INTRODUCTION
In this article, we first estimate age-related shoulder heights of woolly mammoths (Mammuthus primigenius) from the Gravettian archaeological site Kraków Spadzista and, using these estimates along with a demographic analysis of the mammoth population and postulated causes of death, interpret the life conditions of the mammoth population. We hope to provide a realistic determination of the length of time involved in the formation of the site and the possible reasons for the bones being accumulated high on a steep-sided hill. We acknowledge that more studies are needed.
Our interpretation of the demographic history and life conditions of the Kraków Spadzista mammoths follows from fine-scale mortality profiling (Haynes and Klimowicz, Reference Haynes and Klimowicz2016). We think the mammoth mortality profile at the site is a record of important recurring events affecting the local population, taking place over a relatively limited time span while the assemblage accumulated. This interpretation, when considered in the context of archaeological, taphonomic, and paleoenvironmental analyses, is an important part of our model explaining the concentration of mammoth bones.
The shoulder height study reported here is an initial experiment in estimating mammoth stature by using two related mathematical approaches. Our objective is to assign probable ontogenetic ages to different ranges of stature, allowing an estimate of how the Kraków Spadzista mammoths compare with other multimammoth assemblages. One value of this kind of study is evident in publications by Baryshnikov et al. (Reference Baryshnikov, Kuzmina and Hrabryi1977) and Nikolskiy and Pitulko (Reference Nikolskiy and Pitulko2013), who demonstrated that meaningful mortality profiling is possible from measurements of limb elements taken from jumbled remains that are without associated dentitions, as is the case at Kraków Spadzista. Another value is that estimations of shoulder height allow comparison of geographic and temporal clines among different regional subpopulations of mammoths, ostensibly reflecting evolutionary responses to diverse climatic conditions, or the quality of local forage.
Radiometric dates are reported here exactly as published and unrounded, with one standard deviation in age, and have been calibrated using Calib 7.10 online; calibrated ages are 2σ ranges, rounded to nearest 10 yr.
BACKGROUND
The site known as Kraków Spadzista (formerly Kraków Spadzista Street) in southern Poland is ~2 ha in extent, located on the easternmost part of Sowiniec horst, a tectonic upland plateau of varying relief that has a steep drop on the north and gentler slopes to the west and south (Kozłowski et al., Reference Kozłowski, Van Vliet, Sachse-Kozłowska, Kubiak and Zakrzewska1974; Wilczyński et al., Reference Wilczyński, Wojtal and Sobczyk2012; Łanczont et al., Reference Łanczont, Mroczek, Madeyska, Komar, Holub, Żogała, Sobczyk and Wilczyński2016). The site is ~50 m above the current floodplain terraces of the Rudawa and Vistula Rivers, which are ~0.5 km and ~1+ km distant, respectively.
At the site, three zones of hunter-gatherer activity are distinct: zone I, a campsite; zone II, a lithic workshop and animal processing area; and zone III, an accumulation of woolly mammoth bones (Wilczyński et al., Reference Wilczyński, Wojtal and Sobczyk2012). This last zone is ~200 m2 in size and includes excavated trenches B + B1 + B3. Eight of 10 radiometric dates from this zone range from 24,000 ± 300 14C yr BP (28,660–27,600 cal yr BP) to 23,020 ± 180 14C yr BP (27,660–26,980 cal yr BP) (Wojtal et al., Reference Wojtal., Sobczyk and Wilczyński2015). Two younger 14C dates, 19,450 ± 120 14C yr BP (23,760–23,040 cal yr BP) and 20,600 ± 1050 14C yr BP (27,030–22,450 cal yr BP), are possibly indicative of a later occupation but may also be unreliable because the youngest date was from a carbonized bone and the next youngest was from a bone with low collagen content (Drucker et al., Reference Drucker, Rivals, Münzel and Bocherens2016).
The mammoth bones in Kraków Spadzista are buried in fine-grained sediments that overlie waterlogged and calcareous rocks, within a small karstic paleodepression (Łanczont et al. (Reference Łanczont, Madeyska, Mroczek, Hołub, Komar, Kusiak, Łącka, Żogała and Bogucki2013, Reference Łanczont, Madeyska, Mroczek, Hołub, Żogała and Bogucki2015a, Reference Łanczont, Madeyska, Mroczek, Komar, Łącka, Bogucki, Sobczyk and Wilczyński2015b, Reference Łanczont, Mroczek, Madeyska, Komar, Holub, Żogała, Sobczyk and Wilczyński2016). Most of the lithics and animal bones in the site are within a silt sediment disturbed by periglacial solifluction processes, which formed lobes and involutions (Łanczont et al., Reference Łanczont, Madeyska, Mroczek, Hołub, Żogała and Bogucki2015a, Reference Łanczont, Madeyska, Mroczek, Komar, Łącka, Bogucki, Sobczyk and Wilczyński2015b). This deposit, below a partially denuded Eemian–early glacial paleosol, corresponds to a younger part of Marine Oxygen Isotope Stage (MIS) 3 (Interplenivistulian age). The site was occupied by Gravettian hunter-gatherers in the latter part of MIS 3, probably for no more than a few hundred years (Sobczyk, Reference Sobczyk2012, cited in Łanczont et al., Reference Łanczont, Mroczek, Madeyska, Komar, Holub, Żogała, Sobczyk and Wilczyński2016).
Up to 2016, excavations in all zones of Kraków Spadzista yielded bone fragments and teeth of at least 86 woolly mammoths and a little more than three dozen other animals in eight taxa (Wilczyński et al., Reference Wilczyński, Wojtal and Sobczyk2012; Wojtal et al. Reference Wojtal., Sobczyk and Wilczyński2015; and Wojtal unpublished counts). More than 2000 lithic specimens were also found in the zone III mammoth bone accumulation, including shouldered points, backed blades, and burins, among other artifact classes. The zone III assemblage in Kraków Spadzista is considered to be a Gravettian foragers’ bone dump, near the camp and lithic workshop, but is also thought to be where the mammoths had been serially killed (and perhaps occasionally found dead) and butchered by people (Wojtal and Sobczyk, Reference Wojtal and Sobczyk2003, Reference Wojtal and Sobczyk2005; Wilczyński et al., Reference Wilczyński, Wojtal and Sobczyk2012; Kufel-Diakowska et al., Reference Kufel-Diakowska, Wilczyński, Wojtal and Sobczyk2016). Backed lithic specimens that were analyzed from the mammoth bone accumulation (Kufel-Diakowska et al., Reference Kufel-Diakowska, Wilczyński, Wojtal and Sobczyk2016) show damage and microwear indicative of use as projectile points, confirming that Gravettian people killed at least some of the mammoths. Human butchering of the mammoths is evidenced by a few cut marks on mammoth elements (Wojtal and Sobczyk, Reference Wojtal and Sobczyk2005, p. 202) and the presence of burned large bone fragments, suggesting their use as fuel while still fresh and greasy. Most burned bone fragments were not found in the zone III accumulation, but in another part of the larger site (Wilczyński et al., Reference Wilczyński, Wojtal and Sobczyk2012; Wojtal and Wilczyński, Reference Wojtal and Wilczyński2015).
Paleoenvironment at the time the Kraków Spadzista mammoth bones were deposited
Kraków Spadzista is similar to other Gravettian sites in central Europe, in that it was occupied during the last part of MIS 3, when short-term oscillations occurred in temperature. According to Musil (Reference Musil2010), it was unusual in not being abandoned just before or at the onset of the maximum cold part of the last glacial maximum (LGM), as were many major Gravettian sites such as Předmostí and Dolní Věstonice I and II, in the Czech Republic, and Willendorf and Krems-Wachtberg in Austria (Fig. 1).

Figure 1 (color online) Maps showing named sites in central Europe (top) and in Russia (bottom).
Pryor et al. (Reference Pryor, O’Connell, Wojtal, Krzemińska and Stevens2013) analyzed stable isotopes in mammoth teeth from zone III at Kraków Spadzista and concluded that the remains had accumulated in a Dansgaard-Oeschger cold event; the radiometric dates cited previously suggest this event could have been during Greenland stadial 4 or 3, which had approximate starting dates of 28.6 and 27.54 ka, respectively (Rasmussen et al., Reference Rasmussen, Bigler, Blockley, Blunier, Buchardt, Clausen and Cvijanovic2014). During and after deposition, solifluction during cold periods affected the sediments enclosing the mammoth bones, and at least one ice wedge had moved cultural materials downward, all indicative of permafrost conditions (Kalicki et al., Reference Kalicki, Kozłowski, Krzemińska, Sobczyk and Wojtal2007). Cold conditions interrupted pedogenesis, which had begun during relatively warmer periods, and also interrupted invertebrate activity in the sediments and the etching of bone surfaces by plant roots.
Łanczont et al. (Reference Łanczont, Madeyska, Mroczek, Hołub, Komar, Kusiak, Łącka, Żogała and Bogucki2013, Reference Łanczont, Madeyska, Mroczek, Hołub, Żogała and Bogucki2015a, Reference Łanczont, Madeyska, Mroczek, Komar, Łącka, Bogucki, Sobczyk and Wilczyński2015b, Reference Łanczont, Mroczek, Madeyska, Komar, Holub, Żogała, Sobczyk and Wilczyński2016) sampled pollen and sediments from the entire Kraków Spadzista site and provided relatively detailed reconstructions of sediment deposition and changing vegetational communities. Pollen and spore profiles from the site’s zones I and II have gaps in the record, but data pertaining to the time of deposition of the mammoth bones indicate dry conditions with rather poor vegetational diversity and sparse plant coverage (Łanczont et al., Reference Łanczont, Mroczek, Madeyska, Komar, Holub, Żogała, Sobczyk and Wilczyński2016). An analysis of mammalian associations from the site (Péan and Wojtal, Reference Péan and Wojtal2003) also concluded the landscape was open.
