Introduction
One of the difficult perceptual tasks with high perceptual load for human listeners is to recognize speech in a noisy ‘cocktail party’ speech-listening environment with multiple people talking (Cherry, Reference Cherry1953) , because the attended speech is under not only energetic masking that occurs at the peripheral level but also informational masking that interferes with the processing of the target talker's utterances at more central (i.e. cognitive) levels of processing (Schneider et al. Reference Schneider, Li and Daneman2007).
More specifically, under ‘cocktail party’ environments with multiple people talking (Cherry, Reference Cherry1953), two types of masking components contribute to the difficulty of speech recognition: energetic masking and informational masking (e.g. Freyman et al. Reference Freyman, Helfer, McCall and Clifton1999; Brungart et al. Reference Brungart, Simpson, Ericson and Scott2001; Li et al. Reference Li, Daneman, Qi and Schneider2004; Helfer & Freyman, Reference Helfer and Freyman2005, Reference Helfer and Freyman2009; Wu et al. Reference Wu, Wang, Chen, Qu, Li, Wu, Schneider and Li2005; Rakerd et al. Reference Rakerd, Aaronson and Hartmann2006; Huang et al. Reference Huang, Huang, Chen, Qu, Wu and Li2008, Reference Huang, Huang, Chen, Wu and Li2009; Wu et al. Reference Wu, Li, Gao, Lei, Teng, Wu and Li2012b ). Energetic masking occurs when peripheral neural activity elicited by a signal is overwhelmed by that elicited by maskers, leading to a degraded or lost neural representation of the signal. Informational masking occurs when signals and maskers are similar along some informational dimensions, particularly when both target signals and maskers are speech sounds, causing confusion between the target and masker and/or uncertainty regarding the target. Relative to energetic masking, informational masking involves more higher-order central processing (Schneider et al. Reference Yang, Chen, Huang, Wu, Wu, Schneider and Liang2007).
Under such adverse listening conditions, it is not unusual for a listener to ask the attended speaker repeat the sentence(s). The beneficial ‘say-it-again’ effect is caused by using some perceptually and/or cognitively unmasking primes, including prior knowledge about part of target-sentence content (Freyman et al. Reference Freyman, Balakrishnan and Helfer2004; Yang et al. Reference Yang, Chen, Huang, Wu, Wu, Schneider and Liang2007; Helfer & Freyman, Reference Helfer and Freyman2009; Wu et al. Reference Wu, Li, Gao, Lei, Teng, Wu and Li2012b ), familiarity with the target talker's voice (Yang et al. Reference Yang, Chen, Huang, Wu, Wu, Schneider and Liang2007; Huang et al. Reference Huang, Xu, Wu and Li2010), and visual working memory of the speaker's movements of speech articulators (Wu et al. Reference Wu, Cao, Wu and Li2013a , Reference Wu, Li, Tian, Wu, Wang and Li b ).
In studies of auditory speech priming (ASP), when a meaningless (nonsense) target sentence with a number of keywords is co-presented with a two-talker speech masker (which causes informational masking of the target sentence at both perceptual and cognitive levels; see Schneider et al. Reference Schneider, Li and Daneman2007), recognition of the last keyword of the target sentence is improved if the early segment of the same sentence (including the first or the first two keywords) recited by the target talker's voice (i.e. the auditory speech prime) is temporally pre-presented in quiet before the target/masker co-presentation (Freyman et al. Reference Freyman, Balakrishnan and Helfer2004; Yang et al. Reference Yang, Chen, Huang, Wu, Wu, Schneider and Liang2007; Wu et al. Reference Wu, Li, Gao, Lei, Teng, Wu and Li2012b ). Freyman et al. (Reference Freyman, Balakrishnan and Helfer2004) have suggested that the speech prime helps listeners focus attention more quickly on the target speech, thereby facilitating recognition of the last keyword in the target stream against informational masking. Up to date, the brain substrates specifically underlying the unmasking effect of ASP have not been reported in the literature. It has been reported that subjective clarity of degraded (noise-vocoding) speech can be enhanced by prior knowledge of speech content (Davis et al. Reference Davis, Johnsrude, Hervais-Adelman, Taylor and McGettigan2005; Sohoglu et al. Reference Sohoglu, Peelle, Carlyon and Davis2014) and the underlying mechanisms include top-down modulation of speech-signal processing at the processing stage of the auditory cortex (Sohoglu et al. Reference Sohoglu, Peelle, Carlyon and Davis2012; Wild et al. Reference Wild, Davis and Johnsrude2012). It is of interest to know whether the brain substrates underlying auditory prime-induced unmasking of speech are similar to those underlying prior speech-knowledge-induced improvement of clarity of degraded speech.
Compared with that in healthy listeners, the correct percentage of target-keyword recognition in patients with schizophrenia is lower not only under noise-masking conditions but also under speech-masking conditions (Wu et al. Reference Wu, Cao, Zhou, Wang, Wu and Li2012a , Reference Wu, Li, Tian, Wu, Wang and Li2013b ). More in detail, our previous studies have revealed that the difference in the threshold (in signal:masker ratio, SMR) for recognizing target keywords between people with first-episode schizophrenia and their healthy controls is 1.0 dB when the masker is steady-state speech-spectrum noise, but increases to 1.7 dB when the masker is two-talker speech. Also, the threshold difference between people with chronic schizophrenia and their healthy controls is 1.9 dB when the masker is the noise, but increases to 3.0 dB when the masker is the speech. Interestingly, although people with schizophrenia perform worse than healthy listeners in speech recognition against informational masking (Wu et al. Reference Wu, Cao, Zhou, Wang, Wu and Li2012a , Reference Wu, Li, Tian, Wu, Wang and Li2013b ), they retain the ability to use the temporally pre-presented auditory-speech primes to improve (unmask) target-speech recognition under informational masking conditions (Wu et al. Reference Wu, Cao, Zhou, Wang, Wu and Li2012a ). Knowing whether the brain substrates normally underlying the ASP in healthy listeners are also functional in listeners with schizophrenia is important for understanding the nature of the brain plasticity associated with schizophrenia.
