Introduction
Numerous host features affect parasite abundance, including body size, host behaviour, the density or size of the host population, and the host immune response (Poulin, Reference Poulin2007). Epidemiological models predict that density-dependent transmission has a principal influence on abundance of directly transmitted parasites (Anderson and May, Reference Anderson and May1979, Reference Anderson and May1991). Furthermore, parasite population dynamics have been related to host density among a number of helminths (Krkošek, Reference Krkošek2010). Host density has been associated with abundance of directly transmitted strongylid nematodes both within and across mammalian species (Arneberg et al., Reference Arneberg, Skorping, Grenfell and Read1998) and the indirectly transmitted tapeworm (Anoplocephala gigantea) across 18 populations of black rhinoceros (Diceros bicornis) (see Stringer and Linklater, Reference Stringer and Linklater2015). Yet, studies examining the relationship between host density and abundance of helminths are relatively rare (but see Krkošek (Reference Krkošek2010) for other examples), and theory does not predict a simple relationship between them, due to temporal variation that results in complex host–parasite dynamics (Arneberg et al., Reference Arneberg, Skorping, Grenfell and Read1998). Furthermore, the complexities of many helminth life cycles make them difficult to examine experimentally.
Ever since the pioneering work of Scott (Reference Scott1982), the guppy (Poecilia reticulata)-Gyrodactylus spp. (Monogenea) host–parasite system has been the subject of numerous investigations examining the effects of host factors on the population dynamics of a helminth parasite (Scott and Anderson, Reference Scott and Anderson1984; Scott, Reference Scott1985a, Reference Scott, Rollinson and Anderson1985b; Harris, Reference Harris1988, Reference Harris1989; Johnson et al., Reference Johnson, Lafferty, Van Oosterhout and Cable2011; Richards et al., Reference Richards, van Oosterhout and Cable2012). Various other studies made use of different fish–gyrodactylid systems to examine different aspects of parasite population biology. The salmon (Salmo salar)–Gyrodactylus salaris host–parasite system was used to determine parameters of parasite population growth (Cable et al., Reference Cable, Harris and Bakke2000). The three-spined stickleback (Gasterosteus aculeatus)–Gyrodactylus gasterostei system was used to describe the effects of detachment and feeding on embryo development and survival (Cable et al., Reference Cable, Tinsley and Harris2002b). In addition, the goldfish (Carassius auratus)–Gyrodactylus kobayashii was used to evaluate the effect of host population size on parasite transmission (Zhou et al., Reference Zhou, Zou, Wu, Wang, Marcogliese and Li2017). The ease of manipulation and quantification of this fish–helminth system makes it ideal to experimentally examine questions related to the population dynamics and transmission of parasites, especially under confined conditions such as aquaculture.
The viviparous reproduction, where gyrodactylids proliferate directly on the host without any free-living developmental stages or intermediate hosts, and short generation time can lead to rapid population growth of these parasites (Scott, Reference Scott1982; Scott and Anderson, Reference Scott and Anderson1984). Indeed, the life history characteristics of Gyrodactylus spp., their overall pathogenicity, asexual and sexual reproduction (Harris, Reference Harris1989; Cable and Harris, Reference Cable and Harris2002), and the induction of acquired resistance by the host are more similar to microparasites (e.g., bacteria, protozoans) than they are to helminth macroparasites (Tadiri et al., Reference Tadiri, Kong, Fussmann, Scott and Wang2019), and Scott (Reference Scott1982) makes the point that gyrodactylids do not conveniently fit in either category. However, it is precisely these characteristics which render them a suitable model to examine disease dynamics in a helminth parasite.
