Introduction
Panic disorder (PD) is characterized by recurring, unexpected panic attacks involving extreme fear and strong physiological reactions like dizziness, sweating and chest pain (American Psychiatric Association, 2013). Besides actual panic attacks, PD is accompanied by a constant concern about future attacks (American Psychiatric Association, 2013). This anticipatory anxiety is based on the uncontrollability and aversiveness of panic-related events (Helbig-Lang et al. Reference Helbig-Lang, Lang, Petermann and Hoyer2012) and commonly leads to avoidance behavior (Kessler et al. Reference Kessler, Chiu, Jin, Ruscio, Shear and Walters2006; White et al. Reference White, Brown, Somers and Barlow2006). Indeed, the experience of anticipatory anxiety seems to be more strongly related to avoidance behavior than to the actual occurrence or frequency of panic attacks (Cox et al. Reference Cox, Swinson, Norton and Kuch1991; Basoglu et al. Reference Basoglu, Marks, Kilic, Swinson, Noshirvani, Kuch and O'Sullivan1994). Accordingly, Grillon et al. (Reference Grillon, Lissek, Rabin, McDowell, Dvir and Pine2008) showed that the anxiety experienced when anticipating unpredictable aversive stimuli characterizes PD patients compared to healthy controls (HC).
A widely acknowledged neuroanatomical hypothesis attributes PD symptomatology to a low-threshold fear network (Gorman et al. Reference Gorman, Liebowitz, Fyer and Stein1989; Gorman et al. Reference Gorman, Kent, Sullivan and Coplan2000) and is supported by neuroimaging studies pointing towards abnormalities in amygdala, hippocampus, frontal cortex, insula, thalamus and hypothalamus (for review see Dresler et al. Reference Dresler, Guhn, Tupak, Ehlis, Herrmann, Fallgatter, Deckert and Domschke2013; Grambal et al. Reference Grambal, Hlustik and Prasko2015). The few studies that investigated the neural basis of anticipatory anxiety in PD patients present inconsistent findings with both hyperactivation (Wittmann et al. Reference Wittmann, Schlagenhauf, John, Guhn, Rehbein, Siegmund, Stoy, Held, Schulz, Fehm, Fydrich, Heinz, Bruhn and Strohle2011) and hypoactivation (Boshuisen et al. Reference Boshuisen, Ter Horst, Paans, Reinders and den Boer2002; Tuescher et al. Reference Tuescher, Protopopescu, Pan, Cloitre, Butler, Goldstein, Root, Engelien, Furman, Silverman, Yang, Gorman, LeDoux, Silbersweig and Stern2011) of amygdala, hyperactivation (Wittmann et al. Reference Wittmann, Schlagenhauf, John, Guhn, Rehbein, Siegmund, Stoy, Held, Schulz, Fehm, Fydrich, Heinz, Bruhn and Strohle2011; Gorka et al. Reference Gorka, Nelson, Phan and Shankman2014; Wittmann et al. Reference Wittmann, Schlagenhauf, Guhn, Lueken, Gaehlsdorf, Stoy, Bermpohl, Fydrich, Pfleiderer, Bruhn, Gerlach, Kircher, Straube, Wittchen, Arolt, Heinz and Strohle2014) and hypoactivation (Boshuisen et al. Reference Boshuisen, Ter Horst, Paans, Reinders and den Boer2002) of insula, and hyperactivation (Boshuisen et al. Reference Boshuisen, Ter Horst, Paans, Reinders and den Boer2002) and hypoactivation (Tuescher et al. Reference Tuescher, Protopopescu, Pan, Cloitre, Butler, Goldstein, Root, Engelien, Furman, Silverman, Yang, Gorman, LeDoux, Silbersweig and Stern2011) of anterior cingulate cortex (ACC), among other regions. Besides other methodological differences, these studies exhibit great variation regarding the duration of threat anticipation. While some studies used sustained anticipatory contexts (Boshuisen et al. Reference Boshuisen, Ter Horst, Paans, Reinders and den Boer2002; Tuescher et al. Reference Tuescher, Protopopescu, Pan, Cloitre, Butler, Goldstein, Root, Engelien, Furman, Silverman, Yang, Gorman, LeDoux, Silbersweig and Stern2011; Gorka et al. Reference Gorka, Nelson, Phan and Shankman2014), others studies used short anticipatory cues (Wittmann et al. Reference Wittmann, Schlagenhauf, John, Guhn, Rehbein, Siegmund, Stoy, Held, Schulz, Fehm, Fydrich, Heinz, Bruhn and Strohle2011; Wittmann et al. Reference Wittmann, Schlagenhauf, Guhn, Lueken, Gaehlsdorf, Stoy, Bermpohl, Fydrich, Pfleiderer, Bruhn, Gerlach, Kircher, Straube, Wittchen, Arolt, Heinz and Strohle2014), likely explaining inconsistent findings among these studies. PD patients seem to show both phasic and sustained altered brain responses during anticipation of aversive stimuli, although the precise role and temporal characteristics of different brain regions are unclear.
In the context of anticipatory anxiety, the investigation of temporal characteristics of involved brain regions is important. Distinct regions have been shown to be involved in phasic and sustained responding during threat contexts (Grupe & Nitschke, Reference Grupe and Nitschke2013). While the amygdala is associated with short-term responses, the bed nucleus of the stria terminalis (BNST) shows sustained responding to anxiety states during unpredictable threat (Davis et al. Reference Davis, Walker, Miles and Grillon2010; Avery et al. Reference Avery, Clauss and Blackford2016). The BNST, as part of the so-called extended amygdala, is a small but heterogeneous region with respect to nuclei and receptors (Lebow & Chen, Reference Lebow and Chen2016). It seems to play a central role in salient information processing and sustained threat monitoring (Mobbs et al. Reference Mobbs, Yu, Rowe, Eich, FeldmanHall and Dalgleish2010; Somerville et al. Reference Somerville, Whalen and Kelley2010; Avery et al. Reference Avery, Clauss and Blackford2016; Lebow & Chen, Reference Lebow and Chen2016). BNST functioning has been suggested to be highly relevant for pathological anxiety states (Lebow & Chen, Reference Lebow and Chen2016) and anxiolytic treatment (Hammack et al. Reference Hammack, Guo, Hazra, Dabrowska, Myers and Rainnie2009; Davis et al. Reference Davis, Walker, Miles and Grillon2010; Hazra et al. Reference Hazra, Guo, Dabrowska and Rainnie2012). With regard to PD patients, BNST functioning has been associated with sustained anxiety (Dresler et al. Reference Dresler, Guhn, Tupak, Ehlis, Herrmann, Fallgatter, Deckert and Domschke2013) and hypersensitivity to uncertainty (Grillon et al. Reference Grillon, Lissek, Rabin, McDowell, Dvir and Pine2008). Despite the fact that these are two cardinal features of the disorder (Helbig-Lang et al. Reference Helbig-Lang, Lang, Petermann and Hoyer2012), studies on BNST responsivity in PD are completely lacking.
