Introduction
It is not uncommon that a host is infected with two or more species of pathogens, including viruses, bacteria and parasites. A globally distributed parasite, Toxoplasma gondii, is one of the most successful parasites and can infect and propagate in almost all nucleated cells found within warm-blooded animals including humans (Tenter et al., Reference Tenter, Heckeroth and Weiss2000; Hill et al., Reference Hill, Chirukandoth and Dubey2005). As a typical opportunistic pathogen, T. gondii usually establishes long-term latent infections, which are largely asymptomatic in immune-competent individuals. However, in fetus, newborns and immunocompromised patients, T. gondii infection will develop into potentially life-threatening toxoplasmosis (Montoya and Liesenfeld, Reference Montoya and Liesenfeld2004), which reflects the nature of toxoplasmosis that its outcome is largely influenced by host immune status.
Extensive studies have been carried out, using animal models to discover the progression of Toxoplasma infection and factors influencing the host–parasite relationship. A key driver that controls T. gondii infection in laboratory rodents is the balance of expression of the enzymes inducible nitric oxide synthase (iNOS) and Arginase-1 (Li et al., Reference Li, Zhao, Zhu, Ren, Nie, Gao, Gao, Yang, Zhou, Shen, Wang, Lu, Chen, Hide, Ayala and Lun2012; Zhao et al., Reference Zhao, Zhang, Wei, Li, Wang, Yi, Shen, Yang, Hide and Lun2013; Wang et al., Reference Wang, Gao, Yi, Geng, Gao, Shen, Lu, Wen, Hide and Lun2014; Gao et al., Reference Gao, Yi, Wu, Geng, Shen, Lu, Hide, Lai and Lun2015). High iNOS expression and low arginase expression is associated with resistance to T. gondii infection while the reverse expression levels are associated with susceptibility. However, there are significant differences between animal models in the laboratory and natural infections found in wild animals (Beura et al., Reference Beura, Hamilton, Bi, Schenkel, Odumade, Casey, Thompson, Fraser, Rosato, Filali-Mouhim, Sekaly, Jenkins, Vezys, Haining, Jameson and Masopust2016) including differences in environment and in immune status. Parasitism in wild animals is common and rodents in the wild are highly likely to be infected with multiple pathogens. Thus, understanding the immune responses in relation to co-infection of T. gondii with other pathogens has great potential to be both interesting and relevant in nature (Catarinella et al., Reference Catarinella Arrea, Chinchilla Carmona, Guerrero Bermúdez and Abrahams1998; Onah and Wakelin, Reference Onah and Wakelin1999).
It is widely documented that members of the genus Trypanosoma can induce severe depression of the host immune system (Sztein and Kierszenbaum, Reference Sztein and Kierszenbaum1992; Onah and Wakelin, Reference Onah and Wakelin1999). For example, Trypanosoma brucei induced suppression of responses to Trichinella spiralis in vaccinated NIH mice (Onah and Wakelin, Reference Onah and Wakelin1999). Chagas' disease at the acute stage by Trypanosoma cruzi infection is linked to immunosuppression in both patients and laboratory animals which facilitates the multiplication and dissemination of parasites in the mammalian host (Onah and Wakelin, Reference Onah and Wakelin1999). These raise questions as to whether and how other Trypanosoma species influences host immunity.
In the case of pathogens of rodents, Trypanosoma musculi and Trypanosoma lewisi are two globally distributed parasites of mice and rats, respectively. Trypanosoma lewisi can have prevalences of up to 56% rats (Liu and Liu, Reference Liu and Liu1990; Desquesnes et al., Reference Desquesnes, Ravel and Cuny2002; Dobigny et al., Reference Dobigny, Gauthier, Houéménou, Dossou, Badou, Etougbétché, Tatard and Truc2019) and has also been considered as pathogenic to humans (Lun et al., Reference Lun, Wen, Uzureau, Lecordier, Lai, Lan, Desquesnes, Geng, Yang, Zhou, Jannin, Simarro, Truc, Vincendeau and Pays2009; Reference Lun, Reid, Lai and Li2015; Truc et al., Reference Truc, Büscher, Cuny, Gonzatti, Jannin, Joshi, Juyal, Lun, Mattioli, Pays, Simarro, Teixeira, Touratier, Vincendeau and Desquesnes2013; Lin et al., Reference Lin, Lai, Zheng, Wu, Lukeš, Hide and Lun2015). Many previous studies have suggested that T. lewisi infection could induce an immunosuppressive effect on its hosts, resulting in a greater susceptibility to infection by other pathogens, including Salmonella typhimurium, Cryptococcus neoformans and Toxoplasma gondii (Nielsen et al., Reference Nielsen, Sheppard, Holmes and Tizard1978; Catarinella et al., Reference Catarinella Arrea, Chinchilla Carmona, Guerrero Bermúdez and Abrahams1998; Gross et al., Reference Gross, Guerrero, Chinchilla and Jarstrand-Hall2006; Carrera et al., Reference Carrera, Carmona, Guerrero and Castillo2009). Trypanosoma musculi has similar biological characteristics to T. lewisi but restricted to mice and can also induce an effect on experimental infection of mice with Toxoplasma (Albright and Albright, Reference Albright and Albright1980; Piccolo-Johanning et al., Reference Piccolo-Johanning, Kellerman-Guterman, Valerio-Campos and Chinchilla-Carmona2013).
Herein, our study aims to investigate the effect of trypanosome (T. lewisi and T. musculi) infection in rats and mice on infection with T. gondii, and to uncover the role of iNOS and NO in the trypanosome-induced immunoregulation.
