Introduction
Chiropterans are nocturnal and widely dispersed mammals, representing approximately 20% of the recognized mammalian species in the world (Fenton and Simmons, Reference Fenton and Simmons2015). These flying mammals display long life spans and can occupy diverse and numerous habitats in both natural and urban environments. These characteristics result in their high capacity as seed dispersers as well as parasite dispersion, making these animals important contributors to biodiversity (Luis et al. Reference Luis, Hayman, O'Shea, Cryan, Gilbert, Pulliam, Mills, Timonin, Willis, Cunningham, Fooks, Rupprecht, Wood and Webb2013).
Bats are ancient hosts of Trypanosoma sp. (Trypanosomatida; Protozoa), a parasite genus transmitted between several vertebrate species and blood-sucking invertebrate vector worldwide. Bat trypanosomes included in the Trypanosoma cruzi clade were already described in Asia, Africa, the Americas and Europe (Lima et al. Reference Lima, Espinosa-Álvarez, Hamilton, Neves, Takata, Campaner and Teixeira2013, Reference Lima, Espinosa-Alvarez, Pinto, Cavazzana, Pavan and Carranza2015; Barbosa et al. Reference Barbosa, Mackie, Stenner, Gillett, Irwin and Ryan2016). Within this clade, T. cruzi, a zoonotic parasite responsible for Chagas disease that is currently considered a worldwide problem (Coura et al. Reference Coura, Albajar-Viñas and Junqueira2014). This taxon displays marked heterogeneity, and six discrete typing units (DTUs) are currently recognized (TcI–TcVI) in addition to a putative TcVII DTU, which was previously associated with bats (Tcbat) and has already been observed infecting humans (Zingales et al. Reference Zingales, Miles, Campbell, Tibayrenc, Macedo, Teixeira, Schijman, Llewellyn, Lages-Silva, Machado, Andrade and Sturm2012; Ramírez et al. Reference Ramírez, Hernández, Montilla, Zambrano, Flórez and Parra2014). Other than T. cruzi, all species from the subgenus T. (Schizotrypanum) are described to be restricted to bats, although Trypanosoma dionisii was recently described in cardiac tissue in one human (Dario et al. Reference Dario, Rodrigues, da Silva Barros, das Chagas Xavier, D'Andrea, Roque and Jansen2016).
The most accepted theory to explain the origin of the trypanosomatids from cruzi clade indicates bats are the ancestral hosts (the bat seeding hypothesis), and their flying capacity is responsible for the dispersal of some species, such as T. dionisii, between the Old and New Worlds (Hamilton et al. Reference Hamilton, Cruickshank, Stevens, Teixeira and Mathews2012). Recent molecular and phylogenetic studies have corroborated this theory, describing new species of bat trypanosomes within the T. cruzi clade, including Trypanosoma erneyi and Trypanosoma livingstonei in African bats (Lima et al. Reference Lima, Da Silva, Neves, Attias, Takata, Campaner and Teixeira2012, Reference Lima, Espinosa-Álvarez, Hamilton, Neves, Takata, Campaner and Teixeira2013), Trypanosoma wauwau in South and Central America bats (Lima et al. Reference Lima, Espinosa-Alvarez, Pinto, Cavazzana, Pavan and Carranza2015) and Trypanosoma teixeirae in an Australian little red flying fox (Barbosa et al. Reference Barbosa, Mackie, Stenner, Gillett, Irwin and Ryan2016). In Brazil, bats have been found to be infected by T. cruzi (TcI, TcII and Tcbat), T. rangeli, T. c. marinkellei, T. dionisii and T. wauwau (Lima et al. Reference Lima, Espinosa-Alvarez, Pinto, Cavazzana, Pavan and Carranza2015; da Costa et al. Reference da Costa, Nunes, Leite, Ferreira, Tonhosolo, da Rosa and Marcili2016).
The Acre state is in the Amazon basin, the biome that contains the greatest biodiversity in the world (Hoorn et al. Reference Hoorn, Wesselingh, Ter Steege, Bermudez, Mora, Sevink and Jaramillo2010). The Amazonian region that previously was free from Chagas disease and where only the enzootic transmission cycle of T. cruzi existed, started to be considered as endemic for this disease (Coura and Junqueira, Reference Coura and Junqueira2015). In the state of Acre, 13 autochthonous cases were reported between 1988 and 2015, which contrasts the 25 cases reported in 2016 (data from the Secretary of Health from Acre State). Considering the recent higher effort for case identification and the dispersion of the municipalities that reported cases in a huge area of the state, this historical report of cases is underestimated. There are an estimated 59 species of bats in Acre State in both forest fragments and urban areas. The most abundant species are those from the genera Artibeus, Carollia and Phyllostomus (Bernard et al. Reference Bernard, Tavares and Sampaio2011). Despite their abundance and dispersion within a biome characterized by its biodiversity, the diversity of trypanosomes infecting bats from Acre state is completely unknown. The aim of this study was to describe the chiropterofauna and their associated trypanosomes in areas with different ecological landscapes and degrees of human disturbance in one urbanized and one rural municipality from Acre State in the Brazilian Amazon.
