Introduction
Advances in diagnosis and treatment have led to improved clinical outcomes of pediatric brain tumor patients. The 5-year survival rate of these patients has increased from 55% in the 1970s to over 70% in more recent cohorts (Armstrong et al., Reference Armstrong, Liu, Yasui, Huang, Ness, Leisenring and Packer2009; Gurney et al., Reference Gurney, Kadan-Lottick, Packer, Neglia, Sklar, Punyko and Robison2003; Ostrom et al., Reference Ostrom, de Blank, Kruchko, Petersen, Liao, Finlay and Barnholtz-Sloan2015). With improved treatment outcomes, research on long-term survivorship of childhood brain tumors has reported adverse health, disrupted quality of life, and impaired cognitive and social outcomes (Gurney et al., Reference Gurney, Krull, Kadan-Lottick, Nicholson, Nathan, Zebrack and Ness2009; Kirchhoff et al., Reference Kirchhoff, Krull, Ness, Armstrong, Park, Stovall and Leisenring2011; Robinson, Fraley, Pearson, Kuttesch, & Compas, Reference Robinson, Fraley, Pearson, Kuttesch and Compas2013). These findings highlight the importance of investigating neural underpinnings associated with childhood brain tumor and its treatment.
Working memory impairment is considered among the primary neurocognitive deficits that contribute to poor long-term outcomes of brain tumor survivors (Palmer, Reference Palmer2008; Wolfe, Madan-Swain, & Kana, Reference Wolfe, Madan-Swain and Kana2012). The core deficits in attention, processing speed, and working memory are associated with treatment-related global cognitive deficits (Dennis et al., Reference Dennis, Spiegler, Obonsawin, Maria, Cowell, Hoffman and Ehrlich1992; Reeves et al., Reference Reeves, Palmer, Reddick, Merchant, Buchanan, Gajjar and Mulhern2006; Waber et al., Reference Waber, Pomeroy, Chiverton, Kieran, Scott, Goumnerova and Rivkin2006). Working memory deficits appear to mediate general cognitive abilities in cancer survivors (Schatz, Kramer, Ablin, & Matthay, Reference Schatz, Kramer, Ablin and Matthay2000).
Therefore, it is not surprising that working memory is a common deficit among many neurological conditions, such as schizophrenia (Perlstein, Dixit, Carter, Noll, & Cohen, Reference Perlstein, Dixit, Carter, Noll and Cohen2003), multiple sclerosis (Sweet, Rao, Primeau, Durgerian, & Cohen, Reference Sweet, Rao, Primeau, Durgerian and Cohen2006), and traumatic brain injury (Medaglia et al., Reference Medaglia, Chiou, Slocomb, Fitzpatrick, Wardecker, Ramanathan and Hillary2012) because working memory is a core executive function and is an essential component for higher-order cognitive processes in humans (Baddeley, Reference Baddeley2012; Smith & Jonides, Reference Smith and Jonides1997, Reference Smith and Jonides1998). The distributed neural network of the frontal-parietal system has been reported consistently in the functional magnetic resonance imaging (fMRI) literature. Greater prefrontal and parietal blood-oxygen level dependent (BOLD) activations observed in fMRI are associated with increased working memory load (Owen, McMillan, Laird, & Bullmore, Reference Owen, McMillan, Laird and Bullmore2005).
However, current understanding of the neural mechanisms underlying working memory impairments in adult survivors of childhood brain tumors is limited, and little fMRI research with childhood brain tumor survivors has been reported. To date, two research groups have reported on the feasibility of the letter n-back paradigm in pediatric survivors of childhood brain tumors (Robinson et al., Reference Robinson, Pearson, Cannistraci, Anderson, Kuttesch, Wymer and Compas2014a, Reference Robinson, Pearson, Cannistraci, Anderson, Kuttesch, Wymer and Compas2014b; Wolfe et al., Reference Wolfe, Madan-Swain, Hunter, Reddy, Banos and Kana2013). In nine adolescent survivors of pediatric posterior fossa tumors, Wolfe and colleagues (Reference Wolfe, Madan-Swain, Hunter, Reddy, Banos and Kana2013) identified a frontal-parietal activation pattern using a contrast of 0-, 1-, and 2-back combined versus fixation. This study demonstrated a broadly typical activation pattern for survivors during the combined vigilance and working memory load n-back task. A control group, however, was not used in this study so it is unclear how this activation may differ from neurotypical adolescents.
