Introduction
Episodes of mass mortalities and disease outbreaks affecting corals and other benthic sessile suspension feeders (BSF) are recorded with an increasing frequency all over the world (Ward & Lafferty, Reference Ward and Lafferty2004) including in the Mediterranean Sea (Rivetti et al., Reference Rivetti, Fraschetti, Lionello, Zambianchi and Boero2014). Anomalously high temperature periods and ocean acidification in consequence of climate change seem to be major pressures for BSF in the Mediterranean Sea (Cerrano et al., Reference Cerrano, Bavestrello, Bianchi, Cattaneo-Vietti, Bava, Morganti, Morri, Picco, Sara, Schiaparelli, Siccardi and Sponga2000; Perez et al., Reference Perez, Garrabou, Sartoretto, Harmelin, Francour and Vacelet2000; Coma et al., Reference Coma, Linares, Ribes, Diaz, Garrabou and Ballesteros2006; Garrabou et al., Reference Garrabou, Coma, Bensoussan, Bally, Chevaldonné, Cigliano, Diaz, Harmelin, Gambi, Kersting, Ledoux, Lejeusne, Linares, Marschal, Pérez, Ribes, Romano, Serrano, Teixido, Torrents, Zabala, Zuberer and Cerrano2009) and also at the global scale (Harvell et al., Reference Harvell, Mitchell, Ward, Altizer, Dobson, Ostfeld and Samuel2002); local increases of pollutants and sedimentation created by anthropogenic activities are important threats at regional scales (Lohrer et al., Reference Lohrer, Hewitt, Thrush, Lundquist, Nicholls and Liefting2003; Shahidul Islam & Tanaka, Reference Shahidul Islam and Tanaka2004; Thrush et al., Reference Thrush, Hewitt, Cummings, Ellis, Hatton, Lohrer and Norkko2004; Fabricius, Reference Fabricius2005; Maina et al., Reference Maina, de Moel, Zinke, Madin, McClanahan and Vermaat2013). Human activities that increase sedimentation as a result of coastal development (dredging, land filling, runoff from coastal construction etc.) threaten 25% of the world's coral reefs (Burke et al., Reference Burke, Reytar, Spalding and Perry2011). Increased sedimentation can cause smothering and burial of coral polyps, shading, tissue necrosis and population explosions of bacteria in coral mucus (Erftemeijer et al., Reference Erftemeijer, Riegl, Hoeksema and Todd2012). Most studies dealing with the effects of sedimentation are concerned with photosynthetic symbionts bearing scleractinian corals, but octocorals and other heterotrophic BSF are also adversely affected by heavy sedimentation via burial, smothering or clogging of feeding and/or respiratory surfaces (Fabricius et al., Reference Fabricius, Golbuu and Victor2007; Bell et al., Reference Bell, McGrath, Biggerstaff, Bates, Bennett, Marlow and Shaffer2015; Hendrick et al., Reference Hendrick, Hutchison and Last2016).
The Sea of Marmara is surrounded by seven cities, including Istanbul, Kocaeli and Bursa, which are highly industrialized and overpopulated. Consequently, various anthropogenic disturbances impact the Sea of Marmara, particularly along the Istanbul coasts (Özsoy et al., Reference Özsoy, Çağatay, Balkıs, Balkıs and Öztürk2016). This area has undergone significant changes over the last few decades owing to a rapid increase in industrialization and urbanization, particularly along its southern coasts (Kurt et al., Reference Kurt, Karaburun and Demirci2010). In spite of such anthropogenic disturbances, dense assemblages of endemic gorgonians were reported in the Sea of Marmara very close to southern Istanbul coasts (Topçu & Öztürk, Reference Topçu and Öztürk2015). Together with other BSF, they are important components of the invaluable Mediterranean coralligenous community, with significant effects on the structure, biomass and biodiversity of these communities (Ballesteros, Reference Ballesteros2006; Bertolino et al., Reference Bertolino, Cerrano, Bavestrello, Carella, Pansini and Calcinai2013; Ponti et al., Reference Ponti, Perlini, Ventra, Grech, Abbiati and Cerrano2014).
Recently, a massive die-off of BSF covered by high amounts of sediments was observed along Prince Islands coasts in the north-eastern Sea of Marmara. The purpose of our study was to determine the BSF species affected and to estimate the intensity of the reported incident along Prince Islands coasts. We also aimed to elucidate possible contributing causes and to describe histopathological changes in affected coral soft tissues.
Materials and methods
Study area
The Sea of Marmara is connected to the Aegean Sea by the Çanakkale Strait (Dardanelles) and to the Black Sea by the Istanbul Strait (Bosphorus). Circulation within the Sea of Marmara is characterized by a two-layer stratification, with a brackish upper layer originating from the Black Sea flowing southwards and a lower Mediterranean layer flowing northwards. The two layers are separated by a permanent halocline, located at 15–30 m depth depending on the season and location (Beşiktepe et al., Reference Beşiktepe, Sur, Özsoy, Latif, Oǧuz and Ünlüata1994). In the lower layer, the salinity is about 38.5 with temperature stable at 14–15°C throughout the year. The study area is located on the southern coasts of Prince Islands in the north-eastern Sea of Marmara (Figure 1A). Study sites were selected amongst stations that had the highest recorded octocoral density and diversity (Topçu & Öztürk, Reference Topçu and Öztürk2015). The communities on rocky bottoms in the subhalocline layer of southern Prince Islands coasts are dominated by BSF (Figure 1B), represented mainly by tubicolous polychaetes, echinoderms (the most common being Antedon mediterranea (Lamarck, 1816), Ophiothrix fragilis (Abildgaard in O.F. Müller, 1789) and Ocnus planci (Brandt, 1835)), several sponge species and anthozoans (the most common being Paralcyonium spinulosum Delle Chiaje, 1822, Parazoanthus axinellae (Schmidt, 1862), Sagartia spp., Paramuricea macrospina (Koch, 1882) and other alyconarians) (Supplementary material 1 and 2; Topçu & Topaloğlu pers. observ.).

