Introduction
Rice blast disease, caused by the fungus Magnaporthe oryzae, remains one of the most important constraints to rice production worldwide. During the past decade, rice blast epidemics have occurred in many countries, especially in the important rice cultivation area. This is despite the availability of many blast disease resistance genes. More than 100 blast resistant genes have been identified (Hayashi and Yoshida, Reference Hayashi and Yoshida2009). Pid3 was identified from a comparison of nucleotide-binding sites–leucine-rich repeat (NBS–LRR) sequences between the indica rice variety, ‘Digu’ and the japonica rice variety, ‘Nipponbare’. It encodes a 924-amino-acid polypeptide. The allele of the Pid3 gene in ‘Nipponbare’, was identified as a pseudogene due to a nonsense mutation at the nucleotide position 2208. However, this mutation was not found in any indica rice varieties, African cultivated rice varieties, or in AA genome-containing wild rice species (Shang et al., Reference Shang, Tao, Chen, Zou, Lei, Wang, Li, Zhao, Zhang, Lu, Xu, Cheng, Wan and Zhu2009).
Pigm resistant gene was located on chromosome 6 (Deng et al., Reference Deng, Zhu, Shen and He2006). It was introduced from Chinese indica rice cultivar Gumei4 (GM4). Pigm locus is a cluster of 13 NLR genes including three intact transcribed NLRs (R4, R6 and R8), ten potential pseudogenes and one Ty3/gypsy retrotransposon (Deng et al., Reference Deng, Zhai, Xie, Yang, Zhu, Liu, Wang, Qin, Yang, Zhang, Li, Zhang, Wu, Milazzo, Mao, Wang, Xie, Tharreau and He2017). The allelism test showed that Pigm was allelic to Pi2 and Pi9 (Deng et al., Reference Deng, Zhu, Shen and He2006). Resistance spectrum test showed that Pigm confers broader-spectrum resistance to blast isolates from different rice growing regions than Pi9/Pi2/Pizt/Piz (Deng et al., Reference Deng, Zhu, Xu, Chen, He, Wang and Valent2009).
Pi54 (which was earlier known as Pikh) is one of the major blast resistant genes and has been observed to show resistance against many isolates of the blast pathogen in India (Sharma et al., Reference Sharma, Madhav, Singh, Shanker, Jana, Dalal, Pandit, Singh, Gaik and Singh2005). The Pi54 gene was originally identified from rice variety ‘Tetep’ and mapped on chromosome 11 (Xu et al., Reference Xu, Hayashi, Wang, Kato, Fujimura and Kawasaki2008). The gene has been cloned through map-based strategy and it encodes a nucleotide-binding site – leucine-rich repeat (NBS–LRR) domain-containing protein (Ramkumar et al., Reference Ramkumar, Srinivasarao, Mohan, Sudarshan, Sivaranjani, Gopalakrishna, Neeraja, Balachandran, Sundaram, Prasad, Shobha Rani, Rama Prasad, Viraktamath and Adhav2011). Pi54 was found to be widely distributed in several cultivars such as ‘Charnak’ (India), ‘Tetep’ (Vietnam), ‘Tadukan’ (Philippine), ‘Roshia 33’ (Russian), ‘Dawn’ (America) and ‘Fuji120’, ‘Mutsunishiki’ and ‘Chugoku31’ (Japan) (Kiyosawa, Reference Kiyosawa1972).
DNA markers offer an efficient and rapid means of selecting for the presence of multiple blast resistant genes without performing extensive progeny testing or disease screening (Fjellstrom et al., Reference Fjellstrom, Conaway-Bormans, Marchetti, Shank and Park2004). Landrace rice varieties are an important genetic resource that provides desirable resistant genes for both biotic and abiotic stresses. They adapt well to the local environment and have a great genetic potential for rice improvement. The screening for rice blast resistant genes using specific DNA markers can be helpful in identifying desirable genetic resources for blast resistance in rice breeding programmes. We genotyped rice blast resistant genes in Thai landrace rice accessions using gene-specific markers for the blast resistant genes Pid3, Pi54 and Pigm. Our results showed that Thai landrace rice germplasm contains several blast resistant genes which could serve as a good source for blast resistance breeding programmes in the future.