Materials and methods
Animals
Brown Norway (BN), Fischer 344 (F344) and Lewis (LEW) rats were purchased from the Vital River Experimental Animal Centre, Beijing, China. Sprague-Dawley (SD) and Wistar (WST) rats and four strains of mice (Swiss Webster, BALB/c, NIH and C57BL/6) were purchased from the Experimental Animal Centre, Guangdong Province, China. All rats used in this study weighed 180–220 g and mice weighed 20–25 g. Animals were kept in a specific pathogen-free environment with free access to food and water. The protocols were approved by the Institutional Review Board of Animal Care at Sun Yat-Sen University under license of 3167227.
Parasites
Tachyzoites of T. gondii RH-GFP strain (Nishikawa et al., Reference Nishikawa, Xuenan, Makala, Vielemeyer, Joiner and Nagasawa2003) and cysts of T. gondii Prugniaud strain were obtained as below. Swiss Webster mice were intraperitoneally inoculated with 1 × 105 tachyzoites of RH-GFP. Peritoneal cavities of mice were harvested by injection with ice-cold D-Hanks 3 days post infection (dpi). Viable tachyzoites were harvested with differential centrifugation (50 × g 8 min at 4°C and 1500 × g for 10 min at 4°C) and resuspended in RPMI 1640 medium with 10% FBS. Swiss Webster mice were orally infected with T. gondii Prugniaud strain and tissue cysts were obtained from brains as previously described (Brinkmann et al., Reference Brinkmann, Remington and Sharma1987; Letscher-Bru et al., Reference Letscher-Bru, Pfaff, Abou-Bacar, Filisetti, Antoni, Villard, Klein and Candolfi2003). Briefly, mice were anaesthetized by CO2 at 60 dpi and the brain was removed and homogenized in 1 mL PBS (pH 7.2). Cysts within 10 μL samples were carefully quantified by microscopy using a coverslip (22 × 22 mm) at 100 × magnification. Cyst in infected rats was determined similarity after homogenized in 2 mL PBS.
Two kinds of haemoflagellates, including T. lewisi (strain CPO02, isolated from wild rats, Rattus norvegicus, in Guangzhou, China) and T. musculi (provided by Dr Philippe Vincendeau from the Université de Bordeaux II), were used in this study (Vincendeau et al., Reference Vincendeau, Caristan and Pautrizel1981; Tang et al., Reference Tang, Lan, Wen, Zhang, Desquesnes, Yang, Hide and Lun2012). SD rats were intraperitoneally inoculated with 1 × 106 trypomastigotes of T. lewisi, while Swiss Webster mice were intraperitoneally inoculated with 1 × 105 trypomastigotes of T. musculi. Parasitemia in infected animals was monitored daily. Usually, at 7–9 dpi, animals were killed by CO2 treatment and the blood was drawn from the heart and diluted to designed concentration with PBS.
Methodology of animal experiments
In vivo experiments were conducted with four groups of rats (6 each) and five groups of mice (10–13 each). In rats, ‘Tl’ group was inoculated with 1 × 106 T. lewisi trypomastigotes, while ‘Tg’ group was inoculated with 200 cysts of the T. gondii Pru strain. ‘Tl,Tg’ and ‘Tg,Tl’ groups were firstly inoculated with former and then 4–6 days later inoculated with latter, respectively. In mice, ‘Tm’ group was inoculated with 1 × 105 T. musculi trypomastigotes, ‘Tg’ group was inoculated with 5 cysts of the T. gondii Pru strain. ‘Tm,Tg’ and ‘Tg,Tm’ groups were separately inoculated with indicated parasites with the interval of 5 days, while ‘Tg + Tm’ group was inoculated on both parasites on the same day. Infected animals were monitored for 50 (rats) or 30 (mice) days until sacrificed.
In vitro experiments were carried out with pre-infected five SD rats (1 × 106 T. lewisi trypomastigotes) and six Swiss Webster mice (1 × 105 T. musculi), Rat and mouse peritoneal macrophages were isolated at 6 dpi and cultured in RPMI-1640 medium containing 10% fetal bovine serum (FBS) at 37°C with 5% CO2. Another five SD rats and six Swiss Webster mice were intraperitoneally inoculated with a saline solution as a control.
Peritoneal macrophage isolation and cultivation
The method for isolation of macrophages, described by El-Mahmoudy et al. (Reference El-Mahmoudy, Matsuyama, Borgan, Shimizu, El-Sayed, Minamoto and Takewaki2002) was used in this study. Animals, sacrificed by CO2 treatment, were injected intraperitoneally with 5 mL (mouse) or 15 mL (rat) ice-cold D-Hank's solution and then peritoneal cells were harvested and separated by centrifugation at 250 × g for 10 min at 4°C. The cells were then washed by D-Hank's solution and suspended in RPMI-1640 medium containing 10% FBS and penicillin (100 U mL−1) and then left to adhere for 2 h at 37°C in an incubator with 5% CO2. Non-adherent cells were removed after 4 hours.
Determination of T. gondii intracellular multiplication
Rat or mouse macrophages were challenged with T. gondii RH strain tachyzoites at a multiplicity of infection (moi) 1:1. Extracellular T. gondii were then washed out after 1 h incubation together, at which the time point was defined as 1 h for the start of the experiment. Thereafter, the cells were observed with an inverted fluorescence microscope at the desired time. The numbers of T. gondii were counted in 100 host macrophages and an average determined.