Materials and methods
Study areas
Bats were captured in two municipalities from Acre State, north Brazil: Rio Branco, the capital of the state (09°58′29″S/67°48′36″W), and Xapuri, 175 km away from the former (10°10′95″S/68°30′16″W). Characteristic of Amazonia, the climate is tropical-humid, displaying high levels of regular rainfalls and elevated temperatures. The rainy period is from October to March, whereas April to September has the lowest rain volumes. In Rio Branco, captures were conducted in Parque Zoobotânico (PZ), which is the highest vegetation area of the municipality; it belongs to the Acre Federal University and consists of 150 ha of secondary vegetation that has been preserved since 1983. In Xapuri, captures occurred in Seringal Cahoeira (SC), a well-preserved area of almost 25 thousand ha of pristine vegetation that is 30 km from the centre of the municipality alongside management areas of Hevea brasiliensis. Expeditions were conducted four times to each locality during the wet and dry seasons, in March and August 2014 and June and November 2015.
In each area (PZ and SC), the following three localities were selected according to a gradient of preservation and vegetation characteristics (Fig. 1): Solid Ground (A1 and A4), areas consisting of dense forest with native (A4) or secondary (A1) vegetation with palm trees and bamboo as well as lower stratum with clean aspect; Sandbank (A2 and A5), a less dense vegetation area mainly consisting of palm trees near the floodplains of rivers with high flow during the wet season and characterized by an open canopy forest and spots of dense forest with emergent trees; and Open areas (A3 and A6) near dwellings of human activity (A3) or consisting of rubber trees associated with banana and various timber species (A6).
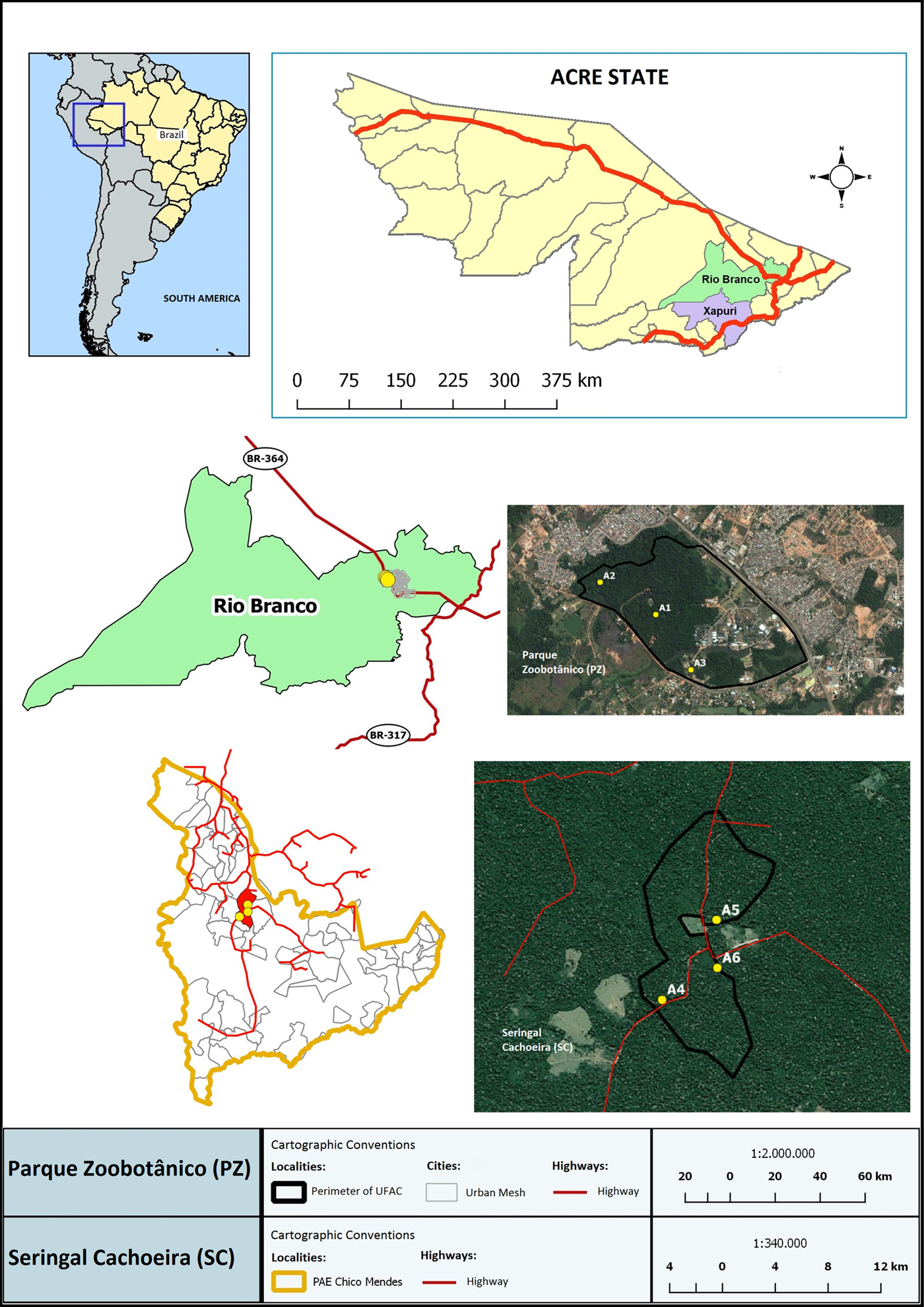
Fig. 1. Geographical location of study area: Mapping of the bat collected areas in Acre state (Amazon Biome). Bats were collected in an urban forest: Parque Zoobotânico – PZ (A1–A3); and one more preserved area: Seringal Cachoeira – SC (A4–A5) in Rio Branco and Xapuri municipalities, respectively. On the left of the figure is the study site in Brazil, highlighting the Acre state and the Brazilian and South America limits.