Materials and methods
Plant materials
A total of 226 rice accessions, including 19 accessions of upland rice from the North, 99 accessions of lowland rice from the Northeast, 45 accessions of floating rice from the Northeast and 40 accessions from the South, 21 recommended rice and two susceptible checks ‘KDML105’ and ‘Nipponbare’ rice varieties were provided by the Ubon Ratchathani Rice Research Center (online Supplementary Table S1). Rice seeds were grown in a greenhouse at the Faculty of Agriculture, Ubon Ratchathani University for DNA extraction.
DNA preparation and PCR amplification
Genomic DNA was prepared from young leaves using the CTAB method (Lodhi et al., Reference Lodhi, Ye, Weeden and Reisch1994, with minor modification). Amplification of DNA fragments was carried out using specific primers of Pid3 (Shang et al., Reference Shang, Tao, Chen, Zou, Lei, Wang, Li, Zhao, Zhang, Lu, Xu, Cheng, Wan and Zhu2009), Pigm (Deng et al., Reference Deng, Zhu, Shen and He2006) and Pi54 (Ramkumar et al., Reference Ramkumar, Srinivasarao, Mohan, Sudarshan, Sivaranjani, Gopalakrishna, Neeraja, Balachandran, Sundaram, Prasad, Shobha Rani, Rama Prasad, Viraktamath and Adhav2011). The primer sequences are shown in Table 1. PCR amplification was performed in a 20 µl reaction mix containing 100 ng template DNA, 5 picomoles of each primer, 0.1 mm dNTPs, 10 × PCR buffer and 1 U of I-Tag™ DNA polymerase (Intron). The temperature profile for PCR amplification was 1 cycle of 94°C for 5 min, followed by 35 cycles of 94°C for 45 s, 55–60°C for 45 s and 72°C for 60 s, and a final extension at 72°C for 5 min. The PCR products were separated by 1.0% agarose gel electrophoresis in 0.5 × TBE (Tris-borate-EDTA) buffer, the size of an amplified fragment was estimated by 1 kb Gene Ruler™ Express DNA ladder (Fermentas, Inc., Waltham, USA) stained with ethidium bromide, visualized and photographed using an infinity 3000 gel photographic system (Vilber Lourmat, Germany). Genotypic screening was done at least three times for each of the gene-specific primers.
Table 1. Gene-specific primer sequences of Pid3, Pigm and Pi54 resistant genes

For the Pid3-dCAPS1 marker, the PCR product was digested with XbaI restriction enzyme. The reaction was carried out in 15 µl, consisting of 1.5 µl of 10 × buffer, restriction endonuclease XbaI 1 unit and 10 µl PCR product, at 37°C for 2 h. The digested PCR fragments were subjected to 3% agarose gel electrophoresis in 0.5 × TBE buffer, stained with ethidium bromide, visualized and photographed.
PCR product sequence confirmation
To confirm the sequences of blast resistant genes from gene-specific primers, PCR products were purified with a Qiaquick gel extraction kit (QAIGEN, Germany) and sequenced at Macrogen, Korea using primers from each allele type, including resistant and susceptible alleles.
Inoculation and evaluation of blast disease reaction
For blast inoculation, 3-week-old seedlings of 10 Thai landrace rice accessions which were identified as conferring two or three blast resistant alleles were investigated – namely: Ngo Phuean, Pue Kaset, Niao Saeng Mu Woe, Lueang Hom, Cha No Nai, Lai San, Huai Laeng, Pi I Su Mae Fang, Khao Ko Phae and Ngo Se Ra. In addition, IR64 (recommended rice cultivar from IRRI and KDML105, both susceptible varieties, were inoculated with a mixture of 20 blast isolates collected in the North and Northeast of Thailand (10 isolates in 2005 and 10 isolates in 2010)) (online Supplementary Table S2). Each isolate was cultured on rice flour agar medium (2% rice flour, 0.2% yeast extract and 2.0% agar) and incubated at 25°C under 12 h per day of fluorescent light condition for 8–10 d. Fungal cultures were scraped to induce the sporulation and conidia were harvested 2 d later using sterile distilled water. Conidia suspensions of each blast isolate were adjusted to a concentration of 5 × 104 conidia/ml using sterile distilled water with 0.5% gelatin before making a conidia suspension mixture. Twenty-five millilitres of conidia suspension was spayed to 21-d one seeding of each rice variety. The inoculated plants were placed into sealed containers to maintain humidity and then grown in the dark for 24 h. These plants were then transferred to a greenhouse and disease reaction was examined 6–7 d after inoculation following according to Sirithunya et al. (Reference Sirithunya, Sreewongchai, Sriprakhon, Toojinda, Pimpisithavorn, Kosawang and Smitamana2008).
