Introduction
Chagas disease is an anthropozoonosis prevalent in Latin America, although cases have been reported in Europe, USA, Japan, New Zealand, Australia and Canada. This disease is caused by the protozoan Trypanosoma cruzi (Lidani et al., Reference Lidani, Andrade, Bavia, Damasceno, Beltrame, Messias-Reason and Sandri2019). According to the World Health Organization, it is estimated that 8 million people are infected by T. cruzi worldwide leading to more than 10 000 deaths per year (WHO/TDR, 2012). After the acute phase, the infected subjects can develop chronic disease characterized by cardiomyopathy and megaviscera (Pérez-Molina and Molina, Reference Pérez-Molina and Molina2018). The available treatment for Chagas disease is limited to nifurtimox and benznidazole, however they are only effective in the acute phase (Lacerda et al., Reference Lacerda, Pelegrini, De Oliveira, Vasconcelos and Grossi-de-Sá2016). Therefore, there is a pressing need for new and efficient alternatives for the treatment of Chagas disease.
Antimicrobial peptides (AMPs) are promising candidates for the treatment of infectious diseases (Lacerda et al., Reference Lacerda, Pelegrini, De Oliveira, Vasconcelos and Grossi-de-Sá2016; Giovati et al., Reference Giovati, Ciociola, Magliani and Conti2018). AMPs from animal venom have been described by antichagasic activity (Bandeira et al., Reference Bandeira, Bandeira-Lima, Mello, Pereira, De Menezes, Sampaio, Falcão, Rádis-Baptista and Martins2018; Mello et al., Reference Mello, Lima, Menezes, Bandeira, Tessarolo, Sampaio, Falcão, Rádis-Baptista and Martins2017; Lima et al., Reference Lima, Mello, Bandeira, De Menezes, Sampaio, Falcão, Morlighem, Rádis-Baptista and Martins2018; Freire et al., Reference Freire, Torres, Lima, Monteiro, de Menezes, Martins and Oliveira2020). VmCT1 is an AMP isolated from the venom of the scorpion Vaejovis mexicanus, which contains 13 amino acid residues and an amidated C-terminal extremity (Phe-Leu-Gly-Ala-Leu-Trp-Asn-Val-Ala-Lys-Ser-Val-Phe-NH2). It presents antimicrobial activity against Gram-positive and Gram-negative bacteria (Ramírez-Carreto et al., Reference Ramírez-Carreto, Quintero-Hernández, Jiménez-Vargas, Corzo, Possani, Becerril and Ortiz2012).
The designed Arg-substituted analogues of VmCT1 with a higher net positive charge (+3), than the wild-type (+2) showed higher antimicrobial activity against Gram-positive, Gram-negative bacteria and fungi (Pedron et al., Reference Pedron, Araújo, da Silva Junior, da Silva, Torres, T and Oliveira Junior2019). Here, we evaluated the antichagasic activity of the scorpion venom peptide VmCT1 and its Arg-substituted analogues against the epimastigote, trypomastigote and amastigote forms of T. cruzi, as wells as, their mechanism of action.
Methodology
Solid-phase peptide synthesis, purification and analysis
The peptide synthesis has been previously detailed by Torres et al. (Torres et al., Reference Torres, Pedron, Araújo, Silva, Silva and Oliveira2017). Briefly, we performed solid-phase peptide synthesis on a peptide synthesizer (PS3-Sync Technologies), fluoromethyloxycarbonyl (Fmoc) strategy and the peptides were anchored to a Rink Amide resin with a substitution degree of 0.52 mmol g−1. The dry protected peptidyl-resin was cleaved by exposure to TFA/anisole/water (95:2.5:2.5, v:v:v) for 2 h at room temperature. The crude peptides were precipitated with anhydrous diethyl ether, separated from the ether-soluble reaction components by filtration, extracted from the resin with 60% acetonitrile in water, and lyophilized, according to Pedron et al. (Reference Pedron, Torres, Lima, Silva, Silva and Oliveira2017).
The crude peptides were purified by semi-preparative reverse-phase high-performance liquid chromatography on a Delta Prep 600 (Waters Associates) and the selected fractions containing the purified peptides were pooled and lyophilized. The peptides were characterized by liquid-chromatography electrospray-ionization mass spectrometry using a Model 6130 Infinity mass spectrometer coupled to a Model 1260 high-performance liquid chromatography system (Agilent), which conditions have been previously described in details by Torres et al. (Reference Torres, Pedron, Araújo, Silva, Silva and Oliveira2017).