Diet of the Kraków Spadzista mammoths
Łanczont et al. (Reference Łanczont, Mroczek, Madeyska, Komar, Holub, Żogała, Sobczyk and Wilczyński2016) interpreted the Gravettian-era landscape as having “rather poor” vegetational cover, steppe-like with small patches of scattered trees, herbs, and grasses, as supported by Péan and Wojtal (Reference Péan and Wojtal2003). The grass provided most if not all of the mammoth diet. Drucker et al. (Reference Drucker, Rivals, Münzel and Bocherens2016) analyzed dental microwear and stable isotopes from a sample of Kraków Spadzista mammoth specimens and concluded that the mammoths were all grazers, with little or no other types of forage in the diet such as tree bark or fruits. Drucker et al. (Reference Drucker, Rivals, Münzel and Bocherens2016) provide proxy support for the existence of adequate grass and forb communities in open habitats with little tree cover, as is known from other parts of Pleistocene central Europe.
Abnormalities and pathologies of the Kraków Spadzista mammoths
Krzemińska and others (Krzemińska Reference Krzemińska2008, Reference Krzemińska2014; Krzemińska and Wojtal, Reference Krzemińska and Wojtal2014; Krzemińska and Wędzicha, Reference Krzemińska and Wędzicha2015; Krzemińska et al., Reference Krzemińska, Wojtal and Oliva2015) described and illustrated abnormalities in Kraków Spadzista mammoth bones, as did Leshchinskiy (Reference Leshchinskiy2012). Krzemińska (Reference Krzemińska2014) thought it probable that metabolic problems account for the pathological conditions in the mammoth bone assemblage, suggestive of calcium and phosphate disorders resulting from lengthy periods of malnutrition and insufficient intake of minerals and vitamins. Leshchinskiy (Reference Leshchinskiy2012, p. 156) also thought the “destructive changes” seen on >50% of the 11,000 mammoth remains he examined were attributable to “metabolic imbalance presumably originating from the mineral deficiency.” Niven and Wojtal (Reference Niven and Wojtal2002) speculated that “furrows” on mammoth tooth crowns were perhaps another pathology that could be a sign of widespread dental hypoplasia in the population, resulting from mineral imbalance; the furrows were not caused by variations in diet, because mammoths both with and without dental furrowing had the same grazing diet (Drucker et al., Reference Drucker, Rivals, Münzel and Bocherens2016).
Taxonomic analysis of the Kraków Spadzista bone assemblage
It is likely that the mammoths had been dismembered directly at the site (Wojtal, Reference Wojtal2007) and their bones discarded there, because hundreds of smaller bones such as hyoids, sesamoids, phalanges, and caudal vertebrae are present, elements that are not meaty, hardly useful as raw materials, and unlikely to have been transported to the site from kills elsewhere.
A small number of bones were also identified from eight mammalian taxa other than M. primigenius (Table 1) in Kraków Spadzista zone III. Some of them were possibly dumped in zone III when Gravettian foragers cleaned their camp (zone I). Those bits of animals may have come from hunted individuals whose other bones were discarded elsewhere. The few bones of carnivores may have come from individuals that had been scavenging mammoth remains and were killed on-site by humans or other carnivores.
Table 1 Identifiable nonmammoth number of identified specimens (NISPs) and minimal number of individuals (MNIs) from zone III of Kraków Spadzista, and (in parentheses) the combined totals from all three zones of Kraków Spadzista.

Age distribution of Kraków Spadzista mammoths based on mandibular dentition
The mammoth mandibles and teeth recovered at the site show the typical growth characteristics of proboscideans, in which worn teeth are replaced by progressively larger teeth erupting from behind in the jaw. Ontogenetic ages are usually assigned to mammoth dentitions by comparative study of dental wear stages in Asian or African elephants. The Kraków Spadzista mammoth age distribution has been analyzed more than once, but all results are in general agreement; Kubiak and Zakrzewska (Reference Kubiak and Zakrzewska1974), Lipecki and Wojtal (Reference Lipecki and Wojtal1996), Wojtal (Reference Wojtal2001, Reference Wojtal2007), and Wojtal and Sobczyk (Reference Wojtal and Sobczyk2005) concluded that the mortality profile appears catastrophic, or nonselective, in that the proportions of age classes are generally what would be expected in a living population.
Haynes and Klimowicz (Reference Haynes and Klimowicz2016) suggested the age distribution of the death assemblage reflects serial depletion of some cohorts over a span of 50–100 yr. Less than 10% of mammoth individuals in the assemblage (Fig. 2) are >30 AEY (African [elephant] equivalent years; see the “Mortality profiling of Kraków Spadzista mammoths” section for explanation), which is the period of life when distal and proximal long bone epiphyses in females of extant elephants and woolly mammoths will have fused to limb bone diaphyses. Epiphyses in males will not all be fused until individuals are >45 AEY old (Deraniyagala, Reference Deraniyagala1955, table VI; Lister, Reference Lister1999, table 2; Haynes, Reference Haynes2017, fig. 1). The Kraków Spadzista assemblage contains very few limb elements with fused epiphyses.

Figure 2 (color online) Age distribution of mammoths from Kraków Spadzista zone III. This graph depicts the data from 82 mandibles but does not include the isolated teeth, which cannot be matched to these mandibles; continuing research on the teeth will probably increase the minimal number of individuals to well over 100. AEY, African (elephant) equivalent years.
MATERIALS AND METHODS
The excavated materials from more than 40 yr of research in all parts of the Kraków Spadzista site are curated at the Institute of Systematics and Evolution of Animals, Polish Academy of Sciences (Kraków, Poland). We examined and measured bone and teeth specimens for several different purposes, as described subsequently. In the subsections that follow, we describe our different data sources and what we looked for in the data. In the “Results” section, we report our original findings, and in the “Discussion,” we consider these and others’ results and speculate about possible meaning.
Mortality profiling of Kraków Spadzista mammoths
Mammoths are often assigned ontogenetic ages in AEY, widely misdeciphered as “African elephant years.” The initials AEY stand for “African-equivalent years,” an analytic tool introduced by vertebrate paleontologist J. Saunders (Reference Saunders1977), who meant for the term to denote ages assignable to M. columbi in cases where the stages of dental wear and progression were similar to those identified in Loxodonta africana, the African elephant (Laws, Reference Laws1966). In this article, we use AEY as a convenience for assigning ontogenetic ages to M. primigenius based on placement in the Laws system that correlates L. africana ontogenetic ages and dental wear, as revised by Stansfield (Reference Stansfield2015).
The most recent mortality profile from Kraków Spadzista (Haynes and Klimowicz, Reference Haynes and Klimowicz2016) is graphically presented in Figure 2. This graph shows percentages of individuals analytically identified in age groups defined by Laws (Reference Laws1966), along with the estimated ontogenetic age ranges in the groups, extrapolated from revisions to the Laws system (Stansfield, Reference Stansfield2015).
Shoulder height estimates of Kraków Spadzista mammoths
In 2015, we measured identified limb elements from Kraków Spadzista, with an aim to reconstruct shoulder heights and compare these with the reconstructed statures in other multimammoth assemblages. Our measurements are reported here in centimeters, rounded to one decimal place after all calculations were completed. We compared our measurements and estimates of shoulder heights with the data from other sites (discussed in the “Results”).
It is impossible to assemble a skeleton or even a complete limb of a fully grown individual in the Kraków Spadzista sample, because bones are so incomplete or the diaphyses had been separated from unfused epiphyses. Tibia diaphyses are the most complete of the long bones; tibia diaphyses without fused epiphyses, which come from animals under ~30 AEY, represent ~91% (n = 20) of the total number of tibias with measurable diaphyses (n = 22). A similar proportion of individuals <30 AEY (93%) is also represented in analyzed mammoth mandibles and dentitions, as seen in Figure 2 (Haynes and Klimowicz, Reference Haynes and Klimowicz2016). The similarity might mean that the high proportion of unidentifiable bone specimens in the analyzed sample (n = 16,967 vs. the identifiable 6414) has not introduced serious bias when it comes to reconstructing proportions of different age-related body sizes.
Lister and Stuart (Reference Lister and Stuart2010) estimated shoulder height by applying regression equations derived from measurements of mounted skeletons, which probably smooths variable growth curves for different individuals. We tried this approach, but before we could use the regression equations, we had to estimate greatest bone lengths for the specimens that did not have epiphyses in place, done by using measured average relationships between midshaft circumferences (MCs), diaphysis lengths (DLs), and greatest lengths of complete elements with both epiphyses in place. We call this approach to estimating skeletal shoulder height (SSH) a “ratio + regression” method; results of this method are designated SSH-1.