Results
Examination of Pid3 blast resistant gene using the Pid3-dCAPS-1 DNA marker: A total of 226 rice accessions including 203 Thai landrace rice accessions, 21 recommended rice cultivars and two check varieties were examined for the presence of the Pid3 blast resistant allele using the Pid3-dCAPS-1 DNA marker, a Pid3 gene-specific marker. Two hundred and two rice accessions (approximately 89% of the total) carried the Pid3 resistant allele, generating a 178 bp amplification product, which cannot be digested by XbaI restriction enzyme included nine Northern landrace rice varieties, 94 Northeast lowland rice varieties, 43 Northeast floating rice varieties, 15 recommendation rice varieties and 40 South landrace rice varieties (online Supplementary Table S1). The other 24 rice accessions and ‘Nipponbare’ contained the Pid3 susceptible allele, showing a 153 bp DNA product after digestion with XbaI enzyme (Fig. 1).

Fig. 1. Detection of DNA-specific markers for three rice blast resistant genes; Pid3(a), Pigm (b) and Pi54 (c). (R, resistant allele; S, susceptible allele; X, new allele; Uncut, uncut with enzyme; M, molecular DNA ladder; KDML, rice variety KDML105; NPB, rice variety Nipponbare).
To validate the presence of the resistant and susceptible alleles of Pid3, 10 random samples before digestion with the XbaI restriction enzyme, which consisted of five varieties with the susceptible allele ‘Pue Kaset’, ‘Lueang Hom’, ‘Pi I Mae La’, ‘Da Ya li’ and ‘Nipponbare’ and five varieties with the resistant allele ‘Ngo Phuean’, ‘KDML109-1-119’, ‘E Pit’, ‘Pa Ya chom’ and ‘Khiao’ were sequenced and compared with the Pid3 sequence from NCBI (online Supplementary Fig. S1). The results of a sequence alignment showed that samples with the resistant allele, 178 bp, have no XbaI enzyme recognition site. However, samples with the susceptible allele, containing an XbaI enzyme recognition size, resulted in shortened PCR products (from 178 to 153 bp). ‘KDML105’ was shown to have the resistant allele but ‘Nipponbare’ had the susceptible allele.
Examination of the Pigm blast resistance genes using the S29742 InDel marker
A Pigm gene-specific InDel marker was used to screen for resistant and susceptible alleles of Pigm in Thai landrace rice accessions, recommended rice varieties and in the check varieties (online Supplementary Table S1). From a total of 226 samples, 160 samples (about 70%) contained resistant allele with 555 bp PCR products, 46 samples (about 20%) contained the susceptible allele with 461 bp PCR products, and 17 samples (about 10%) contained a novel allele with 900 bp PCR products (Fig. 1). The 160 rice samples with the resistant allele included 15 landrace rice accessions from the North, 33 landrace rice accessions from the South, 66 landrace rice accessions from the Northeast, 37 floating landrace rice accessions from the Northeast and nine recommended rice varieties. Both of the susceptible check varieties, ‘KDML105’ and ‘Nipponbare’, showed the susceptible allele. Seventeen landrace rice accessions exhibited a novel allele at 900 bp including five accessions from South and 11 accessions from the Northeast (Table 2).
Table 2. The distribution of alleles for the rice blast resistant genes; Pid3, Pigm and Pi54 genes in 203 Thai landrace rice varieties and in 23 recommended rice varieties

a Three rice varieties cannot give amplification with Pigm(t) gene-specific primers
To validate the allele sequence of the Pigm(t) gene, 12 random PCR products were selected for sequencing from five rice varieties ‘Chiang Mai’, ‘Luk Dang’, ‘Beb Nok Bao’, ‘Cho’ and ‘Cho Dang’ with the resistant allele, five accessions ‘Pi I Su Mae Fang’, ‘I Pong’, ‘Namh Nuan’, ‘Do Sam Duean’ and ‘Nipponbare’ with the susceptible allele and two accessions ‘Loot Nee’ and ‘Med Yai’ with the novel allele. The results from sequence alignment confirmed that the resistant allele (555 bp) was 94 bp longer than the susceptible allele (461 bp) and that the novel allele (900 bp) was much longer than both the resistant and susceptible alleles (online Supplementary Fig. S2).