A dissociation of phasic amygdala and sustained BNST responding during threat anticipation has been reported by studies in healthy subjects (Alvarez et al. Reference Alvarez, Chen, Bodurka, Kaplan and Grillon2011; Grupe et al. Reference Grupe, Oathes and Nitschke2013; Somerville et al. Reference Somerville, Wagner, Wig, Moran, Whalen and Kelley2013; Herrmann et al. Reference Herrmann, Boehme, Becker, Tupak, Guhn, Schmidt, Brinkmann and Straube2016) and specific phobia patients (Münsterkötter et al. Reference Münsterkötter, Notzon, Redlich, Grotegerd, Dohm, Arolt, Kugel, Zwanzger and Dannlowski2015). The role of phasic and sustained brain responses during threat anticipation in PD patients is yet unknown and has not been investigated within one and the same experiment. Besides dissociable threat responses, amygdala and BNST are suggested to be embedded in distinct functional networks during anticipatory anxiety (McMenamin et al. Reference McMenamin, Langeslag, Sirbu, Padmala and Pessoa2014; Herrmann et al. Reference Herrmann, Boehme, Becker, Tupak, Guhn, Schmidt, Brinkmann and Straube2016). While a few studies investigated amygdala connectivity in PD patients (Demenescu et al. Reference Demenescu, Kortekaas, Cremers, Renken, van Tol, van der Wee, Veltman, den Boer, Roelofs and Aleman2013; Kircher et al. Reference Kircher, Arolt, Jansen, Pyka, Reinhardt, Kellermann, Konrad, Lueken, Gloster, Gerlach, Strohle, Wittmann, Pfleiderer, Wittchen and Straube2013; Lueken et al. Reference Lueken, Straube, Konrad, Wittchen, Strohle, Wittmann, Pfleiderer, Uhlmann, Arolt, Jansen and Kircher2013), there are no studies on connectivity patterns in PD patients during anticipatory anxiety, especially with regard to BNST.
The aim of the current fMRI study was to investigate phasic and sustained neural responses in PD patients during anticipation of temporally unpredictable aversive stimuli (human screams). Based on its potential relevance for PD pathophysiology, we were especially interested in BNST functioning and in delineating the time courses of activation in amygdala and BNST, due to their distinct roles during threat anticipation. We used two different analysis models to disentangle phasic and sustained brain responses and hypothesized that increased phasic amygdala activation and sustained BNST activation would be evident in PD patients relative to HC. We also expected anticipation of aversive v. neutral sounds to induce alterations in a typical emotion-processing network consisting of insula, ACC, and frontal cortex. Finally, we conducted psychophysiological interaction (PPI) analysis to elucidate functional connectivity patterns of amygdala and BNST.
Methods and materials
Subjects
In total 19 PD patients and 19 HC were recruited for the study through public advertisement and in collaboration with an outpatient clinic. Two patients had to be excluded, one due to technical problems during fMRI scanning and one because the experiment was aborted after the practice trials. The final sample consisted of 17 PD patients and 19 HC, matched for gender, age, and education (for characterization, see online Supplementary Table S1). Exclusion criteria were neurological disorders, traumatic brain injury, psychotic or bipolar disorder, and drug abuse or dependence within the past 10 years. PD patients with and without agoraphobia were included and were diagnosed with a structured clinical interview according to DSM-IV criteria (SKID; Wittchen et al. Reference Wittchen, Wunderlich, Gruschwitz and Zaudig1997). PD patients scored significantly higher than HC on the Panic and Agoraphobia Scale (PAS; Bandelow, Reference Bandelow1997), Agoraphobic Cognitions Questionnaire (ACQ; Ehlers et al. Reference Ehlers, Margraf and Chambless1993), Body Sensations Questionnaire (BSQ; Ehlers et al. Reference Ehlers, Margraf and Chambless1993), Mobility Inventory for Agoraphobia (MI; Ehlers et al. Reference Ehlers, Margraf and Chambless1993), Anxiety Sensitivity Index (ASI; Reiss et al. Reference Reiss, Peterson, Gursky and McNally1986), and the Beck Depression Inventory (BDI; Beck et al. Reference Beck, Steer and Brown1996). Comorbidities of PD patients included depressive disorder (n = 2), eating disorder (n = 1), dysthymic disorder (n = 1), obsessive compulsive disorder (n = 1), generalized anxiety disorder (n = 2), social anxiety disorder (n = 1), somatization disorder (n = 2), and specific phobia (n = 1). Three PD patients took antidepressant medication and were stabilized on such medication, and three patients received therapy at the time of study participation. The study conforms to the Declaration of Helsinki and was approved by the local ethics committee. All participants gave written informed consent prior to the experiment.