It has been reported that people with schizophrenia experience more difficulties in filtering irrelevant/distracting sensory stimuli to prevent information overflow that leads to various cognitive dysfunctions (Gottesman & Gould, Reference Gottesman and Gould2003; Braff & Light, Reference Braff and Light2005). They also perform worse than healthy listeners in speech perception particularly under informational speech-masking conditions (Wu et al. Reference Wu, Cao, Zhou, Wang, Wu and Li2012a , Reference Wu, Li, Tian, Wu, Wang and Li2013b ; Zheng et al. Reference Zheng, Wu, Li, Wu, She, Liu, Wu, Mao, Ning and Li2016). Up to date, marked progress has been made in understanding the brain regions that are related to speech (informational) masking of target speech in healthy people (Scott et al. Reference Scott, Rosen, Wickham and Wise2004, Reference Scott, Rosen, Beaman, Davis and Wise2009; Ding & Simon, Reference Ding and Simon2012; Scott & McGettigan, Reference Scott and McGettigan2013; Evans et al. Reference Evans, McGettigan, Agnew, Rosen and Scott2016). For example, the study of Evans et al. (Reference Evans, McGettigan, Agnew, Rosen and Scott2016) showed that both the mid-posterior superior temporal gyrus (STG) and the superior temporal sulcus exhibit higher activity as informational content of masking sounds is increased, suggesting that masking speech is processed within the same pathway for processing target speech. However, only a very small number of studies have been reported in the literature on brain substrates underlying perceptual cue (spatial or non-spatial)-induced unmasking of speech recognition in either healthy listeners or listeners with schizophrenia (Zheng et al. Reference Zheng, Wu, Li, Wu, She, Liu, Wu, Mao, Ning and Li2016). Using functional magnetic resonance imaging (fMRI), in both healthy listeners and listeners with schizophrenia, this study was to search for: (1) the brain substrates specifically underlying the unmasking effect of ASP; and (2) the brain mechanisms underlying schizophrenia-related speech-recognition deficits under speech-masking conditions.
Method
Participants
With the recruiting criteria used in our previous studies (Wu et al. Reference Wu, Cao, Zhou, Wang, Wu and Li2012a , Reference Wu, Li, Tian, Wu, Wang and Li2013b ; Zheng et al. Reference Zheng, Wu, Li, Wu, She, Liu, Wu, Mao, Ning and Li2016), participants with schizophrenia, who were diagnosed with the Structured Clinical Interview for DSM-IV (SCID) (First et al. Reference First, Gibbon, Spitzer and Williams1996), were recruited in the Guangzhou Brain Hospital. Patients with diagnoses of schizo-affective or other psychotic disorders were not included. Demographics-matched healthy listeners (selected for controls) were recruited from the community around the hospital with the recruiting criteria used previously (Wu et al. Reference Wu, Cao, Zhou, Wang, Wu and Li2012a , Reference Wu, Li, Tian, Wu, Wang and Li2013b ; Zheng et al. Reference Zheng, Wu, Li, Wu, She, Liu, Wu, Mao, Ning and Li2016). They were telephone interviewed first and then those who passed the interview were screened with the SCID as used for patient participants. None of the selected healthy controls had either a history of Axis I psychiatric disorder as defined by the DSM-IV or a family history of psychiatric illness based on self-report.
Note that some potential patient participants were excluded from this study if they had co-morbid diagnoses, substance dependence and/or other conditions that affected experimental tests [including hearing loss, abuse and/or dependence of alcohol and/or drugs, a treatment of electroconvulsive therapy (ECT) within the past 3 months, a treatment of trihexyphenidyl hydrochloride with a dose of more than 6 mg/day, and/or an age younger than 18 years or older than 59 years]. For the purpose of improving sleeping, some of the patient participants received benzodiazepines based on doctors’ advice.
Both patient participants and their guarantees gave their written informed consent for participation in this study. Demographics-matched healthy listeners (controls) were recruited from the community around the hospital with the recruiting criteria used previously (Wu et al. Reference Wu, Cao, Zhou, Wang, Wu and Li2012a ). The procedures of this study were approved by the Independent Ethics Committee of the Guangzhou Brain Hospital.
In all, 17 healthy participants and 25 patients participated in the study. One healthy listener and three patients were excluded from data analyses due to their excessive head movement (more than 2.5 mm in translation and/or 2.5° in rotation from the first volume in any axis) during fMRI scanning. The remaining 16 healthy listeners (seven males and nine females) and 22 patients (14 males and 8 females) were included in both behavioral testing and fMRI data analyses. All participants were right-handed with normal pure-tone hearing thresholds at each ear (<30 dB hearing level) at frequencies between 125 and 8000 Hz. Their first language was Mandarin Chinese.
All patient participants were clinically stable during their participation and they received antipsychotic medications during this study with the average chlorpromazine equivalent of 521 mg/day based on the conversion factors described by Woods (Reference Woods2003). The locally validated version of the Positive and Negative Syndrome Scale Test (Si et al. Reference Si, Yang, Shu, Wang, Kong, Zhou and Li2004) was conducted on the day of fMRI scanning for all participants. The speech recognition test was conducted after the fMRI scanning. The characteristics of the patients and healthy participants are shown in online Supplementary Table S1.
Stimuli and procedures
There were three types of speech stimuli in total: target speech, masking speech, and priming speech. Spoken by a young female talker (Talker A), target speech stimuli were Chinese nonsense phrases with three words and each word contained two syllables. They were syntactically ordinary but not semantically meaningful (see Wu et al. Reference Wu, Li, Tian, Wu, Wang and Li2013b ). For example, the English translation of a phrase is ‘retire his ocean’ (keywords are in italics). Obviously, the sentence frame provided no contextual support for recognizing keywords. The speech masker was a 47-s loop of digitally combined continuous recordings for Chinese nonsense sentences (whose keywords did not appear in target phrases) spoken by two other young female talkers (Talkers B and C; Yang et al. Reference Yang, Chen, Huang, Wu, Wu, Schneider and Liang2007). There were two types of priming conditions: (1) ASP condition – the prime (stimulus) was identical to the target phrase except that the last (second) keyword was replaced by a white noise with both the duration of the longest second keyword among the target phrases and the sound level 10 dB lower than that of target stimuli (following Freyman et al. Reference Freyman, Balakrishnan and Helfer2004); (2) auditory non-speech priming (ANSP) condition (i.e. the speech-priming control condition) – the prime was a white noise with both the duration of the longest target phrase and the sound level 10 dB lower than that of target stimuli. In addition, during fMRI scanning a non-speech baseline stimulation condition (the controlling condition for both ASP and ANSP conditions) was used with the presentation of white noise whose duration was equal to that of the longest target phrase and whose sound level was 10 dB lower than that of target stimuli.