Different researchers have speculated that live shoulder height (LSH) exceeds SSH in proboscideans by ~5–7% (Lister and Stuart, Reference Lister and Stuart2010), because of the added soft tissue over the bones. In our calculations, we used a value of 6% and multiplied SSH-1 by 1.06 to project the LSH-1. The definition of LSH is the straight-leg height when standing, measured on one side of the body from forefoot bottom to the skin directly covering the upper extent of the vertebral border of the scapula, which may be ossified cartilage. The ridge formed by the skin and soft tissue crossing between the uppermost borders of both scapulas in quadrupeds is called the withers, and is often the highest point on the back, posterior to the neck. In mounted mammoth skeletons, the highest point on the back is sometimes artificially arranged to be the dorsal ends of spinous processes of several thoracic vertebrae, but we think these examples of mounts have been stretched upward in the mounting to make the skeletons taller (also suggested by Larramendi, Reference Larramendi2016).
Our second approach to estimating stature relied exclusively on ratios. Lister and Stuart (Reference Lister and Stuart2010, p. 184) observed that some researchers “assume a percentage contribution of each long bone length to shoulder height, and use it to estimate shoulder heights from isolated bones.” This practice is simple and seems to be fairly reliable. Deraniyagala (1941, cited in Deraniyagala, Reference Deraniyagala1955, p. 58) did this sort of calculation for living Elephas maximus, and found LSH = 2.65 times the length of the femur. Osborn (Reference Osborn1907) published measurements from mounted Mammuthus spp. skeletons; the SSH of a M. columbi was 2.56 times the length of a femur, and the SSH of a M. meridionalis was 2.82 times the length of the femur. The lengths of forelimb and hind-limb elements on average certainly do appear to be consistent percentages of skeletal height for different mammoth species (Garutt, Reference Garutt1964). Larramendi (Reference Larramendi2016, table 3) calculated that the tibia’s greatest length on average is 23.2% of M. primigenius SSH, the femur’s greatest length on average is 40.98% of SSH, and the ulna’s articular length (AL) on average is 25.64% of SSH.
We had no associated skeletons and only incomplete limb elements from Kraków Spadzista to work with, so we had to estimate greatest bone lengths by comparing diaphysis circumference versus DL, and DL versus greatest length (when both epiphyses are in place), before projecting SSH. This “ratio-only” method to estimate stature first produced SSH-2, which was then multiplied by 1.06 to project the in-flesh stature, designated LSH-2. We note that both our methods make use of ratio-derived calculations from limited samples, and the two methods are not fully independent.
Because of the small sample of complete measurable bones and the variability in bone sizes and growth parameters in proboscideans (Lister and Stuart, Reference Lister and Stuart2010; Larramendi, Reference Larramendi2016), we do not claim that either the ratio-only or the ratio + regression method for prediction is more accurate.
In Lister and Stuart (Reference Lister and Stuart2010), the radius is said to be the most reliable bone for predicting SSH, but no complete radius (diaphysis with both fused epiphyses) has been found at Kraków Spadzista. We could not evaluate how the features of any still-growing radius compared with the necessary measurements of lengths and circumferences for this element, and therefore we did not attempt to estimate shoulder height from the radius.
We used measurements from Kraków Spadzista ulnas, femurs, and tibias for estimating stature. Supplementary Tables A1, A2, and A3 show all measurements.
Ulnas
At least one ulna in the assemblage may have been fully grown with both epiphyses fused before fragmentation, but it is missing its entire distal end; however, the proximal end of the radius has fused to the ulna’s proximal end, which is related to advanced age (Harington et al., Reference Harington, Mol and van der Plicht2012, fig. 7), and may occur around the same time the distal epiphyses of radius and ulna fuse to the diaphyses. In L. africana, the proximal ends of radius and ulna fuse together and the distal epiphyses of both elements fuse to the diaphyses late in life, probably a decade or more after 30 AEY, which is a clue that the fused proximal radius-ulna from Kraków Spadzista belonged to an old female (>45 AEY?), supported by its modest size.
Lister and Stuart (Reference Lister and Stuart2010) did not calculate a regression equation for mammoth ulnas, because published measurements are “problematic” and apparently inconsistent in how they were taken. We measured MCs of diaphyses and greatest widths of complete proximal articulating surfaces of 28 Kraków Spadzista ulnas (Figure 3). We also measured the DL (= distance between proximal articular surface [measured on lateral margin] and distal metaphysis) of 12 ulnas with these features (Supplementary Table A2), to compare with MC (measured with a cloth tape).

Figure 3 (color online) A Kraków Spadzista ulna from a still-growing mammoth, showing where measurements were made of maximum width of proximal articular surface, diaphysis length, and midshaft circumference of diaphysis; note this last measurement was taken from the proximal articular surface to the distal end of the diaphysis without epiphysis and is not equal to the smallest circumference of diaphysis in von den Driesch (Reference von den Driesch1976).
We found stable linear relationships between MC of the ulna and the maximum width of the proximal articular surface (Figure 4), and between MC and DL. Note that DL as used here for the ulna does not include the olecranon process and its epiphysis. These stable relationships imply that linear relationships also exist among other growth variables, such as between DL and AL, which is the distance from proximal articular surface to the distal end of the attached distal epiphysis (Larramendi, Reference Larramendi2016).

Figure 4 (color online) Relationship between midshaft circumference and maximum width of the proximal articular surface of measurable ulnas from Kraków Spadzista.
The ratios used in these calculations are tentative. The AL of the ulna is proposed to be ~1.18 × DL, an average based on estimated measurements of two nearly complete Kraków Spadzista ulnas (F12/13-2096 and 2329) (Supplementary Table A1), actual measurements of a Polish unprovenienced and unnumbered specimen (large and presumably male) in the Institute of Systematics and Evolution of Animals, Polish Academy of Sciences (Kraków), and measurements made by GH in 1987 of a grab sample of five ulnas (numbers missing or unreadable, four being relatively small and presumably female) in a collection from Berelëkh (arctic Siberia, Russia) at the Zoological Institute, USSR (now Russian) Academy of Sciences, Leningrad (now St. Petersburg). The SSH-2 is calculated by multiplying AL by 3.9 (Larramendi, Reference Larramendi2016, table 3).
Femurs
Many femoral diaphyses from Kraków Spadzista are incomplete (Figure 5), but midshafts are mostly well preserved.

Figure 5 (color online) Selection of femur diaphyses from Kraków Spadzista. Specimen on the far left is fetal. None had fused epiphyses.
We measured MC of the more complete femurs (which is not the same measurement as the smallest circumference of the diaphysis, “CD,” in von den Driesch [Reference von den Driesch1976]; Supplementary Table A2). To predict DL of individuals, we multiplied the MC by a coefficient that increased at successive stages of growth. The coefficients vary for this element because its allometric scaling with growth seems greater than what was noted with the other elements in our study. The coefficients were either derived from measurements of specimens from individuals of known/estimated ontogenetic ages at different stages of growth or extrapolated from tables and/or photographs with scale bars of those specimens (Baryshnikov et al., Reference Baryshnikov, Kuzmina and Hrabryi1977, fig. 8; Dubrovo, Reference Dubrovo1982, table 11 and plate 6; Maschenko, Reference Maschenko2002, table 23 and p. 109, photos 23 and 24; Maschenko et al., Reference Maschenko, Gablina, Tesakov and Simakova2006), as well as from measurements made by PW of a small sample from the Gravettian Czech Republic site Milovice I (specimens 48196, 48263, and 69745). The coefficients are 2 to 2.2 for specimens with MC up to 13 cm, 2.3 to 2.39 for specimens >13 and <18 cm, 2.4 for specimens of 18 to <19.6 cm, and 2.5 for larger specimens. The greatest length (GL = length of complete diaphysis with attached proximal and distal epiphyses) was estimated by multiplying DL by 1.23, an average coefficient derived partly from measurements taken in 1987 of complete specimens from several sites stored at the Zoological Institute of the USSR (now Russian) Academy of Sciences in Leningrad (now St. Petersburg) (Haynes, Reference Haynes1991, p. 26, table 2.3B) and partly extrapolated from photographs of specimens from other sites (Baryshnikov et al., Reference Baryshnikov, Kuzmina and Hrabryi1977, fig. 3; Maschenko, Reference Maschenko2002, photo 24). To estimate SSH, GL was multiplied by 2.44, an average coefficient derived from measurements of complete femurs in 19 mammoth skeletons (Larramendi, Reference Larramendi2016, fig. 6 and table 3).
Tibias
Two complete tibias with fully fused proximal and distal epiphyses are present in the Kraków Spadzista assemblage (small and probably female, specimens E11 1897 and I1 040 in Supplementary Table A3), allowing us to measure the contribution that the diaphyses made to their GL. Another set of measurements was taken on a complete but unprovenienced and unnumbered tibia from Poland, stored in the Institute of Systematics and Evolution of Animals, Polish Academy of Sciences (Kraków). Parts of suture lines were still visible where fusion had occurred on these specimens. The GL of the tibia averaged 1.16 × DL. Estimating the ratio of the DL to GL was needed in order to project the SSH for specimens lacking epiphyses. We found that in the sample of the still-growing tibias without epiphyses (Figure 6), a fairly consistent relationship exists between MC and DL (Figure 7), giving us more confidence that the ratio between tibia GL and DL is also reasonably consistent for all ages. We point out that Lister and Stuart (Reference Lister and Stuart2010, p. 185) consider the tibia one of the least reliable long bones in its contribution to shoulder heights, compared with other elements: “Tibia length poorly reflects continuing size increase of the animal” due to its early completion of growth, according to Lister (Reference Lister1999), “while radius keeps up with [growth in height] the longest” and does not complete linear growth until relatively late in life, at least in male Mammuthus. This caution about use of tibias applies to both males and females; in the case of males, statural growth continues for decades after the tibias have completed growth, unlike in females, whose statural growth ends much sooner after their tibias reach maximum lengths (Haynes, Reference Haynes2017, fig. 1). One possible implication is that the relationship of tibia GL to LSH in females is different than it is in males; therefore, using a ratio derived from measurements of mostly older adult males will incorrectly estimate shoulder heights for adult females.