Transmission of gyrodactylids mainly depends on contacts among fish hosts (Bakke et al., Reference Bakke, Harris, Jansen and Hansen1992; Tadiri et al., Reference Tadiri, Kong, Fussmann, Scott and Wang2019). Attachment on the body surface of fish makes it feasible to monitor the presence of gyrodactylids in a non-lethal manner (Harris, Reference Harris1988). Numerous studies have made use of the guppy-Gyrodactylus laboratory model to examine effects of parasite density, host immune response, challenge infections, population connectivity, parasite aggregation, host social behaviour, and pollution on parasite population dynamics (Scott, Reference Scott1982, Reference Scott1985a, Reference Scott, Rollinson and Anderson1985b; Scott and Anderson, Reference Scott and Anderson1984; Scott and Robinson, Reference Scott and Robinson1984; Gheorgiu et al., Reference Gheorgiu, Marcogliese and Scott2006; Richards et al., Reference Richards, van Oosterhout and Cable2010; Johnson et al., Reference Johnson, Lafferty, Van Oosterhout and Cable2011; Tadiri et al., Reference Tadiri, Scott and Fussmann2018). Given the importance of host density in epidemiological models of parasite transmission (Anderson and May, Reference Anderson and May1979; McCallum et al., Reference McCallum, Barlow and Hone2001), another study demonstrated that population size of Gyrodactylus turnbulli increased with guppy density under laboratory conditions, although the occurrence of epidemics was independent of host density (Johnson et al., Reference Johnson, Lafferty, Van Oosterhout and Cable2011). However, other than this latter work, no study has directly addressed the effects of host density on population dynamics of a gyrodactylid on fish.
In addition to the most important transmission pathway by host–host contact, contact between hosts and detached gyrodactylids is also an important transmission route (Bakke et al., Reference Bakke, Harris, Jansen and Hansen1992; Cable et al., Reference Cable, Scott, Tinsley and Harris2002a). Gyrodactylids may become detached from their hosts as a result of host immune response (Lester, Reference Lester1972; Scott and Anderson, Reference Scott and Anderson1984), host death (Cable et al., Reference Cable, Scott, Tinsley and Harris2002a), or during the host–host contact and locomotion on the host (Bakke et al., Reference Bakke, Cable and Harris2007).
Gyrodactylus kobayashii is the dominant species on fins, scales and gills of the goldfish (Li et al., Reference Li, Li, Wu and Wang2014). The parasite requires three to four weeks to reach maximum mean abundance in a host population under experimental conditions (Zhou et al., Reference Zhou, Zou, Wu, Wang, Marcogliese and Li2017). Here, the goldfish-G. kobayashii model was used to investigate effects of host density on population growth of gyrodactylids in an experimental model system and the role of detached parasites in transmission. We predicted (1) that transmission of G. kobayashii to goldfish would be density-dependent, and (2) that detached parasites could successfully infect fish in this model system.
Materials and methods
Preparation of fish and establishment of infection mode
Goldfish were collected from a fish farm in Wuhan, China, and stocked at density of 3 fish L−1 in several 100 L aquaria for two weeks. To obtain gyrodactylid-free goldfish, all fish were treated in three consecutive baths of 1:10 000 formalin solution for 12 h at 48-h intervals. After 30 days of feeding, 10 treated goldfish were randomly selected, anaesthetized with 0.02% MS-222 (tricaine methane sulphonate) and examined with a dissecting microscope to confirm their gyrodactylid-free status. Specimens of G. kobayashii, which was identified as Li et al. (Reference Li, Li, Wu and Wang2014), then were inoculated onto the caudal fin of anaesthetized goldfish to initiate a stock source of parasites. To obtain more specimens of G. kobayashii for the experiment, some gyrodactylid-free goldfish were regularly introduced to the aquarium with infected goldfish (Zhou et al., Reference Zhou, Zou, Wu, Wang, Marcogliese and Li2017).