Parasites and LLC-MK2 cells culture
LLC-MK2 kidney cells (ATCC CCL-7), epimastigote and trypomastigote forms of T. cruzi Y strain were kindly donated by the Laboratório de Bioquímica de Parasitas (Instituto de Química, Universidade de São Paulo, São Paulo, Brazil). As previously described by Bandeira et al. (Reference Bandeira, Bandeira-Lima, Mello, Pereira, De Menezes, Sampaio, Falcão, Rádis-Baptista and Martins2018), LLC-MK2 were maintained at 37°C under 5% CO2 atmosphere in Dulbecco's Modified Eagle Medium (DMEM) (Vitrocell, São Paulo, Brazil) containing 10% of foetal bovine serum (FBS) and antibiotics (penicillin 100 U mL−1 and streptomycin 130 μg mL−1) (Bandeira et al., Reference Bandeira, Bandeira-Lima, Mello, Pereira, De Menezes, Sampaio, Falcão, Rádis-Baptista and Martins2018). These cells were infected by trypomastigote forms of T. cruzi to obtain amastigote forms and to maintain trypomastigote forms. The infection cycle was maintained weekly at 37°C in a 5% CO2 atmosphere in DMEM medium (Vitrocell, São Paulo, Brazil) supplemented with antibiotics (penicillin 100 U mL−1 and streptomycin 130 μg mL−1) and a 2% FBS. Epimastigotes were maintained weekly in Liver Infusion Tryptose (LIT) medium 10% FBS at 28°C (Meira et al., Reference Meira, Guimarães, Dos Santos, Moreira, Nogueira, Tomassini, Ribeiro, de Souza, Ribeiro Dos Santos and Soares2015).
Peptides activity assay against T. cruzi trypomastigote forms
The trypomastigote forms of T. cruzi were obtained according to the methodology described by Bandeira et al. (Reference Bandeira, Bandeira-Lima, Mello, Pereira, De Menezes, Sampaio, Falcão, Rádis-Baptista and Martins2018). Briefly, the trypomastigote forms of T. cruzi obtained by infecting LLCMK2 cells with trypomastigote in T-25/75 cm2 flasks were harvested (800 g for 7 min), plated in 96-well plates (1 × 106 parasites/well) with 2-fold dilutions of VmCT1, [Arg]3-VmCT1, [Arg]7-VmCT1 and [Arg]11-VmCT1 (concentration ranging from 25 to 0.19 μmol L−1 of peptide), PBS as negative control and benznidazole as positive control (concentrations ranging from 1000 to 15.6 μmol L−1; Roche®, Basileia, Switzerland) and incubated at 37°C under 5% CO2 atmosphere in DMEM medium (Vitrocell, São Paulo, Brazil) supplemented with antibiotics (penicillin 100 U mL−1 and streptomycin 130 μg mL−1) and 10% FBS (Adade et al., Reference Adade, Carvalho, Tomaz, Costa, Godinho, Melo, Lima, Rodrigues, Zingali, Souto-Padrón and Souto-Padron2014). After 24 h of incubation, cell viability was measured by counting in a haemocytometer chamber. Parasites treated with sterile PBS were considered negative controls (100% viability) and all experiments were carried out in triplicate.
Cytotoxicity to mammalian cells
Cell viability was measured using a standard 3-(4,5-Dimethylthiazol-2-yl)-2,5-Diphenyltetrazolium Bromide (MTT) assay (Vanden Berghe et al., Reference Vanden Berghe, Grootjans, Goossens, Dondelinger, Krysko, Takahashi and and Vandenabeele2013). Briefly, LLC-MK2 cells were plated in DMEM medium 10% FBS supplemented with antibiotics (penicillin 100 U mL−1 and streptomycin 130 μg mL−1), treated with the same range of concentrations in 2-fold dilutions described in the previous section for VmCT1, [Arg]3-VmCT1, [Arg]7-VmCT1 or [Arg]11-VmCT1, and benznidazole and incubated at 37°C for 24 h. The cells were incubated for 4 h in the presence of MTT (Amresco, Ohio, USA; 5 mg mL−1) after that a 10% sodium dodecyl sulphate (SDS; Vetec, São Paulo, Brazil) solution was added to solubilize the formazan product. Cell viability was assessed by absorbance at 570 nm on a microplate reader (Biochrom® Asys Expert Plus) (Bandeira et al., Reference Bandeira, Bandeira-Lima, Mello, Pereira, De Menezes, Sampaio, Falcão, Rádis-Baptista and Martins2018). Cells treated with sterile PBS were considered negative controls (100% viability). The EC50 was determined by non-linear regression and the selectivity index (SI) was calculated by the formula: EC50 of LLC-MK2/EC50 of trypomastigote forms (Nwaka and Hudson, Reference Nwaka and Hudson2006).