Experimental design
During scanning, participants saw one of two cues (hash or percent sign) that announced the presentation of either an aversive or neutral sound. Cues were presented from trial onset until the end of sound presentation. Sounds were chosen from the International Affective Digitized Sounds database (IADS-2; Bradley & Lang, Reference Bradley and Lang1999) and consisted of human screams (#275, 276, 277) as aversive stimuli and water sounds (#172, 726, 377) as neutral stimuli. Such aversive stimuli are known to represent threat (Herrmann et al. Reference Herrmann, Boehme, Becker, Tupak, Guhn, Schmidt, Brinkmann and Straube2016). The duration of the sound clips was shortened to a representative sequence of 4 s and the sound intensity was set to a constant level. For the purpose of familiarization, each of the six sounds was presented once before the start of the actual experiment. Additionally, participants were instructed about the assignment of the two cues to the aversive and neutral condition (counterbalanced across participants).
In total, the experiment consisted of 11 aversive and 11 neutral trials, which were presented in pseudorandomized order. Anticipation intervals were variable in length to keep the presentation of the sounds temporally unpredictable. The majority of anticipation intervals lasted 16 s (7 per condition) to allow for investigation of sustained brain responses, while there were four shorter intervals (2 × 3 s, 1 × 5 s, 1 × 10 s per condition). A similar study by Herrmann et al. (Reference Herrmann, Boehme, Becker, Tupak, Guhn, Schmidt, Brinkmann and Straube2016) used anticipation intervals of up to 35 s, reporting behavioral and neural correlates of anxiety in healthy volunteers. As demonstrated by Berns et al. (Reference Berns, Chappelow, Cekic, Zink, Pagnoni and Martin-Skurski2006) anticipation becomes more aversive with increasing duration of the anticipation interval. To differentiate between HC and PD patients, known to be particularly sensitive to sustained uncertainty, our study thus used a threshold design with shorter anticipation intervals. Participants were told that the sounds could be presented at any time after cue appearance. After each trial, a white fixation cross was shown for 15 s before the subsequent trial started. In total the experiment lasted 11 min and 40 s.
After scanning, the participants had to rate the two cues as well as the six sounds with regard to valence (1 = very unpleasant, 9 = very pleasant, with 5 = neutral), anxiety (1 = not anxiety-inducing, 9 = highly anxiety-inducing) and arousal (1 = not arousing, 9 = highly arousing) on a nine-point Likert scale (Self Assessment Manikin; Bradley & Lang, Reference Bradley and Lang1994). Rating data were analyzed with mixed-model analyses of variance (ANOVAs) using IBM SPSS software (Version 22; IBM, Armonk, New York, USA), with group (PD v. HC) as between-subject factor and condition (aversive v. neutral sounds) as within-subject factor. Post-hoc t tests were used to resolve interactions where necessary. A p value of <0.05 was considered statistically significant.
FMRI
FMRI data were collected with a 3 T magnetic resonance scanner (“Magnetom PRISMA”; Siemens Medical Systems, Erlangen, Germany). Scanning began with a high resolution T1-weighted anatomical scan with 192 slices. Subsequently, functional data were acquired with a T2*-weighted echo-planar sequence (TE = 30 ms, flip angle = 90°, matrix = 92 × 92, FOV = 208 mm2, TR = 2080 ms) consisting of 340 volumes with 36 axial slices (thickness = 3 mm, gap = 0.3 mm, in plane resolution = 2.26 × 2.26 mm).
Functional data were preprocessed and analyzed with BrainVoyager QX (Version 2.8; Brain Innovation, Maastricht, the Netherlands). To ensure adequate saturation, the first four volumes were discarded from each run. During preprocessing, data were corrected for slice time errors and movement artifacts. Anatomical and functional data were co-registered and normalized to fit Talairach space (Talairach & Tournoux, Reference Talairach and Tournoux1988). Finally, data were smoothed spatially [6 mm full-width at half maximum (FWHM) Gaussian kernel] and temporally (high pass filter: 10 cycles per run; low pass filter: 2.8 s; linear trend removal).
Statistical analysis consisted of multiple linear regression of the signal time course at each voxel. The expected blood oxygenation level dependent (BOLD) signal change for each condition was modeled with a canonical double-gamma hemodynamic response function (HRF). We calculated two separate general linear models (GLMs) for the anticipation interval. In the first GLM, the HRF was modeled over the whole anticipation interval (sustained response GLM). The second GLM modeled a phasic HRF as the HRF initiated by the onset (first second) of the anticipation interval (phasic response GLM). In both GLMs, sound presentation and six movement parameters were modeled as predictors of no interest. Afterwards, z-standardized predictor estimates based on voxel-wise statistical maps for each participant were calculated. Random effects analysis with adjustment for autocorrelation following a global AR(1) model across the individual predictor estimates for planned contrasts was performed.
All analyses were small-volume-corrected for a-priori defined regions of interest (ROIs). ROIs for amygdala, insula, ACC, and PFC (lateral, medial) were defined on the basis of the Automated Anatomical Labeling (AAL) atlas included in the Wake Forest University (WFU) PickAtlas software (Tzourio-Mazoyer et al. Reference Tzourio-Mazoyer, Landeau, Papathanassiou, Crivello, Etard, Delcroix, Mazoyer and Joliot2002; Maldjian et al. Reference Maldjian, Laurienti, Kraft and Burdette2003). MNI-coordinates were converted into Talairach space with ICBM2tal (Lancaster et al. Reference Lancaster, Tordesillas-Gutierrez, Martinez, Salinas, Evans, Zilles, Mazziotta and Fox2007). The BNST ROI was defined based on an anatomical atlas (Mai et al. Reference Mai, Assheuer and Paxinos1997; also see Herrmann et al. Reference Herrmann, Boehme, Becker, Tupak, Guhn, Schmidt, Brinkmann and Straube2016) and was dilated by 1 mm to avoid missing relevant activation.
Because of their relevance for anticipatory anxiety, PPI analyses were conducted for significantly activated clusters in the amygdala and BNST as seed regions. Based on the contrast anticipation of aversive v. neutral sounds (psychological predictor) and the signal time course extracted from these seed regions (physiological predictor), we calculated a PPI GLM, which contained the signal time course of the seed region as well as the PPI predictor. Extraction of time courses and convolution with HRF were done with Neuroelf's (http://www.neuroelf.net) ComputeGLM method.