The whole-course scanning consisted of a 10-min auditory-priming functional run and an 8-min structure-scanning run. An event-related fMRI design was used for the functional run. In total, there were 60 scanning trials for the functional run (20 trials for each of the three conditions: ASP, ANSP, and baseline stimulation). For an individual participant, the 60 trials across the three conditions were presented with a random order. The sparse-imaging strategy was used to avoid the effect of machine scanning noise: Sound stimuli were presented only during the pause period between successive scanning periods (Hall et al. Reference Hall, Haggard, Akeroyd, Palmer, Summerfield, Elliott, Gurney and Bowtell1999). Also, to ensure that stimulus-evoked hemodynamic responses peaked within the scanning period (Wild et al. Reference Wild, Davis and Johnsrude2012), the stimulus presentation was so temporally positioned that the midpoint of stimulus presentation occurred about 4200 ms before the onset of the next scanning.
In a scanning trial for examining the priming effect (Fig. 1), either the ASP stimulus or the ANSP stimulus was presented in quiet (without the masker presentation) 600 ms after the offset of the last scanning trial. Immediately after the prime presentation, the target and masker were co-presented and terminated simultaneously. To maintain participants’ attention to stimulus presentation, a two-syllable word, either the last keyword in the target speech phrase (with the 50% possibility) or a different word was presented for 500 ms after the co-presentation of the target and masker speech. The participant was instructed to use a button-press with their right index finger to indicate whether the word on screen was the last keyword in the target-speech phrase.

Fig. 1. Illustration of a functional scanning run that comprised 60 trials [20 trials for each of the three conditions: auditory speech priming (ASP), auditory non-speech priming (ANSP), and non-speech stimulation baseline] presented in random order. Sparse temporal sampling scanning was used. Trial structures of each of the three conditions for the functional run are illustrated separately. The temporal midpoint of the sound stimulus was presented 4200 ms prior to the onset of the next scanning. TR, Time to repeat.
The sound stimuli used in the fMRI scanning experiment were presented through a magnetic resonance-compatible pneumatic headphone system (SAMRTEC, China) driven by Presentation software (version 0.70) without introducing interaural time differences. The target-speech level was 60 dB sound pressure level (SPL) (after attenuation by earplugs) and the SMR was −4 dB. Visual stimuli were presented through a liquid crystal display screen positioned on the head coil (SAMRTEC, China). A brief training was conducted to ensure that participants understood the instruction and knew how to conduct their button-press responses. Speech sentences used in training were different from those in experimental scanning.
A 3.0-Tesla Philips Achieva MRI scanner (Veenpluis 4-6,5680 DA Best, the Netherlands) was used to acquire blood oxygenation level-dependent (BOLD) gradient echo-planar images (spatial resolution: 64 × 64 × 33 matrix with the voxel size of 3.44 × 3.44 × 4.6 mm3; acquisition time: 2000 ms; time to repeat: 9000 ms; echo time: 30 ms; flip angle: 90°; field of view: 211 × 211 mm2). It provided high-resolution T1-weighted structural images (256 × 256 × 188 matrix with a spatial resolution of 1 × 1 × 1 mm3, repetition time: 8.2 ms; echo time: 3.8 ms; flip angle: 7°).
fMRI data processing and analyses
Pre-processing
All fMRI data were processed and analysed using Statistical Parametric Mapping software (SPM8; the Wellcome Trust Centre for Neuroimaging, UK). The pre-processing of data includes the following four stages: (1) the functional images were corrected for head movements; (2) the anatomical images were co-registered with the mean realigned images and normalized to the standard template [UCLA Brain Mapping Center (ICBM) space] using the SPM8 unified segmentation routine; (3) all functional images were warped using deformation parameters generated from the normalization process, including re-sampling to a voxel size of 3.0 × 3.0 × 4.0 mm3; and (4) spatial smoothing was conducted using a Gaussian kernel with 8 mm full-width at half maximum (FWHM). Due to the long time to repeat this sparse-imaging paradigm, no slice timing was necessary.
Random-effect analyses
Random-effect analyses contained two processing levels. At the first level, the onsets and durations for the functional run were modeled using a general linear model according to the condition types. The three stimulation conditions (ASP, ANSP and baseline) were included in the model. Six realignment parameters of head movement were included to account for residual movement-related effects (Friston et al. Reference Friston, Williams, Howard, Frackowiak and Turner1996). At the second level, random-effect analyses were conducted based on the statistical parameter maps from each individual participant to allow population inference.
To assess the overall main effects of participant group and prime type, and the interaction, both the contrast image of ‘ASP > baseline’ and that of ‘ANSP > baseline’ from each participant were entered into a second-level 2 × 2 (group × prime type) full-factor analysis of variance (ANOVA). F contrasts were used.
To localize the brain regions activated by speech stimulation conditions, the contrast images of ‘ASP > baseline’ and that of ‘ANSP > baseline’ from the first-level analyses in each participant were entered into second-level one-sample t tests for the patient group and the healthy-control group, respectively.
To reveal the brain regions related to the ‘ASP effect (ASP > ANSP)’ in each participant group, contrast images of ‘ASP > ANSP’ from the first-level analyses in each participant were entered into the second-level group one-sample t tests in the healthy control group and the patient group, separately. For whole-brain analyses, peak signals that were statistically significant at the p value less than 0.05 [family-wise error (FWE) corrected] were reported.
Psychophysiological interaction (PPI) analyses
PPI analyses (Friston et al. Reference Friston, Buechel, Fink, Morris, Rolls and Dolan1997) were performed to identify the brain regions showing significantly increased functional connectivity with the most critical brain structures (seeds) related to the ASP condition compared with the ANSP condition. The coordinates of the peak voxel from the contrast of ‘ASP > ANSP’ in random-effect analyses were used as the landmarks for the individual seed voxels. A seed region in each participant was defined as a sphere with a 5 mm radius centered at the peak voxel. The time series of seed regions were then extracted, and the PPI regressors, which reflected the interaction between psychological variable (ASP v. ANSP) and the activation time course of the seed regions, were calculated.