Effect of VmCT1 and analogues on T. cruzi amastigote forms
The effects of peptides on the amastigote forms were assessed as described by Lima et al. (Reference Lima, Sousa, Torres, Rodrigues, Mello, Menezes, Tessarolo, Quinet, de Oliveira and Martins2016). Briefly, LLC-MK2 cells were seeded on 24-well plates containing coverslips (13 mm of diameter) and cultured in DMEM medium supplemented with 10% FBS and antibiotics (penicillin 100 U mL−1 and streptomycin 130 μg mL−1), at 37°C, in a 5% CO2 atmosphere, for 24 h. Next, the medium was removed and cells were infected with trypomastigote forms (parasite-host cell ratio of 20:1) in DMEM medium containing 2% FBS. After 48 h of incubation, the infected cells were washed and treated with the half maximal effective concentration (EC50) of trypomastigotes of the peptides VmCT1, [Arg]7-VmCT1 or [Arg]11-VmCT1. The coverslips were collected after 24 h, washed with PBS, fixed in Bouin's solution and stained with Giemsa, according to Lima et al. (Reference Lima, Sousa, Torres, Rodrigues, Mello, Menezes, Tessarolo, Quinet, de Oliveira and Martins2016). Non-treated infected cells were used as negative control and benznidazole at 282 μmol L−1 was used as positive control. Amastigote cells per 100 cells were determined by counting 300 cells from the different groups obtained from different wells.
VmCT1 and analogues activity assay against T. cruzi epimastigote forms
Epimastigote forms of T. cruzi Y strain at exponential stage were harvested (800 g for 5 min), plated in 96-well plates (1 × 106 parasites/well) and exposed to VmCT1, [Arg]3-VmCT1, [Arg]7-VmCT1 or [Arg]11-VmCT1 at concentrations ranging from 100 to 0.78 (2-fold dilutions) in LIT medium supplemented with antibiotics (penicillin 100 U mL−1 and streptomycin 130 μg mL−1) and 10% FBS. Benznidazole (2-fold dilutions with concentrations ranging from 1000 to 15.6 μmol L−1) was used as positive control. Parasite growth inhibition was quantified in a haemocytometer chamber after incubation at 28°C for 24, 48 and 72 h (Rodrigues et al., Reference Rodrigues, Ueda-Nakamura, Corrêa, Sangi and Nakamura2014). Parasites treated with sterile PBS medium were considered negative controls (100% viability) and all experiments were carried out in triplicate.
T. cruzi epimastigote death pathway assays
Flow cytometry was performed to investigate the cell death pathway of epimastigote forms of T. cruzi (Mello et al., Reference Mello, Lima, Menezes, Bandeira, Tessarolo, Sampaio, Falcão, Rádis-Baptista and Martins2017) at exponential stage, when exposed to VmCT1, [Arg]7-VmCT1 and [Arg]11-VmCT1. Briefly, the parasites were treated with the EC50 values of each peptide in LIT medium and incubated for 24 h, washed and stained with different probes (according to the manufacturer's instructions) to evaluate cell death pathway. Phycoerythrin-conjugated annexin V (Annexin V-PE) and/or 7-AAD (7-aminoactinomycin D; BD Pharmingen, California, USA) were used to evaluate the externalization of phosphatidylserine (Annexin V-PE) and/or membrane damage (7-AAD); 2′,7′-Dichlorofluorescin diacetate (DCFH-DA; 20 mmol L−1) was used to evaluate the reactive oxygen species (ROS) formation; and rhodamine 123 (10 μg mL−1) was used to evaluate mitochondrial transmembrane potential. Carbonyl cyanide 4-(trifluoromethoxy)phenylhydrazone (FCCP; 39 μmol L−1) was used as positive control in rhodamine 123 and DCFH-DA assays. Next, the samples were screened in a FACSCalibur flow cytometer (Becton-Dickinson, San Jose, CA), in which 10 000 live events were collected and analysed using the CellQuest software (Becton-Dickinson, San Jose, CA). Epimastigotes treated with PBS were used as negative controls and experiments were carried out in triplicate.
Scanning electron microscopy (s.e.m.)
