INTRODUCTION
Phenylketonuria (PKU) is an autosomal recessive inborn error of phenylalanine metabolism caused by deficiency of phenylalanine hydroxylase (PAH). This enzyme converts phenylalanine into tyrosine, a precursor of dopamine and other catecholamines (Scriver & Kaufman, Reference Scriver, Kaufman, Scriver, Baudet, Sly and Valle2001). Tetrahydrobiopterin, also known as sapropterin, is a necessary cofactor of PAH (Erlandsen & Stevens, Reference Erlandsen and Stevens2001). The PAH gene is located on chromosome 12q23.1 (Scriver & Kaufman, Reference Scriver, Kaufman, Scriver, Baudet, Sly and Valle2001). Systematic newborn screening programs are conducted in several countries since the 1970s. Usual classification scheme of PKU severity is based on pretreatment blood phenylalanine levels. The normal range of blood phenylalanine concentrations is 50–110 µmol/L. Patients with classical PKU (phenylalanine level > 1200 µmol/L) and mild PKU (phenylalanine level between 600 and 1200 µmol/L) require treatment. Patients with phenylalanine levels lower than 600 µmol/L are classified in the group of mild hyperphenylalaninemia. Prevalence rate of PKU varies between countries, from approximatively 1/3500 newborns in Turkey, to 1/15,000 newborns in the United States (Scriver & Kaufman, Reference Scriver, Kaufman, Scriver, Baudet, Sly and Valle2001).
The mutation of the PAH gene leads to PAH deficiency, which increases serum levels of phenylalanine and decreases tyrosine levels, impairing cerebral protein synthesis (de Groot et al., Reference de Groot, Hoeksma, Reijngoud, de Valk, Paans, Sauer and van Spronsen2013). High blood and brain phenylalanine concentrations are toxic and can cause severe and irreversible neurological damage, such as seizures, microcephaly, and intellectual disability. The aim of treatment is to decrease the blood phenylalanine concentration. The dietary phenylalanine restriction is the mainstay of treatment, but some patients with PKU, especially with a higher residual PAH activity, respond to tetrahydrobiopterin administration. PKU is treated as soon as possible after birth with a low phenylalanine diet and consumption of medical food substitutes containing the appropriate mix of essential amino acids, vitamins, minerals, and trace nutrients (Acosta & Matalon, Reference Acosta and Matalon2010). The primary goal of treatment is to achieve an optimal neuropsychological outcome by preventing phenylalanine toxicity. The effectiveness of treatment depends on its early introduction, its quality, and its duration (for a review, see Brumm & Grant, Reference Brumm and Grant2010). Currently, there is no international consensus on the upper targets of phenylalanine level at the different periods of life (for American guidelines, see Camp et al., Reference Camp, Parisi, Acosta, Berry, Bilder, Blau, Bodamer, Brosco, Brown, Burlina, Burton, Chang, Coates, Cunningham, Dobrowolski, Ferguson, Franklin, Frazier, Grange, Greene, Groft, Harding, Howell, Huntington, Hyatt-Knorr, Jevaji, Levy, Lichter-Konecki, Lindegren, Lloyd-Puryear, Matalon, MacDonald, McPheeters, Mitchell, Mofidi, Moseley, Mueller, Mulberg, Nerurkar, Ogata, Pariser, Prasad, Pridjiann, Rasmussen, Reddy, Rohr, Singh, Sirrs, Stremer, Tagle, Thompson, Urv, Utz, van Spronsen, Vockley, Waisbren, Weglicki, White, Whitley, Wilfond, Yannicelli and Young2014; for European guidelines, see van Spronsen et al., Reference van Spronsen, van Wegberg, Ahring, Bélanger-Quintana, Blau, Bosch, Burlina, Campistol, Feillet, Giżewska, Huijbregts, Kearney, Leuzzi, Maillot, Muntau, Trefz, van Rijn, Walter and MacDonald2017).
Early and continuously treated PKU (ECT-PKU) prevents intellectual disability and the most severe neurodevelopmental consequences of PKU (Mitchell et al., Reference Mitchell, Trakadis and Scriver2011). However, there is a higher risk of a slight decrease in intelligence (for a meta-analysis, see DeRoche & Welsh, Reference DeRoche and Welsh2008) and neuropsychological problems (for a meta-analysis, see Moyle et al., Reference Moyle, Fox, Arthur, Bynevelt and Burnett2007). These outcomes are due to a complex array of factors, including phenylalanine toxicity. Phenylalanine-level targets in children with ECT-PKU are supposed to prevent neuropsychological problems, but they’re still higher in comparison with the normal level of non-PKU children. Diamond et al. (Reference Diamond, Prevor, Callender and Druin1997) and Blau et al. (Reference Blau, van Spronsen and Levy2010) hypothesized that neuropsychological impairments may be caused by reduced dopamine and serotonin synthesis. In addition to neurotransmitter dysregulation, Dyer (Reference Dyer1999) and Anderson and Leuzzi (Reference Anderson and Leuzzi2010) related the extent and severity of cognitive deficits to impairment in the maintenance and production of myelin. The prefrontal cortex and dopaminergic activity play a crucial role in higher-order cognitive abilities conceptualized as executive functions (EFs) (Jurado & Rosselli, Reference Jurado and Rosselli2007; Welsh et al., Reference Welsh, Pennington, Ozonoff, Rouse and McCabe1990). In this context, many studies were devoted to EFs (for a review, see Christ et al., Reference Christ, Huijbregts, De Sonneville and White2010a). Less often discussed, impairments have been identified in nonexecutive abilities as well (for a review, see Janzen & Nguyen, Reference Janzen and Nguyen2010).
Earlier qualitative and critical reviews have pointed to a number of neuropsychological problems in children with ECT-PKU. However, the neuropsychological risks associated with ECT-PKU are not fully elucidated. Neuropsychological sequelae have an influence on treatment adjustment and care in PKU; therefore, it is particularly important to understand the nature of those risks. Previous reviews (Christ et al., Reference Christ, Huijbregts, De Sonneville and White2010a; Janzen & Nguyen, Reference Janzen and Nguyen2010) suggested the need for a more comprehensive approach to the neuropsychology of ECT-PKU. These reviews discussed neural underpinnings (i.e., dopamine dysfunction hypothesis/white matter hypothesis), but did not discuss recent advances in biochemical aspects and treatments of PKU.
The first aim of this study was to present a systematic and integrative review of studies of the neuropsychological profile of children and adolescents with ECT-PKU to inform future directions for research. The second aim was to present recent advances in biochemical markers and treatments of PKU, and focus on the role of metabolic control as a determinant of neuropsychological outcome. An argumentative synthesis, as well as clinical and research prospects, is presented in the final section.
METHODS
For the first section of this review, neuropsychological studies of children and adolescents with ECT-PKU were identified through computerized searches of Medline via the PubMed interface, PsycINFO, and PsycARTICLE. Search terms were (phenylketonuria) AND (children) AND (neuropsychology OR cognitive OR cognition OR executive function). This systematic review was based on the PRISMA statements (Moher et al., Reference Moher, Shamseer, Clarke, Ghersi, Liberati, Petticrew, Shekelle and Stewart2015). The flow of studies through the screening process is presented in the PRISMA flowchart (see Figure 1). Abstracts were screened and the inclusion criteria applied.