The cluster-level statistical threshold estimator plugin for BrainVoyager (Goebel et al. Reference Goebel, Esposito and Formisano2006) was used to correct for multiple comparisons. We used an initial voxel-level threshold of p < 0.005 to balance between Type I and II error rates (Lieberman & Cunningham, Reference Lieberman and Cunningham2009) given the normally available patient sample sizes. Based on another study using the same anticipation paradigm in a different patient group (Brinkmann et al. Reference Brinkmann, Buff, Neumeister, Tupak, Becker, Herrmann and Straube2017), we expected effect sizes of around d = 1.2 in the current study. With an initial threshold of p < 0.001, the estimated voxel-level Power to detect even such strong effects is close to chance level (0.60) in our study, while an initial threshold of p < 0.005 (also see Lieberman & Cunningham, Reference Lieberman and Cunningham2009) raises the voxel-level Power to an appropriate level (0.80). Furthermore, we did not include whole-brain analysis to avoid possible inflation of false-positive clusters in parametric analyses due to inhomogeneity of spatial smoothness and spatial autocorrelations across the whole brain with resulting hot spots of false positive clusters in specific regions such as posterior cingulate cortex (Eklund et al. Reference Eklund, Nichols and Knutsson2016). Our ROI approach did not include these critical regions and we restricted our analyses to a homogeneous search space, especially with regard to the BNST, guided by a hypothesis-driven approach. A mask based on predefined ROIs for amygdala, insula, ACC and PFC, and a separate mask consisting of bilateral BNST, were applied to the thresholded maps with an estimated FWHM for spatial smoothness (Forman et al. Reference Forman, Cohen, Fitzgerald, Eddy, Mintun and Noll1995) and an iterative procedure (Monte Carlo simulation) with 1,000 iterations. The minimum cluster size threshold with a cluster-level false positive rate of p < 0.025 (additionally corrected for number of masks) was applied to the statistical maps.
To investigate the influence of behavioral measures on differential brain responses and connectivity, ratings regarding valence, arousal and anxiety for the cues as well as symptom severity as measured by PAS scores (Bandelow, Reference Bandelow1997), level of anxiety as measured by ASI scores (Reiss et al. Reference Reiss, Peterson, Gursky and McNally1986) and level of depression as measured by BDI scores (Beck et al. Reference Beck, Steer and Brown1996) of PD patients were correlated with mean beta values for differential activation and connectivity clusters (anticipation of aversive – neutral sounds) resulting from ROI and PPI analyses (Bonferroni-corrected significance level; phasic model: p ⩽ 0.0019; sustained model: p ⩽ 0.0045).
Furthermore, we were interested in the development of brain responses over the course of the experiment within amygdala and BNST. Therefore, we differentiated between the first and second half of the experiment and calculated ANOVAs with differences between anticipation of aversive and neutral sounds for the first and second half as within-group factor and group (PD v. HC) as between-group factor. This analysis was conducted for differential brain responses within amygdala and BNST.
Results
Behavioral data
The ANOVAs on the ratings of valence, arousal and anxiety for the cues announcing aversive and neutral sounds (Fig. 1) yielded significant main effects for condition (valence: F 1,34 = 6.19, p = 0.018; arousal: F 1,34 = 9.17, p = 0.005; anxiety: F 1,34 = 7.09, p = 0.012) and group (arousal: F 1,34 = 11.03, p = 0.002; anxiety: F 1,34 = 6.30, p = 0.017) as well as significant group × condition interaction effects (valence: F 1,34 = 9.36, p = 0.004; arousal: F 1,34 = 5.77, p = 0.022). Post-hoc t tests showed that PD patients rated the aversive cue as significantly more negative (t 34 = −3.02, p = 0.005) and more arousing (t 34 = 3.78, p = 0.001) than HC, while there were no significant group differences for the neutral cue. PD patients also rated the aversive cue as significantly more negative (t 16 = −3.49, p = 0.003) and more arousing (t 16 = 3.20, p = 0.006) than the neutral cue, while HC showed no differences.

Fig. 1. Ratings for valence (1 = very unpleasant, 9 = very pleasant, with 5 = neutral), arousal (1 = not arousing, 9 = highly arousing), and anxiety (1 = not anxiety-inducing, 9 = highly anxiety-inducing) of panic disorder (PD) patients and healthy controls (HC) for the cue announcing aversive and neutral sounds (a) and for aversive and neutral sounds (b). *p ⩽ 0.05, **p ⩽ 0.001.
The ANOVA for the aversive and neutral sounds (Fig. 1) resulted in significant main effects for condition (valence: F 1,34 = 142.33, p < 0.001; arousal: F 1,34 = 139.33, p < 0.001; anxiety: F 1,34 = 87.60, p < 0.001) and group (valence: F 1,34 = 6.98, p = 0.012; arousal: F 1,34 = 14.02, p = 0.001; anxiety: F 1,34 = 10.45, p = 0.003) as well as significant group × condition interaction effects (arousal: F 1,34 = 8.76, p = 0.006; anxiety: F 1,34 = 9.43, p = 0.004). Post-hoc analysis revealed that PD patients rated the aversive sounds as significantly more arousing (t 34 = 3.47, p = 0.001) and more anxiety-inducing (t 34 = 3.15, p = 0.003), and the neutral sounds as more arousing (t 34 = 2.47, p = 0.019) than HC. Furthermore, PD patients and HC rated the aversive sounds as significantly more arousing (PD: t 16 = 11.13, p < 0.001; HC: t 18 = 6.01, p < 0.001) and more anxiety-inducing (PD: t 16 = 9.56, p < 0.001; HC: t 18 = 4.22, p = 0.001) than the neutral sounds.