The epimastigote forms were treated with the EC50 values of VmCT1, [Arg]7-VmCT1 or [Arg]11-VmCT1 and incubated for 12 h. After incubation, the parasites were fixed for 2 h with 2.5% glutaraldehyde (Electron Microscopy Sciences, Hatfield, Pennsylvania), washed, dehydrated, dried with CO2, coated in gold and observed in a FEG Quanta 450 scanning electron microscope (FEI, Oregon, USA), according to Lima et al. (Reference Lima, Mello, Bandeira, De Menezes, Sampaio, Falcão, Morlighem, Rádis-Baptista and Martins2018).
Statistical analysis
The statistical analysis was performed on GraphPad Prism 6 program (GraphPad Software, San Diego, CA, USA). The half maximal effective concentration (EC50) values were calculated by non-linear regression. Data were analysed using one-way analysis of variance followed by Bonferroni's post-test. Significance was defined as *P < 0.05.
Results
The natural AMP VmCT1 and its three Arg-substituted analogues were evaluated against the three developmental forms of T. cruzi. The wild-type peptide presented EC50 at 1.37 ± 0.13 μmol L−1 against trypomastigote forms and SI higher than 50 (58.16). The analogues [Arg]3-VmCT1 and [Arg]11-VmCT1 showed EC50 against trypomastigote forms at lower concentrations values compared to VmCT1 (Table 1). These analogues presented different toxicity profiles towards LLC-MK2 mammalian cells, [Arg]3-VmCT1 presented higher activity against LLC-MK2 mammalian cells, decreasing the SI to 22. [Arg)11-VmCT1 presented the best selectivity among all the peptides tested (175) (Table 1), whereas [Arg]7-VmCT1 presented similar activity and selectivity to VmCT1.
Table 1. Effect of VmCT1 and analogues against trypomastigote form of Trypanosoma cruzi Y strain and LLC-MK2 mammalian cells, and their respective selectivity index (SI)

EC50 values are expressed in μmol L−1. Data are expressed as means ± standard error of the mean (s.e.m.) of three independent experiments. BZ = benznidazole.
Against the epimastigote form of the parasite, [Arg]3-VmCT1 did not show significant difference on EC50 values compared to VmCT1 at any of the three incubation periods analysed. The analogue [Arg]7-VmCT1 showed the highest activity against epimastigotes compared to the wild-type and the other analogues, especially after 24 h of incubation with the parasites. Although [Arg]11-VmCT1 was the most effective on trypomastigote forms, it was the less active on epimastigote forms (Table 2).
Table 2. Effect of VmCT1 and analogues against epimastigote forms of Trypanosoma cruzi Y strain

EC50 values are expressed in μmol L−1. Data are expressed as means ± standard error of the mean (s.e.m.) of three independent experiments. BZ = benznidazole.
Due to the SI lower than 50 (22), [Arg]3-VmCT1 was not used in further studies. Thus, VmCT1, [Arg]7-VmCT1 and [Arg]11-VmCT1 were tested against amastigote forms of T. cruzi. The three peptides were able to decrease the number of amastigotes per 100 cells after 24 h of incubation (Fig. 1). The mechanism of action of the peptides was assessed by flow cytometry and confirmed by scanning electron microscopy (s.e.m.). The membrane damage caused by the peptides was monitored with 7AAD (indicating plasma membrane lesion necrosis) and Annexin-V (apoptosis marker by the externalization of phosphatidylserine) staining, ROS formation was followed by labelling with DCFH-DA and mitochondrial transmembrane potential was assessed by labelling with rhodamine 123.

Fig. 1. The effect of EC50 from trypomastigote forms of VmCT1, [Arg]7-VmCT1 and [Arg]11-VmCT1 on intracellular amastigote forms of Trypanosoma cruzi. (CT: negative control – PBS).
After 24 h of incubation, the EC50 of VmCT1 increased the 7AAD + /AX- population from 0.2 to 9.2% (Fig. 2a), led to higher formation of ROS (33%) (Fig. 3a) and reduced 56% of the mitochondrial transmembrane potential (Fig. 4a). At the EC50 concentration value of [Arg]7-VmCT1, we observed the increase of the 7AAD + /AX- population to 7% (Fig. 2b), with no significant alterations on the formation of ROS (Fig. 3b) or mitochondrial transmembrane potential (Fig. 4b). The analogue [Arg]11-VmCT1, at EC50 concentration, caused an increase in the 7AAD + /AX- population to 45% (Fig. 2c), a reduction of 60% of mitochondrial transmembrane potential (Fig. 4c) and no significant alterations on the formation of ROS (Fig. 3c). FCCP, an uncoupler, used as positive control was able to increase ROS formation in three times (Fig. 3) and to reduce mitochondrial transmembrane potential about 60% (Fig. 4).