Examination of the Pi54 blast resistance genes using the Pi54 MAS InDel marker
Genotypic screening of the Pi54 resistant gene using the gene-specific marker, Pi54 MAS showed that only 16 rice varieties contained the Pi54 resistant allele with a PCR product size of 359 bp. The 16 rice varieties included 10 landrace rice accessions from the North and 6 recommended rice varieties. The susceptible allele showed a PCR product size of 359 bp which included both ‘Nipponbare’ and ‘KDML105’, the susceptible check varieties (online Supplementary Table S1). No landrace rice accessions from the Northeast or from the South contained resistance allele.
To validate the resistance and susceptible alleles of the Pi54 gene, five landrace rice accessions with resistant allele – namely: ‘Ngo Phuean’, ‘Pue Kaset’, ‘Lai San’, ‘Huai Laeng’ and ‘Khao Ko Phae’, and four landrace rice accessions and ‘Nipponbare’ with susceptible allele – namely: ‘Khuen Din’, ‘Bao Chamuang’, ‘Luk Dang’ and ‘Kao Phuang 74-3-38’ were sequenced and analysed (online Supplementary Fig. S3). The sequence alignment confirmed that the resistant allele (216 bp) was 143 bp shorter than susceptible allele (359 bp).
Evaluation of the blast disease reaction
In order to determine the disease reaction of 12 rice accessions, each containing at least two resistant genes (Pid3, Pigm and Pi54), 20 rice blast isolates were used to inoculate 21-d-old rice seedlings. These 12 rice accessions consisted of 10 landrace rice accessions from the North, one recommended rice variety ‘IR64’ and a susceptible variety ‘KDML105’. Disease reaction was scored as disease severity rating (0–6) a 7 d after inoculation. A disease severity level from 0 to 2 was considered as resistance, a severity level from 3 to 4 was considered as moderate resistance, and a severity level from 5 to 6 was considered as being no resistance to the disease, i.e. susceptible (Roumen et al., Reference Roumen, Levy and Notteghem1997). The results showed that seven landrace rice accessions were resistant to the mixture of rice blast isolates that were used for inoculation and that ‘KDML105’ showed a high level of susceptibility (Table 3). However, two landrace rice accessions ‘Cha No Nai’ and ‘Lai San’ were susceptible to the rice blast inoculation despite of ‘Cha No Nai’ containing the resistant allele from the Pid3 and Pi54 genes and ‘Lai San’ containing the resistant allele from all Pid3, Pigm and Pi54 genes.
Table 3. Disease reaction of selected landrace rice varieties and recommended rice varieties inoculated with a mixture of 20 rice blast fungal isolates
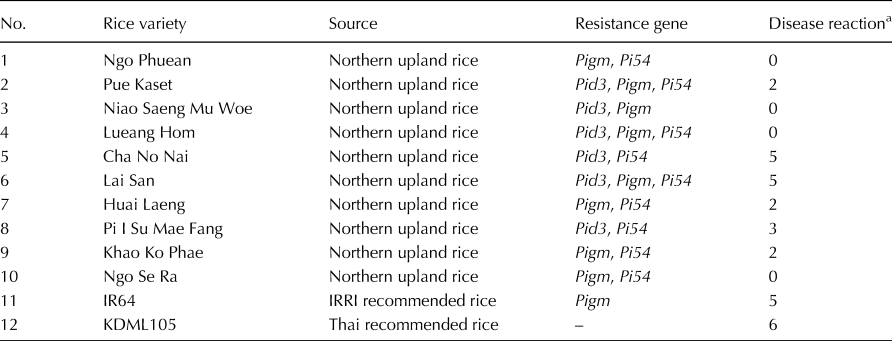
a Disease reaction was scored as disease severity rating (0–6) a 7 d after inoculation. A disease severity level from 0 to 2 was considered as resistance, a severity level from 3 to 4 was considered as moderate resistance, and a severity level from 5 to 6 was considered as being no resistance to the disease, i.e. susceptible (Roumen et al., Reference Roumen, Levy and Notteghem1997).