FMRI data
ROI analysis
Phasic response
The phasic response GLM for the contrast anticipation of aversive v. neutral sounds resulted in several activation differences between PD patients and HC (Table 1). PD patients showed increased activation in right central and basolateral amygdala as compared with HC (Fig. 2). Furthermore, PD patients in contrast to HC showed hyperactivation in dACC, anterior, posterior and mid-insula, as well as dorsomedial PFC (dmPFC), ventromedial PFC (vmPFC), dorsolateral PFC (dlPFC), and ventrolateral PFC (vlPFC) (online Supplementary Fig. S1). Correlational analyses resulted in a significant negative correlation between valence ratings and phasic dmPFC activation (r 16 = −0.722, p = 0.001), in that more negative ratings resulted in more activation. The ANOVA for the investigation of the development of brain responses within the amygdala over the course of the experiment yielded no significant effects.
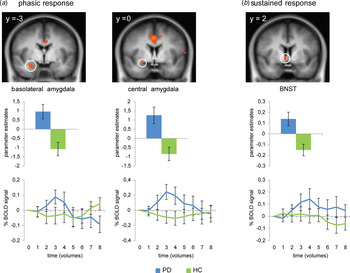
Fig. 2. Panic disorder (PD) patients compared with healthy controls (HC) showed increased phasic responses in right basolateral and central amygdala and an increased sustained response in right bed nucleus of the stria terminalis (BNST) during anticipation of aversive in contrast to neutral sounds. Statistical parametric maps are overlaid on an averaged T1 scan (radiological convention: left = right). Graphs in the middle row display contrasts of parameter estimates (anticipation of aversive v. neutral sounds; mean ± s.e. for activation cluster). Graphs in the bottom row display the relative blood oxygenation level dependent (BOLD) signal change over the anticipatory interval extracted from clusters of increased activation and averaged across time points of all trials with a duration of 16 s and across participants per group. Time points represent the contrast anticipation of aversive v. neutral sounds (mean ± s.e.).
Table 1. Significant phasic activations during anticipation of aversive v. neutral sounds

PD, panic disorder; HC, healthy controls; ACC, anterior cingulate cortex; PFC, prefrontal cortex; dmPFC, dorsomedial prefrontal cortex; vmPFC, ventromedial prefrontal cortex; dlPFC, dorsolateral prefrontal cortex; vlPFC, ventrolateral prefrontal cortex; L, left; R, right; (x, y, z), Talairach coordinates of maximally activated voxel (activation threshold: p < 0.025 corrected).
Sustained response
For the sustained response GLM, PD patients compared with HC showed increased activation in right BNST during anticipation of aversive v. neutral sounds (Fig. 2). This contrast also led to increased activation in bilateral anterior insula as well as dmPFC, vmPFC, dlPFC, and vlPFC in PD patients compared with HC (Table 2, online Supplementary Fig. S1). The ANOVA for the investigation of the development of brain responses over the course of the experiment within the BNST resulted in a significant main effect of group (F 1,34 = 11.94, p = 0.001).
Table 2. Significant sustained activations during anticipation of aversive v. neutral sounds
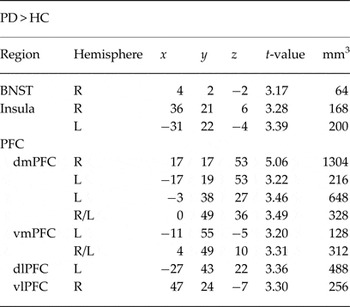
PD, panic disorder; HC, healthy controls; BNST, bed nucleus of the stria terminalis; PFC, prefrontal cortex; dmPFC, dorsomedial prefrontal cortex; vmPFC, ventromedial prefrontal cortex; dlPFC, dorsolateral prefrontal cortex; vlPFC, ventrolateral prefrontal cortex; L, left; R, right; (x, y, z), Talairach coordinates of maximally activated voxel (activation threshold: p < 0.025 corrected)
PPI
The phasic GLM resulted in two activation clusters in the right amygdala, which served as seed regions for PPI analyses (Table 3, Fig. 3). The time course of the central amygdala seed was positively associated with phasic left amygdala, dACC, mid-insula, dmPFC, vmPFC, dlPFC, and vlPFC activation in PD patients compared with HC. Furthermore, PD patients in contrast to HC showed significant phasic hyperconnectivity between the basolateral amygdala seed and rostral ACC (rACC), as well as hypoconnectivity with anterior insula and dlPFC. Using the significant cluster in the right BNST that emerged from the sustained GLM as seed region, we observed positive sustained psychophysiological interaction of the time course in this region with rACC, dmPFC, vmPFC, and dlPFC as well as negative association with dlPFC (Table 3, Fig. 3). Correlational analyses resulted in a significant positive correlation between ASI scores and hypoconnectivity between basolateral amygdala and anterior insula (r 16 = 0.710, p = 0.001).
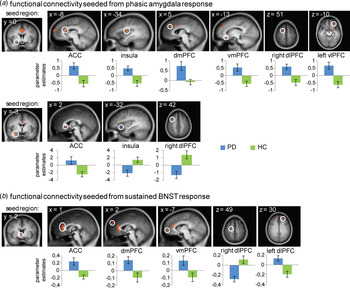
Fig. 3. Differential phasic activation in central amygdala showed increased functional connectivity with anterior cingulate cortex (ACC), insula, dorsomedial prefrontal cortex (dmPFC), ventromedial prefrontal cortex (vmPFC), right dorsolateral prefrontal cortex (dlPFC) and left ventrolateral prefrontal cortex (vlPFC) in panic disorder (PD) patients compared with healthy controls (HC) during anticipation of aversive in contrast to neutral sounds. Differential phasic activation in basolateral amygdala showed increased functional connectivity with ACC as well as decreased functional connectivity with insula and right dlPFC in PD patients compared with HC. Differential sustained activation in bed nucleus of the stria terminalis (BNST) showed increased functional connectivity with ACC, dmPFC, vmPFC, and left dlPFC as well as decreased functional connectivity with right dlPFC in PD patients compared with HC. For reasons of clarity, only the most significant activation cluster per brain region is displayed. Statistical parametric maps are overlaid on an averaged T1 scan (radiological convention: left = right). Graphs display contrasts of parameter estimates (anticipation of aversive v. neutral sounds; mean ± s.e. for activation cluster). R, right; L, left.