Figure 6 (color online) Examples of Kraków Spadzista tibias without epiphyses (all of which were unfused), showing where diaphysis length was measured. The far left specimen on the bottom row is fetal.

Figure 7 (color online) Relationship between Kraków Spadzista tibia midshaft circumference and diaphysis length.
RESULTS
Apparent trends in body size
The LSH-1 and LSH-2 values based on the two largest Kraków Spadzista femurs are within ~2% of each other: 267.2 and 264.2 cm for LSH-1s, and 260.9 and 258.5 cm for LSH-2s (Supplementary Table A1). The LSH-1 and LSH-2 values based on the two Kraków Spadzista tibias with fused epiphyses are not so similar, about 10–11% different: 254.9 and 246.7 cm for LSH-1, and 230.7 and 221.2 cm for LSH-2. We tentatively suggest the LSH-2 values based on ratios are likely to be underestimates.
The MCs of the two complete and fully fused Kraków Spadzista tibias (24.3 and 25.4 cm) are modest, which suggests they were from females, when compared with a much larger unprovenienced adult mammoth tibia (probably male) from Poland (MC = 31 cm) and measurements of tibias from southwestern Russia (Baigusheva Reference Baigusheva1980, p. 76) from which circumferences were extrapolated from reported diaphysis widths of bones deemed to be from males (range = 33.3–36.0 cm). The shoulder heights based on bone measurements that we present here may be averages, minima, or maxima that Kraków Spadzista female mammoths reached in life.
Table 2 shows results of our extrapolations of LSH-1 and LSH-2 using mostly incomplete ulnas, femurs, and tibias. A value for LSH-1 could not be calculated from ulna measurements, because there is no regression equation to apply; however, the ratio-only method applied to the largest Kraków Spadzista ulna produces an estimated LSH-2 of 256.1 cm, which is similar to estimates based on largest femurs. The LSH values are admittedly rough estimates because of natural variability in mammoth growth rates, as well as variability in ratios of limb bone lengths to stature, and (importantly) the incomplete nature of the bones available in the Kraków Spadzista sample. The two fully grown tibias and the two largest ulnas are thought to be from adult females, while the largest still-growing tibias are thought to be from young adult males 18–30 AEY in age.
Table 2 Estimates of maximum live shoulder heights (LSHs) of adult mammoths from the Kraków Spadzista sample, using two methods. Note the large estimates from the still-growing tibias with unfused epiphyses, which are probably from males 18–30 African (elephant) equivalent years.

Figure 8a graphically shows our postulated LSHs for a sample of mammoths from various sites, which are discussed subsequently with references, arranged in proposed or known age groups that increase from left to right. Figure 8b shows a sample of our postulated correlations of LSHs and age groups in the Kraków Spadzista assemblage. We note again that some ranges of shoulder heights are based on small samples.
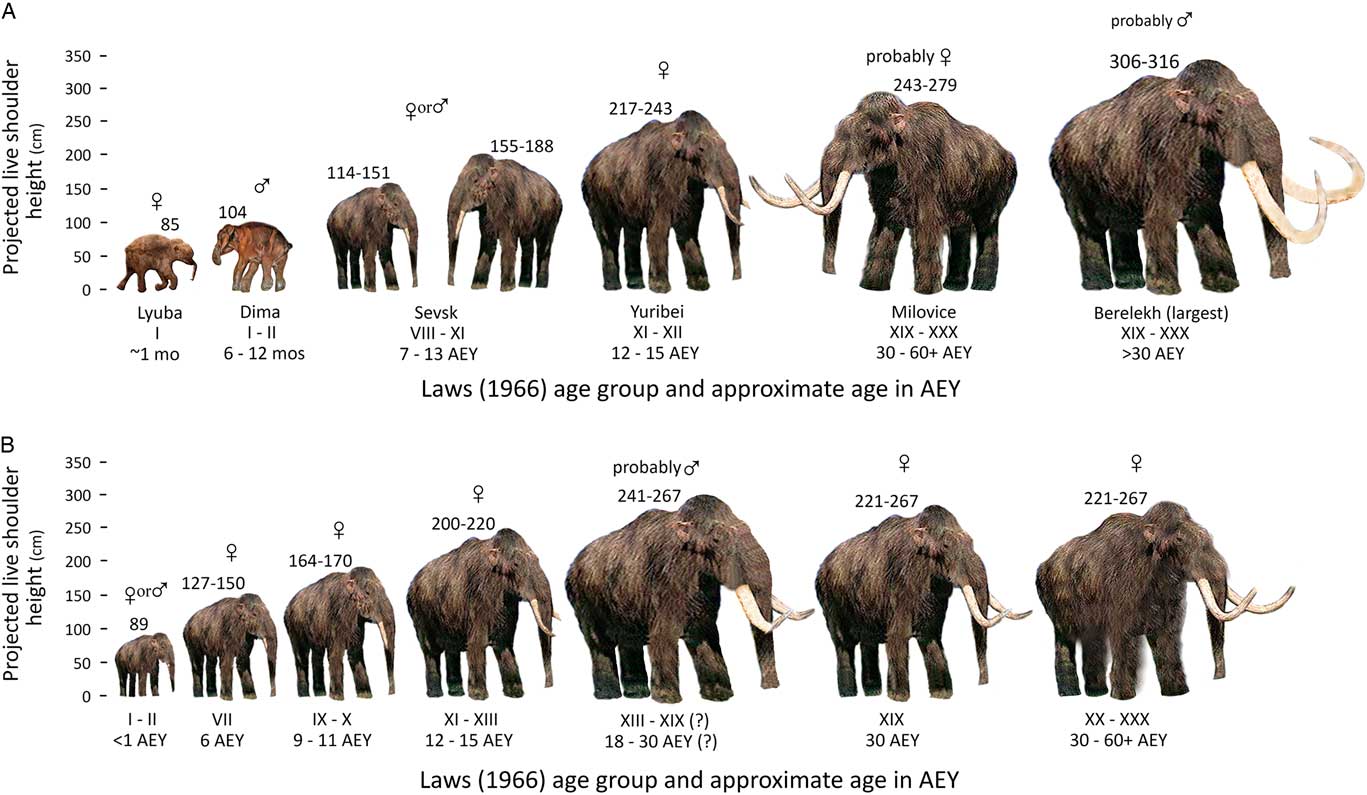
Figure 8 (color online) Postulated shoulder heights for Mammuthus primigenius: (A) from different parts of Eurasia and (B) from Kraków Spadzista. The ranges reflect the differences in the estimates, which were made using two different methods and in some cases different bone elements. AEY, African (elephant) equivalent years.
Table 3 lists the localities used in the calculations of possible LSHs. Kraków Spadzista is located at ~50°N latitude. The following individuals in Figure 8a (see Fig. 1 for the location of sites) are from ~6000 km east and north of Kraków Spadzista, in arctic Siberia: Lyuba, a calf carcass from the Yamal Peninsula, found at ~70°N latitude and dated 41,910 +550/−450 14C yr BP (~44,380–46,160 cal yr BP); Dima, a male calf carcass also known as the Kirgilyakh or Magadan mammoth (Shilo et al., Reference Shilo, Lozhkin, Titov and Shumilov1983), found at ~62°N latitude and dating probably to ~41,000 ± 1100 14C yr BP (~42,700–46,340 cal yr BP) (Lozhkin and Anderson, Reference Lozhkin and Anderson2016); a female mammoth carcass found at Yuribei, ~70°N latitude (Sokolov, Reference Sokolov1982), dating to the beginning of the Boreal period ~10 ka (Ukraintseva, Reference Ukraintseva2013); and a male mammoth from the Berelëkh mass bone assemblage, found at ~70°N latitude (Baryshnikov et al., Reference Baryshnikov, Kuzmina and Hrabryi1977; Vereshchagin, Reference Vereshchagin1977), with numerous 14C dates; 13,700 ± 80 14C yr BP (16,860–16,260 cal yr BP) is favored by Lozhkin and Anderson (Reference Lozhkin and Anderson2018) for most of the mammoth remains.
Table 3 Sites with element measurements that were used in Figure 8 to estimate live shoulder height by age group, arranged from geologically oldest to youngest. MNI, minimal number of individuals.