Effect of host density on infection
Gyrodactylid-free immature goldfish with a mean standard length 4.9 ± 0.4 cm were randomly assigned to five groups, each containing 12 fish to maintain the same population size. To obtain host densities of 2, 1, 0.5, 0.25 and 0.125 fish L−1 (Table 1), five different sizes of aquaria were used: 20 × 28 × 11 cm, 29 × 28 × 15 cm, 58 × 28 × 15 cm, 46 × 36 × 29 cm and 67 × 44 × 33 cm. If goldfish died during the experiment, the dead fish were left in the aquarium for 12 h, and then replaced with gyrodactylid-free goldfish (not included in the data analysis). A single goldfish in each aquarium was chosen as the primary fish (donor fish) for parasite inoculation. The donor fish was anaesthetized with 0.02% MS-222 and placed in a Petri dish. Using a dissecting microscope, each donor fish was inoculated with five gravid G. kobayashii on the caudal fin, and then returned to its original aquarium. Among the head, gills, body surface, pectoral fin, ventral fin, anal fin, dorsal fin and caudal fin of goldfish, the highest proportion with 37.0% was found on the caudal fin, and the proportion changed little during different infection periods (Zhou, Reference Zhou2018). To ensure consistent and repeatable parasite observation on the same fish and minimize handling, G. kobayashii was screened non-invasively only on caudal fins of goldfish. On each sampling day, each fish was examined for G. kobayashii using a dissecting microscope to minimize handling after anaesthetization with 0.02% MS-222. These fish subsequently were returned to their own aquarium for further parasite screening. Fish were maintained in dechlorinated tap water at 20 ± 1°C and 12 h light–dark cycle, and fed with commercial pellet feed at 2% of the estimated total fish biomass every day. The water was stirred by an aeration device during the entire experiment to prevent sedimentation of food particles and provide oxygenation. Faeces and uneaten feed were removed regularly, and one-third of the water was changed every 3 days. The fish were examined every two days during the first month, and every six days during the second month. The experiment was run for 58 days.
Table 1. Total mean abundance and mean prevalence of Gyrodactylus kobayashii on caudal fin of goldfish (Carassius auratus) at five densities (0.125, 0.25, 0.5, 1, and 2 fish L−1) maintained the same population size of 12 over the 58-day experiment

N, number of replicates; TP, the total number of G. kobayashii examined on caudal fin of goldfish; s.d., standard deviation.
Fitting of the exponential model for population growth
Intrinsic population growth of gyrodactylids was determined on individual, isolated goldfish. More than 100 gyrodactylid-free goldfish were stocked at density of 3 fish L−1 under the same laboratory conditions as above to ensure availability of enough infected fish for the experiment. On day 0, each fish was anaesthetized and inoculated with five gravid G. kobayashii on the caudal fin, then stocked separately in a 20 × 12 × 10 cm tank containing 0.5 L dechlorinated water. To record all the gyrodactylids, G. kobayashii was examined on the gills, fins and body surface of each goldfish. 7–10 fish were killed after anaesthetization and gills were removed for parasitological examination each day. To reduce effects of the host immune response (Zhou et al. Reference Zhou, Li, Zou, Zhang, Wu, Li and Wang2018), the experiment was completed on day 9. A total of 85 goldfish was used in the experiment.
An exponential-growth model (Pt = P 0 × ert) was used to fit the population growth of gyrodactylids, where Pt is the parasite population size on all goldfish in a tank on a sampling day, P 0 is the initial population size and r is the population growth rate of gyrodactylids (Scott, Reference Scott1982; Scott and Anderson, Reference Scott and Anderson1984). The data used for model fitting included both the parasite number on the caudal fin of the isolated goldfish over 9 days and the goldfish used in the host-density experiment. A previous study showed that mean abundance on goldfish declined between 21 and 28 days, indicating the immune response is causing the death rate to exceed the birth rate (Zhou et al., Reference Zhou, Li, Zou, Zhang, Wu, Li and Wang2018). Furthermore, given that mean abundance first peaked at 24 days before declining at a density of 0.5 fish L−1, only the data from day 0 to day 24 in the host-density experiment were used in the analysis of population growth of G. kobayashii to reduce the effects of host resistance. Intrinsic population growth rate of G. kobayashii was also estimated by the exponential-growth model using the parasite numbers on the gills, body surface and fins of the isolated goldfish over 9 days. Data analysis was performed by MATLAB®.
Reinfection of detached gyrodactylids
To test whether detached G. kobayashii could reattach on goldfish and if the reinfection was associated with host density, a cage (10 × 10 × 10 cm) with a single goldfish infected with 20 gyrodactylids on the caudal fin was placed in the upper water of an aquarium (50 × 36 × 29 cm) containing 11 gyrodactylid-free goldfish. The cage was covered with double plastic mesh (1 cm) to avoid contact between the goldfish inside and outside of the cage. Two densities (0.25 and 1 fish L−1) of goldfish were used by controlling water volume (46 × 36 × 29 and 11 × 36 × 29 cm). The goldfish were maintained as described above. Parasites were counted on the caudal fin of each goldfish after anaesthetization, and each fish was returned to its own aquarium for subsequent parasitological examination later. The sampling was performed on days 1, 3, 5 and 7.