Measurement of iNOS and arginase activity
Nitrite content as a reflection of NO production was determined by the Griess reaction as described (Ding et al., Reference Ding, Nathan and Stuehr1988). Briefly, 100 μL supernatant or standard solution (NaNO2) was incubated in triplicate with 100 μL of Griess reagent (0.5% sulfanilamide, 0.05% naphthyldiaminedihydrochloride in 5% H3PO4) for 10 min. The plates were read at 550 nm in an ELISA reader (Multiskan MK3, Thermo Labsystems, Finland).
Arginase activity of purified macrophages was measured by a colorimetric method as described by Corraliza et al. (Reference Corraliza, Campo, Soler and Modolell1994). Briefly, 10 mm MnCl2 and 0.5 M L-arginine were successively added to macrophage lysates for 1 h at 37°C. The reaction was stopped by addition of an acid solution (H2SO4: H3PO4: H2O = 1:3:7) and the urea generated by arginase was analyzed by addition of a-isonitrosopropiophenone at 100°C for 45 min. The coloured product was quantified by absorption at 550 nm in an ELISA reader. Arginase activity was determined as the amount of urea produced from the total protein of peritoneal macrophages.
Measurement of gene expression by mRNA analysis
Total RNA from treated and non-treated macrophages was extracted using Trizol Reagent (Invitrogen, Carlsbad, USA) according to the manufacturer's instructions. Total RNA was converted to cDNA using a set of oligo (dT) primer and SuperScript TM III First-Strand Synthesis System (Invitrogen, Carlsbad, USA). cDNA (1 μg) was used as a template for amplifying iNOS, arginase-1 and GAPDH (internal standard) genes by polymerase chain reaction (PCR) using the following primers: arginase-1, 5′-AAG AAA AGG CCG ATT CAC CT-3′ and 5′-CAC CTC CTC TGC TGT CTT CC-3′, 201 bp; rat-iNOS, 5′-CTA CCT ACC TGG GGA ACA CCT GGG-3′ and 5′ -GGA GGA GCT GAT GGA GTA GTA GCG G-3′, 442 bp; mouse-iNOS, 5′-GCC TCG CTC TGG AAA GA-3′ and 5′-TCC ATG CAG ACA ACC TT-3′, 499 bp; GADPH, 5′ -AAT GCK TCC TGY ACC ACC AAC TGC-3′ and 5′ -TTA GCC AWA TTC RTT GTC RTA CCA GG-3′, 513 bp. For semiquantitative PCR, the cycling protocol was: 94°C for 1 min, 60°C for 1.5 min and 72°C for 1.8 min. For arginase-1, rat-iNOS, mouse-iNOS, 27 cycles were used, but for GAPDH, only 20 cycles were used. Amplified DNA products were separated on 1% agarose gel and photographed using an electronic documentation system (Biostep, Germany) after staining with ethidium bromide.
Measurement of gene expression using western Blotting
Cells were lysed in SDS loading buffer, fractionated in SDS-PAGE and transferred onto immunoblot polyvinylidene difluoride membrane (Pall, USA). The membrane was probed using rabbit polyclonal iNOS antibody (Thermo, USA) and rabbit polyclonal arginase-1 antibody (Santa Cruz, USA). β-tubulin was stained with antibody (NOVUS, USA) as a control. Horseradish peroxidase-labelled secondary antibodies (Cell Signaling, USA) and DAB (3,3′,5,5′-tetramethylbenzidine) Detection Kit (Tiangen, China) were used for antibody detection. Relative expression of iNOS and Arginase was calculated by normalizing with the β-tubulin control.
Statistical analysis
Cyst counts from each group are given as a mean ± standard error of mean (s.e.m.), and presented using GraphPad Prism version 5. Other results are expressed as mean ± standard deviation (s.d.). Odds ratios were calculated with 0.5 added to counts to avoid dividing with zero (Glas et al., Reference Glas, Lijmer, Prins, Bonsel and Bossuyt2003) and performed two-tailed χ 2-test with Yates's correction. The student´s t-test was used to compare differences between two unpaired samples and statistical differences were designated by */# (P<0.05), **/## (0.001< P < 0.01) and ***/### (P < 0.001).
Results
Survival in animals co-infected with T. gondii and T. musculi/T. lewisi is associated with splenomegaly
As shown in Supplementary Table 1, rats are highly resistant to T. gondii infection, as all showed zero mortality. However, infection of T. lewisi may kill rats of SD, BN, LEW and WST strains, except F344. In generally, additional T. gondii infection may not change the mortality caused by T. lewisi, only Tl,Tg group of BN rats showed significant high mortality. Considering the parasitemia, T. lewisi could survive in the blood for more than 40 days in four strains of rats (BN, F344, SD, WST) (Supplementary Fig. 1), the LEW strain rats were so resistant to T. lewisi that no parasitemia was observed. In WST groups, all rats developed similar levels of parasitemia. In F344 and SD groups, lower parasitemia peaks of T. lewisi were found in those that were co-infected with T. gondii than in singly infection groups (Tl vs Tl,Tg/Tg,Tl: F344, P = 0.0079/0.0005; SD, P < 0.0001/0.0001), while BN missed many data due to animal deaths. Spleen indexes [spleen weight (mg) per body weight (g)] in rats on 60 dpi (Table 1) showed T. lewisi infection resulted significant splenomegaly, which T. gondii resulted splenomegaly F344 rats but reverse effect in other rats. In the case of co-infection, all rats had higher spleen indexes than the T. gondii groups.