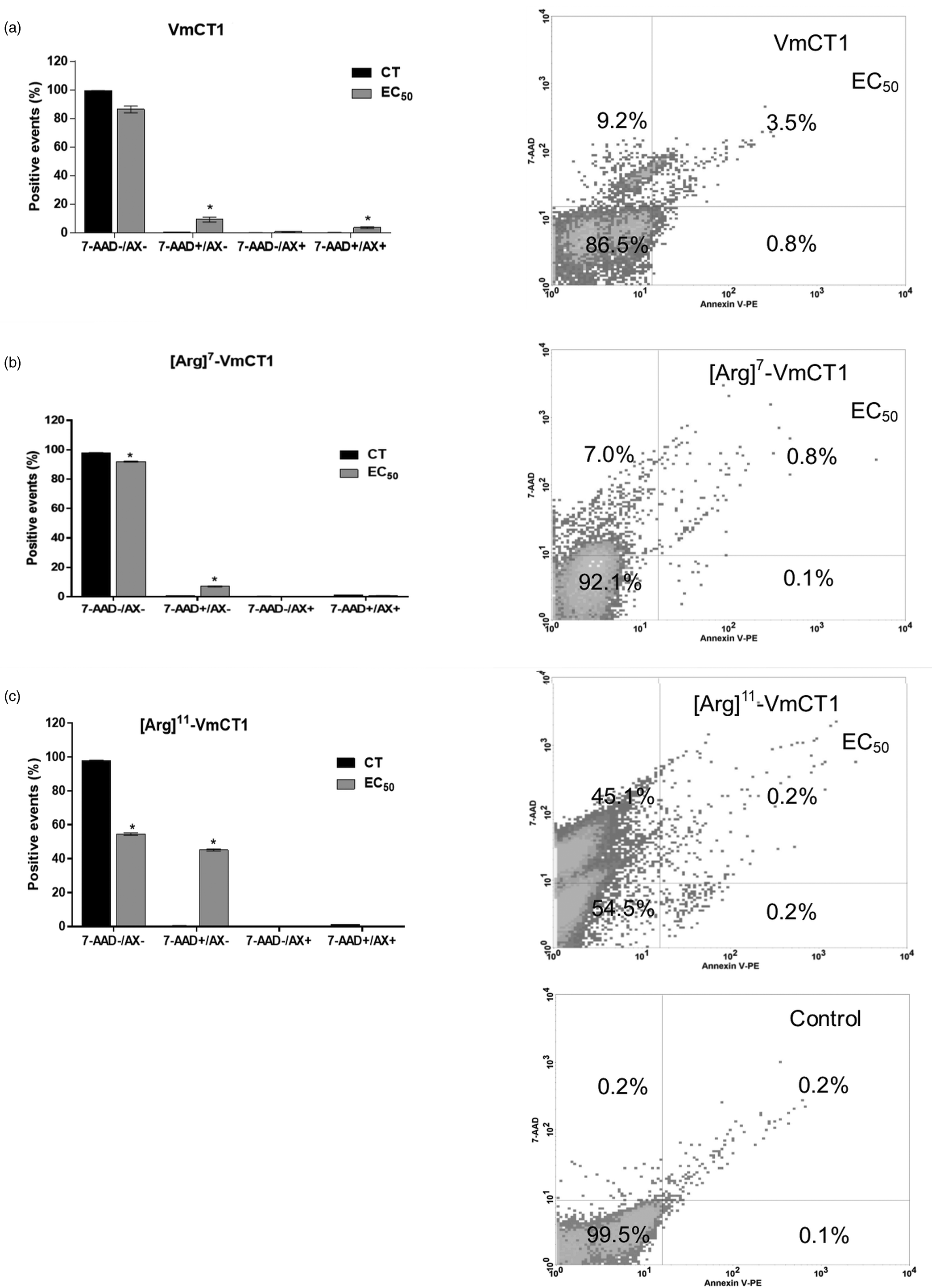
Fig. 2. Flow cytometry of Trypanosoma cruzi epimastigote forms treated with (a) VmCT1 at 13.4 μmol L−1, (b) [Arg]7-VmCT1 at 5.4 μmol L−1 and (c) [Arg]11-VmCT1 at 42.8 μmol L−1 for 24 h. Percentages values are averages of 10 000 live events from three independent experiments (CT: negative control – PBS).