In a separate study, Robinson and colleagues (2014b) examined 17 child and adolescent brain tumor survivors on average 5.64 years post diagnosis of heterogeneous brain tumors and locations. They compared the survivors to 15 similar-age children. Whole-brain examination of the group by n-back load (0-, 1-, 2-, and 3-back) interaction revealed a significant cluster in the left dorsal anterior cingulate cortex (DACC) (Robinson et al., Reference Robinson, Pearson, Cannistraci, Anderson, Kuttesch, Wymer and Compas2014b). More specifically, the survivors showed significant activation in left DACC on the 1- and 2-back relative to baseline, whereas the comparison group showed significant deactivation on these contrasts. Across groups, n-back total accuracy was negatively correlated with BOLD response during the 1-back (−.40) but not correlated with BOLD response during 0-, 2-, or 3-back. Furthermore, the left DACC BOLD response during the 1-back positively correlated with parent report of executive dysfunction (i.e., .36; BRIEF Global Executive Composite) and was negatively associated with the WISC-IV Working Memory Index (i.e., −.37). Combined, these findings suggest the importance of separating the role of vigilance (i.e., 1-back, identifying a letter that immediately repeats itself) from working memory in the contribution to clinical measures of executive dysfunction. With this same sample and fMRI data, the authors (Robinson et al., Reference Robinson, Pearson, Cannistraci, Anderson, Kuttesch, Wymer and Compas2014a) took a non-traditional approach of identifying regions of activation in each group separately on specific contrasts (i.e., 2- vs. 0-back, and 3- vs. 0-back) and then “force-prescribed” these cluster regions onto the other group. Although not a direct comparison between groups at the whole-brain level, activity levels in these prescribed regions were extracted from both groups and correlated with multiple coping and emotional functioning measures. In survivors, the left prefrontal cortex (during 2-back vs. 0-back) correlated with ratings of Anxiety/Depression (−.49), Attention Problems (−.61), and Disengagement Coping (−.61). The authors report that the survivors who tended to use disengagement coping were less likely to recruit the frontal regions. In summary, these three studies have shown the feasibility of using the letter n-back paradigm in fMRI studies of survivors of pediatric brain tumors. Each demonstrated traditional areas of activation with this paradigm and highlighted the importance of sample characteristics, level of task difficulty, and diverse analytic approaches. The authors suggest the possibility of frontal dysfunction in survivors but more work is needed directly comparing survivors to healthy controls and deconstructing the meaning of BOLD activation changes with performance correlations. Furthermore, little is known about the neural correlates of working memory BOLD response in long-term adult survivors of pediatric brain tumors.
The current fMRI study aimed to identify the neural mechanisms underlying working memory difficulty in adult survivors of childhood posterior fossa brain tumors relative to a healthy and demographically matched control sample. Whole-brain fMRI analyses relative to healthy controls were used. We found shared or common regions of brain activation in the frontal-parietal working memory network across groups (i.e., conjunction analyses) and, therefore, confirmed the underlying network was used similarly across both groups and similar to the n-back literature. In addition, standardized clinical measures of working memory (Auditory Consonant Trigrams and Digit Span Backwards) were examined for group differences and in relationship to the change in BOLD signal in activated regions during the n-back.
Methods
Participants
The study was reviewed and approved by the local institutional review boards. Adults who had been treated for a pediatric posterior fossa brain tumor were identified from a large southeastern hospital system database, a previous longitudinal childhood brain tumor study, and an advertisement published in an annual newsletter circulated by the state Brain Tumor Foundation in which survivors were encouraged to call to learn more about the study. Before being enrolled in the study, written consent was provided by all adult participants. Parental consent and participant assent was obtained from two individuals who were 17 years old.
Participants were excluded if English was not their first language, if they met criteria for pervasive developmental disorders, if they indicated a diagnosis of neurofibromatosis, or if they had experienced any other significant neurological insult (e.g., traumatic brain injury). All participants were over the age of 18 (with the exception of two 17-year-old survivors) and were at least 5 years past their most recent diagnosis (with the exception of one survivor who was 4.5 years post diagnosis). Information about the brain tumor and treatments were obtained from a thorough retrospective medical records review. None of the survivors had history of progressions or recurrences of their brain tumor. Twenty-seven posterior fossa brain tumor survivors completed both neuroimaging and neuropsychological testing. Ten participants’ data were excluded due to either unacceptable motion artifacts in images (>3 mm, 8 survivors) or artifact in the cortical regions (2 survivors). In total, 17 adult survivors of posterior fossa tumors were included in the current study. To address potential selection bias, we evaluated whether there were significant differences in behavioral performance when comparing survivors with acceptable imaging and survivors with artifact; t test analyses indicated that there were no significant differences between the two groups.
Control participants were recruited through the institutional psychological research participant pool at a large urban public university and community fliers. Control participants were screened for a history of as well as current psychopathology with the Structured Clinical Interview for DSM-IV-TR Axis 1 (First, Spitzer, Gibbon, & Williams, Reference First, Spitzer, Gibbon and Williams1997). In addition, individuals with a history of neurological or developmental conditions were excluded to ensure that these individuals represented a healthy comparison group. Therefore, no participants in the comparison group had a history of or were diagnosed with attention deficit hyperactivity disorder (ADHD). Three survivors were diagnosed with acquired ADHD during childhood but none were taking stimulant medications. Cognitive performance and BOLD response data for these three survivors were not outliers, nor appreciably different from the other survivors.