Sample collection
Bats were captured using 10 mist nets (12 m × 3 m, 35 mm mesh) per night, which were arranged approximately 50 cm from the ground. The nets were installed in the early evening, maintained over 6 h from 6:00 to 12:00 pm (two nights in each area), and checked every 30 min. The total effort was the same for the six areas, which was 2,160 m2-h per night; 4320 m2-h per expedition in each area; 12 960 m2-h per municipality in each expedition and 51 840 m2-h in the four expeditions conducted to each municipality.
Pregnant and/or lactating bat females were removed from the net and immediately released. The others were individually placed in cloth bags and transported to the field laboratory where they stayed until the next morning when the collection procedures were performed. For all collected specimens, the morphological characteristics and body measurements were recorded for age estimations and taxonomic identification, which was determined using the available bibliography (Gardner, Reference Gardner and Gardner2007; Díaz et al. Reference Díaz, Aguirre and Barquez2011).
In the field laboratory that was established exclusively for this purpose, bats were anesthetized (9:1, ketamine chloridrate 10% and acepromazine 2%), and their blood was collected by cardiac puncture. The collected blood was processed as follows: (i) for fresh blood examination, one drop was set between a glass slide and coverslip and observed in an optical microscope at 400× magnification; and (ii) for hemoculture, approximately 0·6 mL of blood was cultured in tubes containing Novy-Mc Neal-Nicole medium with either a liver infusion tryptose medium or Schneider's Insect Medium overlay (0·3 mL in each one).
Parasitologic diagnostic procedures and DNA extraction
Fresh blood examination was performed in the field laboratory and considered positive when at least one parasite or a flagellar movement beyond blood cells was observed. Hemocultures were transported to LABTRIP and analysed every other week for up to 5 months (usually three because of bacterial contamination). The positive hemocultures were amplified, cryopreserved and deposited in the Coleção de Trypanosoma de Mamíferos Silvestres, Domésticos e Vetores, COLTRYP/FIOCRUZ (www.coltryp.fiocruz.br). The amplification of the positive hemoculture means that the observed parasites were allowed to multiply spontaneously at 27 °C until the stationary phase (when cells stopped to multiply), and after what, the flagellates were harvested and DNA was extracted as described below.
We sometimes observed in the culture tubes the presence of flagellates morphologically identical to trypanosomatids, but that did not grow, that is, we could not establish a culture of these parasites. In these cases, the liquid phase of the cultures were harvested, centrifuged and the pellet subjected to DNA extraction for further molecular characterization. To this sediment we gave the name ‘culture mass’. Positive hemocultures also point to infective potential to vectors since we were able to obtain parasites starting from 300 µL.
Positive cultures and/or culture mass were washed with phosphate-buffered saline solution and incubated with proteinase K (100 µg mL−1) and 0·5% of sodium dodecyl sulphate at 56 °C for 2 h. Then, DNA was extracted by the classical phenol–chloroform method (Vallejo et al. Reference Vallejo, Guhl, Chiari and Macedo1999) and then quantified in a NanoDrop 1000 Spectrophotometer (Thermo Scientific®), and the final concentration was adjusted to 50 ng µL−1.
Molecular characterization and phylogenetic analyses
A nested polymerase chain reaction (PCR) targeting the 18S rRNA gene (~600–800 bp) was performed as described by Noyes et al. (Reference Noyes, Stevens, Teixeira, Phelan and Holz1999) with external primers TRY927F (5′GAAACAAGAAACACGGGAG3′) and TRY927R (5′CTACTGGGCAGCTTGGA 3′) for 30 cycles at 94 °C for 30 s, 55 °C for 60 s and 72 °C for 90 s. The products from the first amplification were diluted 1:10 in sterile deionized water, and 2 µL was used as template for the second-round PCR with the following internal primers: SSU561F (5′TGGGATAACAAAGGAGCA3′) and SSU561R (5′CTGAGACTGTAACCTCAAAGC3′) using the same cycling conditions. The products derived from the second-round reaction were electrophoresed in a 2% agarose gel run at 90 V for 1·5 h in Tris-acetate EDTA buffer, which was stained with ethidium bromide and visualized by illumination with UV light. Six samples obtained from the first expedition were characterized by other primer pairs from the same 18S rRNA gene (V7V8) using PCR conditions described elsewhere (Borghesan et al. Reference Borghesan, Ferreira, Takata, Campaner, Borda, Paiva, Milder, Teixeira and Camargo2013).