Table 1. Summary of the spleen index of rats infected with T. gondii and/or T. lewisi

Spleen index = spleen weight (mg)/body weight (g). n = 6. */***, vs Tg group, P < 0.05 or P < 0.001, respectively; #/##/###, vs control group, P < 0.05, P < 0.01 or P < 0.001, respectively.
As shown in Table 2 and Supplementary Fig. 2, T. musculi is nonlethal to mice with 100% survival. However, mice are highly susceptible to T. gondii infection (even with the avirulent Pru strain) and showed various levels of mortality, as C57BL/6, the most susceptible mice, survived only 15% (2/13) and BALB/c, the most resistant mice, all alive. In co-infected groups, there was generally a decreased survivability compared to both singly infected controls, but only significant for the ‘Tm,Tg’ group of BALB/C mice (P = 0.025).
Table 2. Summary of survivability of mice infected with T. gondii and/or T. musculi

The columns refer to the following: Tm (infected singly with T. musculi); Tg (infected singly with T. gondii); Tm,Tg or Tg,Tm (infection with T. musculi first followed by infection with T. gondii 5 days later or vice-versa); Tm + Tg (both parasites infected simultaneously). The term ‘ref’ refers to the reference for the odds ratio.
a, P = 0.013; b, P = 0.050; c, P = 0.054; d, P = 0.057.
During the infection of T. musculi (30 days) only the parasitemia found in ‘Tm,Tg’ group of BALB/C mice was significantly higher than the ‘Tm’ group at their peaks between days 9 and 12 (P = 0.0418), while other groups were not (Supplementary Fig. 2). Importantly, most C57BL/6 mice died before showing high parasitemia, indicating the death may be due to immune-storm of co-infection.
Spleen indexes in surviving mice on 60 dpi (Table 3) showed either T. gondii or T. musculi infection resulted significant splenomegaly, whereas co-infection generally enhanced the splenomegaly, compared with the T. gondii groups. Data for C57BL/6 mice were limited as few survived.
Table 3. Summary of the spleen index of mice infected with T. gondii and/or T. musculi

Spleen index = spleen weight (mg)/body weight (g). n = 6. */***, vs Tg group, P < 0.05 or P < 0.001, respectively; ##/###, vs control group, P < 0.01 or P < 0.001, respectively.
Toxoplasma gondii cyst burden in the brains of co-infected animals
We investigated the variance in susceptibility to T. gondii in mice and rats when co-infected with T. musculi (mice) and T. lewisi (rats). A surprising result was that mice of Swiss Webster and NIH improved their resistance, to a greater or lesser degree, to the T. gondii PRU strain when co-infected with T. musculi (Fig. 1). Heavy cyst burdens were detected in the brains of mice infected with T. gondii only (Mean ± s.e.m. of group ‘Tg’: BALB/c, 355 ± 71.81, n = 13; NIH, 3629 ± 626.9, n = 10; Swiss Webster, 23 198 ± 3472, n = 3), while lower numbers of brain cysts were detected in the brains of T. musculi pre-infected groups (Mean ± s.e.m. of group ‘Tm, Tg’: BALB/c, 206 ± 96.36, n = 6; NIH, 1353 ± 508.4, n = 8; Swiss Webster, 124.3 ± 15.67, n = 3) with the difference being significant in the NIH (P = 0.015) and Swiss Webster strains (P < 0.001) (Fig. 1). However, when T. musculi was coinfected with T. gondii at the same time (Tm + Tg) or subsequently (Tg, Tm) there was little influence on cyst burden, in the mice strains except in the case of Swiss Webster where a significant drop in cyst number occurred when T. gondii infected mice were post-infected with T. musculi. Cyst burden in C57BL/6 mice could not be determined due to high lethality (more than 85%) after infection with T. gondii.

Fig. 1. Differences in Toxoplasma cyst numbers in the brains of four strains of mice. Each strain of mice was divided into four groups, which were coinfected with 5 cysts from the T. gondii Pru strain and 105 trypomastigotes of T. musculi or singly infected with T. gondii. Tg (infected singly with T. gondii); Tm,Tg or Tg,Tm (infection with T. musculi first followed by infection with T. gondii 5 days later or vice-versa); Tm + Tg (both parasites infected simultaneously). The cyst numbers in the brains of all inoculated mice were detected 60 days later. The mean and standard error of the mean of each group was indicated. Significant differences are indicated */***, vs Tg group, P < 0.05 or P < 0.001, respectively; #/##, vs Tg,Tm group, P < 0.05 or P < 0.01, respectively.
Interestingly, in contrast to the mice, we found (Fig. 2) that rat strains F344 and BN pre-infected with T. lewisi showed greater susceptibility to T. gondii than the T. gondii singly infected rats (Mean ± s.e.m. of group ‘Tl, Tg’: F344, 2103 ± 234.6, n = 5; BN, 936.0 ± 259.5, n = 5; SD, 90.0 ± 60.0, n = 5; vs group ‘Tg’: F344, 216.0 ± 47.6, n = 5, P < 0.001; BN, 0 ± 0, n = 5, P < 0.01; SD, 0 ± 0, n = 5, P = 0.17). However, there was no significant difference between the other coinfected groups (Tg, Tl i.e. post-infection with T. lewisi) and the T. gondii infected groups (Tg). In the case of the remaining rat strains, WST and LEW, these are very resistant to T. gondii infection and no Toxoplasma cysts were found at all (by microscopic examination and T. gondii-PCR) even when coinfected with T. lewisi (data not shown).