Figure 8a also includes the following mammoths from nearer Kraków Spadzista in central and eastern Europe (see Fig. 1): two juvenile mammoths from Sevsk in Russia (Maschenko, Reference Maschenko2002), located at ~52°N latitude, ~1000 km east–northeast of Kraków Spadzista, with a bone date of 13,950 ± 70 14C yr BP (17,180–16,600 cal yr BP) and a tusk date of 13,680 ± 60 14C yr BP (16,780–16,270 cal yr BP); and five adult mammoths (probably female) from Milovice I (Oliva, Reference Oliva2009), located at ~50°N latitude in Czech Republic, about 500 km southwest of Kraków Spadzista, with 10 radiometric dates on Gravettian charcoal and bones, ranging from 25,900 +510/−480 14C yr BP (30,980–29,000 cal yr BP) to 17,500 ± 1100 14C yr BP (23,700–18,600 cal yr BP). Five of the dates are ~25,000–24,000 14C yr BP, suggesting that most material at the site is a millennium older than the age of the mammoth bone accumulation at Kraków Spadzista.
LSH values for the specimens in Figure 8a were calculated using various methods, depending on the data available. Lyuba was measured on a photograph with a scale bar in Rountrey et al. (Reference Rountrey, Fisher, Tikhonov, Kosintsev, Lazarev, Boeskorov and Buigues2012), and also checked against published media reports. Dima’s height has been variously reported in media releases as 90 cm and 104 cm tall (our extrapolated LSH from photographs of the carcass with scale bar is about 104 cm). The range of shoulder heights of the two Sevsk specimens was extrapolated using Lister and Stuart’s (Reference Lister and Stuart2010) regression equations and the ratio-only method applied to the smallest and largest tibia GL measurements assigned to age group III (7–13 yr in Maschenko, Reference Maschenko2002, table 23); the Yuribei mammoth’s possible height was calculated from the GL of one tibia, using the two methods. The range of shoulder heights for Milovice mammoths was calculated from measurements taken of five complete tibias, using the two methods. The Berelëkh mammoth’s height was calculated using the two methods applied to the GL of the largest femur, which was extrapolated as 113 cm from a graph in Baryshnikov et al. (Reference Baryshnikov, Kuzmina and Hrabryi1977, fig. 8), in agreement with Maschenko (Reference Maschenko2002, table 23), and fitted to the regression in Lister and Stuart (Reference Lister and Stuart2010, table 16), producing an estimated LSH-1 of 306.4 cm; a second calculation was made by extrapolating GL of one tibia from a photograph having a scale bar (Baryshnikov et al., Reference Baryshnikov, Kuzmina and Hrabryi1977, fig. 4), appearing to be 65.6 cm long; this number was then fitted to the tibia regression in Lister and Stuart (Reference Lister and Stuart2010, table 16), producing an LSH-1 of 316.4 cm. We note that this tibia’s extrapolated GL was greater than the GL of any specimen on a graph in Baryshnikov et al. (Reference Baryshnikov, Kuzmina and Hrabryi1977), which is a plot of tibia length against proximal epiphysis width, on which ~57.0 cm is the maximum GL, a number also cited by Maschenko (Reference Maschenko2002, table 23).
Problems with calculating shoulder heights
Laws et al. (Reference Laws, Parker and Johnstone1970) using a sample of 793 L. africana specimens from Murchison Falls Park South in Uganda calculated three different growth equations, because of different rates and time spans of growth. One equation was for males under 20 yr old, another for males over 21 yr old, and the third for females 0–60 yr old. Shrader et al. (Reference Shrader, Ferreira, McElveen, Lee, Moss and van Aarde2006) measured shoulder heights of known-age African elephants and found that growth curves were indistinguishable for males and females up until ~9 yr of age, beyond which growth rates diverged. Similar observations have been made of male and female growth in Asian elephants (McKay, Reference McKay1973; see Van Valkenburgh et al., Reference Van Valkenburgh, Hayward, Ripple, Meloro and Roth2016, fig. S2). Shrader et al. (Reference Shrader, Ferreira, McElveen, Lee, Moss and van Aarde2006) also discovered that age-specific shoulder heights were comparable in the first part of the life span for elephants in widely separated and isolated African populations.
Different growth curves are needed for calculating accurate shoulder heights of male and female M. primigenius, although only one set of regression equations for specific skeletal elements is currently available (Lister and Stuart, Reference Lister and Stuart2010, table 16). These equations have been applied in studies of subadult and adult woolly mammoths (e.g., Nikolskiy and Pitulko, Reference Nikolskiy and Pitulko2013). The regression parameters in Lister and Stuart (Reference Lister and Stuart2010) differ, of course, for specific limb elements and were derived from measurements of mounted skeletons and individual long bones of Eurasian Mammuthus spp. Besides M. trogontherii and M. meridionalis in the measured sample, the M. primigenius individuals that were measured in Lister and Stuart (Reference Lister and Stuart2010) include 15 males of >30 AEY, 1 male of 28 AEY, 3 males of unknown AEY, 2 females of 40 and 60 AEY, 1 female of 12 AEY, and 1 female of unknown AEY.
The available regression equations are derived from a sample heavily weighted toward adults, mainly older males. Some results of applying the regression equations either greatly overestimate or underestimate shoulder heights for the youngest animals. For example, the LSH-1 projected from a fetal tibia at Kraków Spadzista is 112.8 cm, which is ~33% larger than the stature extrapolated from photographs and published reports of the frozen newborn M. primigenius calf Lyuba (85 cm) (Rountrey et al., Reference Rountrey, Fisher, Tikhonov, Kosintsev, Lazarev, Boeskorov and Buigues2012). The LSH-1 of the Kraków Spadzista tibia is unrealistic for a fetal mammoth. Keeping in mind that there may be expectable differences between far northern and a temperate zone mammoth calves—Lyuba lived in the High Arctic and died ~45,000 cal BP, whereas the Kraków Spadzista fetal individual came from a temperate midlatitude site and dates later, to the LGM (as defined by Hughes and Gibbard, Reference Hughes and Gibbard2015)—the regression method does not produce an acceptable estimate. Our alternative ratio-only method produces an LSH-2 estimate of 71.5 cm for the fetal Kraków Spadzista individual, which is reasonable in light of the size of 1-month-old Lyuba. This LSH-2 estimate for a Kraków Spadzista fetal mammoth is also in close agreement with the LSH-2 estimate (70.7 cm) based on the smallest Kraków Spadzista ulna.
We do note that the bones of very young individuals may have significantly different proportions from those of adults. More research is needed to confirm if our methods are reliable when applied to mammoth calves, juveniles, and adolescents, whose epiphyses may not be the same proportions of total lengths as those of fully grown adults.
An example of how the regression equations might underestimate stature of young animals is seen with a Kraków Spadzista fetal femur; the regression equation produces an LSH-1 of 26.7 cm; this is almost the same value as the probable GL of the femur (26.3 cm). This result is clearly unrealistic. Our alternative approach using ratios to estimate LSH-2 from this bone is more reasonable—the projected LSH-2 is 68.1 cm, similar to the LSH-2 (71.5 cm) that was projected by applying the ratio-only method to the fetal tibia. We think these examples should lead to caution about applying the regression equations to juvenile mammoths.
The regressions did produce realistic LSH-1 values from two complete and fully fused Kraków Spadzista tibias (246.7 and 254.9 cm), which agree well with the LSH-2 estimate from the largest ulna (256.1 cm). These values are 10–12% greater than the tibia-based projected LSH-2 values using the alternative ratio-only method (221.2 and 230.7 cm).
DISCUSSION
We begin with an extended consideration of shoulder height estimates. Our attempts to reconstruct statures are based on incomplete and dissociated bones, without any way to control for age or sex; therefore, we present these results as tentative estimates.
The estimated LSHs of Kraków Spadzista adult female mammoths are relatively small compared with estimates from Milovice I
A comparison of the mammoths from Kraków Spadzista and Milovice I (Oliva, Reference Oliva1988, Reference Oliva2009; Péan and Patou-Mathis, Reference Péan and Patou-Mathis2003; Svoboda et al., Reference Svoboda, Péan and Wojtal2005; Brugère and Fontana, Reference Brugère and Fontana2009; Brugère et al., Reference Brugère, Fontana and Oliva2009) is appropriate, because both archaeological sites are similar. Both had Gravettian lithics buried in loess, are relatively close in age compared with the older Siberian mammoths (Fig. 1), and their sediments contain evidence of cold conditions after deposition of the bones, such as ice wedges. The published mammoth minimal number of individuals (MNI) at Milovice I is 51 (Brugère and Fontana, Reference Brugère and Fontana2009, fig. 10); the published mammoth MNI at Kraków Spadzista is 86 (Wojtal and Sobczyk, Reference Wojtal and Sobczyk2005), although this number may increase as research continues. All parts of the mammoth skeleton were present at both sites, including elements from newborn and fetal animals, and the assemblages have few cut-marked mammoth bones. However, there are important differences. In Milovice I, the mortality profile of one of the site’s 14 areas has been published as greatly dominated by juveniles and subadults (Péan and Patou-Mathis, Reference Péan and Patou-Mathis2003; Svoboda et al., Reference Svoboda, Péan and Wojtal2005), as at Kraków Spadzista, but a more recent analysis of the mandibles and lower teeth from this area and all others in Milovice I indicates a very selective mortality profile dominated by prime adults (Haynes, G., Klimowicz, J., Wojtal, P., Wilczyński, J., unpublished data). Each of the 14 designated areas of Milovice I was interpreted as a separate occupation, and not as part of a single contiguous accumulation (Brugère and Fontana, Reference Brugère and Fontana2009; Brugère et al., Reference Brugère, Fontana and Oliva2009), based on the impossibility of refitting bone fragments. Even so, the relatively limited extent of all the areas together makes us wonder if the site is indeed a single entity, like Kraków Spadzista.