Discussion
We demonstrate that gene-specific DNA markers can be successfully used to identify resistant allele of rice blast resistant genes. Thai landrace rice germplasm contains valuable gene pool for rice blast resistance breeding programme. Each rice blast resistant genes, Pid3, Pigm and Pi54, distribute differently in Thai landrace rice germplasm. The Pid3 gene was found in more than 80% of Thailand rice germplasm including rice varieties from North and Northeast and South. Our result was consistent with the previous study by Shang et al. (Reference Shang, Tao, Chen, Zou, Lei, Wang, Li, Zhao, Zhang, Lu, Xu, Cheng, Wan and Zhu2009) that most rice varieties contained the Pid3 resistant allele. Similar to the rice blast resistant gene, Pigm can also be found in rice varieties from all regions of Thailand. On the other hand, rice blast resistant gene, Pi54, was found only in rice varieties in the North of Thailand. In general, co-evolution between rice and the rice blast fungus occurs and the virulent fungal strains will force the rice resistant allele to evolve. This system displays a very high level of specificity between the rice and fungus (Lee and Cho, Reference Lee and Cho1990). Sirithunya et al. (Reference Sirithunya, Sreewongchai, Sriprakhon, Toojinda, Pimpisithavorn, Kosawang and Smitamana2008) reported that rice blast isolates in the North and Northeast of Thailand displayed high genetic diversity. This high level of diversity may be the cause of the development of resistant alleles in landrace rice in the North and Northeast of Thailand. In comparison, landrace rice varieties from the South have less demand for coevolution due to the lower diversity of fungi in that region.
CAPs and InDel markers, used to identify rice blast resistant genes in this study, have different mechanisms of co-evolution between resistant and susceptible alleles. CAPs marker for Pid3 gene detects a point mutation causing an amino acid change in resistant protein leading to different disease reaction. Restriction enzyme, BamHI, was used to cut the PCR product to reveal the different size of resistant and susceptible alleles. InDel marker for Pigm and Pi54 detect the PCR produces with different size between resistant and susceptible alleles. The size difference between these alleles may be caused by a deletion or insertion (InDel mutation) of nucleotides in the Pigm gene. The InDel mutation will lead to different amino acid sequences and alter both the function and the ability of the resistant gene. In addition, the novel allele of Pigm is much longer than the original resistant and susceptible alleles. It is possible that the transposon element may move into the Pigm gene and lead to the novel allele. This novel allele is most likely to be the loss of function of the resistant allele as Sharma et al. (Reference Sharma, Madhav, Singh, Shanker, Jana, Dalal, Pandit, Singh, Gaik and Singh2005) previously reported that the size difference between the two alleles of the Pi54 gene was a result of the insertion of transposon element in the exon region, which leads to the loss of the resistance function.
Most rice varieties with two or three resistant alleles are originated from the North of Thailand. The pathogenicity test with a mixture of rice blast fungus isolated in Thailand suggested that resistant phenotype tended to increase when the number of resistant alleles in rice varieties increases. However few rice varieties, which contain several resistant alleles but showed susceptible disease reaction. This result may be explained that the rice blast isolates used in this screening were not recognized or specific to the blast resistant genes under investigation or the promoter that drive the expression of these genes are not function correctly.
In summary, our results showed that the screening of genotypes to assure data about resistant and susceptible alleles of rice blast resistant genes in landrace rice germplasm is extremely important. The information generated from this study will assist in rice blast resistance breeding programmes and in landrace rice germplasm management and conservation.
Supplementary material
The supplementary material for this article can be found at https://doi.org/10.1017/S1479262119000224.
Acknowledgements
We thank Ubon Ratchathani Rice Research Center for providing samples of landrace rice cultivars. This research was supported by funding from Ubon Ratchathani University, Kasetsart University Research and Development Institute (KURDI), Center of Excellence on Agricultural Biotechnology, Science and Technology Postgraduate Education and Research Development Office (PERDO), Commission on Higher Education, Ministry of Education and the National Research Council of Thailand (NRCT) in the 2019 fiscal year. Miss Wattanaporn Teerasan received financial support to conduct part of this study from the International Affairs Division, Kasetsart University.