Fig. 2. Differences in Toxoplasma cysts numbers in the brains of five strains of rats. Each strain of rats was divided into three groups, which were coinfected with 200 cysts of T. gondii Pru strain and 106 trypomstigotes of T. lewisi or only infected with T. gondii. Tg (infected singly with T. gondii); TL,Tg or Tg,TL (infection with T. lewisi first followed by infection with T. gondii 5 days later or vice-versa). The cyst numbers in the brains of all inoculated rats were detected 60 days later. The mean and standard error of the mean of each group was indicated. Significant differences are indicated **, P < 0.01; ***, P < 0.001.
Variation in NO/urea production and iNOS/arginase expression in the peritoneal macrophages of mice or rats is related to the proliferation status of T. gondii
It has been previously demonstrated that there is competition for the substrate arginine between the enzymes iNOS and arginase (Li et al., Reference Li, Zhao, Zhu, Ren, Nie, Gao, Gao, Yang, Zhou, Shen, Wang, Lu, Chen, Hide, Ayala and Lun2012). Therefore, we analyzed the level of NO/urea production and iNOS/arginase expression in peritoneal macrophages isolated from Sprague Dawley rats (SD) and Swiss Webster mice which were pre-infected with T. lewisi and T. musculi, respectively. Increased amounts of NO production was found in primary cultured peritoneal macrophages taken from mice pre-infected with T. musculi (25.82 ± 1.88 μ m from T. musculi pre-infected mice after culture for 24 h compared with 4.44 ± 2.80 μ m from control mice at 24 h, P = 0.0004) (Fig. 3A). In addition, a higher level of iNOS mRNA expression (reference gene, GADPH) was observed in peritoneal macrophages from mice pre-infected with T. musculi whereas iNOS mRNA expression could not be detected in control mouse peritoneal macrophages (Fig. 3C). Western blot analysis (reference protein, β-actin) demonstrated a slightly higher expression of iNOS protein in T. musculi pre-infected mouse than normal mouse peritoneal macrophages. Measurement of arginase mRNA expression and urea production, in primary cultured peritoneal macrophages from mice, showed higher levels in the control group (0.34 ± 0.04 mm from control mice after 24 h culture and 0.23 ± 0.05 mm from T. musculi pre-infected mice at 24 h, P = 0.043) (Fig. 3B). However, there is no distinct difference in arginase expression between control and T. musculi pre-infected mice by western blot analysis (Fig. 3D). This suggests that the drop in urea production in the pretreated mice was probably due to competition, by increased iNOS expression. for the arginase/iNOS substrate arginine.

