kg ha−1 mm−1) was determined using the following formula: WUE = fresh fruit yield (kg ha−1)/total seasonal crop ET (mm; Bhattarai et al. Reference Bhattarai, Singh, Angadi, Begna, Saini and Auld2020a; Hao et al. Reference Hao, Xue, Marek, Jessup, Hou, Xu, Bynum and Bean2015). Irrigation WUE (IWUE, kg ha−1 mm−1) was calculated with the following formula: fresh fruit yield (kg ha−1)/total seasonal water applied (mm).
Statistical Analysis
Data collected for each parameter were analyzed using ANOVA with a randomized complete block design in R version 3.5.2 (R Core Team 2018) using Agricolae package version 1.2–8. Herbicide treatments were kept as fixed factors and replication was kept as a random effect. Data for each year were analyzed separately. Data for weed control and dry weed biomass were transformed using square root arcsine transformation to normalize the data prior to analysis (Saini et al. Reference Saini, Singh and Deb2020). To compare the treatment means, the LSD test at a 5% significance level was used. Figures were created using SigmaPlot software version 14 (Systat Software, San Jose, CA).
Results and Discussion
Weather Conditions
The daily rainfall and minimum, maximum, and average temperatures observed during the pumpkin growing seasons of 2019 and 2020 are presented in Figure 1. During the pumpkin growing seasons, the total rainfall received was 146 mm in 2019 and 50 mm in 2020, and the average air temperature was 27.27 C in 2019 and 28.47 C in 2020. Overall, maximum and minimum air temperatures during the entire growing season were 42.30 C and 16.17 C, respectively, in 2019; and 44.06 C and 9.83 C, respectively, in 2020. Higher average air temperature and lower rainfall in 2020 suggested that weather conditions were more stressful in 2020 than in 2019. Rainfall was lower in 2020 (50 mm) than in 2019 (146 mm), which necessitated higher application of irrigation in 2020 (466 mm) than in 2019 (366 mm).

Figure 1. Daily rainfall, and minimum, maximum and average air temperatures observed during the growing period of pumpkin from June 24th to September 25th in 2019, and from June 8th to September 3rd in 2020 in Lubbock, TX.
Weed Control
The most common weeds present were Palmer amaranth (Amaranthus palmeri S. Watson), silver leaf nightshade (Solanum elaeagnifolium C.), spotted spurge (Euphorbia maculata L.), redroot pigweed (Amaranthus retroflexus L.), purslane (Portulaca oleracea L.), and tumble pigweed. Effects of herbicide treatments on weed control and dry weed biomass are presented in Table 1. In both years, significant weed control was observed with all of the herbicide treatments. Weed control was significantly higher with ammonium nonanoate (88% to 98%) than with clove oil + cinnamon oil (40% to 69%) in both years. Increase in herbicide concentration did not improve weed control with either herbicide. Dry weed biomass was significantly lower in all the herbicide treatments compared to the untreated control at 33 DAP. Dry weed biomass production was significantly lower with ammonium nonanoate than with clove oil + cinnamon oil. Increased herbicide concentration did not result in more effective suppression of biomass production with either herbicide. The results for weed control with ammonium nonanoate are in agreement with the results of the other studies in which ammonium nonanoate was found to be very effective, providing weed control greater than 90% with application rates of 3% to 6% (Webber et al. Reference Webber, Shrefler and Brandenberger2010a, 2013). Lanini (Reference Lanini2010) reported that clove oil + cinnamon oil at 9% application rate resulted in 38% weed control for 19-d-old weeds and 100% weed control for 12-d-old weeds.