Fig. 3. Flow cytometry analysis of the formation of ROS in Trypanosoma cruzi epimastigotes exposed to peptides. 2′,7′-dichlorofluorescein (DCF) fluorescent signal and relative fluorescence values from experiments with T. cruzi epimastigotes in exponential stage that were treated for 24 h with (a) VmCT1 at 13.4 μmol L−1, (b) [Arg]7-VmCT1 at 5.4 μmol L−1 and (c) [Arg]11-VmCT1 at 42.8 μmol L−1. The measurements were obtained from three independent experiments. (CT: negative control – PBS).

Fig. 4. Flow cytometry analysis of mitochondrial transmembrane potential changes in Trypanosoma cruzi epimastigotes exposed to peptides. Rhodamine 123 (Rho 123) fluorescent signal and relative fluorescence values from experiments with T. cruzi epimastigotes in exponential stage that were treated for 24 h with (a) VmCT1 at 13.4 μmol L−1, (b) [Arg]7-VmCT1 at 5.4 μmol L−1 and (c) [Arg]11-VmCT1 at 42.8 μmol L−1. The measurements were obtained from three independent experiments. (CT: negative control – PBS).
These results were confirmed by s.e.m. images, which showed pore formation on the parasite membrane in the treatment with the three peptides (Fig. 5), indicating that these peptides damaged the membrane of the parasite.

Fig. 5. Scanning electron microscopy of Trypanosoma cruzi epimastigotes treated with peptides. (a) Control epimastigotes treated with PBS, (b) treated with EC50 of VmCT1, (c) [Arg]7-VmCT1 and (d) [Arg]11-VmCT1. Scale bar: 5 μm.
Discussion
Natural AMPs from animal venoms have been reported as antiprotozoal agents (Vinhote et al., Reference Vinhote, Lima, Menezes, Mello, de Souza, Havt, Palma, Santos, Albuquerque, Freire and Martins2017; Bandeira et al., Reference Bandeira, Bandeira-Lima, Mello, Pereira, De Menezes, Sampaio, Falcão, Rádis-Baptista and Martins2018; Lima et al., Reference Lima, Mello, Bandeira, De Menezes, Sampaio, Falcão, Morlighem, Rádis-Baptista and Martins2018). They also are promising molecular scaffolds for drug engineering, aiming antimicrobial agents with low toxicity and higher biological activity (Ortiz et al., Reference Ortiz, Gurrola, Schwartz and Possani2015; Fratini et al., Reference Fratini, Cilia, Turchi and Felicioli2017). These molecules are abundant in scorpion venoms, such as VmCT1 in V. mexicanus smihi scorpion venom. Previously, we designed VmCT1 analogues exploring different physicochemical and structural properties which led to increased antimicrobial activity and lower cytotoxicity (Pedron et al., Reference Pedron, Torres, Lima, Silva, Silva and Oliveira2017; Reference Pedron, Araújo, da Silva Junior, da Silva, Torres, T and Oliveira Junior2019). Due to the biotechnological potential of VmCT1 and VmCT1-derived peptides, we analysed their antichagasic effects. We evaluated these peptides against the T. cruzi main forms (epimastigotes, trypomastigotes, amastigotes) and their cell death pathway.
A previous work with stigmurin, AMP isolated from venom of the scorpion Tityus stigmurus with trypanocidal effect and low hemolytic activity, performed a rational design obtaining the peptides StigA6, StigA16 with higher net charge and hydrophobic moment, being able to inhibit the parasites with lower concentrations, when compared to native stigmurin (Amorim-Carmo et al., Reference Amorim-Carmo, Daniele-Silva, Parente, Furtado, Carvalho, Oliveira, Santos, Silva, Silva, Silva-Júnior, Monteiro and Fernandes-Pedrosa2019). In this work, against trypomastigotes, native VmCT1 presented an EC50 of 1.37 ± 0.13 μmol L−1 and the EC50 of the analogues decreased (0.67–1.15 μmol L−1). Although the low decrease on EC50 of trypomastigotes, the SI of one of the analogues increased in three times, from 58 to 175.