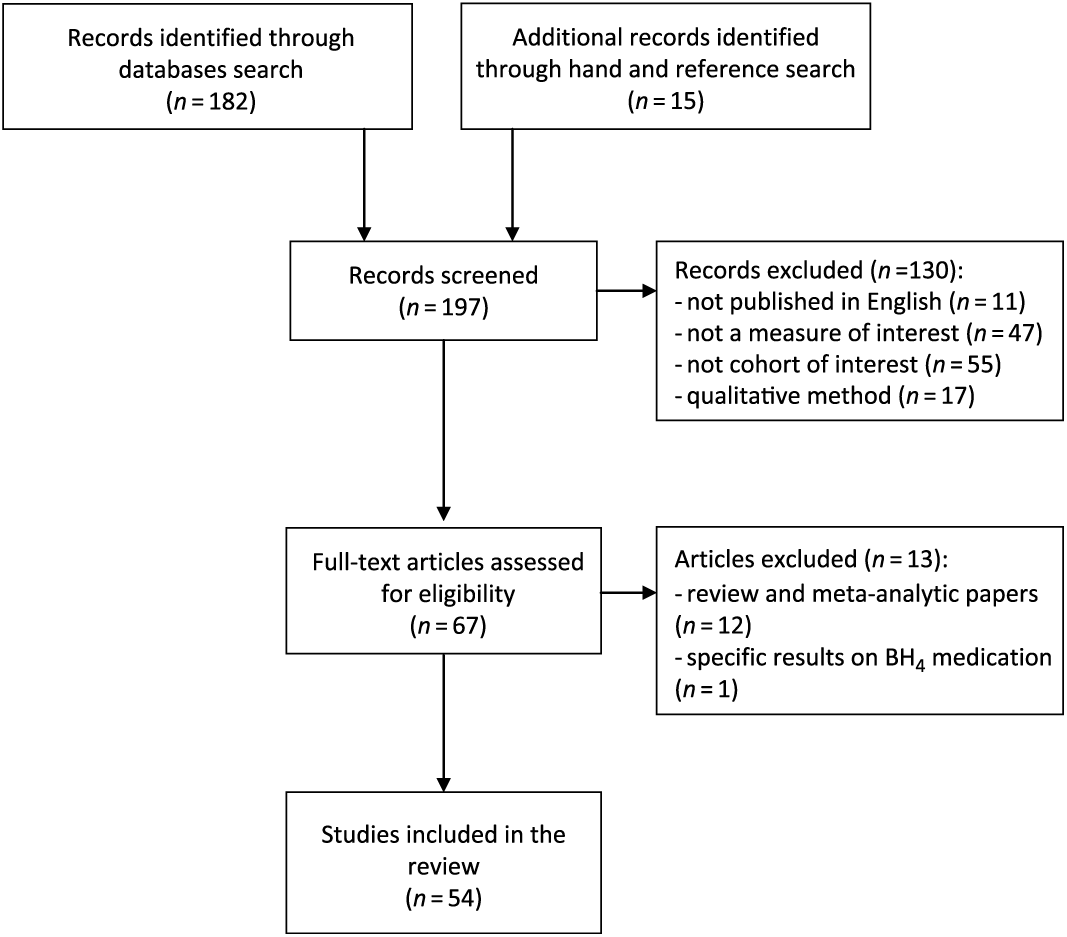
Fig. 1. PRISMA flowchart from search results.
The review included (1) English language studies; (2) prospective and retrospective studies; (3) longitudinal and cross-sectional studies; (4) studies with an experimental group of children and/or adolescent with ECT-PKU; (5) studies that compared the performances of children with ECT-PKU to a control group or test norms using quantitative methods; and (6) studies on intellectual functioning, nonverbal abilities (visual perception, visual–spatial abilities, visual construction, and motor skills), language and academic achievement, memory, EFs, and information processing speed. Based on the current understanding of executive dysfunction in attention-deficit hyperactivity disorder (ADHD) (Barkley, Reference Barkley1997; Sonuga-Barke et al., Reference Sonuga-Barke, Sergeant, Nigg and Willcutt2008), we included a review of ADHD symptoms in the review of EFs.
The review did not include (1) case studies; (2) review and meta-analytic papers; (3) studies of adults with PKU; (4) studies of children/adolescents with PKU who were treated after 60 days of life or had stopped their treatment early; (5) studies of participants with mild hyperphenylalaninemia; and (6) studies of children with ECT-PKU who used tetrahydrobiopterin medication.
Of the 197 studies retrieved from the search, we considered 54 eligible for analysis. Overall, the studies ranged in publication date from 1981 to the end of 2016. We summarized the methodology and results of each article in three tables (before 2000, Table 1; from 2000 to 2009, Table 2; from 2010 to 2017, Table 3).
Table 1. Neuropsychological findings before 2000

PKU(h) = subgroup of patients with high phenylalanine levels; PKU(l) = subgroup of patients with low phenylalanine levels; PKU(c) = subgroup of children with ECT-PKU; PKU(a) = subgroup of adolescents with ECT-PKU.
See Supplementary Material for the list of abbreviations used for the tools.
aAge-controlled; bGenre-controlled; cSocioeconomic status-controlled; dIntelligence Quotient-controlled; *Impairments.
Table 2. Neuropsychological findings from 2000 to 2009

PKU(h) = subgroup of patients with high phenylalanine levels; PKU(l) = subgroup of patients with low phenylalanine levels; PKU(c) = subgroup of children with ECT-PKU; PKU(a) = subgroup of adolescents with ECT-PKU; PKU(wma) = subgroup of patients with white matter abnormalities; PKU(wwma) = subgroup of patients without white matter abnormalities.
See Supplementary Material for the list of abbreviations used for the tools.
aAge-controlled; bGenre-controlled; cSocioeconomic status-controlled; dIntelligence Quotient-controlled; eDiabetic patients controls; fADHD patients controls; *Impairments.
Table 3. Neuropsychological findings from 2010 to 2017

PKU(h) = subgroup of patients with high phenylalanine levels; PKU(l) = subgroup of patients with low phenylalanine levels; PKU(c) = subgroup of children with ECT-PKU; PKU(a) = subgroup of adolescents with ECT-PKU.
See Supplementary Material for the list of abbreviations used for the tools.
aAge-controlled; bGenre-controlled; cSocioeconomic status-controlled; dIntelligence Quotient-controlled; *Impairments.
The second part of this paper presents findings from studies of neuropsychological profiles within the context of recent advances in biochemical markers and treatments of PKU.
Table 4 and Figure 2 summarize results and key recommendations from the extensive review.
Table 4. Synthesis of findings and key recommendations


Fig. 2. Synthesis of results of review on neuropsychological profile in ECT-PKU children. Note. Intell., Intelligence; VP, Visual perception; VS, Visual–spatial abilities; VC, Visual construction; Prax., Praxis; Lang., Oral language; Spell., Spelling; Read., Reading; Math., Mathematics; Mem, Explicit memory; Efs, Executive functions; Speed, Processing speed.