The individual contrast images, which reflected the effects of PPI between the seed regions and other brain areas, were subsequently subjected to the second-level one-sample t tests in each of the participant groups to identify the brain regions showing increased co-variation with the activity of the seed regions in analyses of the ASP condition against the ANSP condition. Then individual participants’ contrast images were entered into the second-level two-sample t tests for group comparisons. In PPI analyses, peak signals that were statistically significant at a p value less than 0.05 (FDR corrected) were reported.
Functional connectivity analyses (partial correlation)
It has been established that partial correlation can be used as an effective measure of functional connectivity between a given pair of brain regions by attenuating the contribution of other sources of covariance (Hampson et al. Reference Hampson, Peterson, Skudlarski, Gatenby and Gore2002; Liu et al. Reference Liu, Liang, Zhou, He, Hao, Song, Yu, Liu, Liu and Jiang2008, Reference Liu, Yu, Zhang, Liu, Duan, Alexander-Bloch, Liu, Jiang and Bullmore2014). In this study, to search for schizophrenia-related changes in functional connectivity of speech processing-related brain regions, both the activation clusters from ‘ASP > baseline’ and those from ‘ANSP > baseline’ SPM files in healthy controls and patient participants were extracted and used as the seed regions for examining the functional connectivity (p < 0.05, FWE corrected) (MarsBaR: region of interest toolbox for SPM; http://marsbar.sourceforge.net/). The clusters representing the same brain region activated by ‘ASP > baseline’ and ‘ANSP > baseline’ in patients and controls were combined as the seed for functional connectivity analyses of this brain region. The correlation coefficient obtained by partial correlation between a pair of variables after filtering out contributions from all other variables was included in the dataset (Salvador et al. Reference Salvador, Suckling, Schwarzbauer and Bullmore2005; Liu et al. Reference Liu, Liang, Zhou, He, Hao, Song, Yu, Liu, Liu and Jiang2008, Reference Liu, Yu, Zhang, Liu, Duan, Alexander-Bloch, Liu, Jiang and Bullmore2014) and converted to Z-scores with the Fischer transform as the strengths of functional connectivity. Thus, significant differences in functional connectivity between healthy controls and patients were identified by significant differences in Z-score (see Liu et al. Reference Pu, Rolls, Guo, Liu, Yu, Xue, Feng and Liu2014).
Correlation between speech-recognition performance and functional connectivity
Spearman correlation analyses were performed using SPSS 16.0 software to investigate the correlation between the behavioral performance (averaged percentage correct of target speech recognition across the ASP and ANSP listening conditions) and the strength of functional connectivity (Z-scores of functional connectivity) of a pair of brain regions. The null hypothesis was rejected at the level of 0.05.
Behavioral testing
The behavioral testing was conducted after the fMRI scanning experiment. Acoustic signals, calibrated by a sound-level meter (AUDit and System 824; Larson Davis, Inc., USA), were delivered from a notebook-computer sound card (ATI SB450 AC97) and presented to participants via earphones (model HDA 600). The target speech level was 60 dB SPL. The SPL of the speech masker was adjusted to produce two SMRs: −4 and −8 dB. There were two within-subject variables: (1) priming type (ASP, ANSP), and (2) SMR (−8, −4 dB). For each participant, there were four testing conditions and 20 trials (also 20 target-sentence presentations) for each condition. The presentation order for the four combinations of priming type and SMR were partially counterbalanced across participants using a Latin square order.
In a trial, the participant, who was seated at the center of a quiet room in the hospital, pressed the ‘Enter’ key on a computer keyboard to start the presentation of the auditory priming stimulus. Either an ASP stimulus or an ANSP stimulus was presented in quiet (without the masker presentation) after the key press. Immediately after the prime-presentation phrase, the target and masker were presented and terminated simultaneously. After the masker/target co-presentation was finished, the participant was instructed to loudly repeat the whole target phrases as best as he/she could. The experimenters, who sat quietly behind the participant, scored whether each of the two syllables for each of the two keywords in the target phrases had been identified correctly.
Target speech recognition performance was valued by the percentage of correct response of the second keywords in target phrases. The percentage correct scores were entered into ANOVAs or Spearman correlation with the strength of functional connectivity (Z-scores of functional connectivity) of brain region pairs using SPSS 16.0 software (USA). The null hypothesis was rejected at the level of 0.05.
Results
The speech-priming effect and the participant-group effect on speech recognition
Fig. 2 shows comparisons in group-mean percentage-correct recognition of the last target keyword (top panels) and those in group-mean ASP-induced speech-recognition improvement (bottom panels, the ASP effect: the difference in percentage correct between the ASP condition and the ANSP condition) between healthy listeners and patients when the SMR was either −4 or −8 dB.

Fig. 2. Top panels: Comparisons in group-mean percentage-correct recognition of target keywords between the healthy-listener group and the patient group when the signal:masker ratio (SMR) was either −4 or −8 dB. Bottom panels: Comparisons in group-mean auditory speech priming (ASP)-induced improvement in recognition of target keywords between the healthy listener group and the patient group when the SMR was either −4 or −8 dB. ANSP, Auditory non-speech priming. * p<0.05.
To statistically examine the differences in percentage of correct identification of target speech across experimental conditions, a 2 (group: control, patient) by 2 (priming type: ASP, ANSP) by 2 (SMR: −4, −8 dB) three-way ANOVA showed that the main effects of group (F 1,144 = 138.76, p < 0.001), priming type (F 1,144 = 13.27, p < 0.001) and SMR (F 1,144 = 52.27, p < 0.001) were all significant. However, all the two-way interactions and the three-way interaction were not significant. Post-hoc tests further confirmed that consistent with previous studies (Wu et al. Reference Wu, Cao, Zhou, Wang, Wu and Li2012a , Reference Wu, Li, Tian, Wu, Wang and Li2013b ), patients performed worse in speech recognition than healthy controls (Fig. 2 top panels) when SMR was either −4 dB (for each of the two priming conditions, p < 0.001) or −8 dB (for each of the two priming conditions, p < 0.001).
Moreover, similar to healthy listeners (Fig. 2 bottom panels), patients were able to use the ASP stimulus to improve their target-speech recognition when the SMR was either −4 dB (F 1,72 = 6.880, p = 0.011) or −8 dB (F 1,72 = 7.192, p = 0.009).