Table 3 Significant functional connectivity differences between PD and HC during anticipation of aversive v. neutral sounds

PD, panic disorder; HC, healthy controls; ACC, anterior cingulate cortex; dmPFC, dorsomedial prefrontal cortex; vmPFC, ventromedial prefrontal cortex; dlPFC, dorsolateral prefrontal cortex; vlPFC, ventrolateral prefrontal cortex; L, left; R, right; (x, y, z), Talairach coordinates of maximally activated voxel (activation threshold BNST seed: p < 0.025 corrected; activation threshold amygdala seeds: p < 0.0125 corrected)
Discussion
The aim of this study was to investigate neural correlates of unpredictable threat anticipation in PD patients, with a focus on the dissociation between amygdala and BNST. While the amygdala showed phasic responding during the first second of the anticipatory interval, the BNST showed a sustained response across the whole anticipatory interval. Furthermore, we observed phasic activation in dACC, insula, and medial and lateral PFC. The sustained model revealed increased activation in anterior insula and medial and lateral PFC. With regard to amygdala and BNST, PPI analysis demonstrated partly distinct phasic and sustained functional connectivity patterns.
In contrast to the phasic amygdala response, PD patients compared with HC showed sustained activation in BNST during threat anticipation. Animal research suggests that BNST activation reflects anxiety, heightened vigilance, and preparedness in contexts of sustained threat (Walker et al. Reference Walker, Miles and Davis2009; Davis et al. Reference Davis, Walker, Miles and Grillon2010; Fox et al. Reference Fox, Oler, Tromp, Fudge and Kalin2015). This is in line with studies in healthy subjects that demonstrated increased BNST activation during sustained threat anticipation (Alvarez et al. Reference Alvarez, Chen, Bodurka, Kaplan and Grillon2011; Grupe et al. Reference Grupe, Oathes and Nitschke2013; Somerville et al. Reference Somerville, Wagner, Wig, Moran, Whalen and Kelley2013; Herrmann et al. Reference Herrmann, Boehme, Becker, Tupak, Guhn, Schmidt, Brinkmann and Straube2016) and a relationship between BNST hyperactivation and temporal and physical proximity to threat (Mobbs et al. Reference Mobbs, Yu, Rowe, Eich, FeldmanHall and Dalgleish2010; Somerville et al. Reference Somerville, Whalen and Kelley2010). So far, increased BNST activation during anticipatory anxiety has only been shown in specific phobia (Straube et a l. Reference Straube, Mentzel and Miltner2007; Münsterkötter et al. Reference Münsterkötter, Notzon, Redlich, Grotegerd, Dohm, Arolt, Kugel, Zwanzger and Dannlowski2015), while no such hyperresponsiveness has been reported for PD or other anxiety and stress-related disorders. The increased BNST activation in PD patients in the current study suggests exaggerated levels of anxious apprehension (Alvarez et al. Reference Alvarez, Chen, Bodurka, Kaplan and Grillon2011; Grupe et al. Reference Grupe, Oathes and Nitschke2013; Somerville et al. Reference Somerville, Wagner, Wig, Moran, Whalen and Kelley2013) and hyperreactivity to uncertainty during anticipation of aversive stimuli (Grupe & Nitschke, Reference Grupe and Nitschke2013).
Amygdala showed phasic responding during anticipation of aversive v. neutral sounds in PD patients compared with HC. Given its pivotal role in fear and anxiety (LeDoux, Reference LeDoux2007), amygdala is also considered a central structure for PD (Gorman et al. Reference Gorman, Kent, Sullivan and Coplan2000; Kim et al. Reference Kim, Dager and Lyoo2012). Consistent with our results, Wittmann et al. (Reference Wittmann, Schlagenhauf, John, Guhn, Rehbein, Siegmund, Stoy, Held, Schulz, Fehm, Fydrich, Heinz, Bruhn and Strohle2011) found amygdala hyperactivation in response to briefly presented cues that indicated the appearance of agoraphobic or neutral pictures in PD patients with agoraphobia. However, contrary to our results, Boshuisen et al. (Reference Boshuisen, Ter Horst, Paans, Reinders and den Boer2002) found amygdala deactivation during anticipation of a panic attack in PD patients as compared with HC. This could be attributed to the fact that the study by Boshuisen et al. (Reference Boshuisen, Ter Horst, Paans, Reinders and den Boer2002) had only one anticipatory period that lasted 20 min and was analyzed as a whole. This is more comparable to our sustained analysis model, which revealed no amygdala activation. Other studies which only considered sustained anticipation of aversive stimuli also did not find an amygdala response (e.g. Straube et al. Reference Straube, Mentzel and Miltner2007; Alvarez et al. Reference Alvarez, Chen, Bodurka, Kaplan and Grillon2011; Grupe et al. Reference Grupe, Oathes and Nitschke2013; Herrmann et al. Reference Herrmann, Boehme, Becker, Tupak, Guhn, Schmidt, Brinkmann and Straube2016). However, as the current study shows, it is possible to detect amygdala hyperactivation in sustained anticipation paradigms by using a phasic analysis model (also see Alvarez et al. Reference Alvarez, Chen, Bodurka, Kaplan and Grillon2011; Grupe et al. Reference Grupe, Oathes and Nitschke2013; Herrmann et al. Reference Herrmann, Boehme, Becker, Tupak, Guhn, Schmidt, Brinkmann and Straube2016). Together, these findings support rapid threat processing in the amygdala (Alvarez et al. Reference Alvarez, Chen, Bodurka, Kaplan and Grillon2011; Grupe et al. Reference Grupe, Oathes and Nitschke2013; Herrmann et al. Reference Herrmann, Boehme, Becker, Tupak, Guhn, Schmidt, Brinkmann and Straube2016). The role of the amygdala in the deployment of attentional resources (Davis & Whalen, Reference Davis and Whalen2001; Grupe & Nitschke, Reference Grupe and Nitschke2013) indicates that PD patients showed enhanced vigilance as well as biased threat expectancies early during the anticipatory interval (Öhman & Mineka, Reference Öhman and Mineka2001).