RESULTS
Neuropsychological Profile of Children and Adolescents with ECT-PKU
Intellectual functioning
Intellectual functioning was assessed in 63% of the articles in the literature. In 41% of these studies, intellectual level was measured as a control variable. In all studies on intellectual functioning, the mean Full Scale Intelligence Quotient (FSIQ) score of children with ECT-PKU group was within the normal range. The mean FSIQ of the group with ECT-PKU was compared with a control group or test norms in most studies (73% vs. 6%, respectively). In 52% of these studies, children with ECT-PKU performed significantly lower than controls or test norms. Approximately half of the studies on intellectual functioning used complete intelligence tests (e.g., WISC—Weschler Intelligence Scale for Children). The other half used abbreviated versions of these scales or g factor tests (e.g., WASI—Wechsler Abbreviated Scale of Intelligence). A majority of studies that identified lower intellect used entire rather than abbreviated tests (62% vs. 36%, respectively).
Aside from the slight downward shift in FSIQ, only five studies have compared verbal and perceptual reasoning in children with ECT-PKU to controls or normative samples. And results were mixed. To our knowledge, only two studies have compared Verbal Intelligence Quotient (VIQ) to Performance Intelligence Quotient (PIQ) within group, again with mixed results. Cappelletti et al. (Reference Cappelletti, Cotugno, Goffredo, Nicolò, Bernabei, Caviglia and Di Ciommo2013) found no significant discrepancy between VIQ and PIQ, whereas Griffiths et al. (Reference Griffiths, Demellweek, Fay, Robinson and Davidson2000) found lower PIQ than VIQ.
Visual perception, visual–spatial and visual construction abilities, and motor skills
Visual perception was assessed in seven studies (13% of the literature). Findings suggested that visual perception was intact. Mixed results were found concerning sensitivity to visual contrast (Diamond & Herzberg, Reference Diamond and Herzberg1996; Stemerdink et al., Reference Stemerdink, van der Molen, Kalverboer, van der Meere, Huisman, de Jong, Slijper, Verkerk and van Spronsen1999).
Seven studies examined visual–spatial and visual construction abilities (13% of the literature). Only two studies used simple spatial tasks (spatial subtests of British Abilities Scales and Beery-Developmental Test of Visual Motor Integration scales). Impairments were not revealed in such tasks. Deficits were mainly demonstrated on tasks with more complex demands. Six studies used visual constructive tasks, including five that used the copy component Rey–Osterrieth Complex Figure Test (ROCF). Deficits were identified in four of these studies.
Four studies examined praxis (7% of the literature). Only fine motor skills and higher-order motor control were explored. Simple motor tasks (e.g., Purdue Pegboard or Tapping) and complex motor tasks requiring coordination and planning (e.g., target tracking) were used. Impairment was consistently reported for both simple and complex motor tasks.
Language and academic achievement
Language can be subdivided into subdomains such as receptive and expressive language, as well as specific areas of functioning that include phonology, syntax, semantics, and pragmatics. There are no studies that have systematically examined all these aspects of language in children with ECT-PKU. In particular, no studies have examined phonology and pragmatic abilities. Five studies (9% of the literature) used oral language tests. Impairments were identified in only one of those studies (Soleymani et al., Reference Soleymani, Keramati, Rohani and Jalaei2015). The ECT-PKU group performed significantly lower than controls in both receptive and expressive language, as well as syntax and semantics. This group with ECT-PKU displayed a lower FSIQ and VIQ. Intellectual level was not specified in the other four studies on oral language.
Academic difficulties have been described in children with ECT-PKU (Stemerdink et al., Reference Stemerdink, Kalverboer, van der Meere, van der Molen, Huisman, de Jong, Slijper, Verkerk and van Spronsen2000). Reading and spelling were examined in five studies (9% of the literature) and mathematics in six studies (11% of the literature). All these studies used composite scores from achievement tests (e.g., Wide Range Achievement Test). Two of five studies have shown a deficit in reading and only one study has shown a deficit in spelling. To our knowledge, no study systematically examined basic reading and writing skills, as well as reading comprehension. Four out of six studies have shown a deficit in mathematics. Only one work examined both calculations and applied word problems (Chang et al., Reference Chang, Gray and O’Brien2000). Children with ECT-PKU performed significantly lower than test norms for calculations, not for applied word problems.
Memory
Explicit memory was examined in nine studies (17% of the literature). Five studies assessed visual memory with the delayed recall condition of the ROCF or the Rey Visual Design Learning Test, and eight studies used verbal memory tasks (only with word-list-learning tasks). Six out of nine memory studies (67%) reported impairment in visual (4/5 studies) as well as verbal memory (5/8 studies).
Although memory functions can be subdivided into specific processes (i.e., encoding, storage, and retrieval), no study systematically examined all these aspects. Learning was consistently measured in word-list- or design-learning tasks (eight studies). Impaired learning was found in five of these studies. In word-list-learning tasks, it is possible to implement executive strategies to enhance learning (e.g., semantic clustering). In the only two studies that have measured “cluster scores,” children with ECT-PKU consistently had a lower use of this strategy. No word-recognition scores were used in the literature. The storage component of memory in children with ECT-PKU was assessed with delay-recall scores in only three studies and was consistently preserved.
EFs and attention-deficit hyperactivity disorder
EFs were widely investigated by 78% of the studies in the literature, with impairments identified in 86% of these studies. Forty-two different executive tasks were listed. In 88% of the studies on this topic, various tasks implying “cool” EFs (see “cool/hot” executive taxonomy, Zelazo & Müller, Reference Zelazo, Müller and Gaswami2002) were used. Cool EFs are elicited by more purely cognitive aspects of EFs, which are characterized by relatively abstract and decontextualized problems. Cool EFs in these studies encompassed abilities such as planning, inhibition, shifting, and working memory (Lehto et al., Reference Lehto, Juujärvi, Kooistra and Pulkkinen2003; Levin et al., Reference Levin, Culhane, Hartmann, Evankovich, Mattson, Harward, Ringholz, Ewing-Cobbs and Fletcher1991). Hot EFs are required for problems that are characterized by high affective involvement or demand flexible appraisals of the affective significance of stimuli. Hot EFs were investigated with only one recent study, which concluded that adolescents with ECT-PKU have a degree of impairment on social-cognitive functioning and social skills (Jahja et al., Reference Jahja, van Spronsen, De Sonneville, van der Meere, Bosch, Hollak, Rubio-Gozalbo, Brouwers, Hofstede, de Vries, Janssen, van der Ploeg, Langendonk and Huijbregts2016). EFs in everyday life were explored in four studies (only with the parental version of the BRIEF—Behavior Rating Inventory of Executive Function). Three studies displayed an executive impairment in everyday life, especially in the metacognition domain. None of these studies compared the BRIEF parental rating to executive performance-based tests. When executive dysfunction was found, its specificity was not systematically studied by comparing it to intellectual level (45% of the studies on EFs controlled this variable). Executive difficulties persisted in 89% of studies on EFs when FSIQ was controlled. Only four studies (i.e., 9% of the studies) on EFs have examined executive dysfunction specificity by comparing it to nonexecutive cognitive abilities (Antshel & Waisbren, Reference Antshel and Waisbren2003a; Griffiths et al., Reference Griffiths, Robinson, Davies, Hayward, Lewis, Livingstone and Plews2005; Stemerdink et al., Reference Stemerdink, van der Molen, Kalverboer, van der Meere, Huisman, de Jong, Slijper, Verkerk and van Spronsen1999; Welsh et al., Reference Welsh, Pennington, Ozonoff, Rouse and McCabe1990). In all these studies, executive impairments were still observed when nonexecutive abilities were controlled.