Modifications on physicochemical parameters such as net positive charge, mean hydrophobicity and mean hydrophobic moment can influence on biological activities (Pedron et al., Reference Pedron, Torres, Lima, Silva, Silva and Oliveira2017; Reference Pedron, Araújo, da Silva Junior, da Silva, Torres, T and Oliveira Junior2019; Torres et al., Reference Torres, Pedron, Araújo, Silva, Silva and Oliveira2017; Reference Torres, Pedron, Higashikuni, Kramer, Cardoso, Oshiro, Franco, Silva Junior, Silva, Oliveira Junior, Lu and de la Fuente-Nunez2018). [Arg]3-VmCT1, [Arg]7-VmCT1 and [Arg]11-VmCT1 presented mean hydrophobicity values of 0.65, 0.71 and 0.66, respectively – estimated by the heliQuest server (Gautier et al., Reference Gautier, Douguet, Antonny and Drin2008); which are lower than the wild-type's mean hydrophobicity (0.82) and reported as the reason that led these peptides to higher antimicrobial activity towards microorganisms (Pedron et al., Reference Pedron, Araújo, da Silva Junior, da Silva, Torres, T and Oliveira Junior2019). Here, we observed similar effect when the peptides were tested against T. cruzi.
According to the WHO/TDR, one criteria for considering a molecule as a potential antitrypanosomal agent is presenting a SI higher than 50 (Nwaka and Hudson, Reference Nwaka and Hudson2006). VmCT1, [Arg]7-VmCT1 and [Arg]11-VmCT1 presented SI values higher than the criteria limit, and among all the peptides, [Arg]11-VmCT1 is the most promising antichagasic molecule, with the highest SI (175) and EC50 values against trypomastigote forms lower than 1 μmol L−1.
The selectivity of the AMPs to pathogens is related to their cationicity. It is an important physicochemical feature that influences peptide–membrane interactions (de la Fuente-Nunez et al., Reference de la Fuente-Nunez, Torres, Mojica and Lu2017; Torres et al., Reference Torres, Sothiselvam, Lu and de la Fuente-Nunez2019), once it is related to the initial electrostatic interactions between AMPs and the negatively charged phospholipids present in the membranes of microorganisms (Lohner, Reference Lohner2017; Stutz et al., Reference Stutz, Müller, Hiss, Schneider, Blatter, Pfeiffer, Posselt, Kanfer, Kornmann, Wrede, Altmann, Wessler and Schneider2017). Arg residues present a side chain guanidium group with delocalized positive charge density (Armstrong et al., Reference Armstrong, Mason, Anderson and Dempsey2016), which its replacement in strategical sites could increase the SI.
The replacement of serine by arginine at position 11 led the guanidyl side chain group of this amino acid to interact with water molecules and lipids groups of phospholipids, promoting the formation of hydrogen bonds that can lead to destabilization and disruption or permeabilization of membranes. Furthermore, in previous analyses by circular dichroism spectroscopy, when the peptides were exposed to negatively charged lipid vesicles of POPC:POPG (palmitoyl oleoyl phosphatidylcholine: palmitoyl oleoyl phosphatidylglycerol, 3:1, mol:mol, 10 mmol L−1), [Arg]11-VmCT1 analogue presented lower helical content than the other analogues, which also could be related to the best effect (Pedron et al., Reference Pedron, Araújo, da Silva Junior, da Silva, Torres, T and Oliveira Junior2019).
Beyond selectivity, the effect on intracellular forms has also fundamental importance, because they are the forms that maintain the infection during the chronic phase, phase in which the current treatment is only 20% effective (Urbina and Docampo, Reference Urbina and Docampo2003; Morilla and Romero, Reference Morilla and Romero2015). In this work, VmCT1, [Arg]7-VmCT1 and [Arg]11-VmCT1 were able to decrease the number of amastigote/100 cells after 24 h of incubation at concentrations lower than 1.5 μmol L−1, being lower than 1 μmol L−1 on [Arg]11-VmCT1.
AMPs from arthropods have been showed different action mechanisms (Sabiá Júnior et al., Reference Sabiá Júnior, Menezes, de Araújo and and Schwartz2019), such as mastoparan from Polybia paulista wasp venom, which inhibits glyceraldehyde-3-phosphate dehydrogenase from T. cruzi (TcGAPDH), a key enzyme in the glycolytic pathway (Vinhote et al., Reference Vinhote, Lima, Menezes, Mello, de Souza, Havt, Palma, Santos, Albuquerque, Freire and Martins2017), while Polybia-CP, another AMP from the same venom did not show the same enzymatic inhibition, but showed apoptotic effect (Freire et al., Reference Freire, Torres, Lima, Monteiro, de Menezes, Martins and Oliveira2020). Otherwise, M-PONTX-Dq3a from Dinoponera quadriceps ant venom showed necrotic mechanism on T. cruzi. (Lima et al., Reference Lima, Mello, Bandeira, De Menezes, Sampaio, Falcão, Morlighem, Rádis-Baptista and Martins2018). In this work, epimastigotes treated with the peptides (VmCT1, [Arg]7-VmCT1 and [Arg]11-VmCT1) presented higher effect at lower times of incubation, suggesting a fast action mechanism, such as necrosis. They also showed an increase in membrane permeation on flow cytometry analyses.