Another difference is the proportion of identified bones with carnivore tooth marks, which was reported as very low at Milovice I (0.1%) compared with at Kraków Spadzista (~6%) (Wojtal and Sobczyk, Reference Wojtal and Sobczyk2005; Brugère and Fontana, Reference Brugère and Fontana2009; Brugère et al., Reference Brugère, Fontana and Oliva2009).
The mammoths at Milovice appear to be larger than those at Kraków Spadzista. Five fully fused tibias from Milovice I were measured to produce LSH-1 estimates of 265.9 to 279.3 cm. We acknowledge that tibias are not as reliable as other long bones for estimating stature, because they end their growth earlier in life than other limb elements, and therefore may underestimate stature. Adult stature probably varied over time and space, but it appears that woolly mammoth male LSH in the late Pleistocene probably fell in the range of 290–315 cm, and probably larger in Europe (Garutt, Reference Garutt1964; Lister and Stuart, Reference Lister and Stuart2010, supplementary table B; Larramendi, Reference Larramendi2016). The Milovice LSH-1 estimates based on complete tibias are ~4–13% smaller than the expected full-growth stature of an adult male; the alternative ratio-only method to estimate LSH-2 from the five complete Milovice I tibias produced values (243.0 to 258.1 cm) that are ~16–23% less than the average expected stature of a full-grown adult male, here considered as ~300 cm for convenience. These substantial differences between the Milovice estimates and expected male stature lead us to propose the Milovice tibias are from adult females. Notably, Milovice I LSH-1 and LSH-2 estimates based on five complete tibias are as much as 13% larger than the Kraków Spadzista shoulder height estimates based on two complete tibias (Supplementary Table A2). We suggest the Milovice I and Kraków Spadzista mammoth populations were not comparable in body size for their geographic location in central Europe, perhaps correlating to the 1000 yr age difference. We acknowledge that both site samples are small, and further study is necessary.
Bone measurements and estimated shoulder heights indicate that postadolescent male mammoths are present in the Kraków Spadzista assemblage
Note in Supplementary Table A1 that two Kraków Spadzista incomplete femurs with the largest measured MC produced projected LSH-1 values of 264.2 and 267.2 cm, which are similar to LSH-2 values (258.5 and 260.9 cm). We think these femurs were from still-growing males, because the epiphyses probably had not yet fused to diaphyses. In spite of the small differences in estimated LSH using the two different approaches, the projected shoulder heights based on these femurs are acceptable for still-growing young adult males (M. primigenius) that were no more than ~30 AEY in age. Male proboscideans continue growing in stature at least one and probably two more decades after this age (Haynes, Reference Haynes2017, fig. 1) and reach much larger statures.
We doubt that mammoth males >30 AEY are present in the Kraków Spadzista assemblage, because the estimated LSH values based on the still-growing femurs with largest MC and unfused epiphyses are ~8–17% smaller than the expected shoulder height of an average fully grown adult male M. primigenius.
Also note in Supplementary Table A2 that the LSH-2 values projected for the two largest Kraków Spadzista tibia diaphyses lacking epiphyses exceed the LSH-2 values projected from the two fully fused tibia specimens. In other words, at least two tibias were still growing but were already larger in MC than the two fully grown tibias. We propose that these still-growing specimens are also from young adult males under ~30 AEY, or alternatively from large adolescent males 15–18 AEY that were still with their family herd(s).
Figure 9 shows probable sexual dimorphism and female size variability in tibias of L. africana and M. primigenius. Specimens a and b are from African elephants; specimen c is from Poland, but the provenience is unknown; specimens d and e are from Kraków Spadzista mammoths; also shown are tibias from three Berelëkh mammoths. The projected LSH-1 values for the two Kraków Spadzista specimens (Supplementary Table A2) are 254.9 and 246.7 cm, and for the larger unprovenienced Polish specimen, the value is 297.6, which is ~17–21% larger, strongly suggesting it is from an adult male >35–40 AEY. The smaller Kraków Spadzista examples are from adult females of different statures. Halámková (Reference Halámková2009, figs. 4–8) measured distal limb elements (magnum, hamatum, scaphoid, cuneiform, and ectocuneiform) from Kraków Spadzista and Milovice I and also suggested a small number of possible postadolescent male mammoths are represented, as well as some large but probably immature males and mature females, in agreement with our conclusions.

Figure 9 (color online) Tibias of Loxodonta africana and Mammuthus primigenius at approximately the same scale, showing probable sexual dimorphism and female size variability. The L. africana tibias from Zimbabwe and the M. primigenius tibias from Poland were photographed by GH; the image of the M. primigenius tibias from Berelëkh was digitally scanned and enlarged from a small figure in Baryshnikov et al. (Reference Baryshnikov, Kuzmina and Hrabryi1977). Specimens a and b are in cranial view, and specimens c, d, and e are in lateral view; specimen c has unknown provenience but is probably from a male mammoth; specimens d and e are from Kraków Spadzista female mammoths. The Berelëkh tibias, from a probable male and two probable females, are in cranial view.
For comparison, Baryshnikov et al. (Reference Baryshnikov, Kuzmina and Hrabryi1977, figs. 4 and 9) presented information about fully fused tibias from the Berelëkh multiple-mammoth locality. Although some mammoth bones in this site may date to different times (Pitulko et al., Reference Pitulko, Basilyan and Pavlova2014; Lozhkin and Anderson, Reference Lozhkin and Anderson2018), we assume that only specimens with similar preservation were selected for analysis in the study. The largest full-grown tibia appears to be >60.0 cm in greatest length, roughly extrapolated using the scale bar in a photograph. Two other fully grown but much smaller tibias are shown with this specimen in a photograph (Baryshnikov et al., Reference Baryshnikov, Kuzmina and Hrabryi1977, fig. 4) (see Fig. 8); the projected LSH-1 values of these three individuals are 316.4, 267.5, and 244.7 cm. The smaller tibias belong to mammoths whose shoulder heights were ~15–23% less than that of the largest individual. Similarly, Larramendi (Reference Larramendi2016) found a 23% difference between statures of male and female mammoths. We think the largest tibia is from an adult male, and the other two are from adult females. The male–female difference in stature is very similar to the difference seen with L. africana in Uganda (Laws et al., Reference Laws, Parker and Johnstone1970), where the oldest adult male elephant was 21.8% taller than oldest females.
The different statures suggest at least one full-grown adult male M. primigenius is present in Berelëkh, along with full-grown adult females. Our bone measurements from Kraków Spadzista suggest that no full-grown male mammoths >30 AEY are in the assemblage.
Based on the tibias in Figure 9, the postulated adult female mammoth statures at Berelëkh vary by at least 8–9%. In L. africana, adult female stature also may vary by ~9%, based on in-flesh measurements of individuals 18–40 yr old (in Laws age groups XIII–XXII and over) (Haynes, Reference Haynes1991, table A10). At Kraków Spadzista, the two fully grown tibias indicate adult female mammoth statures (LSH-1) varied by at least 3.3%. Variability in mature female stature may result from slowing of growth in some individuals because of relatively young age at first conception, the burden of multiple pregnancies before age 30 AEY, poor nutrition under subnormal range conditions, or simple genetic factors.
Why are there no full-grown adult male mammoths at Kraków Spadzista?
Laws et al. (Reference Laws, Parker and Johnstone1970) found that the mean age of male sexual maturity varied significantly among L. africana populations in East Africa. Sperm production in several subpopulations began on average in Laws age group X (11–13 yr), and males were fully sexually mature by Laws age group XII (~15 yr). Thus, at about 12–15 yr, L. africana males begin spending less time with their parental family units (Laws et al., Reference Laws, Parker and Johnstone1970). Fisher et al. (Reference Fisher, Rountrey, Beld, Fox, Gohman, Tikhonov, Mol, Buigues, Lazarev and Boeskorov2010) thought the Yukagir adult male M. primigenius from arctic Siberia (see Fig. 1) had been expelled from its matriarchal herd at about age 16 yr (as counted directly from hard tissue growth increments thought to represent single years), showing comparability to what happens with L. africana adolescent males. Adult L. africana males are usually only temporarily attached to family units (Laws et al., Reference Laws, Parker and Johnstone1970; Martin, Reference Martin1978; Moss, Reference Moss1988), as is seen in the summarized Zimbabwe cull data of the 1980s (Haynes, Reference Haynes1991). During the cull operations, mixed herds were targeted by government-sanctioned cull teams, and male-dominated elephant groups were avoided; 5225 animals were shot in the 1983–1985 culls, of which 3320 were males, 87% of which were under 12 yr of age. Laws et al. (Reference Laws, Parker and Johnstone1970) found that about 8.9% of total members in family units in an East African protected area were adult males, while the average in the Zimbabwean protected area was 7.5%. This means that mixed elephant herds of 15–30 members had only 1 or 2 loosely attached adult males. Only some very large groups (>35 associated individuals) contained 2 or more adult males. Thus, the presence of postadolescent but still-growing males in the Kraków Spadzista assemblage and the absence of larger full-grown males reflects the typical proboscidean behavior of few adult males associating with mixed herds. This feature of the assemblage also indicates that older adult males did not die in the same place as members of mixed herds, a characteristic also seen in some L. africana ranges (Haynes, Reference Haynes1991). In addition, large adult male mammoths may have been avoided by Gravettian hunters.