To determine effects of detachment time on survival and infection ability of G. kobayashii, gyrodactylids were detached from the caudal fin using a vortex instrument with 1000 r/min within 30 s. A preliminary experiment established that about 90% gyrodactylids maintain infectivity, which are higher than those collected by fine tweezers. A single gyrodactylid was put in a 6-well plate with dechlorinated water, and 150–300 worms were included in each group. The parasites were examined when detached for 0 (control group), 3, 12, and 24 hours. Worms with visible body movement were considered to be alive and recorded. Then three living worms were inoculated onto the caudal fin of each goldfish with 12–14 replicates. If a gyrodactylid was detected on the gills and body surface of goldfish which was killed after anaesthetization on day 3, the living worm was considered to be infective. Then the percentages of survival and successful infection were calculated.
Statistical analysis
Prevalence (the percentage of the goldfish population infected with G. Kobayashii excluding the donor fish) and mean abundance (the average number of G. kobayashii per fish, including uninfected fish) were calculated on each sampling day (Bush et al., Reference Bush, Lafferty, Lotz and Shostak1997). Mean prevalence and total mean abundance were also calculated throughout the 58-day experiment (average value of all the sampling days).
The relationship between host density and mean abundance of gyrodactylids was analysed using the Pearson correlation coefficient. Linear mixed-effects models (LMM) were used to analyse effects of host density and infection time on mean abundance of parasites (following log-transformation log[x + 1]) using the R package nlme (R Core Team, 2017). In all models, the aquarium was used as a random factor, and host density and infection time were the explanatory variables. The models were compared by the Akaike's information criterion (AIC) using the ‘anova’ function in nlme package and the model with the lowest AIC was deemed the best-fit model for the data. The P values for the variables in all models were also computed by the ‘anova’ function. Multiple comparisons were performed using least significant differences (LSD). Differences in prevalence were tested using the chi-square test among different host densities. In the detachment experiment, differences in mean abundance of G. kobayashii were assessed using the independent samples t-test between the two host densities. Statistical analysis was performed by the software Statistics 10.0 (StatSoft, Inc.). In all cases, the level of significance was set at P < 0.05.
Results
Effect of host density on transmission
Throughout the experiment, two goldfish died in the 2 fish L−1 group (on days 21, 37) and one goldfish died in the 0.125 fish L−1 group (on day 24). Total mean abundance was 3.0, 8.7, 24.8, 41.8 and 29.2 at host densities of 0.125, 0.25, 0.5, 1 and 2 fish L−1, respectively, over the 58-day experiment (Table 1). Total mean abundance in medium (0.5 fish L−1) and high-density groups (1 and 2 fish L−1) was significantly higher than that in low host density groups (0.125 and 0.25 fish L−1) (P < 0.05). There was a significant positive correlation (n = 26, R 2 = 0.3997, P < 0.05) between host density and mean abundance of G. kobayashii throughout the 58-day experiment (Fig. 1). The mean prevalence (75–81%) at high and medium–high host densities was higher than that (55–59%) at low densities throughout the 58-day experiment (Table 1).

Fig. 1. Relationship between host density and mean abundance of Gyrodactylus kobayashii on all goldfish (Carassius auratus) in each treatment throughout the 58-day experiment. The correlation is significant.
At a host density of 0.125 fish L−1, mean abundance was always low (<4.9). At 0.25 fish L−1, mean abundance was low (<6.6), but increased rapidly after day 44. Mean abundance never reached a peak at the two lowest densities over the 58 days. In other density groups, mean abundance increased to a high level on days 24–30, after which it fluctuated within a high range (Fig. 2). During the first 24 days, there were significant differences in mean abundance among the five host densities (Table 2). On days 18–24, mean abundance at high and medium-high host densities (0.5–2 fish L−1) was significantly higher than that at low host densities (0.25 and 0.125 fish L−1) (P < 0.05). At high and medium–high host densities, prevalence increased rapidly and peaked on days 12–24. The maximum prevalence was higher than 90%, and then stabilized at a high level. At low host densities, the maximum prevalence, which was observed on days 15–27, was lower than 90%, and then decreased to a medium level (Fig. 3).