Brain regions activated by speech priming
A 2 (group: control, patient) by 2 (prime type: ASP, ANSP) ANOVA of brain-image data showed that the main effect of priming type (ASP v. ANSP) revealed significant activation in the bilateral STG, bilateral supplementary motor area (SMA), bilateral medial superior frontal area, left posterior middle temporal gyrus (pMTG), right inferior frontal gyrus (IFG), right insular, right precentral, right putamen and right caudate (Fig. 3 top panel and online Supplementary Table S2; F contrasts were significant at p < 0.05 with FWE correction). The main effect of group (control v. patient) elicited significant activation in the left anterior cingulate cortex, left caudate and right putamen at p < 0.001 uncorrected but not at p < 0.05 correction with FDR (Fig. 3 bottom panel). The interaction was not significant at either p < 0.001 uncorrected or p < 0.05 correction with FDR. To further explore the brain substrates underlying the ASP effects, we conducted one-sample t tests and PPI analyses in the healthy control group and the patient group separately.

Fig. 3. Top panels: Voxels that exhibited a main effect of priming type. The activation map is thresholded at p < 0.001 uncorrected and overlaid on the group-average structural image. The color scale indicates the p value corrected family-wise for type I error (FWE). Bottom panels: Voxels that exhibited a main effect of group type. The activation map is thresholded at p < 0.001 uncorrected and overlaid on the structural image averaged across control group and patient group; no voxels survive the p value false discovery rate corrected for type I error.
In healthy listeners, compared with the ANSP condition, introducing the ASP condition significantly enhanced BOLD signals in the bilateral STG, bilateral MTG, left pMTG and left putamen (p < 0.05, FWE corrected) (Fig. 4 a, online Supplementary Table S3).
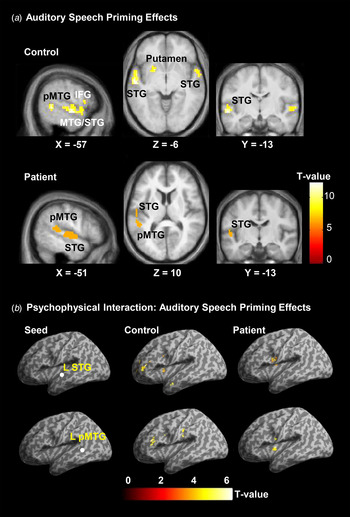
Fig. 4. (a) Activated brain regions associated with the contrast of the auditory speech priming (ASP) listening condition against the auditory non-speech priming (ANSP) listening condition in healthy listeners and patients with schizophrenia. The activation maps were thresholded at p < 0.05 (family-wise error corrected) and overlaid on the group-average structural image. (b) Psychophysiological interaction analyses in healthy listeners (middle column) and listeners with schizophrenia (right column) for revealing the ASP effect (ASP > ANSP)-related functional connectivity of the left superior temporal gyrus/middle temporal gyrus (STG/MTG). Locations of seed regions (left column) are overlaid on the template of SPM8, and the activation maps are overlaid on a template brain with inflated cortex of SPM8. All peaks are significant at p < 0.05 (false discovery rate corrected). pMTG, Posterior middle temporal gyrus; IFG, inferior frontal gyrus; L, left.
In patients, introducing the ASP condition (against the ANSP condition) significantly enhanced BOLD signals only in the left STG and left pMTG (p < 0.05, FWE corrected) (Fig. 4 a, online Supplementary Table S3). Thus, both the left STG and left pMTG were activated by the ASP > ANSP listening-condition contrast in both healthy listeners and patients.
We also analysed the data in which the incorrect button-press trials were removed in each participant. For healthy controls, due to the high correct button-press performance (online Supplementary Fig. S1), the brain-image results without incorrect button press trials were similar to those with both correct trials and incorrect trials (online Supplementary Fig. S2 bottom panels and online Supplementary Table S4). For patient participants, however, activation in the bilateral STG, left MTG and left pMTG became enhanced by removal of incorrect trials (online Supplementary Fig. S2 bottom panels and online Supplementary Table S4).
Functional connectivity of the left STG/pMTG in speech priming
Since both the left STG and left MTG were activated by the ASP > ANSP condition contrast in both healthy listeners and patients, PPI analyses were then conducted to identify the brain regions showing enhanced co-variation specifically with the left STG/MTG induced by the ASP > ANSP contrast. In healthy listeners, enhanced functional connectivity was observed (1) between the left STG and the following brain regions: the left middle frontal gyrus, left pars triangularis of the IFG (TriIFG), left insular, left caudate, left MTG and bilateral putamen, and (2) between the left pMTG and the following brain regions: the left TriIFG, left insular, left caudate and left supra marginal area (Fig. 4 b, online Supplementary Table S5).
In patients, enhanced functional connectivity included the ones (1) between the left STG and the following two brain regions: the left Rolandic operculum and the left caudate, and (2) between the left pMTG and the following two brain regions: the left Rolandic operculum and the left caudate (Fig. 4b , online Supplementary Table S5).
Thus, the left caudate was the only brain structure showing significantly enhanced functional connectivity with both the left STG and left pMTG in both healthy listeners and patients. Moreover, particularly in healthy listeners, the left STG/pMTG had functional connectivity with the left TriIFG and left insular; particularly in patients, the left STG/pMTG had functional connectivity with the left Rolandic operculum. No significant differences in PPI were found between healthy controls and patients in two-sample t tests.
Functional connectivity related to speech recognition under speech-masking conditions
Brain regions activated by either the ASP listening condition or the ANSP listening condition
As mentioned in the Introduction, listeners with schizophrenia perform worse than healthy listeners in speech recognition against informational masking (Wu et al. Reference Wu, Cao, Zhou, Wang, Wu and Li2012a , Reference Wu, Li, Tian, Wu, Wang and Li2013b ). In this study, the (non-speech) noise baseline-stimulation condition was used as the reference for examining the brain regions and functional connectivity induced by the speech-listening conditions.
In both healthy listeners and patient listeners, compared with the (non-speech) noise baseline-stimulation condition, the ASP listening condition significantly enhanced BOLD signals in the bilateral STG, left putamen, left TriIFG, right precentral and right SMA (p < 0.05, FWE corrected) (online Supplementary Fig. S3 and online Supplementary Table S6). In addition, specifically in healthy controls, the activated brain regions also included the right putamen and bilateral thalamus. Specifically in patients, the activated brain regions also included the left precentral, left inferior parietal lobule (IPL), right insular, right lingual and vermis of cerebellum (p < 0.05, FWE corrected).