Fig. 1. (A) Location of the study area in the Mediterranean Sea (a), in the Sea of Marmara (b) and location of the sites along Prince Islands coasts (c). Sampling sites for environmental parameters are shown with stars. Dumping sites are shown with crosses. (B) A typical landscape from rocky bottoms in Prince Islands at 30 m photographed on 16.9.2014 showing Paramuricea clavata (at the centre), Spinimuricea klavereni (at the upper left side), P. macrospina (at the left edge and behind S. klavereni), Paralcyonium spinulosum (at the left side) and several Antedon mediterranea. (C) The same location as in B but photographed on 15.11.2015. Photo credit: M. Öztabak.
Description of the field surveys
Field surveys were conducted from the halocline (where the Mediterranean waters reside, starting from 15–20 m) to a maximum of 40 m depth by scuba diving. Benthic species that showed signs of necrosis or mortality were noted and photographed in August 2015. Quantitative surveys were conducted between October and November 2015. Gorgonians were selected as monitoring models because they constitute key species in the local coralligenous community and as their mortality is easily quantified (Garrabou et al., Reference Garrabou, Coma, Bensoussan, Bally, Chevaldonné, Cigliano, Diaz, Harmelin, Gambi, Kersting, Ledoux, Lejeusne, Linares, Marschal, Pérez, Ribes, Romano, Serrano, Teixido, Torrents, Zabala, Zuberer and Cerrano2009). The abundances and distributions of four gorgonian species (Paramuricea clavata (Risso, 1826), Paramuricea macrospina, Spinimuricea klavereni (Carpine & Grasshoff, 1975), Eunicella cavolini (Koch, 1887)) in the area were recently recorded (Topçu & Öztürk, Reference Topçu and Öztürk2015) and a similar survey was repeated as a part of this study. One m2 quadrats were placed every metre on either side of the 20 m long transect tape laid on the seabed. In case of vertical walls or large boulders, 20 quadrats were placed haphazardly on the sea bottom. The number of gorgonian colonies in each quadrat were noted according to (1) showing no necrosis (healthy), (2) having recent necrosis on >10% of the colony surface (necrosed) and (3) having a completely denuded skeleton as previously described (Linares et al., Reference Linares, Coma, Garrabou, Diaz and Zabala2008a; Garrabou et al., Reference Garrabou, Coma, Bensoussan, Bally, Chevaldonné, Cigliano, Diaz, Harmelin, Gambi, Kersting, Ledoux, Lejeusne, Linares, Marschal, Pérez, Ribes, Romano, Serrano, Teixido, Torrents, Zabala, Zuberer and Cerrano2009). Quantitative surveys at Yassıada (S1 and S2) and Balıkçı Islands (S5 and S6) were repeated at two depths (26 and 35 m) because during qualitative surveys, the distribution of healthy/injured colonies seemed to be different above and below 30 m depth. The percentage of recent/old necrosis (denuded axis or epibiosis by pioneer species/colony overgrowth by more complex organisms such as sponges, bryozoans etc.) (Linares et al., Reference Linares, Coma, Diaz, Zabala, Hereu and Dantart2005) was noted only for S. klavereni as data from previous years were only available for this species.
Sampling of coral material
Sample material was taken from colonies of gorgonians Paramuricea clavata, Spinimuricea klavereni, Eunicella cavolini and the gold coral Savalia savaglia showing early stage lesions but no tissue loss. For this purpose, diseased colonies (N = 9 per species) were collected in November (2015) by scuba diving on the study site twice within a 10-day period. Centrifuge tubes containing 50 ml of sterile PBS were brought by the divers to the depth of the coral colonies and then diseased colony samples (~5 cm in length) were collected into separate tubes under water. The tubes were surfaced and they were brought to the laboratory at +4°C where the collected tissues were processed immediately for histopathology and microbiological examination.
Histopathological examination of coral material
Sample material from S. savaglia colonies was processed for histopathological examination. Approximately 1 cm3 of tissue sample was fixed in 10% buffered formalin, dehydrated in ethanol, cleaned in xylene and embedded in paraffin wax. Sections (5 µm thick) were stained with haematoxylin-eosin (HE) following standardized practices (Culling, Reference Culling1963). The stained tissue sections were examined under the microscope using the image analysis system NIS-Elements BR Microscope Imaging Software (Nikon Instruments).
Microbiological examination of coral material
For microbiological examination, tissue samples (1 cm3) of each specimen were collected aseptically and rinsed with sterile phosphate-buffered saline solution (pH 7.4) to remove planktonic cells. The tissue samples were then placed onto culture media including Marine Agar 2216 (MA) (Difco) and Mycosel Agar (BD) supplemented with chloramphenicol and cycloheximide (Raymundo et al., Reference Raymundo, Couch and Harvell2008). The mucoid layer of each specimen was also sampled by gently touching the coral surface with a sterile loop and streaking onto the same media as described above (Ben-Haim & Rosenberg, Reference Ben-Haim and Rosenberg2002; Raymundo et al., Reference Raymundo, Couch and Harvell2008). All samples were processed in triplicates and all plates were incubated at 22°C for 72 h. All isolated bacterial pure cultures were characterized using standard protocols to assess motility, Gram staining, oxidase and catalase activity, Oxidative/Fermentative (O/F) reaction, growth on TCBS agar and susceptibility to vibriostatic agent O/129. Tests to characterize fungal isolates included growth at 37°C, sporulation and staining with lacto-phenol cotton blue.
Genomic DNA extraction from microbial isolates
The bacterial isolates which displayed the same morphological and biochemical characteristics were selected and inoculated into Marine Broth 2216 (Difco) and incubated overnight at 22°C in a shaking incubator. For the fungal isolates, samples were taken directly from culture media. Genomic DNA was extracted from all isolates with the GeneJET Genomic DNA Purification Kit (Thermo) according to the manufacturer's instructions and used as the template for PCR.