Fig. 2. Changes in mean abundance of Gyrodactylus kobayashii over time on goldfish (Carassius auratus) at five host densities (0.125, 0.25, 0.5, 1, and 2 fish L−1).

Fig. 3. Changes in prevalence of Gyrodactylus kobayashii over time on goldfish (Carassius auratus) at five host densities (0.125, 0.25, 0.5, 1, and 2 fish L−1).
Table 2. Summary of linear mixed-effects model outputs for the measures of mean abundance of Gyrodactylus kobayashii on caudal fin of goldfish (Carassius auratus) during the first 24 days

df, degree of freedom of the model; AIC, Akaike's information criterion; logLik, log-likelihood ratios.
Exponential model fitting for population growth
Population growth of gyrodactylids fitted well with the exponential model on the isolated goldfish and grouped goldfish at densities of 0.25, 0.5, 1 and 2 fish L−1 (Fig. 4), but not at 0.125 fish L−1 owing to the low goodness of fit (Table 3). Population growth rate of gyrodactylids increased from 0.042 to 0.235 (/parasite/day) with host density over 24 days (Table 3). Intrinsic population growth rate was 0.362 on the whole body and 0.260 on the caudal fin of individual, isolated fish over 9 days.
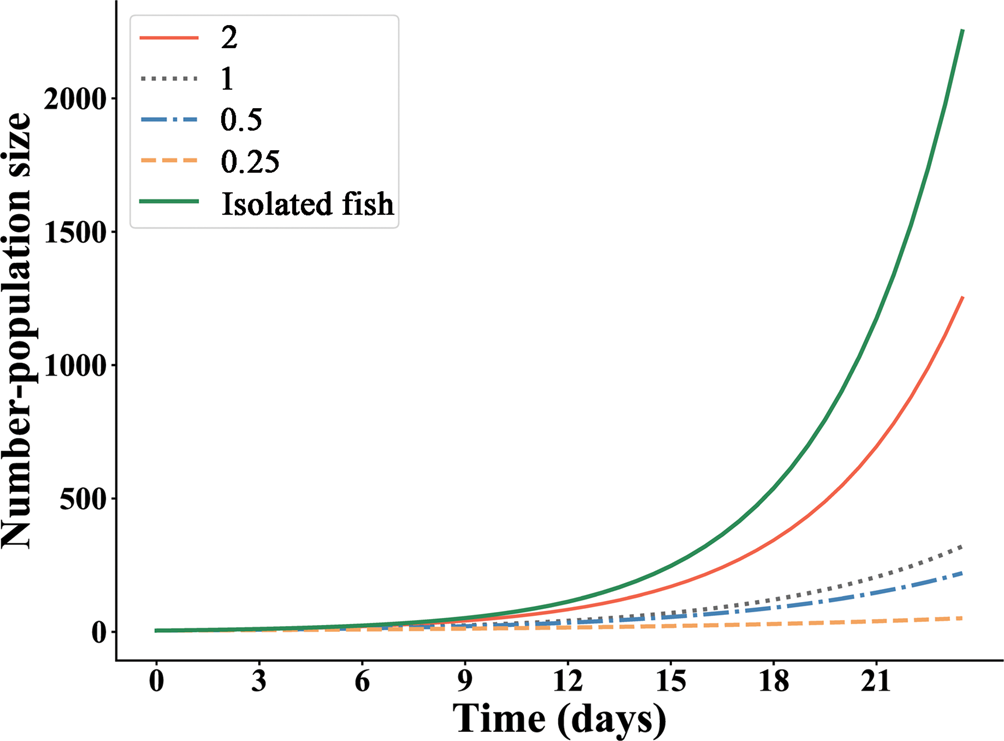
Fig. 4. Fitting of the exponential model for population growth of Gyrodactylus kobayashii on the caudal fin of the individual, isolated goldfish (Carassius auratus) over 9 days and all fish held in aquariums at four densities (0.25, 0.5, 1, and 2 fish L−1) over the first 24 days of the experiment.