Executive deficits could potentially explain an overlap of clinical phenotypes between PKU and ADHD (Barkley, Reference Barkley1997; Sonuga-Barke et al., Reference Sonuga-Barke, Sergeant, Nigg and Willcutt2008). Antshel and Waisbren (Reference Antshel and Waisbren2003a, Reference Antshel and Waisbren2003b) showed that 13% of their sample of children with ECT-PKU met criteria for diagnosis of ADHD (2.5 times higher than controls), with a higher proportion of inattentive symptoms. There was no significant difference for hyperactive/impulsive symptoms. Arnold et al. (Reference Arnold, Vladutiu, Orlowski, Blakely and DeLuca2004) showed that medication for attention problems was used by 26% of their sample of children with ECT-PKU (5 times more than controls). No study has examined EFs related to the presence or absence of ADHD in children with ECT-PKU. One study showed that children with ECT-PKU exhibited more impulsivity than children with a diagnosis of ADHD combined type (without PKU) on a Go/No-Go task (Wiersema et al., Reference Wiersema, van Der Meere and Roeyers2005) but this study did not specify whether some of the ECT-PKU participants met the diagnostic criteria for ADHD.
Processing speed
In some studies (Feldmann et al., Reference Feldmann, Denecke, Pietsch, Grenzebach and Weglage2002; Weglage et al., Reference Weglage, Pietsch, Denecke, Sprinz, Feldman, Grenzebach and Ullrich1999), executive difficulties were interpreted as a slower information processing speed. In five studies, processing speed was controlled in studying executive performances (Antshel & Waisbren, Reference Antshel and Waisbren2003a; Christ et al., Reference Christ, Steiner, Grange, Abrams and White2006; Huijbregts et al., Reference Huijbregts, De Sonneville, van Spronsen, Berends, Licht, Verkerk and Sergeant2003; Janos et al., Reference Janos, Grange, Steiner and White2012; White et al., Reference White, Nortz, Mandernach, Huntington and Steiner2001, i.e., 12% of the studies on EFs). In 60% of these studies, executive difficulties were observed when processing speed was controlled.
Information processing speed was poorly explored with specific tasks. Seven studies (13% of the literature) used simple reaction time tasks or tests involving the processing speed index of Wechsler scales. Six out of seven studies (86%) have shown a slower information processing speed (Anderson et al., Reference Anderson, Wood, Francis, Coleman, Warwick, Casanelia, Anderson and Boneh2004, Reference Anderson, Wood, Francis, Coleman, Anderson and Boneh2007; Antshel & Waisbren, Reference Antshel and Waisbren2003a; Huijbregts et al., Reference Huijbregts, De Sonneville, Licht, van Spronsen, Verkerk and Sergeant2002c, Reference Huijbregts, De Sonneville, van Spronsen, Berends, Licht, Verkerk and Sergeant2003; Janos et al., Reference Janos, Grange, Steiner and White2012).
Cognition, Biochemical Markers, and Treatments
Blood phenylalanine level
Currently, blood phenylalanine level is the most widely used measure to monitor metabolic control and adapt treatment. Average phenylalanine levels (i.e., mean or median) have been shown to predict neuropsychological outcome (Waisbren et al., Reference Waisbren, Noel, Fahrbach, Cella, Frame, Dorenbaum and Levy2007). Early initiated and continued dietary treatment is crucial for the intellectual development of patients (see Brumm & Grant, Reference Brumm and Grant2010). Two meta-analyses (Fonnesbeck et al., Reference Fonnesbeck, McPheeters, Krishnaswami, Lindegren and Reimschisel2013; Waisbren et al., Reference Waisbren, Noel, Fahrbach, Cella, Frame, Dorenbaum and Levy2007) on patients with ECT-PKU showed significant correlations between FSIQ and (1) phenylalanine levels during childhood and adolescence (e.g., critical period) and (2) mean lifetime phenylalanine level. Significant but moderate correlations were found between FSIQ and concurrent phenylalanine levels (i.e., within months prior to evaluation or collected at the same day of evaluation). Moreover, Waisbren et al. (Reference Waisbren, Noel, Fahrbach, Cella, Frame, Dorenbaum and Levy2007) suggested safe upper target of blood phenylalanine concentrations between 320 and 420 μmol/L until the age of 12 years. Fonnesbeck et al. (Reference Fonnesbeck, McPheeters, Krishnaswami, Lindegren and Reimschisel2013) indicated that a mean concentration of 400 μmol/L was associated with an increased risk of an FSIQ less than 85. It should be noted that many candidate studies were excluded from these meta-analyses due to incomplete reporting of data or results. Moreover, there is a notable lack of consistent methodologies across studies considered. From a clinical perspective, this provides a basis for being cautious in interpreting measures of these results. Recent researches suggested that phenylalanine levels continue to have a negative impact on FSIQ scores during adulthood (Koch et al., Reference Koch, Burton, Hoganson, Peterson, Rhead, Rouse, Scott, Wolff, Stern, Guttler, Nelson, de la Cruz, Coldwell, Erbe, Geraghty, Shear, Thomas and Azen2002; Waisbren et al., Reference Waisbren, Noel, Fahrbach, Cella, Frame, Dorenbaum and Levy2007), which requires dietary restriction throughout life [for American guidelines, see Camp et al. (Reference Camp, Parisi, Acosta, Berry, Bilder, Blau, Bodamer, Brosco, Brown, Burlina, Burton, Chang, Coates, Cunningham, Dobrowolski, Ferguson, Franklin, Frazier, Grange, Greene, Groft, Harding, Howell, Huntington, Hyatt-Knorr, Jevaji, Levy, Lichter-Konecki, Lindegren, Lloyd-Puryear, Matalon, MacDonald, McPheeters, Mitchell, Mofidi, Moseley, Mueller, Mulberg, Nerurkar, Ogata, Pariser, Prasad, Pridjiann, Rasmussen, Reddy, Rohr, Singh, Sirrs, Stremer, Tagle, Thompson, Urv, Utz, van Spronsen, Vockley, Waisbren, Weglicki, White, Whitley, Wilfond, Yannicelli and Young2014) and Vockley et al. (Reference Vockley, Andersson, Antshel, Braverman, Burton, Frazier, Mitchell, Smith, Thompson and Berry2014); for European guidelines, see van Spronsen et al. (Reference van Spronsen, van Wegberg, Ahring, Bélanger-Quintana, Blau, Bosch, Burlina, Campistol, Feillet, Giżewska, Huijbregts, Kearney, Leuzzi, Maillot, Muntau, Trefz, van Rijn, Walter and MacDonald2017)]. While a causal relationship between blood phenylalanine levels and brain dysfunction is well-demonstrated, a recent longitudinal study showed that disease-independent individual factors may influence the consequences of the biochemical alteration, contributing to an individual vulnerability to phenylalanine and interindividual variability in FSIQ outcome of ECT-PKU (Manti et al., Reference Manti, Nardecchia, Paci, Chiarotti, Carducci, Carducci, Dalmazzone, Cefalo, Salvatici, Banderali and Leuzzi2017).