PCR amplification and sequence analysis
For bacterial identification, partial 16S rRNA and gyrB (DNA gyrase, subunit B) gene products were amplified and sequenced. The 16S rRNA gene product was amplified using universal bacteria primers S-D-Bact-0008-a-S-20 (5′- AGAGTTTGATCCTGGCTCAG-3′) and S-*-Univ-0536-a-A-18 (5′- GWATTACCGCGGCKGCTG-3′) (Suau et al., Reference Suau, Bonnet, Sutren, Godon, Gibson, Collins and Doré1999). To amplify a part of the gyrB gene, the gyrBBAUP2 (5′- GCGGAAGCGGCCNGSNATGTA-3′) and gyrBBNDN1 (5′- CCGTCCACGTCGGCRTCNGYCAT-3′) primer sets were used (Santos & Ochman, Reference Santos and Ochman2004). For fungal identification, the ribosomal internal transcribed spacer (ITS) region was amplified and sequenced using the ITS4 (5′- TCCTCCGCTTATTGATATGC-3′) and ITS5 (5′- GGAAGTAAAAGTCGTAACAAGG-3′) primer set (White et al., Reference White, Bruns, Lee and Taylor1990). All PCR reactions (50 µl) included ~50 ng template DNA (2 µl), 0.4 µM of each primer (2 × 2 µl), DreamTaq PCR Master Mix (2×) (Thermo Scientific) (25 µl) and nuclease-free water (Thermo Scientific, Arktik) (19 µl). Amplification was performed using a thermal cycler (Thermo Fisher Scientific) with the following parameters for the partial 16S rRNA gene amplification: initial denaturation at 95°C for 3 min, followed by 30 cycles of amplification (denaturation at 95°C for 30 s, annealing at 56°C for 1 min, extension at 72°C for 1 min) and a final extension step of 72°C for 4 min (Suau et al., Reference Suau, Bonnet, Sutren, Godon, Gibson, Collins and Doré1999). For the partial gyrB gene amplification the program was: initial denaturation at 95°C for 5 min, followed by 3 cycles of 1 min at 95°C, 2 min 15 s at 55°C, 1 min 15 s at 72°C, 30 cycles of 35 s at 95°C, 1 min 15 s at 55°C, 1 min 15 s at 72°C and final extension at 72°C for 10 min (Pascual et al., Reference Pascual, Macián, Arahal, Garay and Pujalte2010). And finally, for the amplification of the fungal ITS region, the program was: initial denaturation at 95°C for 10 min, followed by 35 cycles of amplification (denaturation at 95°C for 15 s, annealing at 52°C for 30 s, extension at 72°C for 20 s) and a final extension step at 72°C for 7 min (Schoch et al., Reference Schoch, Seifert, Huhndorf, Robert, Spouge, Levesque, Chen, Bolchacova, Voigt and Crous2012).
After amplification, 10 µl of each PCR reaction was loaded on a 1.5% (w/v) TAE agarose gel containing ethidium bromide (0.5 ug ml−1) and electrophoresis was performed for 40 min at 90 V. PCR products were visualized on a UV transilluminator and fragment sizes were estimated against the GeneRuler 100 bp DNA Ladder (Thermo Scientific). PCR products were purified and sequenced bidirectionally by Medsantek (Istanbul, Turkey). Sequence editing and analysis were performed in Bioedit v7.0.0 (Hall, Reference Hall1999) using the ClustalX 2.1 (Larkin et al., Reference Larkin, Blackshields, Brown, Chenna, McGettigan, McWilliam, Valentin, Wallace, Wilm and Lopez2007) and BLASTN 2.2.20 algorithms (Zhang et al., Reference Zhang, Schwartz, Wagner and Miller2000). All obtained nucleotide sequences have been deposited in the GenBank database.
Environmental parameters
Data from other monitoring programmes were retrieved in order to estimate temporal variation of selected environmental parameters in the surrounding waters of Prince Islands. Silicate concentrations provide an indicator of terrestrial sediment and are hence of particular interest. Data series (3 or 4 months/year) of dissolved oxygen (mg l−1) (2000–2006; 2009; 2011) and silicate (μM) (2001–2009) at 40 m depth off the Kartal coast (E1 in Figure 1) were procured from a water monitoring programme conducted by the IU Institute of Marine Science and Management for İSKİ (Istanbul Water and Sewerage Administration) water monitoring programme. The following data were acquired from the integrated marine pollution monitoring project coordinated by TÜBİTAK Marmara Research Center: (1) temperature (°C), salinity, dissolved oxygen (mg l−1) along vertical profiles in August 2015 and 2016 (E2 in Figure 1); (2) silicate (μM) concentrations along vertical profiles at 10 stations in Prince Islands in August 2016; (3) silicate (μM) concentrations in the surface water at 73 stations over the Sea of Marmara except İzmit Bay in August 2016.
Statistical analysis
The differences in the number of dead, necrosed and healthy colonies at two different depths in Yassıada and Balıkçı Islands were compared by χ2 tests. The relation of the number of damaged colonies with the depth and the slope of sites were analysed by non-parametric Spearman rank correlation tests. The percentages of recent and old tissue necrosis on S. klavereni colonies before and after the mortality were compared by Kolmogorov–Smirnov tests. All statistical tests were effectuated with GraphPad Prism version 6.01 for Windows.