Amplified PCR products were purified using Illustra GFX PCR DNA and a gel band purification kit (GE Healthcare Life Sciences, Little Chalfont, Buckinghamshire, UK). The purified PCR products were sequenced using the corresponding internal reverse primers diluted at 3·2 picomoles with ABI 3730 BigDye Terminator (v3·1) Cycle Sequencing Kit (Applied Biosystems, Foster City, CA, USA) on an Applied Biosystem DNA Analyzer on the PDTIS/FIOCRUZ sequencing platform.
Nucleotide sequences obtained from 18S rRNA were manually edited using the DNASTAR's Lasergene Sequence Analysis Software (Burland, Reference Burland, Misener and Krawetz2000) and aligned using CLUSTALW. The obtained sequences were compared with nucleotide sequences deposited in GenBank using the NCBI BLAST (Basic Local Alignment Search Tool) algorithm to identify Trypanosoma species and/or T. cruzi DTUs. Identification was confirmed when the obtained sequence had a minimum length of 400 bp, an e-value equal to zero, 98% coverage and a minimum of 97% of identity with reference sequences from GenBank. The evolutionary histories at 18S rRNA gene were inferred in Mega 7 (Kumar et al. Reference Kumar, Stecher and Tamura2016) by Maximum Likelihood (ML) using Kimura 2-parameter model of nucleotide substitution with gamma-distributed plus invariant sites (K2+G+I), with bootstrapping at 1000 replicates for nodal support. We used in the analysis reference strains from T. cruzi clade and Herpetomonas ztiplika as outgroup (Table S1).
Statistical analysis
The richness and prevalence of Trypanosoma species (and T. cruzi DTUs) were compared between species, sex and micro habitats, using the Chi-square statistical analysis. The test was performed using software R (Version 2.11.1, R Development Core Team, 2010) considering the level of significance (P < 0·05).
Ethical statements
Bat captures were licensed by the Chico Mendes Institute for Biodiversity Conservation Brazilian (ICMBio – SISBIO), license numbers 44089-1 and 47377-1. All procedures with the bats followed protocols that had been approved by the Ethics Committee of the Fiocruz Animal Use (LW81-12). The carcasses of euthanized animals were deposited as voucher specimens in the collection of the Mammalian Ecology Laboratory of the Acre Federal University (UFAC).
Results
Diversity and abundance of the Chiropteran fauna
The four field excursions resulted in the capture of 367 bat specimens that were included in three families (Phyllostomidae, Emballonuridae and Vespertilionidae), 23 genera and 32 species (Fig. 2). The number of captured bats in the two study areas, PZ and SC, did not differ significantly (P = 0·08). Moreover, the species composition of the chiropteran fauna was specific to each of the two areas (Fig. 2). Additionally, the bat species diversity was significantly higher in SC (n = 29) compared with PZ (n = 17) (P = 0·016). The Artibeus sp and Carollia sp predominated in both areas, and the first was slightly more abundant (35·9% vs 34·3%). The relative abundance of the Artibeus sp was significantly higher in PZ (49·2%) than in SC (18·1%) (P < 0·0001). In contrast, the relative abundance of Carollia sp bats was comparable in the two localities, with 32·6% in PZ and 39·7% in SC (P = 0·10). Data concerning the abundance of bat species in each area of both municipalities are listed in Table S2.
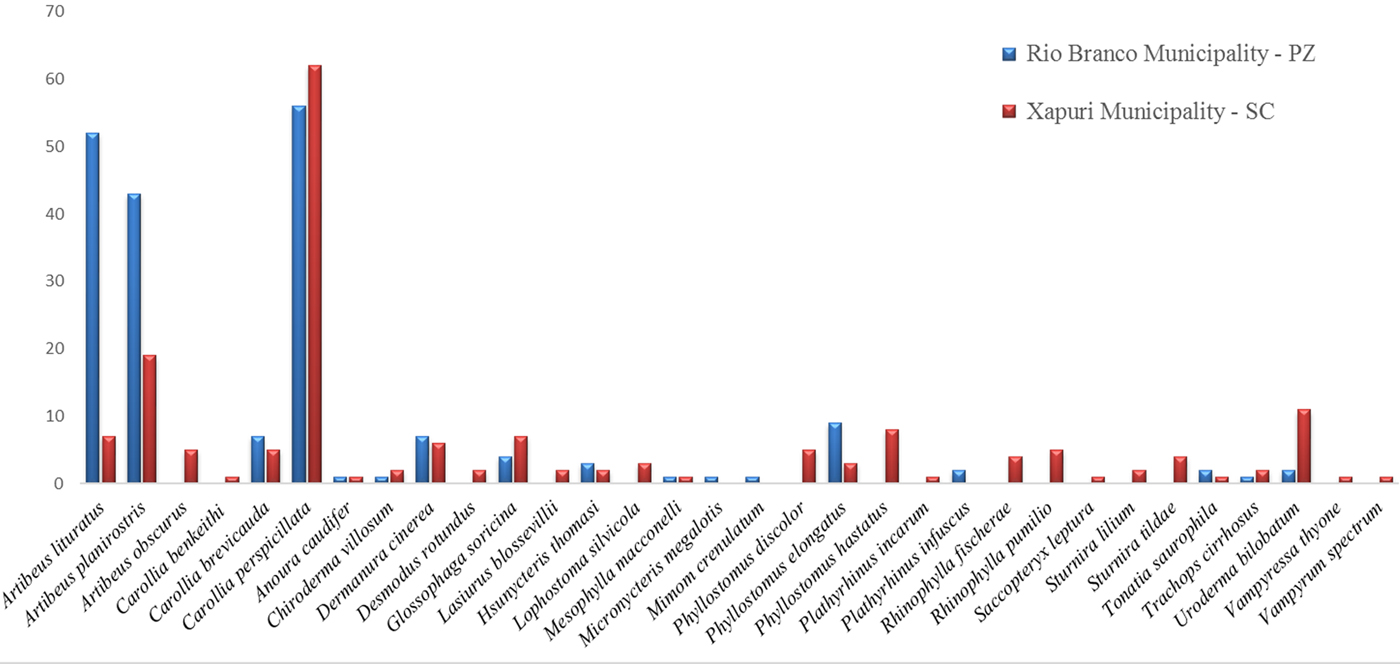
Fig. 2. Diversity and distribution of bats in two municipalities of Acre state, Rio Branco (Parque Zoobotânico –PZ) and Xapuri (Seringal Cachoeira – SC), in the Amazonian region of Brazil. Except for the species from the two most abundant genera (Artibeus and Carollia), the bat species are presented in alphabetical order.