For the other cognitive abilities, correlations between phenylalanine levels and performances in the tests are inconsistent from one task to another [see Christ et al. (Reference Christ, Huijbregts, De Sonneville and White2010a) for EFs; Janzen & Nguyen (Reference Janzen and Nguyen2010) and Luciana et al. (Reference Luciana, Sullivan and Nelson2001) for nonexecutive cognitive abilities]. However, long-term memory performances were intact when the mean blood phenylalanine concentrations (whether concurrent phenylalanine or lifetime phenylalanine levels) were under 360 μmol/L, in comparison with ECT-PKU children with phenylalanine levels above 360 μmol/L (Leuzzi et al., Reference Leuzzi, Pansini, Sechi, Chiarotti, Carducci, Levi and Antonozzi2004b; Smith et al., Reference Smith, Saltzman, Klim, Hanley, Feigenbaum and Clarke2000). Similar results have been obtained for EFs (De Sonneville et al., Reference De Sonneville, Schmidt, Michel and Batzler1990; Diamond et al., Reference Diamond, Prevor, Callender and Druin1997; Huijbregts et al., Reference Huijbregts, De Sonneville, Licht, van Spronsen, Verkerk and Sergeant2002c, Reference Huijbregts, De Sonneville, van Spronsen, Licht and Sergeant2002d; Smith et al., Reference Smith, Saltzman, Klim, Hanley, Feigenbaum and Clarke2000), in particular in younger children (i.e., under 11 years) (Huijbregts et al., Reference Huijbregts, De Sonneville, Licht, Sergeant and van Spronsen2002a). In a recent study with younger ECT-PKU children (i.e., under 12 years), only those patients with phenylalanine levels under 240 μmol/L (rather than 360 μmol/L) obtained similar executive performances than controls (Jahja et al., Reference Jahja, Huijbregts, De Sonneville, van Der Meere and van Spronsen2014). Longitudinal studies of ECT-PKU showed less executive deficits during adolescence, despite a significant increase of phenylalanine levels, raising the hypothesis of a decrease vulnerability of PKU patients against elevated phenylalanine levels with aging (Nardecchia et al., Reference Nardecchia, Manti, Chiarotti, Carducci, Carducci and Leuzzi2015; Weglage et al., 2009). Only few studies employed within-subject designs where phenylalanine levels are manipulated through dietary changes. Strong effects of phenylalanine manipulation were found on EF performances (Huijbregts et al., Reference Huijbregts, De Sonneville, Licht, van Spronsen and Sergeant2002b) and reaction time (Leuzzi et al., Reference Leuzzi, Mannarelli, Manti, Pauletti, Locuratolo, Carducci, Carducci, Vanacore and Fattapposta2014a), but not on short-term and long-term memory, or fine motor coordination (Griffiths et al., Reference Griffiths, Ward, Harvie and Cockburn1998). Sensitivity to increase of phenylalanine was higher in younger (i.e., under 13 years) as compared to older people with PKU (Leuzzi et al., Reference Leuzzi, Mannarelli, Manti, Pauletti, Locuratolo, Carducci, Carducci, Vanacore and Fattapposta2014a).
Fluctuation in phenylalanine concentrations
Recent studies suggested that variability of blood phenylalanine levels over time appeared to correlate inversely and strongly with FSIQ (Burgard et al., Reference Burgard, Schmidt, Rupp, Schneider and Bremer1996; Hood et al., Reference Hood, Grange, Christ, Steiner and White2014; Vilaseca et al., Reference Vilaseca, Lambruschini, Gómez-López, Gutiérrez, Fusté, Gassió, Artuch and Campistol2010) and with executive performances (Arnold et al., Reference Arnold, Kramer, Kirby, Plumeau, Blakely, Cregan and Davidson1998; Hood et al., Reference Hood, Grange, Christ, Steiner and White2014; Jahja et al., Reference Jahja, Huijbregts, De Sonneville, van Der Meere and van Spronsen2014). However, in some studies, the correlation between fluctuation in phenylalanine and FSIQ was at the trend level of significance (Anastasoaie et al., Reference Anastasoaie, Kurzius, Forbes and Waisbren2008) or was not significant (Viau et al., Reference Viau, Wengreen, Ernst, Cantor, Furtado and Longo2011). Different indices of variability in phenylalanine have been used in the literature: SD (standard deviation, degree of dispersion in phenylalanine around a regression line), SEE (standard error of estimate, residual variation in phenylalanine around a regression line), and Spikes (number of phenylalanine levels that were at least 600 μmol/L greater than either the preceding or succeeding phenylalanine level). Interpretation and comparison of findings were limited by inconsistencies in tasks and indices of variability used across the studies.
Phenylalanine-to-tyrosine ratios
PAH deficiency causes increase in blood phenylalanine, decrease in tyrosine, and therefore theoretically an increase in phenylalanine-tyrosine ratio. A high phenylalanine-tyrosine ratio indicates that phenylalanine levels are in a range that could interfere with the transport of available tyrosine across the blood–brain barrier, resulting in a decreased availability of tyrosine for dopamine synthesis. Therefore, phenylalanine-tyrosine ratio might be of interest considering the fact that tyrosine levels are more closely related to dopamine levels than phenylalanine levels (Diamond et al., Reference Diamond, Prevor, Callender and Druin1997). Clinical relevance of this ratio as an outcome measure is unknown. Recent studies suggested that high lifetime phenylalanine-tyrosine ratios (Diamond et al., Reference Diamond, Prevor, Callender and Druin1997; Jahja et al., Reference Jahja, Huijbregts, De Sonneville, van Der Meere and van Spronsen2014; Luciana et al., Reference Luciana, Sullivan and Nelson2001; Sharman et al., Reference Sharman, Sullivan, Young and McGill2009a, Reference Sharman, Sullivan, Young and McGill2009b, Reference Sharman, Sullivan, Young and McGill2015) and high concurrent phenylalanine-tyrosine ratios (Jahja et al., Reference Jahja, Huijbregts, De Sonneville, van Der Meere and van Spronsen2014; Luciana et al., Reference Luciana, Sullivan and Nelson2001) could explain executive impairments in children with ECT-PKU. Currently, it is difficult to determine the threshold above which a high phenylalanine-tyrosine ratio is harmful.