Table 3. Population growth rate, estimated from fitting of the exponential model, of Gyrodactylus kobayashii on the isolated goldfish (Carassius auratus) before day 9 and those held at five densities before day 24. r, population growth rate; R 2, goodness of fit

Reinfection of detached gyrodactylids
Following isolation of the donor fish in the cage, G. kobayashii was detected on the body surface of the other goldfish in the aquarium. On each sampling day, both mean abundance and prevalence at 1 fish L−1 were significantly higher than those at 0.25 fish L−1 (P < 0.05) (Fig. 5).

Fig. 5. Mean abundance (A) and prevalence (B) during reinfection of the detached Gyrodactylus kobayashii on goldfish (Carassius auratus) at host density of 0.25 and 1 fish L−1 on days 1–7. The asterisk (*) represents significant differences between the two host densities.
When detached for 0 and 3 hours, more than 90% gyrodactylids were alive and 85–100% had infection capacity. After 12 and 24 hours of detachment from their hosts however, about 38% gyrodactylids were alive and only 15–20% were successfully infective (Fig. 6).

Fig. 6. Mean abundance of Gyrodactylus kobayashii on goldfish (Carassius auratus) inoculated with three gyrodactylids at four different detachment times on day 3 post-inoculation.
Discussion
Experimental results indicate that transmission of G. kobayashii on goldfish was density dependent at densities of 0.125–2 fish L−1. Population growth was exponential up to 24 days, and was also dependent on host density. Furthermore, experiments showed that transmission occurred via detached parasites, and this was greater at the higher density. Detached gyrodactylids maintained high survival and infection capacity within 3 h of detachment, indicating a limited window for reinfection of detached parasites.
A significant positive correlation was found between host density and mean abundance of G. kobayashii throughout the experiment, and mean abundance in high host density groups was significantly higher than that in low host density groups. These results suggested that population growth of G. kobayashii is dependent on host density under laboratory conditions. Under laboratory conditions, total population size of G. turnbulli on guppies increased with host density at those ranging from 0.07 to 0.6 fish L−1 (Johnson et al., Reference Johnson, Lafferty, Van Oosterhout and Cable2011). Our study differed from the latter in that Johnson et al. (Reference Johnson, Lafferty, Van Oosterhout and Cable2011) found that the occurrence of epidemics in the guppy was independent of host density, and this was likely due to the mating behaviour of the guppy, compared to the goldfish. Population growth of parasites is closely related to transmission strategy. For trophically transmitted parasites and directly transmitted parasites with an infective larva stage, physical contacts between parasites and hosts determine transmission and population growth (Barlow, Reference Barlow1996). However, for the viviparous gyrodactylids, transmission occurs primarily via contacts between infected and susceptible hosts, followed by parasite reproduction directly on the host (Bakke et al., Reference Bakke, Cable and Harris2007). Therefore contacts among hosts and the reproductive capacity, along with the survival of these parasites on their hosts, determine the population growth of gyrodactylids (Scott, Reference Scott1982). Using the guppy-Gyrodactylus model, Tadiri et al. (Reference Tadiri, Kong, Fussmann, Scott and Wang2019) found that parasite population growth was one of the most important factors affecting the strength and severity of infection. Population growth rates observed in our study (0.235 at the highest density) were generally comparable to those for G. turnbulli on guppies at 27.5°C and G. salaris on salmon at 19°C, which ranged from 0.22 to 0.23 (Scott and Nokes, Reference Scott and Nokes1984; Jansen and Bakke, Reference Jansen and Bakke1991). In field surveys, positive correlations were observed between host population density and mean abundance of strongylid nematodes in 19 mammal species (Arneberg et al., Reference Arneberg, Skorping, Grenfell and Read1998), A. gigantea in black rhinoceros (Stringer and Linklater, Reference Stringer and Linklater2015) and parasitic copepods (Lepeophtheirus salmonis) on Atlantic salmon (S. salar) (see Jansen et al., Reference Jansen, Kristoffersen, Viljugrein, Jimenez, Aldrin and Stien2012). The latter is of relevance to aquaculture, as are the results herein.