Distribution of Trypanosoma sp. infection in Chiroptera of Acre State, Amazon Biome
Infection by Trypanosoma sp. was observed in 85 bats (23·2%); four of them were only observed by fresh blood examination, while 81 (22%) bats from 15 genera and 22 species displayed positive hemocultures (Table 1 and Table S2). Of these, 12 (14·8%) also displayed positive fresh blood examination. Infection by Trypanosoma sp. was three times higher in Chiroptera from SC (n = 60; 34·5%) in comparison with Chiroptera from PZ (n = 21; 10·9%) (P < 0·0001).
Table 1. Distribution of Trypanosoma sp. infection in Chiroptera from Rio Branco and Xapuri municipalities, Acre State
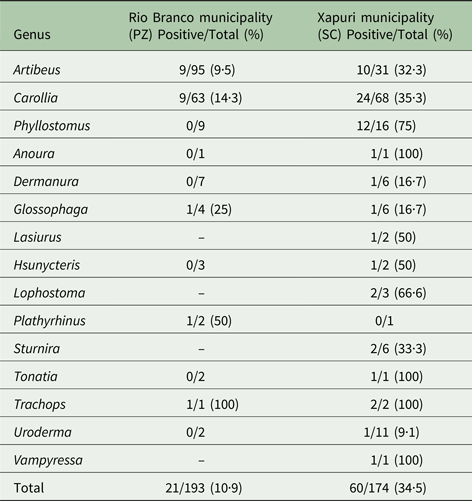
In PZ, the infectivity potential for vectors, expressed by positive hemocultures of the examined bats, was restricted to species from the two most abundant genera, Artibeus and Carollia (85·7%). A distinct enzootical picture was observed in SC, where a higher number of infected bats and overall higher bat species diversity were observed in all collected areas. Overall, the most common Trypanosoma sp. infected bat species was the generalist short tailed bat species Carollia perspicillata, wherein 16·3% were infected.
Of the 81 bats that were positive in the hemoculture, 68 isolates were obtained from 67 bats (two isolates were obtained from one C. perspicillata from PZ – LBT 7063). Three culture mass were also successfully characterized. Infection of the other 11 bats was only diagnosed by the morphological characteristics of the parasites observed in positive cultures.
The diversity of bat species in PZ was higher in area A2, where 12 distinct species could be distinguished among the 86 collected individuals. Additionally, the prevalence of Trypanosoma sp. infection was higher in this area (16·3%) compared with A1 (7·6%) and A3 (4·9%), although there was only a significant difference between A2 and A3 (P = 0·035). In SC, no differences in the Trypanosoma sp. infection were noted among the distinct areas (P = 0·305).
Trypanosoma sp. diversity infecting bats from Acre state
At least five different and one unidentified species and/or T. cruzi DTUs were observed in bats from Acre. Moreover, two mixed infections were also detected; one was identified in the same hemoculture (LBT 5060), and the other was identified in two different hemocultures from the same bat (LBT 7063). Trypanosoma cruzi TcI followed by T. c. marinkellei were the most widely distributed and prevalent trypanosomatid species (Tables 2 and 3). However, whereas T. cruzi TcI was found infecting bats in all areas, 11 of the 12 T. c. marinkellei isolates were derived from bats collected in the three SC areas. A similar feature was observed for T. dionisii wherein five of the six infected bats were from the same municipality. Trypanosoma cruzi TcIV was only detected in the most preserved areas from PZ (A1 and A2) and in SC, and the latter had co-infection with T. c. marinkellei. The two isolates that were characterized as T. rangeli belong to the genotype A and were derived from SC bats (C. perspicillata and Artibeus planirostris). One still-undescribed Trypanosoma species was isolated in co-infection with T. cruzi TcI in a bat from PZ (Tables 2 and 3). One of those samples clustered in the same branch as trypanosomes described in neotropical bats, between T. wauwau and Trypanosoma sp RNMO56 and 63 (Fig. 3). Although parasites were observed in another 11 positive hemocultures, those cultures were not established, and the parasites were characterized as Trypanosoma sp. based on their morphology in axenic cultures (Table 2).