Procedure
Behavioral Measures
Auditory Consonant Trigrams (ACT)
The ACT (Brown, Reference Brown1958; Peterson & Peterson, Reference Peterson and Peterson1959) was administered to all participants as part of a larger cognitive battery as an out-of-scanner behavioral measure of working memory. Participants were asked to remember three consonants (e.g., B-D-T) that were read by the examiner. They were then asked to count backward from a given number to prevent rehearsal of the consonants. After 9, 18, or 36 s, the participants were asked to recall the three consonants. Performance was computed into Z-scores based on normative data (Stuss, Reference Stuss1987).
Wechsler Memory Scale Digit Span
The Digit Span subtest from the Wechsler Memory Scale (Wechsler, Reference Wechsler1997) also was used as a measure of working memory. In addition to the summary normative scaled score, the maximum number of digits recalled on the forward and backward conditions were recorded and analyzed separately, with age co-varied, to differentiate the constructs of attention span (forward condition) and mental manipulation/working memory span (backward condition) within the task.
Wechsler Abbreviated Scale of Intelligence (WASI)
The four subtest version (WASI) was used to estimate IQ (Wechsler, Reference Wechsler2011): Vocabulary, Similarities, Block Design and Matrix Reasoning. WASI standard scores were used for descriptive purposes only. This measure was not used as a covariate due to previously described limitations of this approach in developmental populations (Dennis et al., Reference Dennis, Francis, Cirino, Schachar, Barnes and Fletcher2009).
fMRI Exam with Letter N-Back Working Memory Task Paradigm
Functional MRI data were acquired using a 3 Tesla Siemens Trio MRI scanner using a 12-channel head coil. Cushions and forehead straps were placed around each participant’s head to minimize head movement.
During the n-back task, the participant was asked to monitor a series of letters and to respond yes or no with either his/her index or middle finger on a button box if an item was presented n-items before (ranging from 1 to 3 letters back). A higher “n” value (i.e., 2-back and 3-back) represented a higher load due to higher monitoring, updating and manipulating demands. In contrast, an “n” value of 0 (i.e., the 0-back task) was conceptualized as a measure of vigilance as each participant responded yes or no if a specific letter appeared. In the current study, the 2-back versus 0-back and 3-back versus 0-back contrasts were used to examine working memory more precisely as the common monitoring and vigilance requirements of the tasks were removed.
The task consisted of a block design paradigm with a total of five runs. Eprime (2.0.8) was used for stimuli presentation and accuracy and response time data acquisition. Each run consisted of five different blocks (crosshair, 0-, 1-, 2-, and 3-back blocks) and a fixation period (where a cross was presented on the screen). The blocks were counterbalanced between separate runs to minimize order effects. Each block consisted of fifteen letters, where five pre-specified stimuli were the correct targets and the remaining ten letters were non-targets. Each block was preceded by a screen with written instructions for the task lasting 3000 ms, and each letter stimulus was presented for 500 ms with an inter-stimulus interval of 2500 ms between each letter presentation. The baseline crosshair-fixation block consisted of a cross on the screen which appeared for 500 ms at a time, separated by 2500 ms of blank screen between each cross presentation. Each run lasted approximately 4 min, yielding a total task time of 20 min for all five runs. Working memory was defined as the average percent correct of the 2-back and 3-back conditions across all five runs, while the vigilance condition was defined as the average percent correct on the 0-back task across all five runs.
Participants were trained on the n-back task before they entered the scanner. Participants received a standardized set of instructions on paper. They were given the opportunity to learn the task in an untimed setting and were corrected when they made a mistake. Participants were then administered abbreviated versions of 0- through 3-back conditions on a laptop connected to a button box identical to the one that was used in the scanner. The stimuli on the screen of the laptop were also identical to the screen projected in the scanner to increase familiarity with the format of the task. These steps were taken to ensure that the participants understood the instructions of the task and were familiar with the format and response procedure. This 5-min procedure did not provide the participants with extensive practice or training before the scanner paradigm.