In the early days of infection, host density-dependent transmission was the main cause of population increase of G. kobayashii. Density-dependent transmission for directly transmitted pathogens has been supported by theoretical models (Anderson and May, Reference Anderson and May1979; Earn et al., Reference Earn, Rohani, Bolker and Grenfell2000; McCallum et al., Reference McCallum, Barlow and Hone2001; Smith et al., Reference Smith, Acevedo-Whitehouse and Pedersen2009) and empirical evidence from field surveys (Begon et al., Reference Begon, Feore, Brown, Chantrey, Jones and Bennett1998; Ramsey et al., Reference Ramsey, Spencer, Caley, Efford, Hansen, Lam and Cooper2002; Stringer and Linklater, Reference Stringer and Linklater2015). Generally, more contacts between parasites and hosts occur at higher density of hosts (Barlow, Reference Barlow1996). For pathogens transmitted by host contact, contact among host individuals is critical for transmission dynamics (McCallum et al., Reference McCallum, Barlow and Hone2001; Johnson et al., Reference Johnson, Lafferty, Van Oosterhout and Cable2011). For example, the contact rate between individual brushtail possum Trichosurus vulpecula during the breeding season decreased with reductions in host density (Ramsey et al., Reference Ramsey, Spencer, Caley, Efford, Hansen, Lam and Cooper2002). At the same host density, effective contact rate among goldfish was inferred to be constant at different host population sizes (Zhou et al., Reference Zhou, Zou, Wu, Wang, Marcogliese and Li2017). In the present study, the maximum prevalence was higher at high and medium–high host densities than that at low host densities, which suggested that host density-dependent transmission occurred during the first three to four weeks of the experiment. The increased contacts with density generally increase the number of new infected hosts (density-dependent transmission), but once established, gyrodactylids reproduce quickly and mean abundance increases further due to population growth (Scott, Reference Scott1982; Scott and Nokes, Reference Scott and Nokes1984). The fact that prevalence never peaked at the lowest host densities and mean abundance remained low at the two lowest host densities for at least the first 45 days of the experiment also suggests that host-to-host transmission promotes population growth at higher densities. Overall, mean abundance will be density dependent as populations increase on each individual infected host, until immunity kicks in and parasite mortality increases (Scott, Reference Scott1982, Reference Scott1985a; Zhou et al., Reference Zhou, Zou, Wu, Wang, Marcogliese and Li2017, Reference Zhou, Li, Zou, Zhang, Wu, Li and Wang2018).
When the hosts become infected, population growth of gyrodactylids is generally determined by birth rate and death rate (Scott, Reference Scott1982). Fecundity of gyrodactylids is dependent on water temperature (Scott and Nokes, Reference Scott and Nokes1984; Jansen and Bakke, Reference Jansen and Bakke1991) and age structure of the parasite (Scott, Reference Scott1982). Under the same laboratory conditions, birth and death rates are considered to be constant (Scott, Reference Scott1982). However, accidental death may be variable with host density. Gyrodactylids may become detached from their hosts, which is common during host–host contact, locomotion on the host, or as a result of the host immune response or host death (Scott and Anderson, Reference Scott and Anderson1984; Bakke et al., Reference Bakke, Harris, Jansen and Hansen1992). Furthermore, many parasites are lost during host-to-host transfer (Scott and Robinson, Reference Scott and Robinson1984). In the present study, the death rates of G. kobayashii were inferred to be variable with density based on the fitting of the population growth model. Compared with the intrinsic population growth rate (R = 0.260) measured on the caudal fin of individual, isolated fish, the population growth rate (r) declined from 0.235 to 0.042 with a decrease in host density (Table 3). Under the constant birth and death rate, accidental death rates (d = R–r) were inferred to be 0.218, 0.161, 0.099, 0.083 and 0.025 at host densities of 0.125, 0.25, 0.5, 1 and 2 fish L−1, respectively, which implies a high occurrence of accidental death at low host density during the early weeks of infection. Presumably, more parasites are lost during transfer, or more detached parasites cannot reinfect another host at low densities because contact rates are lower.
During the course of transmission, detachment has been observed in other experimental systems (e.g., Scott, Reference Scott1985a). Large numbers of detached gyrodactylids have been observed in the water column and on the substrate (Scott and Anderson, Reference Scott and Anderson1984; Bakke et al., Reference Bakke, Harris, Jansen and Hansen1992). Detachment was also inferred from the reinfection experiment where uninfected goldfish became infected following addition of the donor fish, which was isolated from the other fish in a double-mesh cage, to the experimental aquarium. Furthermore, a large proportion of detached parasites was inferred based on the higher accidental death rate at low host density (see above).