Furthermore, the current data provide evidence for characteristic functional connectivity patterns in amygdala and BNST for PD patients. Our functional connectivity analysis revealed that phasic amygdala and sustained BNST responding are generally associated with activation in similar brain regions. This seems reasonable, as amygdala and BNST are closely interconnected and operate in similar networks (Walker et al. Reference Walker, Miles and Davis2009; Fox et al. Reference Fox, Oler, Tromp, Fudge and Kalin2015). However, amygdala showed distinct functional coupling with insula. Although both amygdala and BNST showed positive association with ACC and PFC, they were related to distinct subregions within these areas. Therefore, amygdala and BNST seem to be embedded in partly distinct networks associated with phasic and sustained brain responses (McMenamin et al. Reference McMenamin, Langeslag, Sirbu, Padmala and Pessoa2014). Notably, the two seed regions in the amygdala corresponding to central and basolateral amygdala also showed partly diverging functional connectivity. Information is assumed to progress from basolateral to central amygdala (Davis & Whalen, Reference Davis and Whalen2001; LeDoux, Reference LeDoux2007). Although both subregions are linked to threat processing and attentional processes (Etkin, Reference Etkin2010), lateral amygdala is assumed to integrate information (Phelps & LeDoux, Reference Phelps and LeDoux2005), while central amygdala is associated with emotional responding and modulation of vigilance (Davis & Whalen, Reference Davis and Whalen2001; LeDoux, Reference LeDoux2007). Our findings are thus in line with partly different functions of amygdala subregions, engaging different networks within a phasic timeframe.
We found several clusters of activation in the insula, and amygdala showed altered functional connectivity with insula subregions in PD patients during aversive anticipation. Interestingly, our analysis revealed differential phasic activation in mid-, anterior and posterior insula, while sustained activation was only found in anterior insula. Posterior and mid-insula are implicated in the representation of affective bodily feelings and the integration of bodily information from internal and external sources, respectively (Craig, Reference Craig2010). Anterior insula, on the other hand, is associated with subjective feelings as well as assessment of subjective value of situations and is suggested to regulate attentional processes (Craig, Reference Craig2009; Menon & Uddin, Reference Menon and Uddin2010). Moreover, insula activation is considered to reflect increased reactivity to uncertainty during anxiety states and low perceived control (Sarinopoulos et al. Reference Sarinopoulos, Grupe, Mackiewicz, Herrington, Lor, Steege and Nitschke2010; Grupe & Nitschke, Reference Grupe and Nitschke2013; Alvarez et al. Reference Alvarez, Kirlic, Misaki, Bodurka, Rhudy, Paulus and Drevets2015), also in PD patients (Gorka et al. Reference Gorka, Nelson, Phan and Shankman2014). Thus, the current findings indicate hyperreactivity to temporally uncertain aversive stimuli in PD patients. Furthermore, PD patients seem to exhibit an early and phasic elevated focus on bodily feelings, as well as sustained and negatively biased generation of emotional responses, resulting in enhanced subjective feelings of threat (Singer et al. Reference Singer, Critchley and Preuschoff2009; Grupe & Nitschke, Reference Grupe and Nitschke2013). Regarding functional connectivity, an amygdala-insula system has previously been implicated in anticipation of aversive stimuli (Sarinopoulos et al. Reference Sarinopoulos, Grupe, Mackiewicz, Herrington, Lor, Steege and Nitschke2010) and was associated with high trait anxiety (Carlson et al. Reference Carlson, Greenberg, Rubin and Mujica-Parodi2011). Together with the current findings, amygdala and insula seem to exchange information during threat anticipation, possibly for the purpose of attention allocation.
Regarding ACC, our analysis revealed several clusters of phasic activation in dACC, while this region showed no sustained activation. Furthermore, we found increased functional connectivity between amygdala and dACC/rACC, and between BNST and rACC. DACC activation was also found in PD patients anticipating panicogenic symptoms (Boshuisen et al. Reference Boshuisen, Ter Horst, Paans, Reinders and den Boer2002), while rACC hypoactivation was shown in PD compared with PTSD patients during threat of shock (Tuescher et al. Reference Tuescher, Protopopescu, Pan, Cloitre, Butler, Goldstein, Root, Engelien, Furman, Silverman, Yang, Gorman, LeDoux, Silbersweig and Stern2011). However, these studies all used sustained anticipatory periods. Similar to our study, healthy subjects showed phasic ACC activation during unpredictable threat anticipation (Alvarez et al. Reference Alvarez, Chen, Bodurka, Kaplan and Grillon2011; Grupe et al. Reference Grupe, Oathes and Nitschke2013; McMenamin et al. Reference McMenamin, Langeslag, Sirbu, Padmala and Pessoa2014; Herrmann et al. Reference Herrmann, Boehme, Becker, Tupak, Guhn, Schmidt, Brinkmann and Straube2016). Converging evidence highlights a role of the dACC in the appraisal of negative emotion and threat (Etkin et al. Reference Etkin, Egner and Kalisch2011; Maier et al. Reference Maier, Szalkowski, Kamphausen, Perlov, Feige, Blechert, Philipsen, van Elst, Kalisch and Tuscher2012; Kalisch & Gerlicher, Reference Kalisch and Gerlicher2014). Thus, PD patients in the current study seem to exhibit rapid appraisal of the aversive anticipatory context. Increased functional connectivity of dACC with amygdala further suggests that these regions work in concert during initial aversive anticipation. In contrast, rACC is associated with the regulation of emotional responses generated in limbic regions (Etkin e t al. Reference Etkin, Egner and Kalisch2011, Reference Etkin, Buchel and Gross2015), which might be reflected in hyperconnectivity between amygdala as well as BNST and rACC in the current study.