Task-dependent image series were collected using a gradient-recalled T2*-weighted echo-planar-imaging (EPI) sequence based on BOLD contrast. The primary imaging parameters for the BOLD contrast included: 40 slices, 3 mm slice thickness and 0 mm slice gap, repetition time (TR)=2130 ms, echo time (TE)=30 ms, flip angle=90 degrees, nominal resolution=3×3×3 mm3. For anatomical registration, high-resolution T1-weighted structural images were acquired with a three-dimensional (3D) magnetization prepared rapid gradient echo (3D MPRAGE) sequence using the following parameters: 176 sagittal slices, field of view=256 mm×256 mm, 1 mm3 voxel size, TR=2250 ms, TE=3.98 ms, inversion time TI=850 ms, flip angle=9 degrees.
fMRI Data Analyses
fMRI data analysis was conducted using FEAT (fMRI Expert Analysis Tool) Version 6.01, which is part of FSL (fMRIB’s Software Library, www.fmrib.ox.ac.uk/fsl). Data from all five runs were concatenated and preprocessed in the following sequence: motion correction with the MCFLIRT tool of FSL; brain extraction using the BET tool of FSL; slice timing correction; spatial smoothing with Gaussian kernel (full width half maximum=5 mm); high pass temporal filtering. The preprocessed data was then registered to its corresponding high resolution T1 MPRAGE images and subsequently to the standard brain template (MNI152 T1 2 mm) using linear boundary based registration (BBR) (Greve & Fischl, Reference Greve and Fischl2009).
General linear modeling was performed using FILM on individual data. A total of 26 regressors were entered into the GLM setup: 13 base regressors (canonical HRF convoluted 0-, 1-, 2-, 3-back timing blocks, crosshair block, instruction block, interstimulus interval block, six estimated translation and rotation parameters) and their first derivatives. Higher level statistical analyses for contrasts were carried out using FLAME (fMRIB’s Local Analysis of Mixed Effects) stage 1. Z statistic images were thresholded using clusters determined by Z >1.96 and a corrected cluster significance threshold of p=.05. Cluster-extent based thresholding was used for multiple comparisons correction. Group membership (i.e., survivor vs. control) was entered as a regressor in the group level GLM.
The 2-back versus 0-back and 3-back versus 0-back contrasts were chosen to represent working memory relative to vigilance to elicit brain activation related to working memory neurophysiology. The 2-back versus 0-back and 3-back versus 0-back contrasts were further masked by the 2-back versus crosshair and 3-back versus crosshair contrasts, respectively, to identify regions of positive signal change (i.e., activation rather than deactivation). Given that differences on the 2-back versus 0-back contrast may be due to changes in activation levels on the 0-back condition (rather than the working memory 2-back condition), we deemed it necessary to examine the 0-back versus crosshair contrast to determine whether BOLD activations in this contrast existed between the two groups. Specifically, we reasoned that differences on the 2-back versus 0-back contrast may be interpreted in three possible ways: (1) higher activations on the 2-back task in the survivor group compared to the control group, with identical activations on the 0-back vigilance task, (2) lower activations on the 0-back task in the survivor group compared to the control group, with identical activations on the 2-back working memory task, and (3) a combination of higher 2-back activation and lower 0-back activation in the survivor group compared to the control group. Given these three possible interpretations, the 0-back versus crosshair contrast was probed to determine whether group differences existed on the vigilance task.
Spherical regions of interest (ROIs) were created with a radius of 3 mm and centered at the coordinates of the peak value of the Z-score. The percentage of the BOLD signal change was obtained with Featquery in these ROIs and correlated with behavioral performance using bivariate Pearson correlations.
Finally, conjunction analyses were performed to determine group similarities in BOLD response to the 2-back versus 0-back contrast, which is most commonly reported in the literature. Conjunction analyses are used to find common activation patterns across groups by examining voxels that are statistically significant on the contrast in both groups. Conjunction is based on the minimum statistic and tests the null hypothesis that one or more of the effects are null (the “conjunction null”). The conjunction was conducted with FSL easythresh script (http://www2.warwick.ac.uk/fac/sci/statistics/staff/academicresearch/Nichols/scripts/fsl/easythresh_conj.sh) and tested at a level of p=.05. For more details on the model used and the concepts, procedures and assumptions underlying the model, refer to Price and Friston (Reference Price and Friston1997).
Statistical Analyses for Behavioral Data
Independent t tests were used to evaluate whether survivors and controls differed with respect to their performance on all behavioral measures at a significance level of p<.05. Effect sizes were also calculated (Cohen’s d) to illustrate the magnitude of the standardized difference between survivor’s and control’s performance means.
Results
Overall, 17 adult survivors and 17 demographically matched healthy controls were included in the analyses. Groups were matched for gender, age, handedness, and socioeconomic status (see Table 1 for demographic details). Both groups had 11 females and 6 males. Survivors were between 17 and 35 years old, with an average age of 23.2 years (SD=5.9). Survivors were an average of 15.5 years post diagnosis (SD=7.6 years). Nine survivors were diagnosed with a high-grade embryonal tumor, while eight survivors were diagnosed with a low-grade astrocytic tumor. Table 1 provides a complete list of the characteristics of the sample.