Even though the proportion of detached G. kobayashii was not estimated in the reinfection experiment, reinfection was dependent on host density, even after one day. In addition to the most important direct transfer during host contact, there are three other potential transmission routes, such as contact between hosts and detached gyrodactylids on the substrate, contact between host and detached parasites in the water column, and contact between living hosts and infected dead hosts (Bakke et al., Reference Bakke, Harris, Jansen and Hansen1992). Up to 10% of transmission is estimated to occur via contact between host and detached parasites in the water column in G. gasterostei (Harris, Reference Harris1982). But for G. salaris, transmission routes such as indirect transmission from the substrate or direct contact transmission from infected live and/or dead fish (prevalence 44.4%, mean intensity 1.9), were relatively more important than transmission by drifting detached parasites (prevalence 6.4%, mean intensity 1.0) (Soleng et al., Reference Soleng, Jansen and Bakke1999). While we cannot compare the different routes of transmission of detached parasites, in our reinfection experiment mean abundance and prevalence at the higher host density were both significantly greater than those at low density, even after one day, prior to parasite reproduction.
Other species of gyrodactylids give birth to the first-generation offspring about one day after infection at 19–25°C (Scott, Reference Scott1982; Jansen and Bakke, Reference Jansen and Bakke1991). The next offspring are born on day 4 (Scott, Reference Scott1982; Jansen and Bakke, Reference Jansen and Bakke1991). Thus, an increase in infrapopulation numbers after day 1 could be attributed to reproduction, in addition to reattachment. Results herein also suggest that the reattached parasites were able to establish successful reproducing populations with a notable increase after three days at high density. In contrast, no reattachment was observed at low host density after one day and very little after three days, suggesting that reattachment is very limited at low host densities and probably relatively unimportant. This result implies that the contact rate of detached parasites and the recipient fish was greater at the higher density and extremely low at the lower density. The population growth trajectory of G. kobayashii that infected goldfish via detached worms at high density over seven days was comparable to that observed in the density experiment over the same time span at 20°C. Thus, colonization of gyrodactylid through reattachment is capable of leading to vigorous infrapopulations, and could be a significant contributor to parasite spread, at least at high densities.
The movement of detached G. salaris and subsequent establishment of infection in a new host population was restricted by survival time (Olstad et al., Reference Olstad, Cable, Robertsen and Bakke2006). Similarly, infection ability of the detached G. kobayashii was maintained at a relatively high level only within the first 3 hours in our experiment. Thus, detached G. kobayashii have a narrow window to reattach to a new host. Scott (Reference Scott1985a) noted that detached G. turnbulli survived only a mean of 11.6 h. Only one of 14 detached G. salaris could infect Atlantic salmon after 24 h (Olstad et al., Reference Olstad, Cable, Robertsen and Bakke2006). With such a limited window for reattachment, these results together with those from the reattachment experiment reinforce the notion that reattachment may be significant only at high densities.
In summary, density-dependent population growth of G. kobayashii was confirmed under laboratory conditions. Both transmission to new hosts and population growth on individual hosts were density-dependent in the early weeks of infection. Reinfection of the detached gyrodactylids also was observed, but infections were negligible at low density after 3 days compared to high host density. Infectivity of detached gyrodactylids decreased greatly after 3 h. Gyrodactylid colonization through reattachment is capable of leading to vigorous infrapopulations, and could be an important contributor to parasite spread at high host densities.
Acknowledgements
The authors would like to thank the editor and the anonymous reviewer for their valuable suggestions and the time they have invested into reviewing the manuscript.
Authors' contributions
XJ performed the laboratory work, statistical analyses and wrote the article. WL designed the study and wrote the article. DJM revised the manuscript and interpreted the findings. HZ, ML, SW and GW contributed to the interpretation of the findings.
Financial support
This work was funded by the National Key Research and Development Program of China (Grant No. 2020YFD0900300), the National Natural Science Foundation of China (No. 31872604), and China Agriculture Research System of MOF and MARA.
Conflicts of interest
The authors declare there are no conflicts of interest.
Ethical standards
Fish feeding and anaesthetization were in accordance with the recommended guidelines for animal experimentation by the Chinese Association for Laboratory Animal Sciences.