Fig. 3. Inducible nitric oxide synthase (iNOS)/arginase expression and nitric oxide (NO)/urea production in peritoneal macrophages from Swiss Webster mice. A. Comparison of NO production, measured by the Griess reaction, in macrophages of normal and T. musculi pre-infected Swiss Webster mice. B. Comparison of urea production in macrophages of normal and T. musculi pre-infected Swiss Webster mice. Error bars indicate standard deviations of measured values (n = 3). Data were analyzed using the unpaired Student's t-test (two-tailed). * P < 0.05; ** P < 0.01; *** P < 0.001 C. RT-PCR analysis of the expression of iNOS and arginase-1 mRNA. D. Western blotting analysis for expression of the iNOS and arginase-1 proteins.
Unlike the effect of T. musculi on the mouse, NO production was significantly lower in T. lewisi pre-infected rats (15.26 ± 2.03 μ m) than control rats (35.34 ± 3.34 μ m, P < 0.0001) and after only 12 h of culture this difference already existed (P < 0.0001) (Fig. 4A). In addition, it can be seen that the expression of iNOS mRNA (reference gene, GADPH) and protein (reference protein, β-actin) were clearly decreased in SD rats after T. lewisi infection (Fig. 4C and D). However, the urea production and mRNA/protein expression of arginase were significantly higher in T. lewisi pre-infected rats (Fig. 4B–D).
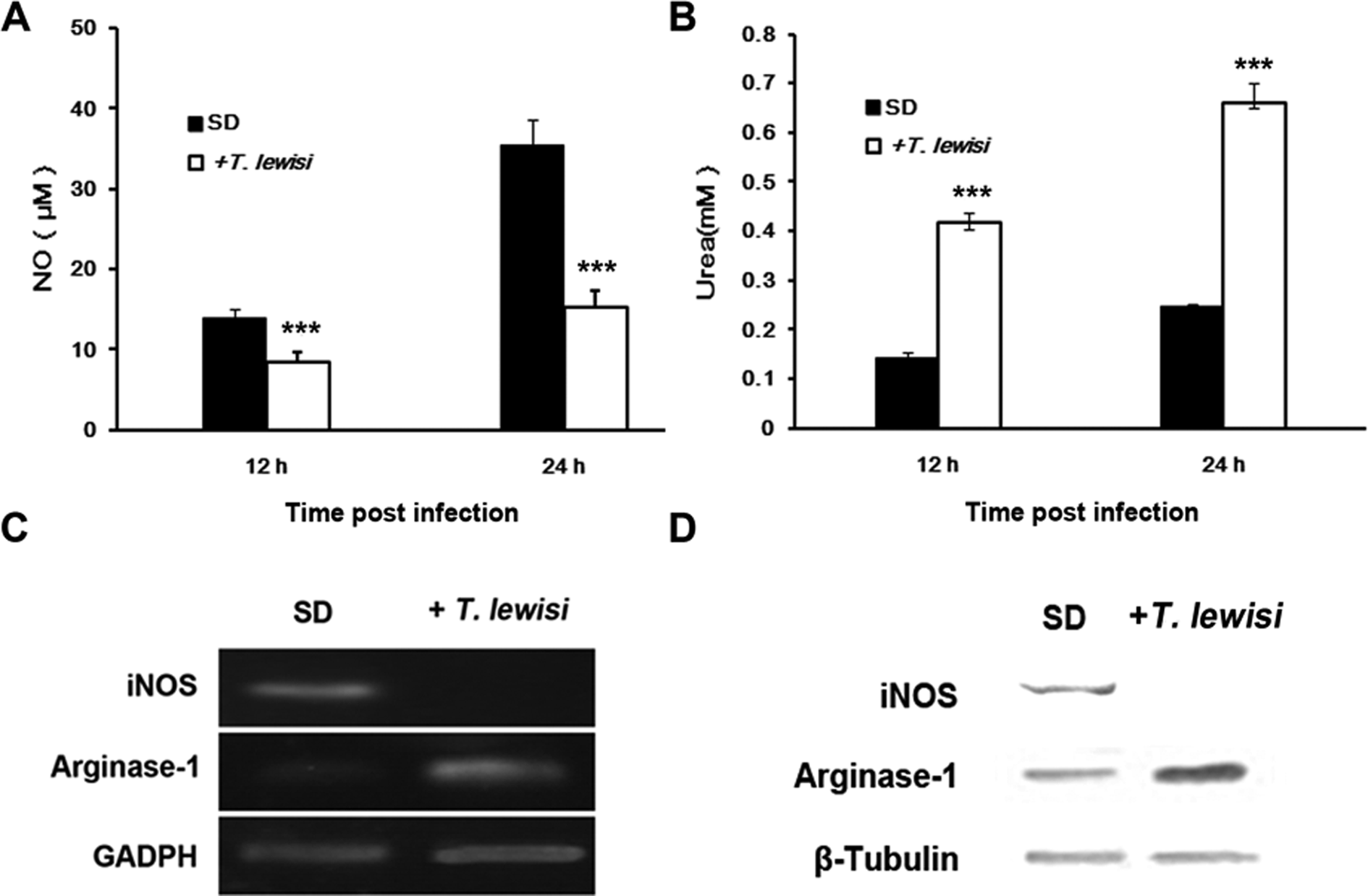
Fig. 4. Inducible nitric oxide synthase (iNOS)/arginase expression and nitric oxide (NO)/urea production in peritoneal macrophages from Sprague-Dawley rats. A. Comparison of NO production, measured by the Griess reaction, in macrophages from normal and T. lewisi infected SD rats. B. Comparison of urea production in macrophages of normal and T. lewisi infected SD rats. Error bars indicate standard deviations of measured values (n = 3). Data were analysed using the unpaired Student's t-test (two-tailed). * P < 0.05; ** P < 0.01; *** P < 0.001 C. RT-PCR analysis for the expression of the iNOS and arginase-1 mRNA. D. Western blotting analysis for expression of the iNOS and arginase-1 proteins.
It is known that NO is an important effector molecule involved in the resistance of rat/mouse peritoneal macrophages to T. gondii infection. In addition, it has been confirmed that a lower level of NO production was detected in the peritoneal macrophages of T. lewisi infected SD rats. Therefore, we expected that there would be a proliferation of T. gondii in macrophages from rats pre-infected with T. lewisi. Indeed, the proliferation of T. gondii tachyzoites (RH strain) dramatically increased in peritoneal macrophages from T. lewisi pre-infected SD rats (Fig. 5) and this was associated with the observed lowered NO production. The reproductive rate of T. gondii tachyzoites is clearly inhibited in the peritoneal macrophages from the T. lewisi uninfected control SD rats.
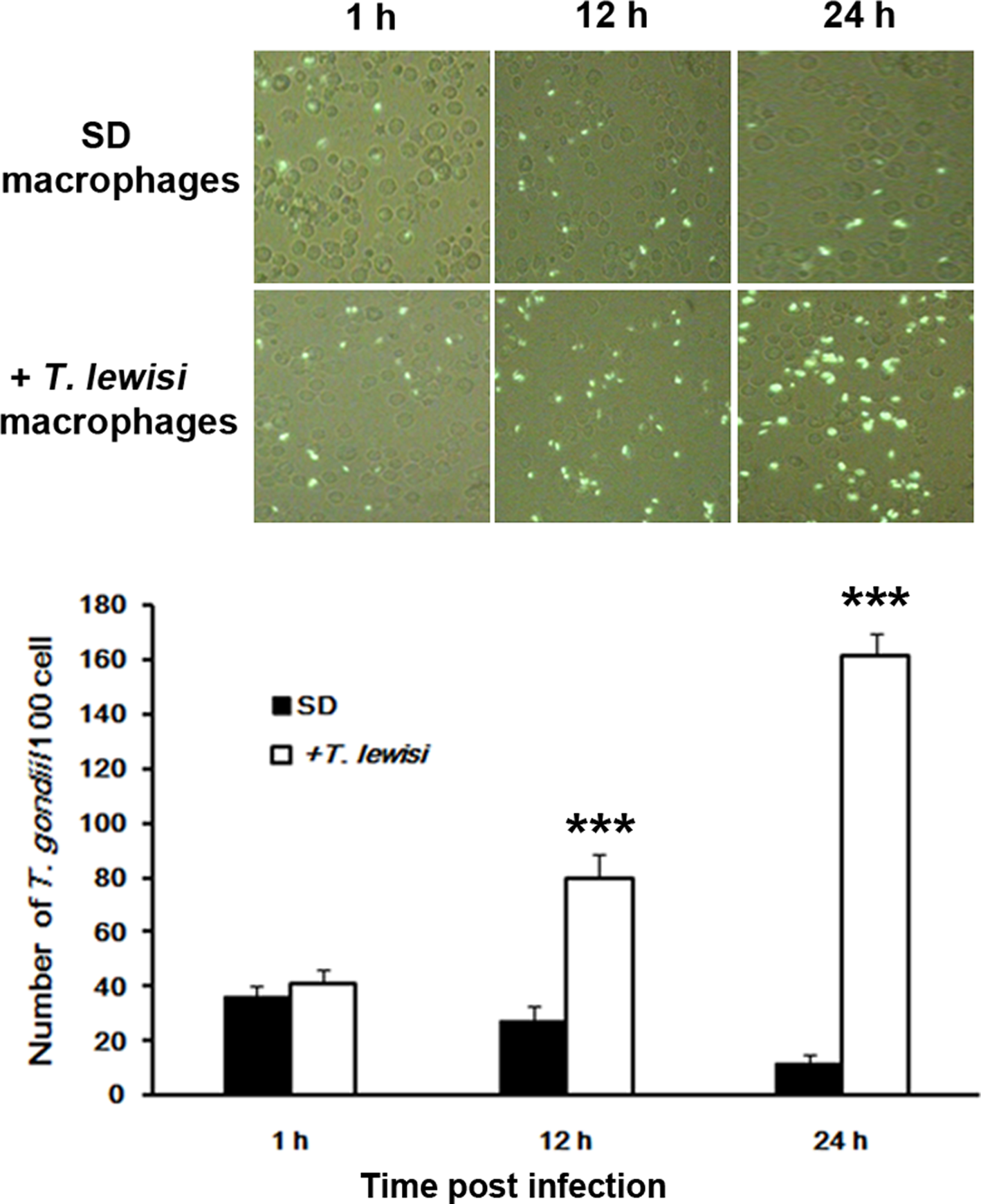