Also as shown by online Supplementary Fig. S3 and online Supplementary Table S6, compared with the non-speech baseline-stimulation condition, in healthy controls the ANSP condition significantly enhanced BOLD signals in the bilateral STG, right putamen, and right SMA, left precentral and left TriIFG (p < 0.05, FWE corrected). In patients, the ANSP condition significantly enhanced BOLD signals in the bilateral STG, bilateral insular, left pars opercularis of the IFG (OperIFG) , left IPL, left postcentral, left cerebellum, right mid-cingulate cortex (MCC), right precentral and right lingual cortex (p < 0.05, FWE corrected).
Schizophrenia-related changes in functional connectivity for speech listening
Based on the results of brain regions activated by either the ASP listening condition (ASP > baseline) or the ANSP listening conditions (ANSP > baseline), functional connectivity of these activated brain areas were examined using partial correlation analyses. The brain region clusters activated by either the ASP- or ANSP-listening condition (against the non-speech baseline listening condition) were used as the seed regions (online Supplementary Table S6) (p < 0.05, FWE corrected) for conducting the comparison in functional connectivity between healthy listeners and patients. More specifically, in a brain region, clusters activated by the ASP-listening condition and those by the ANSP-listening condition were combined across healthy listeners and patient listeners to form a seed region for functional connectivity analyses (for example, all the four activated STG clusters shown in online Supplementary Table S6 across the two priming conditions and the two participant groups were combined to form the STG seed region for the functional connectivity analyses). In total, 20 clusters of seed regions were obtained and used for examining speech recognition-related functional connectivity in both healthy controls and patients: the left STG, right STG, left putamen, right putamen, left TriIFG, right TriIFG, left OperIFG, left precentral, right precentral, right SMA, left insular, right insular, left IPL, left postcentral, right MCC, left thalamus, right thalamus, right lingual, left cerebellum and vermis.
Then Z-scores (which represented the strength of functional connectivity) were obtained based on partial correlation analyses between time series of the total of 190 seed-region pairs for each participant, and compared between healthy controls and patients to reveal the significantly changed functional connectivity (which was related to the schizophrenia-related poor performance in target-speech recognition). Fig. 5a shows Z-scores of all the seed-region pairs whose strengths of functional connectivity were significantly lower in patients than in healthy listeners.

Fig. 5. (a) Strengths of functional connectivity (Z-score) of brain seed-region pairs, which were significantly different between healthy listeners and patients. (b) and (c) Individual participants’ Z-scores for functional connectivity (the abscissa) between the left superior temporal gyrus (L STG) and the left pars triangularis of the inferior frontal gyrus (L TriIFG) were significantly correlated with individual participants’ percentage correct recognition of target speech (the ordinate) in the patient group when the signal:masker ratio (SMR) was −4 or −8 dB, and in the healthy listener group when the SMR was −8 dB. R, Right; Pu, putamen; PreC, precentral.
Correlation between strength of functional connectivity and speech-recognition performance
Spearman correlation analyses were conducted between the percentage correct of target-speech recognition and each of the Z-scores for the seed-region pairs shown in Fig. 5a in both healthy listeners and patients. The results showed that the percentage correct of target-speech recognition was significantly correlated only with the Z-score for functional connectivity between the left STG and the left TriIFG in healthy controls when the SMR was −8 dB (r = 0.512, p = 0.048) and in patients when the SMR was either –8 dB (r = 0.488, p = 0.021) or −4 dB (r = 0.552, p = 0.008) (Fig. 5b and c ). Also, not only was speech-recognition performance in individual patients generally poorer than that in individual healthy listeners, but also the Z-scores (representing the strength of functional connectivity) in individual patients were lower than those in individual healthy listeners.
Discussion
Brain substrates activated by ASP
This study confirms previous reports that in both healthy listeners (Freyman et al. Reference Freyman, Balakrishnan and Helfer2004; Yang et al. Reference Yang, Chen, Huang, Wu, Wu, Schneider and Liang2007; Helfer & Freyman, Reference Helfer and Freyman2009; Huang et al. Reference Huang, Xu, Wu and Li2010; Wu et al. Reference Wu, Cao, Zhou, Wang, Wu and Li2012b , Reference Wu, Cao, Wu and Li2013a ) and listeners with schizophrenia (Wu et al. Reference Wu, Cao, Zhou, Wang, Wu and Li2012 Reference Wu, Li, Gao, Lei, Teng, Wu and Li b ), target-speech recognition against informational masking can be improved by temporally pre-presenting an early part of the target speech (i.e. the speech prime). The behavioral results of this study did not show a significant difference in the priming (unmasking) effect on speech recognition between the two participant groups. More importantly, this study for the first time reveals the brain substrates specifically underlying the speech prime-induced facilitating effect on speech recognition against speech masking in both healthy listeners and listeners with schizophrenia.
In healthy listeners, the BOLD-signal contrast between the ASP condition and the ANSP condition reveals significant enhancement of activation in the following brain regions: the bilateral STG, bilateral pMTG, left MTG, left superior temporal pole and left putamen. In listeners with schizophrenia, the significantly activated brain regions include the left STG and left pMTG. Since both the left STG and left pMTG are activated by the ASP > ANSP contrast in both healthy listeners and listeners with schizophrenia, these two temporal lobe areas must be the most critical to the unmasking effect of ASP.