Results
Qualitative observations
Only organisms that could be identified from the apparent remaining skeletons were determined to species level because mortality had already severely affected the Prince Islands area when observations started in August 2015. The last survey in the area before mortality was in the first week of May 2015 when there were no unusual signs of adverse conditions affecting BSF. Impacts imposed by sedimentation may have started well before the surveys described here. In August 2015, benthic habitats were covered by a brownish deposit. BSF in soft bottom areas, where sea pens were previously recorded, were entirely gone (Supplementary materials 2 and 3). Empty shells of Pinna spp. could be observed at almost all sites (Figure 2B and Supplementary material 3). All alcyonarian species were absent after the event. A few colonies of Paralcyonium spinulosum, a very common species in the Prince Islands area (Topçu & Öztürk, Reference Topçu and Öztürk2013, Reference Topçu and Öztürk2015), could be found at depths below 32 m near Balıkçı Island. Most gorgonians were dead, but some colonies persisted at some sites (Figure 2, see the section below). Some hexacoral species, such as Sagartia spp., Parazoanthus axinellae and Savalia savaglia were still alive. Savalia savaglia colonies showed clear signs of lesions and were covered by a white soft mat which turned brown in October and then disappeared (Figure 3). Almost all massive sponges were absent, sometimes leaving a whitish mat behind (Figure 3). Sponges encrusting beneath other organisms were more abundant. Raspailia (Parasyringella) agnata (Topsent, 1896) and Stelligera stuposa (Ellis & Solander, 1786) which are common in the area (Topaloğlu, Reference Topaloğlu, Özsoy, Çağatay, Balkıs, Balkıs and Öztürk2016; Topaloğlu et al., Reference Topaloğlu, Evcen and Çınar2016) were the only erect sponges remaining but were showing signs of necrosis (Figure 3). Abundances of tolerant hydrozoans such as Obelia dichotoma and Bougainvillia muscus had greatly increased whereas other hydrozoans were absent or less prevalent (Topçu et al., Reference Topçu, Martell, Yılmaz and İşinibilir2016). No echinoderms were observed in the area after the sediment deposition event.

Fig. 2. Gorgonians affected by the mass mortality event: (A) Eunicella cavolini colonies that completely lost their tissues, covered with sediment and pioneer epibionts at Yassıada (S1); (B) a dead Spinimuricea klavereni colony covered with pioneer epibionts and a dead Pinna shell at S11; (C) a dead Paramuricea macrospina colony covered with pioneer epibionts and E. cavolini colonies at S2; (D) dead P. macrospina colonies at S8; (E) P. clavata colony showing loss of tissue (denuded axis parts covered by epibionts) at S9; (F) a dead and epibiont covered S. klavereni with E. cavolini colonies showing partial mortality at S3.

Fig. 3. (A) Colonies of the gold coral, Savalia savaglia, covered by a white soft mat (B, C) which turned brown in October at S6; (D) Stelligera stuposa sponge covered by epibionts at S8; (E–G) sponges that left a whitish mat behind at S10, S5 and S8.
Quantitative observations
Considering all gorgonian species together, the percentage of colonies with completely denuded skeleton varied among sites from 15.8 to 100.0% and was 65.9 ± 33.8% (mean ± SD) for the Prince Islands area (Figure 4A, C). The percentage of necrosed colonies varied from 0 to 47.1% at sites and was 15.2 ± 16.2% (mean ± SD) for the Prince Islands area, while that of healthy colonies varied from 0 to 59.2% at sites and was 19.0 ± 23.9% (mean ± SD) for the Prince Islands area. Densities of affected and healthy colonies were significantly different between the two depth layers (26 and 35 m) at Yassıada and Balıkçı Islands (χ2 = 291.1, P < 0.0001; χ2 = 42.96, P < 0.0001 respectively). However, for