Nutritional status
Even if it is not clear that PKU leads to a specific nutrient deficiency (Camp et al., Reference Camp, Parisi, Acosta, Berry, Bilder, Blau, Bodamer, Brosco, Brown, Burlina, Burton, Chang, Coates, Cunningham, Dobrowolski, Ferguson, Franklin, Frazier, Grange, Greene, Groft, Harding, Howell, Huntington, Hyatt-Knorr, Jevaji, Levy, Lichter-Konecki, Lindegren, Lloyd-Puryear, Matalon, MacDonald, McPheeters, Mitchell, Mofidi, Moseley, Mueller, Mulberg, Nerurkar, Ogata, Pariser, Prasad, Pridjiann, Rasmussen, Reddy, Rohr, Singh, Sirrs, Stremer, Tagle, Thompson, Urv, Utz, van Spronsen, Vockley, Waisbren, Weglicki, White, Whitley, Wilfond, Yannicelli and Young2014), dietary treatment of PKU has been associated with deficiencies in several antioxidant vitamins and cofactors, such as selenium and coenzyme Q10 (Artuch et al., Reference Artuch, Colomé, Sierra, Brandi, Lambruschini, Campistol, Ugarte and Vilaseca2004; Colomé et al., Reference Colomé, Artuch, Vilaseca, Sierra, Brandi, Lambruschini, Cambra and Campistol2003; Lombeck et al., Reference Lombeck, Jochum and Terwolbeck1996; Przyrembel & Bremer, Reference Przyrembel and Bremer2000; van Bakel et al., Reference van Bakel, Printzen, Wermuth and Wiesmann2000). Many nutrients have roles in cognition. For example, selenium status has been linked to attention performances in children with ECT-PKU, but not with FSIQ, verbal learning, fine motor, spatial, and executive tasks (Gassió et al., Reference Gassió, Artuch, Vilaseca, Fusté, Colome and Campistol2008). Currently, there is a paucity of studies on nutritional status and its role as a determinant of neuropsychological outcome.
Tetrahydrobiopterin or sapropterin therapy
The tetrahydrobiopterin cofactor is essential for PAH activity (Erlandsen & Stevens, Reference Erlandsen and Stevens2001). Until recently, the main treatment for PKU was phenylalanine-restricted diet. Since 2008, an additional synthetic analogue to tetrahydrobiopterin has been developed (Burton et al., Reference Burton, Grange, Milanowski, Vockley, Feillet, Crombez, Abadie, Cederbaum, Dobbelaere, Smith and Dorenbaum2007; Levy et al., Reference Levy, Milanowski, Chakrapani, Cleary, Lee, Trefz, Whitley, Feillet, Feigenbaum, Bebchuk, Christ-Schmidt and Dorenbaum2007). A subgroup of patients has been shown to respond to treatment with tetrahydrobiopterin. The frequency of tetrahydrobiopterin responsiveness varies, depending on genotype, severity of PKU, and residual PAH activity (Heintz et al., Reference Heintz, Cotton and Blau2013; Karacic et al., Reference Karačić, Meili, Sarnavka, Heintz, Thöny, Ramadža, Fumić, Mardeşić, Barié and Blau2009; Zhang et al., Reference Zhang, Ye, Qiu, Han and Gu2005). Significant decrease in blood phenylalanine levels were seen in children with ECT-PKU following oral administration of tetrahydrobiopterin (Fiege & Blau, Reference Fiege and Blau2007; Leuret et al., Reference Leuret, Barth, Kuster, Eyer, De Parscau, Odent, Gilbert-Dussardier, Feillet and Labarthe2012; Longo et al., Reference Longo, Siriwardena, Feigenbaum, Dimmock, Burton, Stockler, Waisbren, Lang, Jurecki, Zhang and Prasad2015). Moreover, sapropterin may increase stability in blood phenylalanine levels (Burton et al., Reference Burton, Bausell, Katz, LaDuca and Sullivan2010) and could play a role in microstructural white matter integrity as an evidence using diffusion tensor imaging (White et al., Reference White, Antenor-Dorsey, Grange, Hershey, Rutlin, Shimony, McKinstry and Christ2013). Few studies regarding neuropsychological outcomes of children with ECT-PKU with tetrahydrobiopterin treatment have been conducted. In tetrahydrobiopterin-responsive children with PKU and ADHD symptoms, sapropterin treatment resulted in significant improvement of ADHD inattentive symptoms on the ADHD-Rating Scale (but not on ADHD hyperactivity/impulsivity scores) and on metacognition score of parental BRIEF inventory (but not on behavioral regulation score). These improvements were maintained throughout 26 weeks of treatment (Burton et al., Reference Burton, Grant, Feigenbaum, Singh, Hendren, Siriwardena, Phillips, Sanchez-Valle, Waisbren, Gillis, Prasad, Merilainen, Lang, Zhang, Yu and Stahl2015). In a mixed sample of 12 tetrahydrobiopterin-responsive children and adults with PKU, tetrahydrobiopterin treatment was associated with improvements in working memory n-back task performances after 6 months of treatment. Younger participants with PKU showed greater gains in working memory performance (Christ et al., Reference Christ, Moffitt, Peck and White2013). The first studies comparing ECT-PKU group of children and adolescents treated by tetrahydrobiopterin- versus dietary-treatment did not show significant difference on FSIQ, visual–spatial, fine motor functions, and EFs (Gassió et al., Reference Gassió, Vilaseca, Lambruschini, Boix, Fusté and Campistol2010), or social cognition (Jahja et al., Reference Jahja, van Spronsen, De Sonneville, van der Meere, Bosch, Hollak, Rubio-Gozalbo, Brouwers, Hofstede, de Vries, Janssen, van der Ploeg, Langendonk and Huijbregts2016).
Large neutral amino acid supplements
LAT1, the large neutral amino acid (LNAA) transporter 1, is the predominant transport system for all LNAAs at the blood–brain barrier (Smith, Reference Smith2000). LAT1 shows a high affinity to phenylalanine. As a consequence, brain phenylalanine in PKU is increased and other LNAA concentrations decreased (Pietz et al., Reference Pietz, Kreis, Rupp, Mayatepek, Rating, Boesch and Bremer1999). Therefore, reduced non-phenylalanine LNAAs may possibly impair cerebral neurotransmitter and/or protein synthesis (Pardridge, Reference Pardridge1998; Surtees & Blau, Reference Surtees and Blau2000). LNAA supplementation (excluding phenylalanine) has been used on a limited number of patients with PKU. phenylalanine-free LNAA supplements were shown to potentially have some effect on decreasing blood phenylalanine concentrations (Matalon et al., Reference Matalon, Michals-Matalon, Bhatia, Grechanina, Novikov, McDonald, Grady, Tyring and Guttler2006) but this effectiveness was not consistent nor predictable (Matalon et al., Reference Matalon, Michals-Matalon, Bhatia, Burlina, Burlina, Braga, Fiori, Giovannini, Grechanina, Novikov, Grady, Tyring and Guttler2007). Restoring reduced brain LNAA concentrations in patients with PKU may improve neuropsychological outcome. The clinical significance of reduced non-phenylalanine LNAAs in PKU patients has not been fully elucidated. The use of LNAAs may be beneficial in terms of attention and EFs to adolescents and young adults with elevated blood phenylalanine levels who have stopped or do not follow dietary treatment guidelines (Berry et al., Reference Berry, Brunner, Hunt and White1990; Schindeler et al., Reference Schindeler, Ghosh-Jerath, Thompson, Rocca, Joy, Kemp, Rae, Green, Wilcken and Christodoulou2007). However, optimal composition and dose of LNAA treatment is currently unknown, limiting its clinical application. LNAA is contraindicated in young children due to a lack of data on safety and efficacy.