Fig. 3. Phylogenetic inference of isolate LBT 7063 detected in Carollia perspicillata bat from Rio Branco municipality, Acre state, Brazil, in the T. cruzi clade. Tree construction from SSU rRNA followed the maximum likelihood (ML) method under Kimura's two-parameter model and gamma distributed with invariant sites (K2 + G + I). Numbers at nodes indicate support from 1000 bootstrap replicates. The isolate clustered into the T. cruzi clade associated with trypanosomes described in neotropical bats (Lima et al. Reference Lima, Espinosa-Alvarez, Pinto, Cavazzana, Pavan and Carranza2015). Trypanosoma sp H25, G8 and probably T. sp 15 and 17 have now been named T. noyesi (Botero et al. Reference Botero, Cooper, Thompson, Clode, Rose and Thompson2016)
Table 2. Distribution and diversity of Trypanosoma sp. in Chiroptera of Acre State, Amazon Biome according to distinct study areas and habitats

Characterization was performed using 18S SSU primers that were described by Noyes et al. (Reference Noyes, Stevens, Teixeira, Phelan and Holz1999).
a Morphologically identified.
Table 3. Identification numbers, bat species, locality and molecular characterization of the trypanosomatids using SSUrDNA

The last column includes the GenBank accession number of each deposited sequence.
N.D. not deposited in GenBank due to the presence of ambiguous sites.
a COLTRYP number codes of cultures deposited and cryopreserved in the Coleção de Trypanosoma de Mamíferos Silvestres, Domésticos e Vetores – COLTRYP (www.coltryp.fiocruz.br) of the Laboratório de Biologia de Tripanosomatídeos do Instituto Oswaldo Cruz – FIOCRUZ/RJ/Brazil.
b Characterized by V7V8 primer pairs (Borghesan et al. Reference Borghesan, Ferreira, Takata, Campaner, Borda, Paiva, Milder, Teixeira and Camargo2013).
c Parasites isolated from spleen culture.
The most abundant bat genera were Carollia sp. and Artibeus sp., but Phyllostomus sp. also displayed infections with the greatest diversity of Trypanosoma species (Table 4). Trypanosoma cruzi DTU TcI was the most dispersed Trypanosoma species and DTU. Only two of the 15 infected bat genera (Lophostoma and Sturnira) were not infected by T. cruzi TcI. Trypanosoma dionisii infected bats from four genera, including the most abundant bat genera, Carollia sp. T. marinkellei was mainly observed in Phyllostomus sp. (including one mixed infection with T. cruzi TcIV) as well as in Lophstoma sp. and Artibeus sp. Artibeus sp. was the taxon with the highest Trypanosoma sp species diversity, followed by the most abundant bat genera Carollia sp. and Phyllostomus sp (Table 4).
Table 4. Trypanosoma sp. diversity in bats from Acre State, Amazon Biome

Discussion
Bats are mammals that live for a long time, and their lifespan may easily reach more than two or even three decades (Wilkinson and South, Reference Wilkinson and South2002). Additionally, bats have high displacement capacity covering wide areas, which means that they are highly capable of dispersing parasites for a long time. The majority of the captured bats were included in 21 genera of Phyllostomidae, the ‘leaf nosed’ bat family that represent the most common and diverse bat taxon in Brazil and have a huge ecological importance. This family includes the three genera of hematophagous, as well as small predators of vertebrates, and consumers of pollen, fruits, nectar and insects. The latter correspond to an ancestral food item that is kept in the diet of almost all species of bats to a greater or lesser degree (Gardner, Reference Gardner, Baker, Jones, Knox and Carter1979; Rojas et al. Reference Rojas, Vale, Ferrero and Navarro2011; Carrillo-Araujo et al. Reference Carrillo-Araujo, Taş, Alcántara-Hernández, Gaona, Schondube, Medellín and Falcón2015). Considering that bats are voracious insect predators, it is very likely that bats may infect at least by T. cruzi, T. dionisii and T. rangeli by ingesting infected Triatomine vectors.
Even in the highly preserved SC area, we collected 29 bat species, which is far less than the 59 bat species Bernard et al. (Reference Bernard, Tavares and Sampaio2011) described as extant in Acre State. One possible reason may be that, although well preserved, SC has a history of logging, which may reduce roost offerings and adequate shelters for some species of bats. In addition, we collect in the sub-forest, which therefore decreases the chances of capturing the bats that fly in higher forest strata.