Fig. 5. Comparison of Toxoplasma gondii proliferation in macrophages of T. lewisi infected Sprague-Dawley rats and control animals. A. Analysis of T. gondii proliferation in macrophages by fluorescent microscopy. SD Macrophages, taken from SD rats infected with T.gondii alone; + T. lewisi macrophages, taken from SD rats infected with T.gondii and T. lewisi. B. Number of T. gondii per 100 macrophages counted 1, 12 and 24 h after infection. Error bars indicate standard deviations of measured values (n = 3). Data were analysed using the unpaired Student's t-test (two-tailed) and statistical differences were designated by *(P < 0.05), **(0.001 < P < 0.01) and ***(P < 0.001).
In contrast in mice, it seems that the increase in NO production after infection with T. musculi confers no obvious resistance to the proliferation of T. gondii tachyzoites in the peritoneal macrophages of mice (data not shown). However, in vivo, the infection of mice with T. musculi was observed to extend the survival time of hosts infected with T. gondii tachyzoites (RH strain). This was especially notable when the host was infected by T. musculi before T. gondii (Supplementary Fig. 3).
Discussion
Toxoplasma gondii, as a successful parasite, can infect almost all warm-blooded vertebrates and therefore has the potential to interact with a wide range of other pathogens that infect its potential hosts. Rodents potentially play a key role in T. gondii transmission and this raises the broad question as to whether co-infection with typical rodent trypanosomes, such as T. lewisi and T. musculi, has any effect on the transmission of T. gondii?
Previous research has shown that Trypanosoma lewisi and T. musculi, like other species of trypanosome, can suppress the immunocompetence of hosts and increase susceptibility to opportunistic infections such as Toxoplasma gondii (Carrera et al., Reference Carrera, Carmona, Guerrero and Castillo2009). It has also been demonstrated that T. lewisi and T. musculi can reduce immunity by influencing the function of macrophages (Carrera et al., Reference Carrera, Carmona, Guerrero and Castillo2009). However, the mechanism of these processes of immunosuppression is still unclear. It is well known that peritoneal macrophages of the rat are naturally resistant to T. gondii infection (Dubey and Frenkel, Reference Dubey and Frenkel1998) and recent studies have demonstrated that the mechanism of such resistance is strongly linked to higher expression of iNOS and lower expression of arginase in the rat peritoneal macrophage (Li et al., Reference Li, Zhao, Zhu, Ren, Nie, Gao, Gao, Yang, Zhou, Shen, Wang, Lu, Chen, Hide, Ayala and Lun2012). In this study, we propose that T. lewisi and T. musculi may modulate NO production and iNOS expression in peritoneal macrophages to affect the immunity status of their hosts and that this, in turn, influences infection with T. gondii.