SYNTHESIS, LIMITS, AND PROSPECTIVE
The neuropsychological profile of children with ECT-PKU has been studied across many cognitive domains (for a synthesis, see Figure 2). Consistent with previous reviews, the hypothesis of a potentially specific and central executive impairment in children with ECT-PKU was suggested. Although many studies have found neuropsychological deficits, general consensus has yet to be reached with regard to the nature and the severity of these impairments, their pathogenesis, or their impacts on everyday life. This may stem in part from methodological variations across studies. In this context, several issues stand out and new research opportunities can be proposed (for a synthesis, see key recommendations in Table 4).
A Systematic Analysis of Intellectual Functioning
FSIQ score is indicative of global functional outcome, the final common path of an individual’s gene, biology, cognition, education, and experience (Dennis et al., Reference Dennis, Francis, Cirino, Schachar, Barnes and Fletcher2009). Our review indicates that the intelligence of children with ECT-PKU is usually in the average range, although it can be potentially somewhat lower than the intelligence of demographically and/or age-matched controls (see Figure 2). Research that includes genetically related controls such as unaffected parents and siblings is recommended.
A majority of studies that identify a slight intellectual downward shift in FSIQ used full intelligence scales (compared to abbreviated forms of these scales or g factor tests). In children with ECT-PKU, only a few studies addressed the issue of the difference between verbal and perceptual reasoning. A systematic analysis of these verbal/nonverbal discrepancies may lead to a more refined understanding of the slight downward shift in FSIQ. That said, impairments in EFs, processing speed, and nonverbal functions seem to be the main characteristics of the cognitive profile of children with ECT-PKU (see Figure 2).
Because intelligence tests measure multiple and correlated abilities, it is misguided and generally unjustified to attempt to control for FSIQ differences by matching procedures or by using FSIQ scores as covariates in children with neurodevelopmental disorders (Dennis et al., Reference Dennis, Francis, Cirino, Schachar, Barnes and Fletcher2009). In 41% of the studies, intellectual level was measured in order to compare specific cognitive measures between individuals with the same intellectual level. Future research will need to address these issues of controls and covariates in studying the neuropsychological profile of children with ECT-PKU.
A Comprehensive Examination of Executive Functioning
“Cool EFs” are identified as areas of weakness for children with ECT-PKU (see Figure 2). Some discrepancies in the literature may be related to variations in psychometric properties of tasks or modalities for measurement. Multiple tests of the same executive process (planning, inhibition, shifting, and working memory), within a developmental and conceptual framework of EFs (e.g., Anderson, Reference Anderson2002; Dennis, Reference Dennis, Risberg and Grafman2006; Diamond, Reference Diamond2013), could strengthen findings. There is some recent evidence that executive aspects involved in the emotional, affective, and motivational contexts were impaired (Jahja et al., Reference Jahja, van Spronsen, De Sonneville, van der Meere, Bosch, Hollak, Rubio-Gozalbo, Brouwers, Hofstede, de Vries, Janssen, van der Ploeg, Langendonk and Huijbregts2016), but more systematic research on “hot EFs” is needed to support this. From a clinical point of view, the evaluation of the EFs on everyday life constitutes an essential quality of life index in addition to the laboratory tests (for a review, see Chevignard et al., Reference Chevignard, Soo, Galvin, Catroppa and Eren2012). Behavioral inventories, such as the BRIEF, are often useful tools for EF assessments in individuals with PKU (Huijbregts et al., Reference Huijbregts, Gassió and Campistol2013; van Spronsen et al., Reference van Spronsen, Huijbregts, Bosch and Leuzzi2011). To date, the ratings have been based exclusively on parents’ reports. Recommendations for future studies are to include teachers’ ratings, as well as a systematic analysis of rating scale index scores (e.g., behavioral regulation vs. metacognition). Comparisons with performances on “laboratory tasks” are needed because of their potentially complementary nature (Gioia et al., Reference Gioia, Kenworthy and Isquith2010; McCandless & O’ Laughlin, Reference McCandless and O’Laughlin2007; Toplak et al., Reference Toplak, Bucciarelli, Jain and Tannock2008).
Another finding from a limited number of studies was that executive impairment tended to persist when intellectual level, nonexecutive abilities, and processing speed were controlled. An important challenge for further investigations will be to devise EF assessment protocols that examine quantitative (e.g., number of errors and latency) and qualitative (e.g., fluctuation of performances) aspects of performance, as well as cognitive-process (e.g., strategies) scores in EF performances (Anderson, Reference Anderson2002).
Stevenson and McNaughton (Reference Stevenson and McNaughton2013) suggested that a subset of individuals with ECT-PKU and individuals with ADHD (without PKU) have overlapping dysfunction in cognitive inhibition. If symptoms are similar between these two clinical groups, medication utilized for ADHD could be an additional effective treatment to reduce ADHD-related cognitive and behavioral symptoms in PKU patients. To date, no study has provided a systematic analysis of the links between ADHD and PKU. Further research comparing EFs of children with ECT-PKU with and without ADHD phenotype to children with ADHD without PKU is needed to better understand the pathogenesis of the observed impairments.
An Integrative Approach of Other Cognitive Impairments
Because of the significant overlap between EFs and memory functions, learning difficulties may appear when there are significant executive strategy demands (Janzen & Nguyen, Reference Janzen and Nguyen2010). No study has systematically examined distinct long-term memory processes (i.e., encoding, storage, and retrieval). Moreover, there may be other cognitive aspects involved in memory test performance such as language and visual–spatial processing. Futures studies are needed to understand the interrelationships between learning, EFs, language, and nonverbal abilities.
Children with ECT-PKU seemed to show more deficits in visual construction than in visual–spatial functions. The ROCF was most often used to examine visual construction in children with ECT-PKU. Although there are various abilities involved in this test including visual–spatial processing and EFs, lower ROCF performance may stem from deficits in action planning rather than to visual–spatial deficits per se (Roy et al., Reference Roy, Roulin, Charbonnier, Allain, Fasotti, Barbarot, Stadler, Terrien and Le Gall2010). The nature of nonverbal impairments in children with ECT-PKU needs to be closely examined with assessments that distinguish between specific visual–spatial and visual construction demands, as well as EFs and fine motor skills.
A Neuropsychological Approach of Academic Performance
Language and academic achievement have not been well studied in children with ECT-PKU. Limited studies have identified widespread academic difficulties, consistent with previous review (Janzen & Nguyen, Reference Janzen and Nguyen2010). Overall, it appeared that children with ECT-PKU tend to display substantial deficits in mathematics, more than in written language (see Figure 2). Further studies should systematically investigate each component of written language (e.g., phonology, syntax, and semantics) and mathematics (e.g., calculations and word problems) in order to understand which domains are difficult for children with ECT-PKU.