Flow cytometry also were used to evaluate the intracellular formation of ROS and transmembrane mitochondrial potential (Bandeira et al., Reference Bandeira, Bandeira-Lima, Mello, Pereira, De Menezes, Sampaio, Falcão, Rádis-Baptista and Martins2018). VmCT1 was the only peptide tested that led to an increase on the formation of ROS. The transmembrane mitochondrial potential was decreased by the peptides VmCT1 and [Arg]11-VmCT1 compared to the negative control. In addition, s.e.m. images showed damage on the membrane of parasites treated with all peptides, showing presence of pore.
The plasma membrane disruption is well established as the most characteristic necrotic event (Menna-Barreto, Reference Menna-Barreto2019), meaning all peptides act through necrosis. VmCT1, [Arg]7-VmCT1 and [Arg]11-VmCT1 showed the same action mechanism, implying the Arg-substitution in the positions 7 and 11 were not able to change the cell death pathway.
Some AMPs from animal venoms with necrotic mechanism on T. cruzi, such as crotalicidin (Bandeira et al., Reference Bandeira, Bandeira-Lima, Mello, Pereira, De Menezes, Sampaio, Falcão, Rádis-Baptista and Martins2018), batroxicidin (Mello et al., Reference Mello, Lima, Menezes, Bandeira, Tessarolo, Sampaio, Falcão, Rádis-Baptista and Martins2017) and M-PONTX-Dq3a (Lima et al., Reference Lima, Mello, Bandeira, De Menezes, Sampaio, Falcão, Morlighem, Rádis-Baptista and Martins2018) are in agreement with the criteria of SI>50 from WHO guidelines for prospection of new drugs (Nwaka and Hudson, Reference Nwaka and Hudson2006), as well the peptide family from this work, while benznidazole showed SI<3 in the same strain.
Besides the SI, the haemolytic effect of this peptide family was previously described and we demonstrated a maximal non-haemolytic concentration of 6.3, 1.6, 3.1 and 3.1 μmol L−1 for VmCT1, [Arg]3-VmCT1, [Arg]7-VmCT1 e [Arg]11-VmCT1, respectively (Pedron et al., Reference Pedron, Araújo, da Silva Junior, da Silva, Torres, T and Oliveira Junior2019). [Arg]11-VmCT1, peptide with the best effect, showed no haemolytic effect in concentrations almost four times bigger than EC50 of trypomastigote forms, which is the same concentration able to reduce number of amastigotes per 100 cells.
Benznidazole has been used against chronic Chagas disease for almost 50 years, but treatment failures (6–50%) are reported in recent clinical trials. Other problematic factors include the extended treatment length (often 60–90 days) and frequent toxic side-effects (Campos et al., Reference Campos, Phelan, Francisco, Taylor, Lewis, Pain, Clark and Kelly2017). It also has low penetrability in membrane, being necessary high gradients of concentrations to gain amastigote forms (Morilla and Romero, Reference Morilla and Romero2015). The toxicity, low selectivity and the necessity of high gradients of concentrations of benznidazole contribute with the treatment failures. In this work, we described one peptide able to reduce 50% of trypomastigote (blood circulating) form viability in concentrations lower than 1 μmol L−1. In this same concentration also was able to reduce about 50% the number of amastigote per 100 cells in a short time of incubation, such as 24 h. Although this work showed only in vitro tests, these characteristics are desirable to therapeutic alternatives to benznidazole.
Conclusion
The scorpion venom peptide VmCT1 presented antichagasic activity against the principal developmental forms of T. cruzi, which was improved in potency and selectivity with an Arg-substitution in position 11, maintaining the mechanism of action of VmCT1, which was necrotic. These results revealed that [Arg]11-VmCT1 is a promising candidate for the development of new therapeutics against Chagas disease.
Acknowledgments
The authors are thankful to the Analytical Center Core Facility of the Federal University of Ceará for the technical support on Electron Microscopy studies and the Multiuser Central Facilities (UFABC) for the experimental support.
Financial support
The authors thank Coordenação de Aperfeiçoamento de Pessoal de Nível Superior – Brasil (CAPES) for funding (PNPD 88887.368537/2019-00 and Finance Code 001) and Fundação de Amparo à Pesquisa do Estado de São Paulo (FAPESP) for funding (VXO #2017/03046-2).
Conflict of interest
The authors declare that they do not have conflict of interest.
Ethical standards
Not applicable.