The PZ area, as expected, displayed a significantly lower number of bat species (n = 17). In fact, although it is an area of urban forest that is not frequented by tourists, it suffers anthropic action because there is a research institute in this area where people and vehicles circulate. Carollia sp seem to be well adapted to altered environments, as shown by the similar number of specimens of this taxon collected in both study areas. By contrast, Phyllostomus sp. was mainly collected in SC. In PZ, the generalist bat genus Artibeus predominated.
There are ecological traits of chiropterans that may enhance their exposure to Trypanosoma sp infection. In fact, bats can roost in several natural and artificial habitats and can even modify vegetation to form suitable roosting places as tents that are built by some bat genera. In all these distinct habitats bats may get in contact with triatomines (Stoner, Reference Stoner2000). Palm trees whose leaves are frequently used as refuges by bats, are classical habitat of Rhodnius specimens, an efficient T. cruzi vector that is very common in the Amazon region (Coura and Junqueira, Reference Coura and Junqueira2015). Considering that bats are voracious insect predators, it is very likely that tent building bats may be infected at least by T. cruzi and T. rangeli by ingesting infected vectors.
Bats may have a complex social structure that includes cooperative behaviour even among distinct groups. Cooperation includes roosting, taking care of offspring, grooming, foraging and feeding (Gardner, Reference Gardner and Gardner2007). Food sharing is especially evident in the three hematophagous bat genera. Sharing food from adult animals to their offspring is a common pattern of behaviour in the animal world and may favour parasite transmission. This may especially be the case for mammalian trypanosomatids if the bat meal consists of blood. Of note, blood-feeding bats share food between adults, i.e. adult individuals regurgitate the blood they obtained for both their puppies and adult bats that could not feed themselves (Wilkinson et al. Reference Wilkinson, Carter, Bohn and Adams2016). The care of offspring includes feeding other female pups and creating maintenance clusters of young as a crèche (Wilkinson et al. Reference Wilkinson, Carter, Bohn and Adams2016). Bats are excellent reservoirs of Trypanosoma sp. and probably the ancestral host of T. cruzi (Molyneux, Reference Molyneux, Kreier and Baker1991; Hamilton et al. Reference Hamilton, Cruickshank, Stevens, Teixeira and Mathews2012). Nevertheless, Trypanosoma sp. of bats is a little explored universe. In fact, the increase in the analytical power of the molecular tools has resulted in a growing number of new species of Trypanosoma sp. described in Chiropterans over the last several years. Chagas disease is currently a health threat in the Amazon region and in Acre State, where cases and outbreaks are becoming frequent; moreover, studies about Trypanosoma sp. infection of the mammalian fauna including bat species of Acre State are scarce.
Bats fidelity to their refuges is a factor that favours the establishment and maintenance of triatomine colonies. The association of Cavernicola pilosa with caves and T. cruzi and T. c. marinkellei transmission with bats is a nice example. Knowledge of how animal, especially bat, behaviour can alter and modulate the transmission of their parasites is still in the early stages.
Phyllostomidae constitutes a diverse group that includes generalist feeders such as Phyllostomus sp. as well as specialist feeders such as the hematophagous bat species Desmodus rotundus, essentially frugivores bat species Carollia perspicillata and Artibeus jamaicensis, and nectarivorous Glossophaga soricina. Moreover, insectivory is part of the diet to a greater or lesser extent for most bats (Carrillo-Araujo et al. Reference Carrillo-Araujo, Taş, Alcántara-Hernández, Gaona, Schondube, Medellín and Falcón2015). Phyllostomus sp. displayed a high infection rate (75%) by both T. cruzi and T. c. marinkellei, which were found in single and mixed infections, but Trypanosoma infection in this bat genera was only noted in SC. Although C. pilosa is considered the vector species of these two Trypanosoma species, also Rhodnius species may act as a vector based on it was successfully used in bat xenodiagnosis (Marinkelle, Reference Marinkelle, Lumdsen and Evans1976; Garcia et al. Reference Garcia, Ortiz, Osorio, Torrico, Torrico and Solari2012).
Carollia sp., which also displayed high Trypanosoma sp. infection rates, are considered resilient bats in terms of their diet (they feed on fruits and insects) and roosting sites, although they have also been described as understory specialists (Bernard, Reference Bernard2001). They live in colonies that may include hundreds of individuals that can be set in hollow trees, dense foliage, caves and tunnels, and they mainly forage at night. It is likely these bats acquire the Trypanosoma sp. infection in their roosting sites by ingesting triatomines or by the contaminative route because several of their roosting places are classical habitats of triatomines. This food and habit eclecticism in Carollia sp. probably explains the observation that this taxon not only had the highest rates of T. cruzi infection, but it also demonstrated a great diversity of Trypanosoma species that included T. rangeli, T. dionisii and at least one still-undescribed Trypanosoma species. Of note, we do not know if all trypanosomatids that could not be diagnosed at the species level constitute only a single species or include more than one species.