Moreover, the links between academic performance and cognitive domains need to be examined. As with other neurodevelopmental disorders such as ADHD, autism, or Neurofibromatosis type 1 (Roy et al., Reference Roy, Le Gall, Roulin and Fournet2012), the hypothesis of impairment in EFs and prefrontal network functioning can offer new paradigms for understanding academic difficulties. Executive impairments can adversely affect learning abilities in general and mathematics in particular (Bull et al., Reference Bull, Espy and Wiebe2008).
A Systematic Study of Biochemical Markers and Treatments, in Relation to Neuropsychological Outcome
The phenylalanine concentration is the crucial factor to adjust treatments. However, other parameters such as fluctuation of phenylalanine levels, phenylalanine-tyrosine ratio, and nutritional components (such as selenium) seemed to be correlated with cognitive impairments. These other markers could be interesting in addition to blood phenylalanine measurements but the advantages of these markers still have to be determined.
The goal of the therapy should be to lower blood phenylalanine enough to reach optimal neuropsychological and quality of life outcome, without negative consequences. Dietary compliance remains the cornerstone of the therapy to control phenylalanine levels and to reach suboptimal neuropsychological outcomes. However, the phenylalanine-restricted diet is socially demanding and hard to comply with (MacDonald, Reference MacDonald2000). Starting in adolescence, 60%–80% of the patients were shown to have partially or totally disrupted the treatment (dos Santos et al., Reference dos Santos, de Castro Magalhães, Januário, de Aguiar and Carvalho2006), resulting in a risk of neuropsychological impairment. Other treatments might directly affect cerebral metabolism, increase dietary tolerance, and allow dietary expansion such as sapropterin (for responsive patients) and LNAA (without phenylalanine). Studies with these other treatments are still in their early stages and additional studies are needed to confirm safety and efficacy on patients’ neuropsychological outcome.
Our understanding of the optimal therapies for PKU is still incomplete. Although many studies suggest a primary neurobiological explanation for neuropsychological problems in children with ECT-PKU, psychosocial and quality of life cannot be ignored. In particular, the stress associated with this chronic disorder is likely to play a role in the increased neuropsychological symptoms among children and adolescents with PKU (Weglage et al., Reference Weglage, Grenzebach, Pietsch, Feldmann, Linnenbank, Denecke and Koch2000).
A Developmental Perspective in Etiology-Related Profiles
A number of longitudinal studies suggest that the severity of visual perception, fine motor, and executive disorders may decrease with age, contrasting with stability of FSIQ and slower processing speed with age (Feldmann et al., Reference Feldmann, Denecke, Grenzebach and Weglage2005; Michel et al., Reference Michel, Schmidt and Batzler1990; Nardecchia et al., Reference Nardecchia, Manti, Chiarotti, Carducci, Carducci and Leuzzi2015; Weglage et al., Reference Weglage, Pietsch, Denecke, Sprinz, Feldman, Grenzebach and Ullrich1999). Findings suggest the value of longitudinal study of the age-related course of neuropsychological profiles of children with ECT-PKU, in relation with metabolic control. The hypothesis of an individual resilience or vulnerability to phenylalanine with age has been a scarcely studied topic and deserves further investigations (Manti et al., Reference Manti, Nardecchia, Paci, Chiarotti, Carducci, Carducci, Dalmazzone, Cefalo, Salvatici, Banderali and Leuzzi2017; Nardecchia et al., Reference Nardecchia, Manti, Chiarotti, Carducci, Carducci and Leuzzi2015). Currently, individual factors that account for the vulnerability to phenylalanine have not been identified.
Another challenge in studying the neuropsychology of ECT-PKU is to examine status in relation to metabolic control. Phenylalanine concentrations have strong effects on cognitive performance. Notwithstanding the positive effects of decreasing blood phenylalanine levels, the paucity of studies makes it difficult to reach an international consensus about the phenylalanine-level upper target for optimal development. European guidelines set a phenylalanine blood concentration of 360 μmol/L as the upper target for the first 12 years of life and 600 μmol/L for older children (van Spronsen et al., Reference van Spronsen, van Wegberg, Ahring, Bélanger-Quintana, Blau, Bosch, Burlina, Campistol, Feillet, Giżewska, Huijbregts, Kearney, Leuzzi, Maillot, Muntau, Trefz, van Rijn, Walter and MacDonald2017). American guidelines advise 360 μmol/L throughout life (Camp et al., Reference Camp, Parisi, Acosta, Berry, Bilder, Blau, Bodamer, Brosco, Brown, Burlina, Burton, Chang, Coates, Cunningham, Dobrowolski, Ferguson, Franklin, Frazier, Grange, Greene, Groft, Harding, Howell, Huntington, Hyatt-Knorr, Jevaji, Levy, Lichter-Konecki, Lindegren, Lloyd-Puryear, Matalon, MacDonald, McPheeters, Mitchell, Mofidi, Moseley, Mueller, Mulberg, Nerurkar, Ogata, Pariser, Prasad, Pridjiann, Rasmussen, Reddy, Rohr, Singh, Sirrs, Stremer, Tagle, Thompson, Urv, Utz, van Spronsen, Vockley, Waisbren, Weglicki, White, Whitley, Wilfond, Yannicelli and Young2014; Vockley et al., Reference Vockley, Andersson, Antshel, Braverman, Burton, Frazier, Mitchell, Smith, Thompson and Berry2014). Thus, it might be important to study neuropsychological outcomes of patients with different age and different upper of phenylalanine levels (e.g., ≤240, 240–360, and ≥360 µmol/L). In particular, data about adolescents and adults are scarce. Further data collection within long-term international collaborative studies is needed.
CONCLUSION
Similar to other reviews on the neuropsychological profile of individuals with ECT-PKU, this review suggested a potentially specific and central executive impairment in children with ECT-PKU. However, data are limited or inconsistent with regard to the nature and the severity of the impairments, their persistence over time, their impact on everyday life, and their pathogenesis. Neuropsychological status and outcome remain critically important in determining the threshold phenylalanine level and in medical decision-making for PKU patients. In this context, this review highlights a number of future clinical and research directions. It would be important to use an integrative developmental approach to neuropsychological research with this population. Considering EFs, we recommend a conceptual and longitudinal approach, in particular to incorporate processing speed into the study of EF status and developmental trajectories. Secondly, future investigations of ECT-PKU could focus the complex interrelationships between neuropsychological domains, academic skills, and ADHD symptoms. Finally, as phenylalanine concentrations have strong effects on neuropsychological performances, systematic and additional studies are needed to determine how new treatments that address metabolic control affect neuropsychological outcome and the quality of life of children with ECT-PKU.
SUPPLEMENTARY MATERIAL
To view supplementary material for this article, please visit https://doi.org/10.1017/S1355617719000146
ACKNOWLEDGEMENTS
The authors have no financial interest or conflict of interest to declare.