all sites considered together, no significant correlation could be found between the percentage of impacted colonies and the depth or the degree of slope at sites (r = −0.34 P = 0.25; r = −0.33, P = 0.29 respectively). Among gorgonians, P. macrospina was the most affected species, followed by S. klavereni, P. clavata and E. cavolini (Figure 4B). The percentage of dead colonies in the population of S. klavereni after the mortality event increased by 28-fold (Figure 5A). The percentages of recent necrosis on colonies were significantly different before and after the mortality event (Kolmogorov–Smirnov test, D = 0.96, P < 0.05; Figure 5B). The percentages of old necrosis on colonies were not significantly different before and after the mortality (Kolmogorov–Smirnov test, D = 0.37, P = 0.72; Figure 5B).
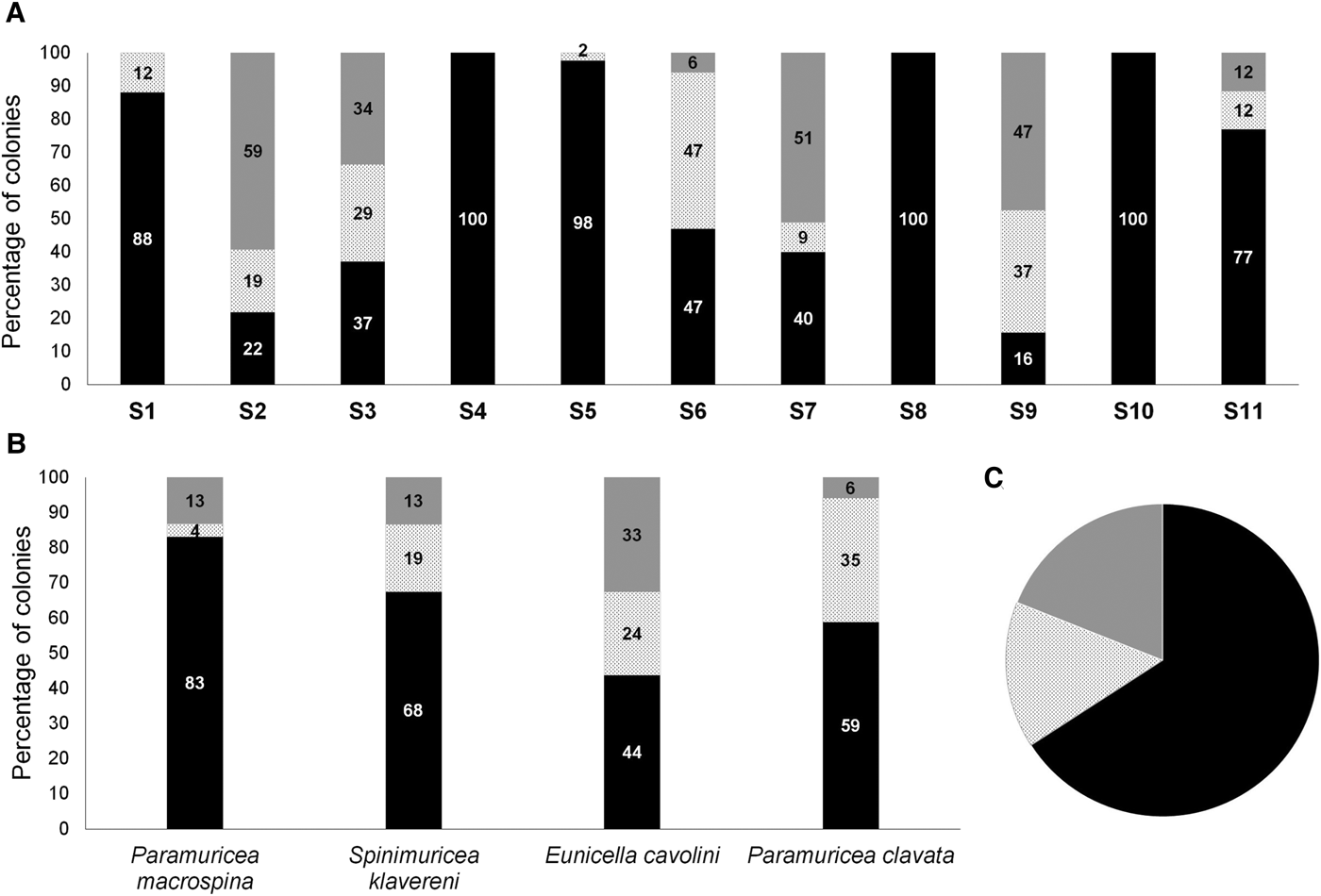
Fig. 4. (A) Percentages of entirely denuded, necrosed and healthy colonies at study sites. (B) Percentages of entirely denuded, necrosed and healthy colonies for each species at the study area. (C) Percentages of entirely denuded, necrosed and healthy colonies, all species combined at Prince Islands.

Fig. 5. (A) Percentages of entirely denuded, necrosed and healthy Spinimuricea klavereni colonies. (B) Percentages of necrosis (mean with range) for S. klavereni colonies; before mass mortality (BMM), after mass mortality (AMM).
Gross pathological and histopathological findings
External examination revealed that all diseased corals showed discolouration and some degree of tissue loss in the form of focal lesions. An unusual white coloured soft mat was observed on the surface of both diseased and dead colonies. Microscopic examination of this mat suggested that it was an abnormally thick multi-species biofilm containing bacteria and numerous fungal hyphae. HE-stained tissue sections of S. savaglia colonies revealed that both the epidermis and gastrodermis were necrotic (Figure 6A, B). Necrosis and exfoliation of mesentery were observed in tissues of diseased colonies (Figure 6C, D) along with sloughed cellular debris (Figure 6E, F).

Fig. 6. Tissue sections of diseased colonies of Savalia savaglia staining with haematoxylin-eosin: necrotic epidermis and gastrodermis and sloughing of epithelium (A, B); necrosis and exfoliation of mesentery (C, D); sloughed cellular debris and denuded mesoglea (E, F).
Microbiological findings
Thirty-nine bacterial and nine fungal isolates were obtained from the diseased coral sample material. All bacterial isolates were observed as Gram-negative curved and motile rods. Three distinguishable isolate variants were recognized according to phenotypic and biochemical characteristics (Table 1). Fungal isolates had branching hyphae producing a filamentous structure, were able to reproduce at 37°C and produced sporangia. Analysis of sequences from bacterial genes (16S rRNA, gyrB) and the fungal ITS region in addition to the morphological and phenotypical characteristics of isolates from Savalia savaglia identified 13 bacterial isolates as Vibrio splendidus (acc. no. KY933309-10), 7 isolates as Vibrio neptunius (acc. no. KY933260-61-62) while all fungal isolates were identified as Mucor circinelloides (acc. no. KY933391-92). The remaining bacterial isolates obtained from coral species other than S. savaglia were identified as members of genus Vibrio (acc. no. MF287166-67-68), but could not be further assigned to species level. All obtained sequences showed >99% similarity to sequences in the GenBank database.
Table 1. Morphological and phenotypic characteristics of the bacterial isolates obtained from diseased corals
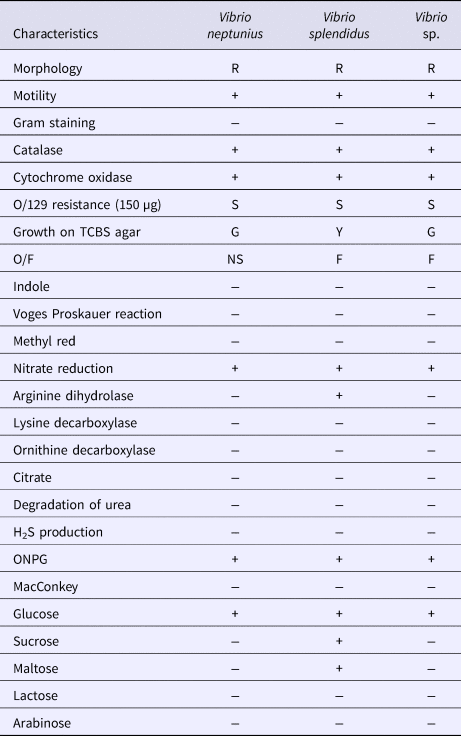
R, rods; −, negative; +, positive; F, fermentative; G, green; NS, non-saccharolytic; S, sensitive; Y, yellow; TCBS, Thiosulphate-Citrate-Bile-Sucrose; O/F, Oxidative/Fermentative test.