a Abbreviation: DAP, days after planting.
b Percentages after the names of the herbicides indicate the concentration of active ingredient of herbicide in the final spray solution.
c Mean values followed by the same letter in each column are not significantly different according to the least significant difference test at P ≤ 0.05. Weeds with injury but not dead were counted to derive weed density values. Weed control (%) = 100 – ([weed density in an experimental unit/weed density in untreated control] × 100).
Gas Exchange Parameters
The Pn and gs were significantly different among the herbicide treatments (Figure 2). The Pn and gs were significantly higher with ammonium nonanoate treatments compared to the untreated weed control but there was not significant difference between clove oil + cinnamon oil treatments and the untreated control on most of the sampling dates in both years. This implies that Pn and gs were lower in treatments that had higher weed density. In a study on spring wheat by Iqbal and Wright (Reference Iqbal and Wright1999), Pn decreased with an increase in weed density. Similarly, Pn of rice decreased as the weed density increased (Wang et al. Reference Wang, Zhang, Xu and Li2019). In the current study, in treatments with higher weed density it is likely that weeds were competing with pumpkin for water. Because of this, water availability for uptake by the pumpkin crop to meet transpiration requirements would have decreased, leading to the closure of stomata, and this is evident from lower gs in treatments with higher weed density. In our study also, a decrease in gs might have caused a decline in leaf photosynthesis rate. Because it is known that gs regulates gas exchange (CO2 intake and water loss) and a decrease in gs could decrease the supply of CO2 (required for Pn) to the parenchyma cells (Parkash and Singh Reference Parkash and Singh2020a, 2020b; Parkash et al. Reference Parkash, Singh, Deb, Ritchie and Wallace2021). More vigorous weeds can result in shading of the crop (Massinga et al. Reference Massinga, Currie and Trooien2003). In our study, Palmer amaranth was the most common weed, and it was taller than pumpkin. This might have caused lower light interception by the pumpkin plants and a subsequent decrease in Pn of shaded leaves due to decreased light interception. But in our study, gas exchange measurements were made on fully expanded and well-illuminated pumpkin leaves. So the observed decrease in photosynthesis rate of well-illuminated leaves was likely caused by stomatal closure due to water-uptake competition between weeds and pumpkin. Overall, it can be inferred that increased weed competition for water led to a decline in the Pn of well-illuminated leaves and the competition for both water and light might have resulted in a decline in Pn of shaded leaves of pumpkin in treatments with high weed density.

Figure 2. Effect of herbicide treatments on the leaf photosynthesis rate (Pn; A and B) and stomatal conductance (gs; C and D) of pumpkin in 2019 and 2020. Percentages after the names of the herbicides in the legend indicate the concentration of active ingredient of herbicide in the final spray solution. Error bars indicate standard errors of the mean (n = 4). On each measurement day, bars with the same letter are not significantly different at P ≤ 0.05.
Plant Biomass and Fruit Yield
Shoot dry biomass accumulation by pumpkin was significantly different among the herbicide treatments (Table 2). Shoot dry biomass of pumpkin was significantly greater with ammonium nonanoate treatments compared to the other treatments and the untreated control. Biomass accumulation was not significantly different between clove oil + cinnamon oil and the untreated control on most of the sampling dates in both years. Better weed control with herbicide treatments resulted in higher pumpkin shoot biomass accumulation in those treatments. Average fruit weight and total fruit yield were significantly different among the treatments (Table 2). Average fruit weight and total fruit yield were significantly higher in ammonium nonanoate treatments compared to both the clove oil + cinnamon oil treatments and untreated controls. For the clove oil + cinnamon oil herbicide, only the higher concentration of the herbicide resulted in significantly higher average fruit weight and total fruit yield compared to the untreated control. Use of higher concentrations of either herbicide did not result in significantly higher average fruit weight and total fruit yield. Treatments with higher weed infestation has smaller fruits and lower total fruit yields. Saini et al. (Reference Saini, Singh and Deb2020) also found that the fruit yield of pumpkin was lower in treatments that had higher weed infestation.
Table 2. Effect of herbicide treatments on shoot dry biomass, average fruit weight, and fruit yield of pumpkin. a, b, c

a Abbreviation: DAP, indicates days after planting.
b Percentages after the names of the herbicides indicate the concentration of active ingredient of herbicide in the final spray solution.
c Mean values followed by the same letter in each column are not significantly different according to the least significant difference test at P ≤ 0.05.
Soil Water Depletion, Evapotranspiration, WUE, and IWUE
Soil water depletion for the entire growing season was not significantly different among all the herbicide treatments at each soil depth in both years (Figure 3). Total soil water depletion in the complete 100-cm soil profile was 7 mm with ammonium nonanoate 6%, 9 mm with ammonium nonanoate 5%, 0 mm with clove oil + cinnamon oil 9%, −4 mm with clove oil + cinnamon oil 4.5%, and −17 mm with untreated control in 2019. Corresponding values of soil water depletion for herbicides treatments in 2020 were 13 mm, 11 mm, 18 mm, 20 mm, and 29 mm, respectively. In a similar study by Massinga et al. (Reference Massinga, Currie and Trooien2003), it was found that under well-watered conditions, soil water content was comparable among the weed-free corn and corn mixed with different densities of weeds.