Table 1. Demographic, Diagnostic, and Treatment Variables of the Sample
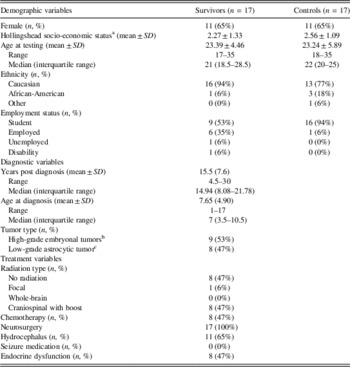
a Current socioeconomic status, calculated using the Hollingshead Four Factor Index of Social Status (Hollingshead, 1975). Family SES was used in instances where the individual reported being financially dependent on their family.
b Eight medulloblastoma, 1 primitive neuroectodermal tumor, not otherwise specified.
c Seven juvenile pilocytic astrocytoma, one Fibrillary astrocytoma.
Behavioral Performance
We predicted that adult survivors of childhood brain tumor would exhibit lower performance on the n-back fMRI paradigm and standardized clinical measures. Results of one-tailed independent t tests showed that there were significant differences between the survivor and control groups on measures of 2-back performance, 3-back performance, WASI, the longest delay of the ACT (36-s condition), Digit Span performance, and the number of digits recalled in the forward condition of Digit Span. On all of these measures, survivors performed significantly lower than controls. The group means, standard deviations, differences in performance on each measure, and the effect sizes of group difference are listed in Table 2.
Table 2. Performance measures of the survivor and control groups

** Indicates significance of p<.01; with regard to directionality, we hypothesized that survivors would perform lower than controls on all measures.
a Forward digits performance remained significantly different between both groups after adding age as a covariate. Activation=percent change in BOLD signal on the 2-back condition as compared to the 0-back condition.
ACT=Auditory Consonant Trigrams.
As a secondary analysis, t tests were conducted to identify the group differences that existed on the percent change of BOLD signal during the activation and behavioral performance when bifurcating the survivors into the low grade astrocytoma group (n=8) versus the high-grade embryonal tumors (n=9). The two groups were matched for age, age at diagnosis and time since diagnosis.
Results showed that there were significant differences between the groups of high-grade embryonal tumor survivors and low-grade astrocytoma survivors on the behavioral performance of the 3-back task, t(15)=2.22, p=.04, d=1.15. Significant differences were present in the ACT 9s (t(15)=5.02; p<.05; d=2.59), 18s (t(15)=2.42; p=.03; d=1.25) and 36s (t(15)=2.42; p=.03; d=1.25) measures. There also were significant differences in the Digit Span scaled score (t(15)=2.86; p=.01; d=1.48), as well as the raw scores of the Digit Span Forward (t(15)=2.86; p=.01; d=1.48) and Backward (t(15)=2.20; p=.04; d=1.14). In all of these analyses, the high-grade embryonal tumor group performed worse than the low-grade astrocytoma group. Analyses of the treatment-related and health-related factors of the two survivor groups indicated that the high-grade embryonal tumor group evidenced higher rates of radiation treatment (χ²(1, n=17)=9.92; p<.001; φ=0.76), chemotherapy treatment (χ²(1, n=17)=13.43; p<.001; φ=0.89), and endocrine dysfunction (χ²(1, n=17)=7.24; p<.001; φ=0.65) when compared to the low-grade astrocytoma group. As such, the treatment-related and health-related factors related to survivorship of a high-grade embryonal tumor group (i.e., radiation, chemotherapy, and endocrine dysfunction) were associated with lower cognitive performance on behavioral working memory tasks.
fMRI Results
We predicted that the neural network recruited in working memory would be disrupted in survivors. Furthermore, we predicted that the correlations between the activation-induced percent change in BOLD signal in prefrontal regions and working memory performance (in and outside of the scanner) would be negatively related.
Whole-brain analyses revealed significant group differences for the 2-back versus 0-back contrast in the left superior frontal/middle frontal gyri and left parietal cortex, with survivors exhibiting higher activations than controls in the cortical structures shown in Figure 1A. The locations and z-values of the peaks and subpeaks that emerged as significantly different between the two groups for the 2-back versus 0-back contrast are summarized in Table 3. No group differences were present when evaluating the 0-back versus crosshair vigilance contrast condition or the 3-back versus 0-back working memory contrast.