The diversity of Trypanosoma species that were found to infect bats from Acre state was astonishing, and further studies may reveal new aspects of Trypanosoma ecology and phylogeny. Additionally, Carollia, Artibeus and Phyllostomus were bat genres that also showed high rates of infection by a great diversity of Trypanosoma species. Concerning T. cruzi DTUs, bats were infected by TcI and TcIV, and both genotypes are associated with Chagas disease in the Amazon biome (Tables 3–4). In the infected bats, T. cruzi DTU TcI was found to infect all but two bat genera. This was an expected result because DTU TcI is ubiquitous in the transmission cycle of T. cruzi in the wild. Lophostoma sp. and Sturnira sp., two bat genera that were not infected by T. cruzi, harboured T. c. marinkellei and T. dionisii, respectively. The number of individuals of these two genera was very low, at only 3 and 2, respectively, and they were only collected in SC – the pristine vegetation area. Both Phyllostomidae and Sturnira are considered very abundant bat genera. Additionally, Lupinus silvicola is considered an abundant bat species. This species has a unique habitat because it is able to prepare and roost in cavities of active termite nests (Dechmann et al. Reference Dechmann, Kalko and Kerth2004).
In addition to Marinkelle's seminal description of T. rangeli infection in bats (Marinkelle, Reference Marinkelle, Lumdsen and Evans1976), there are only a few reports of infection by this taxon in chiropterans. Trypanosoma rangeli infecting Artibeus planirostris and Platyrrhinus lineatus bats was described by Maia da Silva et al. (Reference Maia da Silva, Marcili, Lima, Cavazzana, Ortiz, Campaner, Takeda, Paiva, Nunes, Camargo and Teixeira2009) in central Brazil. Moreover, the authors observed a new lineage of T. rangeli in P. lineatus that was named lineage E. Here, we expand the occurrence area of lineage A of T. rangeli in bats to the northern region of Brazil, i.e. the Amazon biome. This lineage is widely distributed and infects many mammalian host species; therefore, it would be expected to be found in bats from other biomes. It is interesting to note that the two isolates of T. rangeli were obtained from two bats (C. perspicillata and A. planirostris) of the best-preserved area (SC), where the diversity of bats and trypanosomes was higher. The sequence analysis grouped one isolate from a C. perspicillata (LBT 7063) between T. wauwau and Trypanosoma sp RMNO, described in Pteronotus and Tracops cirrhosus bats in northern Brazil (Lima et al. Reference Lima, Espinosa-Alvarez, Pinto, Cavazzana, Pavan and Carranza2015; da Costa et al. Reference da Costa, Nunes, Leite, Ferreira, Tonhosolo, da Rosa and Marcili2016). This result demonstrates that another trypanosome species is circulating in neotropical bats from Acre, reinforcing how little is known about the trypanosome diversity that circulate among bats. Even if it is an apparently well-preserved urban forest, the PZ area already shows a loss of bat and Trypanosoma sp. diversity, as demonstrated by the lower number of bat species captured and lower diversity of Trypanosoma species (Table 2).
The Trypanosomes that did not grow in culture media or presented with non-sustainable growth give a clue about how much remains to be known about the realm of bat trypanosomes without considering the trypanosomes that are not cultivable. In fact, there is still much to uncover about the assemblage of extant Trypanosoma species, their biology and ecology.
One phenomenon that is ripe for a thorough re-evaluation is host specificity in Trypanosomatids. It has been increasingly observed that numerous genotypes and species of Trypanosoma (Schizotrypanum) are far more eclectic with respect to their hosts. This is the case for Tcbat, a T. cruzi genotype that was exclusively associated with bats but has already been observed to infect humans (Ramírez et al. Reference Ramírez, Hernández, Montilla, Zambrano, Flórez and Parra2014), and for T. dionisii, a species of the subgenus T. (Schizotrypanum) that is usually associated with bats and has been detected in the cardiac tissue of a human individual who died from Chagas disease (Dario et al. Reference Dario, Rodrigues, da Silva Barros, das Chagas Xavier, D'Andrea, Roque and Jansen2016). Beyond parasites of medical or veterinary interest, trypanosomes are a fascinating model of the parasite–host interaction that constantly challenges and surprises us.
Supplementary material
The supplementary material for this article can be found at https://doi.org/10.1017/S0031182017001834
Acknowledgements
We acknowledge all people involved in the cooperation agreement between Oswaldo Cruz Institute and Acre Federal Institute as well as the Biologia Parasitária Post Graduation Program. The authors also thank Carlos Ardé and Marcos Antônio dos Santos Lima for technical support with the hemocultures, Dr Alena Iñiguez for the molecular characterization using V7V8 primer pairs, and the professionals from the laboratories of Tripanosomatid Biology and Biology and Parasitology of Wild Reservoir Mammals, Oswaldo Cruz Institute, who assisted us in the field expeditions.
Financial support
Cooperation agreement between IOC/FIOCRUZ and IFAC, CNPq (Edital Universal 2014 and PAPES/FIOCRUZ VII). ALRR is a ‘Jovem Cientista do Nosso Estado’ provided by FAPERJ. AMJ is a ‘Cientista do Nosso Estado’, provided by FAPERJ and financially supported by CNPq (‘Bolsista de Produtividade, nível 1’, CNPq).
Conflict of interest
The authors declare that they have no conflict of interest.