Environmental parameters
Dissolved oxygen at 40 m in the period 2000–2011 was 2.3 ± 0.8 mg l−1 (mean ± SD) (N = 27) and was generally above 1 mg l−1 except in August 2006 and then in 2015. Silicate concentration at 40 m in the period 2001–2009 was 23.52 ± 12.46 µM (mean ± SD) (N = 31) and was generally below 40 µM except in November 2003 and August 2016 (data from 2015 is absent). In August 2015, temperature, salinity and dissolved oxygen were 15.4°C, 38.3 and 0.8 mg l−1 at 40 m depth. In August 2016, ~2 months after the constructions had ended, temperature, salinity and dissolved oxygen were 15.5°C, 38.0, 1.43 mg l−1 at 40 m depth. The mean silicate concentration in surface waters in August 2016 for 73 stations over the Sea of Marmara was 2.35 µM (with a minimum of 0.017 and a maximum of 11.31 µM) while that for 10 stations along Prince Islands was 34.83 µM (with a minimum of 20.1 and a maximum of 76.16 µM).
Discussion
The effects of sedimentation on benthic suspension feeders
The significant decline of benthic macroorganisms that occurred in Prince Islands coasts appeared to be caused mainly by sediment deposits that resulted in the burial, smothering or clogging of feeding and/or respiratory organs. Burial and smothering by sediments is evidenced by the accumulated deposits on the sea bottom and on gorgonian branches (Supplementary material 4). Similar adverse effects on BSF have been reported following sediment dumping, coastal constructions or dredging operations in the Mediterranean Sea (Nepote et al., Reference Nepote, Bianchi, Morri, Ferrari and Montefalcone2017) and elsewhere in the world (Li et al., Reference Li, Huang, Lian, Yang, Ye, Chen and Huang2013; Haywood et al., Reference Haywood, Dennis, Thomson and Pillans2016).
Several episodes of mass mortality involving mainly benthic suspension feeders have been recorded in the Mediterranean Sea during recent decades (Cerrano et al., Reference Cerrano, Bavestrello, Bianchi, Cattaneo-Vietti, Bava, Morganti, Morri, Picco, Sara, Schiaparelli, Siccardi and Sponga2000; Garrabou et al., Reference Garrabou, Coma, Bensoussan, Bally, Chevaldonné, Cigliano, Diaz, Harmelin, Gambi, Kersting, Ledoux, Lejeusne, Linares, Marschal, Pérez, Ribes, Romano, Serrano, Teixido, Torrents, Zabala, Zuberer and Cerrano2009; Rubio-Portillo et al., Reference Rubio-Portillo, Izquierdo-Muñoz, Gago, Rosselló-Mora, Antón and Ramos-Esplá2016). These mortality events coincided with thermal anomalies in seawater temperature that have been causally linked with observed invertebrate mass mortalities (Rivetti et al., Reference Rivetti, Fraschetti, Lionello, Zambianchi and Boero2014, Reference Rivetti, Boero, Fraschetti, Zambianchi and Lionello2017). In the Sea of Marmara, the seawater temperature in the lower layer which would be experienced by the BSF studied here varies between 13.1 and 16.6°C according to monthly long-term data (Kayisoglu, Reference Kayisoglu2016). Because most anthozoans and sponges in the Mediterranean Sea have an upper limit of thermal tolerance around 24°C (Garrabou et al., Reference Garrabou, Bensoussan, Pairaud, Garreau, Somot, Linares, Kersting, Cebrian, de Caralt, Kipson, Ledoux and Frleta-Valić2013) temperature thus appears not to be a cause of the mass mortality reported here. Overall, it is possible to exclude additive effects deriving from global climate change on this event.
Nevertheless, low oxygen levels may have contributed to mortality. Oxygen levels in the lower layer of Prince Islands coast are low in general, being around 1–2 mg l−1. In fact, the lowest oxygen levels recorded during the last 16 years coincided with the reported BSF die-off. These low oxygen conditions might have become even more severe after the heavy sedimentation and caused extreme stress in suspension feeder invertebrates as explained further in the second part of the discussion. Before the observation of massive die-off in late summer 2015, several events that might have increased sedimentation occurred simultaneously in the Prince Islands area. Extensive red tide events developed in May 2015 and lasted for months (Türkoğlu, 2016). In July–August 2015, during the Kurbağalıdere stream rehabilitation process, dredged stream sediments were transported by ships and dumped near Yassıada and Sivriada. This dumping operation was confirmed by media coverage and there were rumours of stream mud dumping taking place near Büyükada (Kemiklidere). In addition, several building constructions and landfill operations began at Yassıada during May 2015 (Figure 7A, B). An aerial picture during construction clearly shows the large amount of terrestrial sediments spreading from the coasts of Yassıada in surrounding seawater (Figure 7D). Although there is no silicate data related to the construction work, it is reasonable to attribute high concentrations in both surface waters and at 40 m depth to these human activities on Yassıada. Such silicates in the seawater are always of terrestrial origin. Surface seawater in particular had almost 15 times higher concentrations of silicates, than other locations in the Sea of Marmara, which clearly illustrate the scale of input from Yassıada. Indeed, three separate sources have likely contributed to the excessive sedimentation in the study area (red-tides, stream sediment dumping and constructions/land filling) and their cumulative effect might have rendered BSF communities even more vulnerable to successive damages.

Fig. 7. Google Earth pictures of Yassıada (A) before and (B) after land filling operations. (C) Yassıada coast (S1) in October 2015 during fieldwork. (D) An aerial photo of Yassıada taken by a flight passenger in February 2016, photo credit: Levon Bağış.
The degree of damage to benthic macroorganisms by heavy sedimentation in various locations of Prince Islands may have depended on several factors acting simultaneously, including species composition, distance to the construction and dumping sites, local currents, the slope and depth of affected sea bottom areas. Sivriada, despite being located very close to the main construction site, was less affected by heavy sedimentation in comparison to other sites probably because the rocky substrates are characterized by vertical walls or a steeply sloping seabed. In fact, vertical substrates do not allow sediment deposition and the communities are less affected with respect to an area with more levelled sea bottom (Nepote et al., Reference Nepote, Bianchi, Morri, Chiantore and Montefalcone2016).