Fig. 1 (A) Areas of significant working memory BOLD activation differences between survivors and controls on the 2-back versus 0-back contrast. Survivors exhibited higher activations than healthy controls in left superior frontal gyrus and parietal cortex regions. See Table 3 for cluster size and peak MNI coordinates. (B) Scatterplots are displayed to illustrate relationships between mean percent signal change in frontal and parietal ROIs and n-back performance for survivors and controls. Left parietal cortex activation was negatively correlated with 0-back performance only r(32)=−0.35, p<.05. Left superior frontal gyrus activation was negatively correlated with the 2-back (r(32)=−0.42, p<.05) and 3-back conditions (r(32)=−0.48, p<.01) but not the lower load n-back conditions. (C) Significant regions of similar working memory BOLD activation among survivors and controls on the 2-back versus 0-back contrast. Conjunction analyses indicated similar bilateral activations in frontal and parietal lobes, thalamus and cerebellum. See Table 5 for cluster size and peak MNI coordinates. All brain images are presented in radiological space. Activation maps were superimposed on a T1-weighted standard MNI152 brain template. Color bar indicates Z-values (results thresholded at p=.05, cluster-based corrections).
Table 3. Location of significant working memory activation differences between survivors and controls: Peaks and subpeaks for the 2-back versus 0-back contrast

Note. Peak voxel coordinated (MNI space) and statistical values (Z>1.96) are listed for significant clusters (P cluster size corrected<.05).
Correlations of Brain Activation and Cognitive Performance
Mean percent signal changes in the two peak regions, identified by whole-brain analyses (i.e., left superior/middle frontal gyri and left parietal cortex), were examined for their relationships with behavioral performance. We predicted that the correlations between the activation induced percent signal change would be negatively related with working memory (convergent support) and less associated with forward attention span or vigilance measures (discriminant support). Pearson bivariate correlations between the signal change and behavioral measures were evaluated to determine whether activation levels in these ROIs in the working memory condition relative to the vigilance condition were correlated with behavioral performances on various working memory measures. Analyses revealed that higher levels of activations in prefrontal regions were associated with lower behavioral performance on higher-load working memory tasks. In addition, higher activity in the left parietal cortex was negatively correlated with 0-back overall accuracy and the longest delay of the ACT task (36s). Bivariate correlations between mean percent signal change in the ROIs and behavioral performance are shown in Table 4. Scatterplots of the relationships between working memory tasks and mean percent signal change in the left superior frontal gyrus and the left parietal cortex are represented in Figure 1B and 2.

Fig. 2 Scatterplots of mean percent signal change in the left superior frontal gyrus ROI and behavioral performance on working memory tasks for survivors and healthy controls. (A) Left superior frontal gyrus activation was negatively correlated with the longest delay condition of the Auditory Consonant Trigram task r(32)=−0.34, p<.05. This ROI was not significantly correlated with the 9s (r(32)=−0.10; p>.05) and 18s (r(32)=−0.18; p>.05) shorter delay conditions of the ACT task. (B) Left superior frontal gyrus activation was negatively correlated with the Digit Span Backward Digits measure, r(32)=−0.36, p<.05, but not the Forward Digits measure, r(32)=−0.27, p<.05. These findings were consistent after covarying for age.
Table 4. Bivariate correlations between mean percent signal change and behavioral performance

*Indicates significance at the.05 level.
**Indicates significance at the.01 level.
a r values are partial correlations after covarying for age.
ACT=Auditory Consonant Trigrams.
Secondary analyses were conducted to determine if certain demographic or treatment variables related to behavioral performance and activations in the peak voxels (i.e., left superior frontal gyrus and left parietal cortex). Bivariate correlations indicated that age at testing of the survivor group was significantly correlated to the level of activation in the left superior frontal gyrus, r(16)=.487, p=.047. No other demographic or treatment variables were related to the level of activation in either ROI. In addition, age at diagnosis of the survivor group was significantly correlated with behavioral performance on the ACT (36s), r(16)=.772, p<.01, but not the other behavioral measures. In short, no demographic variable correlated with n-back performance. However, survivors who were older at the time of their diagnosis performed better on a behavioral measure of working memory. Similarly, time since diagnosis had a statistically significant negative correlation with behavioral performance on the ACT (36s), r(16)=−.519, p=.03. A longer time since diagnosis was related to worse behavioral performance on the working memory measure.
Finally, we conducted t tests to determine whether the tumor grade (i.e., low grade astrocytomas vs. high grade embryonal tumors) affects activation in the aforementioned left prefrontal and parietal regions. There was no significant difference between the two groups with respect to the percent signal change in the superior frontal gyrus or parietal cortex.
Conjunction Analysis
Whole-brain analyses revealed regions commonly activated in both the survivor and control groups were in the right superior parietal lobe, right paracingulate gyrus, right insular cortex, left middle frontal gyrus, right and left thalamus, and right lateral cerebellum (see Table 5). The pattern of activation for the 2-back versus 0-back contrast showed significant overlap among the survivor group and the control group across the frontal-parietal working memory network. Common areas of activation among the two groups are presented in Figure 2C.
Table 5. Location of significant working memory regions of activation conjunction among survivors and controls for the 2-back versus 0-back contrast

Note. Peak voxel coordinated (MNI space) and statistical values (Z>1.96) are listed for significant clusters (P cluster size corrected<.05).