More sustained regulatory functions and action preparation are generally also attributed to ACC (Shenhav et al. Reference Shenhav, Botvinick and Cohen2013; Vogt, Reference Vogt2014). Due to the absence of sustained ACC activation, the current results rather suggest that these functions are fulfilled by other prefrontal areas. We found phasic and sustained hyperactivation in medial and lateral PFC regions in PD patients as compared with HC during aversive anticipation. Medial and lateral PFC together with ACC have been implicated in emotion processing, appraisal, regulation, and expression of fear and anxiety (Etkin et al. Reference Etkin, Egner and Kalisch2011; Maier et al. Reference Maier, Szalkowski, Kamphausen, Perlov, Feige, Blechert, Philipsen, van Elst, Kalisch and Tuscher2012; Kalisch & Gerlicher, Reference Kalisch and Gerlicher2014; Duval et al. Reference Duval, Javanbakht and Liberzon2015). PFC regions are consistently implicated in anxiety disorders, although the picture is less clear for PD (Engel et al. Reference Engel, Bandelow, Gruber and Wedekind2009; Dresler et al. Reference Dresler, Guhn, Tupak, Ehlis, Herrmann, Fallgatter, Deckert and Domschke2013). The multitude of hyperactivated PFC clusters in our study possibly reflects enhanced threat processing and the attempt to downregulate threat-related emotional responding in PD patients.
Evidence for a modulating influence of PFC regions also stems from our functional connectivity analysis. BNST showed increased connectivity with mPFC, while amygdala was positively associated with both medial and lateral PFC. Notably, amygdala and BNST both showed negative association with dlPFC. Enhanced connectivity between amygdala and mPFC/ACC regions was also shown in non-responders to cognitive behavioral therapy and might represent vulnerability for PD (Lueken et al. Reference Lueken, Straube, Konrad, Wittchen, Strohle, Wittmann, Pfleiderer, Uhlmann, Arolt, Jansen and Kircher2013). Additionally, anxiety symptom severity in PD patients was associated with amygdala-mPFC/ACC connectivity during processing of fearful faces (Demenescu et al. Reference Demenescu, Kortekaas, Cremers, Renken, van Tol, van der Wee, Veltman, den Boer, Roelofs and Aleman2013). It has further been suggested that anxiety disorders are not necessarily based on a failure of mPFC to downregulate the amygdala, but that increased mPFC activation might also represent overcompensation (Duval et al. Reference Duval, Javanbakht and Liberzon2015) and lead to enhanced negative affect (Myers-Schulz & Koenigs, Reference Myers-Schulz and Koenigs2012). Keeping this in mind, the current results could indicate that amygdala-mPFC/ACC coupling during anticipatory anxiety increases negative experience in PD patients.
BNST and mPFC have been shown to be structurally and functionally connected (Avery et al. Reference Avery, Clauss, Winder, Woodward, Heckers and Blackford2014; Torrisi et al. Reference Torrisi, O'Connell, Davis, Reynolds, Balderston, Fudge, Grillon and Ernst2015). Accordingly, vmPFC lesions led to decreased BNST activation (Motzkin et al. Reference Motzkin, Philippi, Oler, Kalin, Baskaya and Koenigs2015) and dmPFC showed functional connectivity with BNST under threat of shock (Kinnison et al. Reference Kinnison, Padmala, Choi and Pessoa2012). It is thus assumed that BNST interacts with mPFC to express aversive emotional states in contexts of threat anticipation (Avery et al. Reference Avery, Clauss and Blackford2016). Functional connectivity has also been reported for amygdala and BNST with lateral PFC during anticipatory anxiety in HC (Herrmann et al. Reference Herrmann, Boehme, Becker, Tupak, Guhn, Schmidt, Brinkmann and Straube2016). Assuming that lateral PFC is implicated in the integration of cognition and emotion (Pessoa, Reference Pessoa2008; Duval et al. Reference Duval, Javanbakht and Liberzon2015), this functional connectivity might represent a pathway for the integration of information from multiple sources for evaluation and initiation of action (Pessoa, Reference Pessoa2008).
Our findings should be considered in light of some limitations. A potential limitation is the restricted sample size. However, due to clear a priori hypotheses regarding amygdala and BNST, our findings should be considered a relevant contribution to the field. Furthermore, comorbidities constitute a possible confound of our study. Since PD was the main diagnosis and PD patients commonly present comorbidities (Kessler et al. Reference Kessler, Chiu, Jin, Ruscio, Shear and Walters2006), the exclusion of patients with comorbid diagnoses would have limited the representativeness and generalizability of our findings. Future studies should test the specificity of our findings in larger samples, which would also allow for investigations of the moderating influence of specific variables, such as symptom severity, on brain responses. Additionally, it would be interesting to specifically investigate the influence of uncertainty on the neural correlates of PD patients during threat anticipation, for example by varying the probability of stimulus occurrence indicated by the cue.
To summarize, we provide first evidence for dissociation between amygdala and BNST in PD patients during unpredictable threat anticipation. While we found phasic amygdala and sustained BNST responding, the two regions were also embedded in partly distinct functional networks. Taken together, amygdala and BNST activations possibly mediate pathological fear and anxiety symptoms in PD. Furthermore, phasic and sustained activation was found in subregions of insula, ACC and PFC, suggesting different temporal and functional characteristics during threat anticipation. Our findings imply enhanced responding in emotion-processing regions in PD patients during unpredictable anticipation of aversive stimuli. Since maladaptive responding to uncertainty regarding the occurrence of panic attacks is one of the major burdens in PD (Bouton et al. Reference Bouton, Mineka and Barlow2001; Grillon et al. Reference Grillon, Lissek, Rabin, McDowell, Dvir and Pine2008), uncovering possible neural substrates for chronically increased fear and anxiety is particularly relevant and could provide valuable input for treatment.
Supplementary material
The supplementary material for this article can be found at https://doi.org/10.1017/S0033291717001192
Acknowledgements
This work was supported by the German Research Foundation (DFG: SFB/TRR 58: C06, C07).
Declaration of Interest
None.
Ethical Standard
The authors assert that all procedures contributing to this work comply with the ethical standards of the relevant national and institutional committees on human experimentation and with the Helsinki Declaration of 1975, as revised in 2008. The study was approved by the ethics committee of the University of Muenster (reference number: 2012-294-f-S).