It has been suggested that the STG, particularly the anterior part of the STG, is an early stage in the cortical network for speech perception, mediating speech-sound identity information (Hickok & Poeppel, Reference Hickok and Poeppel2004; Ahveninen et al. Reference Ahveninen, Jaaskelainen, Raij, Bonmassar, Devore, Hamalainen, Levanen, Lin, Sams, Shinn-Cunningham, Witzel and Belliveau2006; Rauschecker & Scott, Reference Rauschecker and Scott2009, Reference Rauschecker, Scott, Hickok and Small2015). The involvement of the left STG in speech perception at the phonetic, lexical–semantic and syntactic levels has been confirmed by both brain imaging (Friederici et al. Reference Friederici, Ruschemeyer, Hahne and Fiebach2003; Scott & Wise, Reference Scott and Wise2003) and functional lesion studies (Boatman, Reference Boatman2004). The left pMTG is involved in retrieval of lexical–syntactic information from the mental lexicon in long-term memory (Snijders et al. Reference Snijders, Vosse, Kempen, Van Berkum, Petersson and Hagoort2009) and has a functional relationship with the left IFG in both processing syntactic structures and constructing grammatical representations of spoken language (Tyler et al. Reference Tyler, Wright, Randall, Marslen-Wilson and Stamatakis2010, Reference Tyler, Cheung, Devereux and Clarke2013; Walz et al. Reference Walz, Goldman, Carapezza, Muraskin, Brown and Sajda2010; Papoutsi et al. Reference Papoutsi, Stamatakis, Griffiths, Marslen-Wilson and Tyler2011). Also, the left pMTG is involved in sentence-level prosody processing in Chinese-speech perception (Tong et al. Reference Tong, Gandour, Talavage, Wong, Dzemidzic, Xu, Li and Lowe2005). Moreover, previous studies have confirmed both the function of the left rostral STG in working memory of sound identities (Arnott et al. Reference Arnott, Grady, Hevenor, Graham and Alain2005) and the function of the left pMTG in the storage of lexical representation (Lau et al. Reference Lau, Phillips and Poeppel2008). These memory functions of the left STG/pMTG are particularly critical to the memory-based speech priming effect. Thus, the speech priming-induced activation of the left STG/pMTG indicates a speech priming-related facilitation of cortical representation of target-speech signals.
It should be noted that in this study, only younger-adult females’ voices were used. Since previous studies have shown that male and female voices are represented as distinct auditory objects in the human non-primary auditory cortex (Weston et al. Reference Weston, Hunter, Sokhi, Wilkinson and Woodruff2015), it is necessary in the future to examine whether similar results can be obtained when male-voice stimuli are used.
ASP-related functional connectivity of the left STG/pMTG
Relative to the ANSP condition, the ASP condition enhances functional connectivity of the left STG/pMTG with the left caudate in both healthy listeners and listeners with schizophrenia. In addition, introducing the ASP condition (against the ANSP condition) enhances functional connectivity of the left STG/pMTG with the left TriIFG, MFG, supramarginal gyrus, insular cortex and putamen in healthy listeners, and with the left Rolandic operculum in listeners with schizophrenia.
The ASP-related functional connectivity of the left STG/pMTG in healthy listeners suggests some critical mechanisms normally underlying the unmasking effect of ASP: (1) since the caudate contributes to speech inhibition and even more general response inhibition (Menon et al. Reference Menon, Adleman, White, Glover and Reiss2001; Ketteler et al. Reference Ketteler, Kastrau, Vohn and Huber2008; Li et al. Reference Li, Yan, Sinha and Lee2008; Ali et al. Reference Ali, Green, Kherif, Devlin and Price2010) and the left IFG plays a role in signal selections among competing sources (Thompson-Schill et al. Reference Thompson-Schill, Bedny and Goldberg2005), the unmasking effect of ASP should be based on both selective attention to target speech and suppression of disruptive speech signals; (2) the left IFG also contributes to multiple speech processes, including syntactic unifications on the basis of interplay with the left pMTG, speech meaning selection, sentence re-interpretation, and speech production (Herholz et al. Reference Herholz, Thiel, Wienhard, Pietrzyk, von Stockhausen, Karbe, Kessler, Bruckbauer, Halber and Heiss1996; Paulesu et al. Reference Paulesu, Goldacre, Scifo, Cappa, Gilardi, Castiglioni, Perani and Fazio1997; Papathanassiou et al. Reference Papathanassiou, Etard, Mellet, Zago, Mazoyer and Tzourio-Mazoyer2000; Schuhmann et al. Reference Schuhmann, Schiller, Goebel and Sack2009; Snijders et al. Reference Snijders, Vosse, Kempen, Van Berkum, Petersson and Hagoort2009; Rodd et al. Reference Rodd, Johnsrude and Davis2012). Since the involvement of the speech-motor system in speech perception is important for facilitating speech perception particularly under ‘cocktail party’ listening conditions (Wu et al. Reference Wu, Chen, Wu and Li2014), functional connectivity of the left STG/pMTG with the left TriIFG normally contributes to the unmasking effect of ASP through not only facilitating masking-speech suppression and target-speech representation (e.g. syntactic unification), but also inducing speech production.
The results of this study reveal that introducing the ASP condition (against the ANSP condition) both activates the STG/pMTG and enhances functional connectivity between the STG/pMTG and the frontal cortex. Thus, it is of importance to know whether the enhanced functional connectivity between the STG/pMTG and the frontal cortex indicates a top-down modulation mechanism underlying the ASP-induced unmasking of speech.
It is known that the left Rolandic operculum is involved in sentence-level speech prosody processing (Ischebeck et al. Reference Ischebeck, Friederici and Alter2008), speech production (Tonkonogy & Goodglass, Reference Tonkonogy and Goodglass1981), and syntactic encoding during speech production (Indefrey et al. Reference Indefrey, Brown, Hellwig, Amunts, Herzog, Seitz and Hagoort2001). It is not clear whether this schizophrenia-enhanced functional connectivity between the left STG/pMTG and the left Rolandic operculum reflects schizophrenia-related neural plasticity underlying the unmasking effect of the speech-priming stimulus. The compensatory mechanism specifically underlying schizophrenia is an important issue (Tan et al. Reference Tan, Callicott and Weinberger2007).