Soft corals (mainly Paralcyonium spinulosum), Paramuricea macrospina and Spinimuricea klavereni were the least tolerant and the first to respond to heavy sedimentation, exhibiting rapid mortality. Partially alive or healthy colonies of Eunicella cavolini were encountered in some locations where other gorgonians were dead (Supplementary material 3). The rapid mortality of S. klavereni and P. macrospina was surprising because these species are considered to be more tolerant to sedimentation due to their higher abundance on horizontal seafloors (Bo et al., Reference Bo, Bavestrello, Canese, Giusti, Angiolillo, Cerrano, Salvati and Greco2010; Topçu & Öztürk, Reference Topçu and Öztürk2016a). Excessive sediment deposits seem to have surpassed their tolerance limit.
Four gorgonians encountered in the region all have different life history traits and their recovery patterns might proceed distinctly in space and time. Spinimuricea klavereni, for example, grows relatively fast and has a high reproductive output throughout the year in the Sea of Marmara (Topçu & Öztürk, Reference Topçu and Öztürk2016a, Reference Topçu and Öztürk2016b). The confamilial gorgonian P. clavata is, on the other hand, a long-lived, slow-growing species, with high reproductive output but low recruitment (Coma et al., Reference Coma, Ribes, Zabala and Gili1995; Linares et al., Reference Linares, Coma, Mariani, Díaz, Hereu and Zabala2008b). Despite slow dynamics, in the north-western Mediterranean Sea where they have been decimated by mass mortalities, populations of P. clavata seem to show a recovering trend (Cerrano et al., Reference Cerrano, Arillo, Azzini, Calcinai, Castellano, Muti, Valisano, Zega and Bavestrello2005; Cupido et al., Reference Cupido, Cocito, Sgorbini, Bordone and Santangelo2008) but not everywhere (Bonhomme et al., Reference Bonhomme, Garrabou, Pérez, Sartoretto and Harmelin2003; Linares et al., Reference Linares, Coma and Zabala2008c). On the positive side, a recovery trend in populations of two octocorals with distinct life history traits, P. clavata and Corallium rubrum (Linnaeus, 1758), was observed even after a drastic mortality increase off the Italian coast (Santangelo et al., Reference Santangelo, Cupido, Cocito, Bramanti, Priori, Erra and Iannelli2015). The conditions of the Sea of Marmara are very different from the north-western Mediterranean Sea and recovery patterns of the gorgonians may proceed faster at the former location, supported by the mesotrophic-eutrophic level of productivity, if anthropogenic sources of sedimentation and other potential disturbances are successfully discontinued.
Histopathological and microbial findings in/on diseased gorgonians and gold corals
Although the link between anthropogenic stressors and disease susceptibility are poorly understood and the exact mechanisms remain unknown, coral diseases associated with water pollution and anthropogenic activities, such as increases in nutrient runoff (Bruno et al., Reference Bruno, Petes, Drew Harvell and Hettinger2003), sewage inputs (Pastorok & Bilyard, Reference Pastorok and Bilyard1985; Coles & Ruddy, Reference Coles and Ruddy1995) and dredging (Sheppard et al., Reference Sheppard, Al-Husiani, Al-Jamali, Al-Yamani, Baldwin, Bishop, Benzoni, Dutrieux, Dulvy and Durvasula2010; Hanley, Reference Hanley2011), have been reported from all over the world. To date, more than 20 distinct coral diseases have been described (Sheridan et al., Reference Sheridan, Kramarsky-Winter, Sweet, Kushmaro and Leal2013), however, primary pathogens have been reported in only a few cases (Peters, Reference Peters2015). Instead, most cases have been attributed to infections by opportunistic microorganisms in corals where the immune system has been weakened by a variety of stress factors (Vezzulli et al., Reference Vezzulli, Pezzati, Huete-Stauffer, Pruzzo and Cerrano2013; Peters, Reference Peters2015).
The gross pathological and histopathological findings we observed such as tissue loss, discolouration and necrosis of mesentery and sloughed cellular debris are similar to previous reports on Vibrio-related disease outbreaks in other coral species (Kushmaro et al., Reference Kushmaro, Banin, Loya, Stackebrandt and Rosenberg2001; Ben-Haim et al., Reference Ben-Haim, Thompson, Thompson, Cnockaert, Hoste, Swings and Rosenberg2003; Raymundo et al., Reference Raymundo, Couch and Harvell2003; Luna et al., Reference Luna, Biavasco and Danovaro2007). The family Vibrionaceae contains Gram-negative facultative anaerobic organisms that are ubiquitous in marine environments. As previously reported, some vibrios can even be associated with healthy tissues of common Mediterranean gorgonians that under extraordinary conditions act as a triggering mechanism of mass mortality events in the coastal NW Mediterranean Sea (Vezzulli et al., Reference Vezzulli, Previati, Pruzzo, Marchese, Bourne and Cerrano2010). Although members are found in the normal microbiota of numerous organisms (Thompson et al., Reference Thompson, Iida and Swings2004) including corals (Krediet et al., Reference Krediet, Ritchie, Paul and Teplitski2013), the Vibrionaceae contains highly pathogenic species for many marine organisms (Thompson et al., Reference Thompson, Iida and Swings2004; Stabili et al., Reference Stabili, Cardone, Alifano, Tredici, Piraino, Corriero and Gaino2012; Blanquer et al., Reference Blanquer, Uriz, Cebrian and Galand2016). To date, members of genus Vibrio associated with coral mortalities, in corals other than Savalia savaglia, include Vibrio shiloi (V. mediterranei) (Kushmaro et al., Reference Kushmaro, Banin, Loya, Stackebrandt and Rosenberg2001; Rubio-Portillo et al., Reference Rubio-Portillo, Izquierdo-Muñoz, Gago, Rosselló-Mora, Antón and Ramos-Esplá2016), Vibrio coralliilyticus (Ben-Haim et al., Reference Ben-Haim, Thompson, Thompson, Cnockaert, Hoste, Swings