Discussion
The current study identified significantly greater levels of percent signal change in the BOLD activations of the left prefrontal lobe and left parietal lobe in adult survivors of childhood posterior fossa brain tumors relative to a healthy demographically similar control group during a verbal working memory task (i.e., working memory 2-back vs. a vigilance 0-back task). These increased BOLD signals in survivors during working memory reflect that the survivor group recruited greater frontal and parietal activations compared to controls. The increased prefrontal activations are consistent with our predictions based on previous neurological samples with the n-back working memory task; increased prefrontal cortex activation has been reported reliably to be related to the working memory demands of the 2-back task. Furthermore, differences during the 2-back working memory task are due to higher activations in survivors relative to controls, as there were no BOLD differences between the two groups on the 0-back versus crosshair contrast.
The frontal activation is specifically associated with working memory as operationalized by 2-back and 3-back scanner task performance as well as the clinical measures of working memory. In addition, greater left superior frontal region activation was associated with lower performance on the 2-back working memory task, as well as lower performance on the clinical measures of working memory (ACT 36-s delay and Digit Span Backwards). Further supporting the specificity of the working memory association with this frontal activation, the percent change in BOLD activation was not associated with forward digit span or shorter delays of the ACT. In contrast, the percent change in BOLD activation in the parietal region was negatively associated with ACT 36-s delay and 0-back accuracy. Together, these findings suggest that the cognitive demands of the more challenging working memory tasks are specifically associated with these increased regions of hemodynamic response and not overall differences in cognitive ability.
Furthermore, the increased parietal lobe activations may be due to increased effort and cognitive demands of verbal working memory, vigilance demands, or even toward basic visual processing of the letters. For instance, it is possible that the upper and lower case changes in the stimuli (e.g., M, t, R, m) elicited greater basic visual processing and phonological working memory demands in the survivors. We developed the stimuli in this manner to ensure that it was a letter/verbal n-back task and to remove the potential confound of shape that sometimes exists when the case of the letter is the same. However, this stimuli specification may have created greater basic visual processing demands that interacted with the working memory demands of the task. It is also possible that increased parietal lobe activations reflect greater vigilance demands. In line with this possibility, behavioral performance on the 0-back (i.e., vigilance task) was significantly correlated with activity in the parietal region, with worse performance associated with higher BOLD signal level. In addition, the upper and lower case stimuli may have also contributed to the greater left-lateralized frontal and parietal working memory network, given the increased phonological verbal working memory. The left hemisphere findings are consistent with prior letter n-back tasks (Owen et al., Reference Owen, McMillan, Laird and Bullmore2005; Robinson et al., Reference Robinson, Pearson, Cannistraci, Anderson, Kuttesch, Wymer and Compas2014b). However, some researchers have suggested the importance of the right prefrontal lobe due to the novelty of the task demands (Hillary et al., Reference Hillary, Medaglia, Gates, Molenaar, Slocomb, Peechatka and Good2011).
Increased prefrontal cortex activation during working memory tasks, as working memory performance declines, has been reported previously in the literature with various neurological populations. Hillary and colleagues suggest that in the context of brain injury, increased BOLD in the prefrontal cortex is not due to a compensatory mechanism or brain reorganization but rather attempts of the neurological group to increase cognitive control in the context of poorer performance (Hillary et al., Reference Hillary, Medaglia, Gates, Molenaar, Slocomb, Peechatka and Good2011). Our findings are in line with this allocation of latent resources theory of the prefrontal lobe which is observed when prefrontal BOLD response increases with greater task demands. The authors explain that these common findings are not due to compensatory recruitment to maintain task performance or brain reorganization but instead due to the fleeting and typical recruitment of existing resources that is also sometimes observed in healthy non-neurological samples. In our study, the percent change in the prefrontal region related to 2-back performance as well as the longest delay of the Auditory Consonant Trigram task and Digit Span Backwards. Furthermore, this transient allocation of prefrontal cognitive control resources was greater in the adult survivors of childhood posterior fossa tumors. It also appeared that the increased parietal activation was associated with poor performance on the ACT; however, this region was not associated with 3- nor 2-back performance but instead was significantly associated 0-back performance. Consequently, it is important for future studies with survivors of childhood brain tumor and other neurological samples to explore the relationship of BOLD activation differences in these regions with other cognitive performance measures.
Although the primary purpose of the study was to determine cerebral regions that are specific to adult survivors of pediatric brain tumors with respect to BOLD response, it was also deemed important to examine the regions that were being similarly activated in both groups. This analysis was performed to ensure that the regions of the brain recruited by survivors to support their performance on a working memory task are consistent with the working memory networks espoused in studies of neurologically healthy controls (Owen et al., Reference Owen, McMillan, Laird and Bullmore2005). Indeed, the current study found that the survivors and controls activated the similar, distributed frontal-parietal working memory network during the 2-back versus 0-back tasks. Comparable activations in the right superior parietal lobe, right paracingulate gyrus, right insular cortex, left middle frontal gyrus, right and left thalamus and right lateral cerebellum were identified in the conjunction analysis. These findings support previous findings and expand to a novel and important group of adult survivors of childhood posterior fossa brain tumors who were on average 15.5 years from diagnosis.