Functional connectivity specifically related to schizophrenia-induced deficits in speech recognition against informational masking
The behavioral-testing results of this study support previous reports that under ‘cocktail party’ listening conditions (Schneider et al. Reference Yang, Chen, Huang, Wu, Wu, Schneider and Liang2007), listeners with schizophrenia perform poorly in recognizing target speech (Wu et al. Reference Wu, Cao, Zhou, Wang, Wu and Li2012a , Reference Wu, Li, Tian, Wu, Wang and Li2013b ; Zheng et al. Reference Zheng, Wu, Li, Wu, She, Liu, Wu, Mao, Ning and Li2016). This study also reveals the brain substrates underlying the schizophrenia-related deficits in speech recognition under the priming conditions: The strength of functional connectivity between a number of speech-processing-related brain regions (including the STG, MTG, putamen, TriIFG, IPL, precentral cortex and SMA) declines in listeners with schizophrenia. Particularly, in listeners with schizophrenia, the strength of functional connectivity between the left STG/pMTG and the left TriIFG is not only correlated with speech-recognition performance but is also lower than that in healthy listeners. Iwashiro et al. (Reference Iwashiro, Suga, Takano, Inoue, Natsubori, Satomura, Koike, Yahata, Murakami, Katsura, Gonoi, Sasaki, Takao, Abe, Kasai and Yamasue2012) have shown that the gray matter volume reduces in the TriIFG in both individuals at clinical high-risk for psychosis and those with first episode of schizophrenia. The declined functional connectivity between the left STG/pMTG and left TriIFG as revealed by this study further confirms the schizophrenia-related functional deficits in the left TriIFG.
In addition to the involvement of the STG, MTG and TriIFG in speech processing as discussed above, the putamen engages in both syntactic processing (Friederici et al. Reference Friederici, Ruschemeyer, Hahne and Fiebach2003) and speech initiation through its functional connectivity with both the left IFG and the left lateral temporal cortex (Booth et al. Reference Booth, Wood, Lu, Houk and Bitan2007). Moreover, the IPL is involved in interpreting sensory information (Zhang & Li, Reference Gao, Tao, Zhang and Chen2014) and works as a sensorimotor interface between the representation of acoustic signals in the auditory cortex and the prediction of sensory consequences of articulatory gestures in the premotor cortex (Olson & Berryhill, Reference Olson and Berryhill2009; Price, Reference Price2012; Du et al. Reference Du, Buchsbaum, Grady and Alain2014). Also, the precentral gyrus is part of the premotor cortex involved in speech processing (Callan et al. Reference Callan, Callan, Gamez, Sato and Kawato2010) including phoneme segmentation (Sato et al. Reference Sato, Tremblay and Gracco2009), categorical speech perception in noise (Du et al. Reference Du, Buchsbaum, Grady and Alain2014) and speech production (Price, Reference Price2012). Finally, the SMA plays a role in planning, preparing, controlling and executing complex movements (Nachev et al. Reference Nachev, Kennard and Husain2008; Price, Reference Price2012; Laviolette et al. Reference Laviolette, Niérat, Hudson, Raux, Allard and Similowski2013; Gao et al. Reference Gao, Tao, Zhang and Chen2014). The reduced strength of functional connectivity between the STG, MTG, putamen, TriIFG, IPL, precentral cortex and SMA may also account for the declined speech recognition under speech-masking conditions in listeners with schizophrenia. Indeed, previous studies have shown that functional connectivity between these brain regions is not only involved in auditory and/or speech processing (Jeong et al. Reference Jeong, Wible, Hashimoto and Kubicki2009; Mhuircheartaigh et al. Reference Mhuircheartaigh, Rosenorn-Lanng, Wise, Jbabdi, Rogers and Tracey2010; Price, Reference Price2010; Turken & Dronkers, Reference Turken and Dronkers2011; Sundermann & Pfleiderer, Reference Sundermann and Pfleiderer2012; Zhang & Li, Reference Liu, Yu, Zhang, Liu, Duan, Alexander-Bloch, Liu, Jiang and Bullmore2014; Kireev et al. Reference Kireev, Slioussar, Korotkov, Chernigovskaya and Medvedev2015; Munoz-Lopez et al. Reference Munoz-Lopez, Insausti, Mohedano-Moriano, Mishkin and Saunders2015) but also impaired in people with schizophrenia (Ford et al. Reference Ford, Mathalon, Whitfield, Faustman and Roth2002; Liu et al. Reference Liu, Kaneko, Ouyang, Li, Hao, Chen, Jiang, Zhou and Liu2012; Leroux et al. Reference Leroux, Delcroix and Dollfus2014; Pu et al. Reference Pu, Rolls, Guo, Liu, Yu, Xue, Feng and Liu2014; Zhang & Li, Reference Zhang and Li2014). Moreover, this study reveals that the strength of functional connectivity between the left STG and left TriIFG is significantly correlated with the speech-recognition performance under the informational masking condition in both healthy listeners and listeners with schizophrenia, and reduces in listeners with schizophrenia. More studies are needed to further examine how schizophrenia-related deficits in functional connectivity between the left STG and left TriIFG affect recognition of speech under the informational masking condition.
Conclusions
-
(1) Under ‘cocktail party’ listening conditions, both healthy listeners and listeners with schizophrenia are able to use temporally pre-presented ASP stimuli to improve their target-speech recognition against informational speech masking. The most critical brain regions underlying this unmasking effect are the left STG and the left pMTG in both healthy listeners and listeners with schizophrenia.
-
(2) In both healthy listeners and listeners with schizophrenia, the left STG/pMTG has ASP-related functional connectivity with the left caudate, suggesting that the inhibitory function of the left caudate is involved in the unmasking effect of ASP.
-
(3) Normally, the left STG and left pMTG have ASP-related functional connectivity with the left TriIFG, suggesting an enhanced functional integration among the following three processes: speech-signal selection, speech-signal representation and speech production.
-
(4) In listeners with schizophrenia, enhanced ASP-related functional connectivity of the left STG/pMTG and the left Rolandic operculum may indicate an ASP-related facilitation in sentence prosody processing and speech production.
-
(5) The poor performance of speech recognition against informational masking in people with schizophrenia is associated with reduced strength of functional connectivity between a number of speech-processing-related brain regions, particularly associated with the impaired functional connectivity between the left STG and the left TriIFG.
Supplementary material
The supplementary material for this article can be found at https://doi.org/10.1017/S0033291716002816
Acknowledgements
Huahui Li assisted in many aspects of this work. This work was supported by the ‘973’ National Basic Research Program of China (2015|CB351800, 2011CB707805); the National High Technology Research and Development Program of China (863 Program: 2015AA016306); the National Natural Science Foundation of China (31170985); the Planned Science and Technology Projects of Guangzhou (2014Y2-00105); the Guangzhou Municipal Key Disciplines in Medicine for Guangzhou Brain Hospital (GBH2014-QN04, GBH2014-ZD06); the Chinese National Key Clinical Program in Psychiatry (201201004); and the China Postdoctoral Science Foundation General Program (2013M530453).
Declaration of Interest
None.