Interpreting the Kraków Spadzista mortality profile
Researchers have analyzed mortality profiles in other Eurasian assemblages of multiple M. primigenius. A comparative sample (see Fig. 1 for locations) includes Nikolskiy and Pitulko (Reference Nikolskiy and Pitulko2013), who interpreted Yana (arctic Siberia) materials; Shpansky (Reference Shpansky2006) and Shpansky and Pecherskaya (Reference Shpansky and Pecherskaya2007), who analyzed Krasniy Yar (subarctic Siberia) materials; Péan (Reference Péan2001), Péan and Patou-Mathis (Reference Péan and Patou-Mathis2003), and Brugère and Fontana (Reference Brugère and Fontana2009), who interpreted different samples from Milovice I (Czech Republic); Maschenko (Reference Maschenko2002), who studied the Sevsk (European Russia) mammoths; and Nikolskiy et al. (Reference Nikolskiy, Basilyan, Sulerzhitsky and Pitulko2010) and Baryshnikov et al. (Reference Baryshnikov, Kuzmina and Hrabryi1977), who examined Berelëkh (arctic Siberia) materials. Table 4 shows data about these studies. Some analysts applied the Laws (Reference Laws1966) system of age assignment, with their results cited here as “AEY”; others did not, and their results are cited as “yr.”
Table 4 Sample of sites with mortality profiles of multiple Mammuthus primigenius, arranged from geologically oldest to youngest. Probable misprints/typos in originals are noted with “?” or have been corrected. AEY, African (elephant) equivalent years; MNI, minimal number of individuals.

The pie charts in Figure 10 graphically depict simplified age distributions of these assemblages. The three charts across the top of Figure 10 are archaeological, and the other three are nonarchaeological. The age ranges assigned by different analysts do not allow clear comparison, but a rough observation is that either juvenile or prime-age adults may dominate archaeological and nonarchaeological assemblages. The domination by either young or adult individuals therefore does not always clearly result from single types of events or processes, such as human hunting choices. Although a domination by adults fits a zooarchaeological model of humans choosing to kill prime adults (e.g., Stiner, Reference Stiner1990), it is apparent that noncultural death processes also create adult-dominated assemblages. As for the assemblages dominated by young animals, we propose that they were often created during times of environmental instability, which elevated juvenile mortality rates from natural causes and sometimes also greatly raised the youngest animals’ vulnerability to human hunting.

Figure 10 (color online) Pie charts comparing age groups at the six Eurasian multiple-mammoth sites in Table 4. AEY, African (elephant) equivalent years.
The Yana locality contains seven loci, including an accumulation of mammoth bones and nearby human occupation sites. The bones of mammoths and other mammals are mostly dated to 28,000–27,000 14C yr BP (32,000–31,000 cal yr BP) (Basilyan et al., Reference Basilyan, Anisimov, Nikolskiy and Pitulko2011; Nikolskiy and Pitulko, Reference Nikolskiy and Pitulko2013, table 1), chronologically placing them in MIS 3 when climates were unstable and rapidly fluctuating. According to Nikolskiy and Pitulko (Reference Nikolskiy and Pitulko2013), 69% of the mammoth individuals in the archaeological locus are “subadult” (<15–18 yr?), selected for killing by people “every several years” to procure tusks of preferred sizes and shapes in order to manufacture hunting weaponry. This same age group would have been the most vulnerable to noncultural stresses as well, and some individuals in the population may have died during unusually severe storms, deep snowfall, flooding, or other effects of environmental instability of the time (Haynes, Reference Haynes1991).
At the archaeological site Milovice I (see Table 4 for approximate chronological age), >80% of mammoths from all areas of the site are >12 AEY, based on analysis of mandibles and teeth (Brugère et al., Reference Brugère, Fontana and Oliva2009; Haynes, Klimowicz, Wojtal, Wilczyński, unpublished data). This domination by adults contrasts notably with the situation at Kraków Spadzista. The domination by adult mammoths at Milovice I might reflect human selectivity in hunting prime adults, perhaps during a period of extreme mammoth vulnerability. We doubt that the adult domination reflects human selectivity in collecting larger mammoth bones from natural death sites for use in making huts or shelters (Oliva, Reference Oliva1988, Reference Oliva2009), because there are so many small and nonutilitarian bones in the assemblage, which also would argue against a taphonomic bias in preservation of larger bones. The significance of the difference between the Milovice I and Kraków Spadzista mortality profiles remains to be further studied.
At the nonarchaeological site Krasniy Yar, dated to the LGM (see Table 4 for approximate chronometric age), a bit more than half the mammoths in the total are <17 yr in estimated age, and most of those are <3 yr old; this also may reflect high mortality rates of the youngest and smallest individuals during a very cold and dry period.
At the nonarchaeological site Sevsk, dated to a cold interval that is one of three cold “subevents” occurring in the span of ~300 yr within Greenland interstadial 1 (Rasmussen et al., Reference Rasmussen, Bigler, Blockley, Blunier, Buchardt, Clausen and Cvijanovic2014) (see Table 4 for approximate chronometric age), ~42% of the individuals are <13 yr old, again possibly reflecting a peak in noncultural deaths of the youngest animals in a mammoth population with already depleted youngest cohorts. Extrapolated shoulder heights of two Sevsk individuals aged 7–13 yr (Maschenko, Reference Maschenko2002, table 23) are relatively small (Fig. 8a) when compared with the 12- to 15-yr-old Yuribei individual and the Kraków Spadzista juveniles of similar estimated ages (Fig. 8b), perhaps attributable to an adaptive reduction in body size in the local subpopulation during a period of environmental instability. The smallest adult is estimated at 190 cm LSH, and the largest at 240–245 cm (Maschenko et al., Reference Maschenko, Gablina, Tesakov and Simakova2006).
At the nonarchaeological site Berelëkh, where mammoth bones had accumulated rapidly in the Bølling warming near the end of the Pleistocene (Nikolskiy et al., Reference Nikolskiy, Basilyan, Sulerzhitsky and Pitulko2010; Pitulko et al., Reference Pitulko, Basilyan and Pavlova2014), about half the individuals are <20 yr old in estimated age, once again potentially reflecting a noncultural death pattern in which the youngest animals had the highest mortality rates in a period of environmental instability. An uncertainty about this site is the set of decisions concerning which bones to collect or not collect during the scientific recovery expedition; the proportions of different age groups may have been affected.
It is unclear if limb bone lengths (and implied shoulder heights) of Berelëkh individuals (Maschenko, Reference Maschenko2002, table 23; Maschenko et al., Reference Maschenko, Gablina, Tesakov and Simakova2006, table 2) show the same unmistakable size reduction seen at a nonarchaeological site Sevsk, which is only a little older, because the different analyses used unmatching ranges of ontogenetic age estimates. However, the largest adults at Berelëkh are estimated to be taller than the largest Sevsk adult (Maschenko et al., Reference Maschenko, Gablina, Tesakov and Simakova2006), suggesting older adult males are not present in the Sevsk sample.
The significance of this brief exploration of a small sample of multimammoth assemblages with direct dating is that there may be a positive correlation between climate-related environmental instability, peak mortality rates in younger mammoths, and increased motivation for people to hunt the more vulnerable mammoths. The high incidence of abnormal and pathological bones at Kraków Spadzista hints at the biological toll taken on very young mammoths when minerals and nutrients at times were in severely short supply, such as during extended dry or very cold periods, which may have lasted for months. A population that is subject to such recurring stresses would have been very vulnerable to human hunting.
Paleoenvironment at Kraków Spadzista and possible effects on mammoth life conditions
Pryor et al. (Reference Pryor, O’Connell, Wojtal, Krzemińska and Stevens2013) extracted stable oxygen and carbon isotopes from the teeth of 13 Kraków Spadzista mammoths and concluded the animals lived together in the same territorial range and died over a relatively short time period. Drucker et al. (Reference Drucker, Rivals, Münzel and Bocherens2016) also found that stable isotopes from Kraków Spadzista teeth were similar enough to indicate those mammoths were part of the same subpopulation. The isotope data show the mammoths died in a cold period, which was part of a cycle of oscillation from cold to warm and back to cold; such instability occurred in brief time spans, perhaps less than a decade.
At any time in this period, the hill where the site is located would have provided minerals and micronutrients different from what was available on the flatlands and floodplains below. Łanczont et al. (Reference Łanczont, Madeyska, Mroczek, Hołub, Żogała and Bogucki2015a, Reference Łanczont, Madeyska, Mroczek, Komar, Łącka, Bogucki, Sobczyk and Wilczyński2015b, Reference Łanczont, Mroczek, Madeyska, Komar, Holub, Żogała, Sobczyk and Wilczyński2016) proposed that freshwater springs were present on the Sowiniec horst during Gravettian times, issuing from calcareous rock, and thus rich in dissolved minerals. Mammoths would have been attracted to mineral sources, especially in times when forage was scarce or when females were pregnant and in need of supplemental micronutrients, such as in late winter or early spring seasons. Nývltový Fišaková (Reference Nývltový Fišaková2009) analyzed dental cementum increments in a sample of Kraków Spadzista fauna and proposed that the main season of human subsistence hunting of herbivores was springtime.