Figure 3. Effect of herbicide treatments on soil water depletion along the 100-cm soil profile. Percentages after the names of the herbicides in the legend indicate the concentration of active ingredient of herbicide in the final spray solution. Bars indicate LSD at P ≤ 0.05 among the herbicide treatments at a given depth. NS indicates no significant difference among the herbicide treatments at a given depth.
ET was not significantly different among all the herbicide treatments in both years (Table 3). The amount of irrigation water applied, and rainfall received were the same for all treatments, and soil water depletion was not significantly different among all the treatments.
Table 3. Effect of herbicide treatments on evapotranspiration, water use efficiency, and irrigation water use efficiency of pumpkin. a, b, c
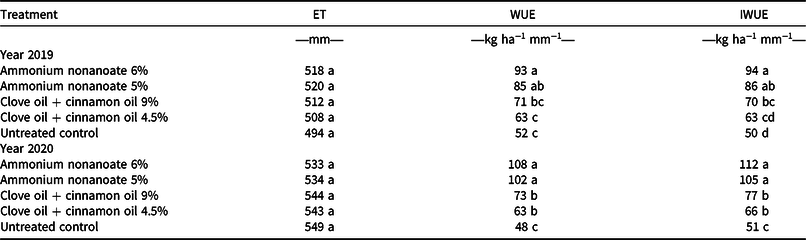
a Abbreviations: ET, evapotranspiration; IWUE, irrigation water use efficiency; WUE, water use efficiency.
b Percentages after the names of the herbicides indicate the concentration of active ingredient of herbicide in the final spray solution.
c Mean values followed by the same letter in each column are not significantly different according to the least significant difference test at P ≤ 0.05.
The WUE and IWUE were significantly different among the herbicide treatments in both years (Table 3). In 2019, WUE was higher by 41, 33, 19, and 11 kg ha−1 mm−1 with ammonium nonanoate 6%, ammonium nonanoate 5%, clove oil + cinnamon oil 9%, clove oil + cinnamon oil 4.5%, respectively, compared to the untreated control. Whereas WUE was higher in 2020 by 60, 54, 25, and 15 kg ha−1 mm−1 with ammonium nonanoate 6%, ammonium nonanoate 5%, clove oil + cinnamon oil 9%, clove oil + cinnamon oil 4.5%, respectively, compared to untreated control. The IWUE followed trend similar to that of WUE in response to herbicide treatments in both years. The WUE is associated with fruit yield and ET, whereas IWUE is associated with fruit yield and irrigation amount. In our study, competition from weeds significantly reduced the amount of biomass produced per unit of water lost as evapotranspiration, resulting in lower yield and ultimately lower WUE. In similar way, weed competition reduced the amount of biomass produced per unit of water applied as irrigation, resulting in lower yield and IWUE in treatments, which had higher weed infestation. In similar studies with corn (Massinga et al. Reference Massinga, Currie and Trooien2003) and wheat (Verma et al. Reference Verma, Singh, Prasad and Meena2015), the WUE of the crops decreased with an increase in the weed density. Overall, ammonium nonanoate herbicide was more effective than the clove oil + cinnamon oil herbicide in controlling the weeds, and resulted in more efficient use of water, producing the highest fruit biomass per unit of water used.
Conclusions
In our study, weed infestation with herbicide treatments was significantly lower than it was for the untreated control. Ammonium nonanoate provided more effective weed control than clove oil + cinnamon oil herbicide. Plant physiological processes, plant growth, and yield were negatively impacted in the treatments that had higher weed infestation. Although herbicide treatments had no significant effect on ET, both rates of ammonium nonanoate resulted in higher WUE and IWUE in both years, whereas with both rates of clove oil + cinnamon oil herbicide, WUE and IWUE were higher than untreated control only in 2020. The WUE and IWUE were higher with ammonium nonanoate, which also resulted in higher fruit yields. Overall, it can be concluded that herbicide treatments that provide effective weed control can prevent yield losses due to weed competition and also increase the WUE of pumpkin in semiarid regions in Texas. With no difference in response to two concentrations, ammonium nonanoate at its lower rate (5%) can be recommended for effective for weed control and for improving yield and WUE of pumpkin in West Texas, in conventional cropping systems. This active ingredient is not allowed for use in organic pumpkin production due to National Organic Program regulations that restrict its use in organic cropping systems to farmstead maintenance and ornamental crops, but this would be a best option for conventional cropping systems. Future research should be conducted to optimize the spray volume for these herbicides.
Acknowledgments
This research received no external funding. We are thankful to Lelton Howel, Reagan Anders, Azeezahmed Shaik, Bishwoyog Bhattarai, Kamalpreet Kaur, and Irish Pabuayon for their help in the field and laboratory work. No conflicts of interest have been declared.