and Rosenberg2003; Bally & Garrabou, Reference Bally and Garrabou2007; Vezzulli et al., Reference Vezzulli, Previati, Pruzzo, Marchese, Bourne and Cerrano2010; Huete-Stauffer et al., Reference Huete-Stauffer, Vielmini, Palma, Navone, Panzalis, Vezzulli, Misic and Cerrano2011), Vibrio harveyi (Luna et al., Reference Luna, Bongiorni, Gili, Biavasco and Danovaro2010), Vibrio owensii (Ushijima et al., Reference Ushijima, Smith, Aeby and Callahan2012) but also unidentified members of genus Vibrio (Cervino et al., Reference Cervino, Hayes, Polson, Polson, Goreau, Martinez and Smith2004). Both Vibrio splendidus and Vibrio neptunius, isolated from diseased corals in the present study, have been shown to cause disease in a variety of organisms such as clams (Gómez-León et al., Reference Gómez-León, Villamil, Lemos, Novoa and Figueras2005), oysters (Lacoste et al., Reference Lacoste, Jalabert, Malham, Cueff, Gelebart, Cordevant, Lange and Poulet2001; Prado et al., Reference Prado, Romalde, Montes and Barja2005), sea cucumbers (Zhao et al., Reference Zhao, Zhang, Xu, Mai, Zhang and Liufu2012), corals (Hall-Spencer et al., Reference Hall-Spencer, Pike and Munn2007) and fish (Gatesoupe et al., Reference Gatesoupe, Lambert and Nicolas1999; Austin et al., Reference Austin, Austin, Sutherland, Thompson and Swings2005). However, to our knowledge, these bacteria have not been associated with or reported as contributing to disease in Savalia savaglia corals until now.
The development of an unusual white mat-like biofilm on holobiont surfaces may have been enabled by low oxygen levels leading to a compromised immune system in affected corals. Our microbial examination of affected corals also revealed the presence of a fungus identified as Mucor circinelloides. Like the two said Vibrio species, this fungus was consistently isolated also from coral mucous and soft tissues showing clear signs of necrosis. Mucor circinelloides is considered an opportunistic mould responsible for tissue necrosis in yellow catfish (Ke et al., Reference Ke, Wang, Li, Gu and Gong2009) as well as cases of mucormycosis (previously called zygomycosis) in immunocompromised humans (Iwen et al., Reference Iwen, Sigler, Noel and Freifeld2007; Dizbay et al., Reference Dizbay, Adisen, Kustimur, Sari, Cengiz, Yalcin, Kalkanci, Gonul and Sugita2009; Karan et al., Reference Karan, Tunçcan, Kalkancı, Arman and Kuştimur2014). According to the scientific literature, moulds in order Mucorales are ubiquitous in nature, but associated mostly with soil/terrestrial habitats, more specifically plants, processed food, dung, compost piles and decaying fruit (Richardson, Reference Richardson2009). It is therefore reasonable to assume that the fungus was artificially introduced to the marine environment and the affected corals either through the Prince Islands runoffs or with the off-shore dumping of highly polluted stream sediments. Our Mucor circinelloides isolates appear to be salt tolerant and able to grow in the marine environment like strains of a common soil fungus Aspergillus sydowii, an emerging opportunistic sea fan pathogen in the Caribbean (Smith et al., Reference Smith, Ives, Nagelkerken and Ritchie1996; Nagelkerken et al., Reference Nagelkerken, Buchan, Smith, Bonair, Bush, Garzon-Ferreira, Botero, Gayle, Harvell and Heberer1997; Geiser et al., Reference Geiser, Taylor, Ritchie and Smith1998). Artificial dissemination of pathogens or opportunistic microorganisms into new habitats, as a result of human activities, is a potential problem that should not be overlooked or underestimated. One would expect indigenous corals to be less resistant to infection and disease caused by such ‘alien’ microorganisms.
Conclusion
A significant decline of BSF, mainly comprising octocorals and sponges, was observed in the Prince Islands region of the north-eastern Sea of Marmara and likely caused primarily by heavy sedimentation. The bulk of observed sedimentation was clearly a direct result of the construction and landfill operations that took place at Yassıada. BSF suffered from burial, clogging or smothering of feeding and respiratory apparatus, and necrosis on body surfaces. However, numerous bacteria belonging to genus Vibrio and an opportunistic mould Mucor circinelloides, whose presence most likely was the result of either off-shore dumping of polluted stream mud or landfill run-offs, were consistently isolated from an unusual white mat-like biofilm covering the surface of diseased corals as well as from necrotic soft tissues. This overgrowth of opportunistic microorganisms may have been made possible by low oxygen levels leading to an impaired immune response in highly stressed corals and thus exacerbated the described BSF die-off. These results suggest that preventive measures are necessary when construction operations occur in the vicinity of sensitive marine habitats.
Supplementary material
The supplementary material for this article can be found at https://doi.org/10.1017/S0025315418001066.
Acknowledgements
The authors thank Serço Ekşiyan, Volkan Narcı, Can Sinmaz, Anıl Durmaz and Can Sarvan for their assistance in fieldwork. Dr Hüsne Altıok, Dr Nuray Balkıs and Dr Çolpan Beken are acknowledged for helping with collecting environmental data. Many thanks to Serço Ekşiyan for sharing video files and to Mehmet Öztabak for pictures in Figure 1 and Supplementary material 1 (photo A). Hydrochemical water column data were obtained from the TUBITAK Marmara Research Center (MRC ECPI), that is coordinating the ‘Integrated Marine Pollution Monitoring (2014–2016)’ Project supported by the Ministry of Environment and Urbanization/General Directorate of EIA, Permits and Control.
Financial support
This work was supported by the Scientific Research Project Coordination Unit of Istanbul University (BAP Project No FBA-2016-20161, BEK-2016-22336 and BEK-2016-24057).