Consistent with previous studies, this study demonstrated that T. lewisi could increase the susceptibility of immunocompetent rats to T. gondii. More importantly, for the first time, it has demonstrated that the host immunosuppression, caused by T. lewisi, acts through inhibiting the expression of iNOS. This consequently lowers the production of NO and, at the same time, increases the expression of arginase and, consequently, urea production. This increase is clearly related to the progression of infection of T. lewisi as it is not found in the delayed co-infection group. The formation of cysts in the brains in different strains of rats, following infection with the T. gondii Prugniaud (cyst-forming) strain, showed rat strain-specific variation. We found that the cyst burden in the brains of F344 rats was significantly worse than in the BN or SD rats in the ‘Tl, Tg’ groups. A previous study has shown that the encystment of Toxoplasma in brains of rats that were pre-infected with T. lewisi is dependent on rat strain but not the inoculation dose of Toxoplasma or the age of the rats (Guerrero et al., Reference Guerrero, Chinchilla and Abrahams1997). Similarly, our previous study (Gao et al., Reference Gao, Yi, Wu, Geng, Shen, Lu, Hide, Lai and Lun2015) demonstrated that different strains of adult and newborn rats (LEW, WST, SD, BN and F344) exhibited remarkable differences in susceptibility to cyst formation with the T. gondii Prugniaud strain and that this was related to the inherent ratio of iNOS/Arginase expression in peritoneal macrophages. For example, that study showed that F344 rats had a higher susceptibility than BN or SD rats in both adult and newborn stages. However, even newborn rats of the LEW or WST strains exhibited a high degree of resistance to cyst formation with either a lower number or absence of cysts in the brain. These results are consistent with the results confirmed by Li and colleagues that showed that lower iNOS expression and NO production were found in F344 and BN rats while LEW and WST rats express higher levels of iNOS with increased NO production (Li et al., Reference Li, Zhao, Zhu, Ren, Nie, Gao, Gao, Yang, Zhou, Shen, Wang, Lu, Chen, Hide, Ayala and Lun2012). Since these inbred lines of rats represent a tiny sample of the phenotypic variability in global rat populations, it is likely, in nature, that there will be a high degree of individual variability in the iNOS/Arginase expression balance found in wild populations of rats. Based on our data, it is likely that, in nature, pre-infection with T. lewisi is detrimental to survivability following infection with T. gondii. We would predict that, in areas of the high prevalence of T. lewisi infections, natural selection would drive rat populations towards higher frequencies of rats with inherent high resistance to T. gondii and inherently high iNOS/Arginase expression ratios (as observed in strains like LEW and WST).
In this study, we found that infection with T. musculi could improve the immunocompetence of mice infected with the Toxoplasma cyst forming strain (Prugniaud). In the ‘Tm, Tg’ group, where pre-infection with T. musculi was performed, there was also a considerable improvement in mouse survivability following infection with T. gondii tachyzoites (RH strain). This improvement was related to the increase in NO production and iNOS expression in mouse peritoneal macrophages found during T. musculi infection. In nature, therefore, this mechanism will drive natural selection to increase the frequency of T. musculi and T. gondii co-infected mice due to improved survivability. We would predict that this will increase the opportunities for the spread of T. gondii to other kinds of hosts in areas where co-infection is high. However, this prediction seems contrary to the previous study by Piccolo-Johanning et al. (Reference Piccolo-Johanning, Kellerman-Guterman, Valerio-Campos and Chinchilla-Carmona2013), who proposed that T. musculi could inhibit the immunocompetence of Swiss Webster mice by producing increased cyst burdens and a shortened lifespan. These differences may be attributed to the use of different T. gondii strains – their use of TFC, TLW and TBT stains may behave differently to the PRU strain we used. Clearly further studies are required to clarify the interactions between different parasite strains with different host strains.
Rats, infected with T. gondii, play a vital role in the epidemiology of toxoplasmosis because they can serve as reservoirs of infection for cats, dogs and possibly for pigs (Dubey and Frenkel, Reference Dubey and Frenkel1998). Therefore, we speculate that the immunosuppressive effect of T. lewisi on rats could, though natural selection, help to drive the distribution of co-infected animals, in nature and that could have a strong influence on the transmission and maintenance of the life cycle of T. gondii. In addition, T. lewisi has been suggested as a potential human pathogen, as it shows resistance to lysis by normal human serum and apolipoprotein L-1, and due to a number of reported cases of human infection (Liu and Liu, Reference Liu and Liu1990; Truc et al., Reference Truc, Büscher, Cuny, Gonzatti, Jannin, Joshi, Juyal, Lun, Mattioli, Pays, Simarro, Teixeira, Touratier, Vincendeau and Desquesnes2013; Lun et al., Reference Lun, Reid, Lai and Li2015). In the rare cases of human infection, it is possible that T. lewisi infection may hasten the onset of toxoplasmosis and lead to more severe symptoms.
In conclusion, we propose that the coinfection of two or more pathogens may be beneficial to the spread and/or maintenance of some parasites, but on the other hand, may also be harmful to the health of the hosts.
Supplementary material
The supplementary material for this article can be found at https://doi.org/10.1017/S0031182021000196
Acknowledgements
Trypanosoma gondii RH-GFP strain was kindly provided by Dr X.N. Xuan of the National Research Centre for Protozoan Diseases, Obihiro University, Obihiro, Japan Obihiro University of Agriculture and Veterinàry Medicine.
Authors' contributions
JM Gao, SQ Yi, QQ Geng and ZR Lun designed the study. JM Gao, ZS Xu and DH Lai drafted the manuscript and undertook data extraction and screening. G Hide, ZR Lun and DH Lai critically reviewed the paper. All authors contributed to the acquisition, analysis or interpretation of data for the work. All authors approved the final version and agree to be accountable for all aspects of the work.
Financial support
This study was supported by grants from the National Science and Technology Major Project of China (2018ZX10101002) and the National Natural Science Foundation of China (31720103918 and 31672276 to ZRL, 31772445 to DHL) and Natural Sciences Foundation of Guangdong Province (2016A030306048).
Conflict of interest
We declare no competing interests.
Ethical standards
The animal ethical approval of sample collection was obtained from the Institutional Review Board of Animal Care at Sun Yat-Sen University (permit no. 3167227).