The current results must be considered within the context of the limitations. Although this study was more homogenous in brain tumor location than previous studies (e.g., posterior fossa), approximately half of the sample was high-grade posterior fossa tumor survivors. Therefore, it is possible that there may be differences in neural underpinnings of working memory in survivors with high-grade and more complex treatment histories (e.g., radiation, endocrine treatment) versus those with low-grade cerebellar tumors with surgery only treatment histories. Preliminary analyses comparing these subsamples showed that the high-grade posterior fossa tumor survivors performed worse than low-grade posterior fossa tumor survivors on a variety of behavioral working memory tasks. However, the level to which they activated the frontal and parietal clusters on the 2-back - 0-back contrast was not significantly different, and both groups had large standard deviations. Therefore, small samples such as those in the current study limit the power to detect differences that may exist with a larger sample size. Future studies may focus on a select group such as medulloblastoma or cerebellar juvenile pilocytic astrocytomas and compare functional BOLD activation of each group during working memory tasks to each other as well as to healthy demographically matched peers.
In addition, this unique sample of long-term survivors was recruited by mailings, newsletters, and word of mouth and may have biased the representativeness of the sample. It is possible that survivors with more severe impairments are more eager to participate in clinical research studies. Conversely, it is equally possible that those who are higher functioning are more able to participate and volunteer for studies. Thus, with this design, it is difficult to know how representative this modest sample of long-term survivors is of other posterior fossa survivors. A larger sample would provide greater power to detect important relationships that we may not have found. Likewise, one may also be concerned about differences in the survivors who participated in the study with good versus poor quality imaging data. We explored this possibility, however, and did not find performance differences between these two groups. Therefore, it is important to consider that the results of this study may reflect the best case scenario of working memory performance and underlying neural correlates in pediatric posterior fossa tumor survivors.
It is important to note that the mean IQ of the control group was in the high average range which could reflect the differences between groups that were found. However, on average the n-back and other neuropsychological performance was comparable to the performance reported in other fMRI studies (Hillary et al., Reference Hillary, Medaglia, Gates, Molenaar, Slocomb, Peechatka and Good2011; Medaglia et al., Reference Medaglia, Chiou, Slocomb, Fitzpatrick, Wardecker, Ramanathan and Hillary2012; Robinson et al., Reference Robinson, Pearson, Cannistraci, Anderson, Kuttesch, Wymer and Compas2014b). The current control group was carefully selected from a larger control sample that was consistent with the state of Georgia census for ethnicity and also included first-generation college students which makes it more consistent with community samples. Larger standard deviations are notable in the survivor group highlighting the increased within group variability across cognitive constructs.
In summary, the current study found that in the context of similar and well-established frontal-parietal working memory network activations, adult survivors of childhood posterior fossa brain tumor also required additional and significantly greater left frontal and left parietal cortex activation in response to increased demands of working memory paradigm compared to controls. There was no evidence of compensatory recruitment or brain reorganization; instead the findings are aligned with the allocation of latent resources theory of the prefrontal lobe. Future studies may consider examining how the neural mechanisms identified may relate to cardiorespiratory fitness (i.e., VO2/FFM) (Wolfe et al., Reference Wolfe, Madan-Swain, Hunter, Reddy, Banos and Kana2013) or attention and internalizing symptoms (Robinsonet al., Reference Robinson, Pearson, Cannistraci, Anderson, Kuttesch, Wymer and Compas2014a, Reference Robinson, Pearson, Cannistraci, Anderson, Kuttesch, Wymer and Compas2014b), as these constructs have been suggested as promising lines of research in pediatric short-term survivors of childhood brain tumors. Furthermore, functional and structural connectivity studies may provide complementary information about how the neural regions identified during working memory work together following treatment for pediatric brain tumor.
Acknowledgments
We express our gratitude to the individuals and families who participated in this study and generously contributed their time and effort. Also, we acknowledge the Developmental Neuropsychology Research Team for helping with data acquisition and management, the staff at the Georgia State University / Georgia Institute of Technology Joint Center for Advanced Brain Imaging, and the Brain Tumor Foundation for helping share information about this clinical research opportunity with long-term brain tumor survivors. This research was supported by a Research Scholar Grant from the American Cancer Society to TZK (#RSGPB-CPPB-114044) and a Georgia State University Brains and Behavior Initiative Graduate Student Fellowship (S.N.). No conflicts of interest exist.