Another possible attractant at the site for mammoths (as it would have been for humans) was the hill’s drier, well drained, and breezier conditions, above the flatlands and floodplains below where insect pests and standing water would have been disagreeable during late winter or early springtime. In modern times, warm downdraft winds from the Tatra Mountains south of the site can significantly raise river valley temperatures in different seasons (Curtis, Reference Curtis1994), and these may have occurred in the Pleistocene. Even in the midst of a cold period, sudden warming might have quickly—if temporarily—thawed river ice and snow cover, causing the Vistula and Rudawa Rivers just below the site to flood and effectively remove access to flatland forage plants for mammoths and other grazing animals. A sudden winter thawing and flooding of lowlands would have forced mammoths to move to higher ground, to avoid the cold standing water.
If mammoths were on the hill to escape lowland flooding, or if they were there to supplement their diet with minerals from the springs, they may have reduced their activity levels to conserve energy. We speculate that mammoths were able to depress metabolism, perhaps for hours, which is a form of hibernation known to be an effective coping mechanism among large mammals. The obvious example of a hibernator would be bears (Ursus spp.), of course, but we are not suggesting mammoths hibernated the way bears are known to do. We are speculating that brown fat deposits in woolly mammoths (as analyzed by Guil-Guerrero et al., Reference Guil-Guerrero, Tikhonov, Rodriguez-García, Protopopov, Grigoriev and Ramos-Bueno2014) allowed animals to become relatively inactive for extended periods of time when energy had to be conserved, such as during periods of forage scarcity or severe weather. The possibility of semihibernation is not as far-fetched for mammoths as it may seem—it is known to be an adaptive strategy for modern Yakutian horses (Equus ferus caballus) which “stay mainly in sleeping position with minimal feeding” (Guil-Guerrero et al., Reference Guil-Guerrero, Tikhonov, Rodriguez-García, Protopopov, Grigoriev and Ramos-Bueno2014, p.5) when weather conditions are harsh. Mid-Holocene frozen horse carcasses and Pleistocene frozen mammoths from Siberia have the same kinds of fatty acids that modern hibernating mammals possess (Guil-Guerrero et al., Reference Guil-Guerrero, Tikhonov, Rodriguez-García, Protopopov, Grigoriev and Ramos-Bueno2014). We think the Kraków Spadzista mammoths were capable of waiting out severe and windy storms, deep snowfalls, flooded lowlands, or other weather-related difficult times by semihibernating. The hilltop location of the site was possibly a refuge area.
We also speculate that mammoths would have been sluggish and inattentive for part of each day, or longer, as are recent African elephants during stressful periods such as sustained drought, when food and water are scarce (Haynes, Reference Haynes1991). Starving elephants and those waiting their turn to gain access to scarce water points are often distracted enough to allow humans on foot to approach much more closely than when the animals are healthy. We suggest mammoths were far less defensively wary during energy-conserving resting states in times of severe environmental stress.
The climate in northern Europe 30,000–20,000 cal yr BP was generally unstable, with geologically rapid fluctuations between cold and warmer conditions, as detected in ice cores and marine and terrestrial records (Ukkonen et al., Reference Ukkonen, Aaris-Sørensen, Arppe, Clark, Daugnora, Lister and Lõugas2011, and references therein). It is possible that short-term weather events such as severe storms or abrupt warming spells were occasional (or frequent) occurrences in such a changeable climatic setting. Although there can be no doubt woolly mammoths with their thick fur and efficient fat deposits were well adapted to cold and dry conditions, and that they could survive a wide variety of climates, including very harsh ones—substantiated by their long-term occupation of geographic regions stretching from north of the Arctic Circle to southern Spain—short-term instability or abrupt weather events would have been difficult to deal with for any animal, especially very cold spells, rapid deep snowfalls, or extreme warming. Being able to semihibernate may have been one more adaptive trait of woolly mammoths.
CONCLUSIONS
We think the Kraków Spadzista mammoths were not driven en masse so far up the high ground by Gravettian hunter-gatherers, because such an unlikely activity would have required coordinated human effort from a large number of people. We also think the huge accumulation of mammoth bones at the site was not amassed piece by piece from natural death sites and carried up the hill by Gravettian people for building shelters, because the presence of nonutilitarian elements in the assemblage argues against such an inefficient activity. We are convinced the Kraków Spadzista mammoths were on the hill to seek necessary minerals, to feed on the plant resources, or to escape flooding or insect pests on the floodplains below. We also think Gravettian foragers were attracted to the site to pick off the most vulnerable or inattentive individuals.
Mammoths, like modern African elephants, were capable of climbing the hill voluntarily. Although African elephants generally avoid climbing steep slopes, they will make the effort when their aim is to reach food, water, mineral licks, or cover that cannot be found any other way. An undated photograph in Beard (Reference Beard1977, p.77) shows a dead L. africana bull, captioned “An elephant at 16,000 feet [~4880 m],” on Mount Kenya, several thousand feet above the plateau at the foot of the mountain, and about 1000 feet (~305 m) shy of the summit. The elephant’s steep and long climb to this elevation would have been through freezing air temperatures. No one knows what this elephant was doing so far up the mountain, but the carcass clearly demonstrates a proboscidean ability to climb.
The drop-off at the very edge of Kraków Spadzista is too steep for mammoths to have scaled, but gentler slopes are located to the west, which mammoths could have climbed in search of whatever resources attracted them. If mammoths were in a fleeting state of semihibernation, they would have been very vulnerable to human hunters, who opportunistically killed and butchered them whenever possible. It is also possible that large gregarious carnivores killed some younger mammoths, because cooperative hunting by extant Crocuta crocuta and Panthera leo is known to kill young elephants in Africa (Salnicki et al., Reference Salnicki, Teichmann, Wilson and Murindagomo2001). We note, however, that Pleistocene Crocuta was no longer present in this part of Europe at the time the Kraków Spadzista mammoths died (Stuart and Lister Reference Stuart and Lister2014), and no bones of Pleistocene Panthera are in the faunal assemblage. In addition, the extremes of damage that hyenas and lions are expected to have created on bones (e.g., Haynes, Reference Haynes1991) are not seen in the Krakow Spadzista materials. Diedrich (Reference Diedrich2014) thought carnivore gnaw damage to middle Pleistocene Paleoloxodon bones was created by Pleistocene hyenas and lions, but some illustrated damage is less severe than what extant hyenas and lions do to Loxodonta bones in Africa (Haynes, G., unpublished manuscript), which we think instead implicates another large Pleistocene carnivore, Canis lupus, the wolf. Some gnaw damage on the Palaeoloxodon bones appears similar to damage done to Kraków Spadzista mammoth bones. We speculate that the wolf is the likeliest culprit in most of the gnawing on Kraków Spadzista bones. The gnaw damage may be from wolves scavenging human-killed and butchered mammoths, but it is also possible that packs of Pleistocene wolves killed some of the smallest mammoths at the site.
In summary, the Kraków Spadzista mammoths represent a periodically stressed local population susceptible to recurring natural die-offs and intensified human hunting. Environmental stress and opportunistic Gravettian hunting preferentially depleted the younger mammoth cohorts several times in the past, but even the relatively depleted cohorts of older adults that had survived earlier mortality events, at a cost to their growth and health, also succumbed. The shape of the mortality profile is a result of serial human hunting of mammoths opportunistically encountered on the hill, in effect a random sampling of animals of different ages from the local mammoth population, along with some natural deaths of mostly the youngest individuals.
Guil-Guerrero et al. (Reference Guil-Guerrero, Tikhonov, Rodriguez-García, Protopopov, Grigoriev and Ramos-Bueno2014) point out that the frozen mammoth carcasses of Siberia contained omega-3 fatty acids, and by extension the mammoths killed by Gravettian hunters at Kraków Spadzista also would have provided this valuable nutrient in human diets. The high value of mammoths for Gravettian people’s diets, together with the extreme vulnerability of mammoths on the high hill during a cold period ~27,000 cal yr BP, are reasonable explanations for the occurrence of the huge mammoth-bone accumulation at Kraków Spadzista.
We acknowledge that these conclusions are interpretive stories based on circumstantial evidence, and even though they make sense, they are not the only stories that could be told about Kraków Spadzista. Another story would be that Gravettian hunters were expert in driving mammoths en masse up the hill and against the cliff edge, where the animals were trapped, speared to death, and butchered. However, the irregular mortality profile of the Kraków Spadzista mammoths, the relatively smaller body sizes of adults compared with those in Milovice I, the notable frequency of pathologies, the climatic instability at the time the assemblage was formed, the limited weathering on the bones, and other taphonomic factors discussed in Wojtal and Sobczyk (Reference Wojtal and Sobczyk2005) together lead us to postulate that the bone accumulation represents weakened and inattentive animals that were mostly killed and butchered individually or in small groups by Gravettian people, possibly occurring along with some mammoths that died naturally.
ACKNOWLEDGMENTS
Research at Kraków Spadzista by PW and Jarosław Wilczyński (Institute of Systematics and Evolution of Animals, Polish Academy of Sciences) was supported by grant 2015/17/B/HS3/0 0165 from the National Science Centre, Poland. GH and JK gratefully acknowledge logistical support from PW and Jarosław Wilczyński, and are thankful for many years of cooperation and support from the Zimbabwe National Parks and Wildlife Management Authority. We also thank the peer reviewers and editors for suggestions that helped us improve the manuscript.
Supplementary material
To view supplementary material for this article, please visit https://doi.org/10.1017/qua.2018.60