Introduction
Freshwater gastropods, a mollusc fauna belonging to class Gastropoda of phylum Mollusca, are well known for their global distribution and ability to live in a variety of freshwater habitats, such as natural and irrigation canals, ponds, water reservoirs, streams, rivers and agricultural areas (Bouchet et al., Reference Bouchet, Rocroi, Fryda, Hausdorf, Ponder, Valdes and Warén2005). Freshwater snails can also be found in a variety of microhabitat types within each habitat, depending on their foraging behaviours, which include climbing on rocks, crawling on the ground surface under the water, attaching to floating plants or objects, attaching to aquatic plants and climbing on the cement border of constructed ponds near the water surface level. They commonly graze for their food and are known to act as microherbivores, macroherbivores and carnivores in various habitats (Upatham et al., Reference Upatham, Kruatrachue, Chitramwong and Chantateme1995; Mekong River Commission, 2006). In ecosystems, freshwater snails can be consumed by other organisms, including fishes and aquatic birds, as well as humans. Some gastropod species are commonly consumed by humans in Thailand, especially groups of viviparids and ampullariids (apple snails).
Serving as an intermediate host of parasitic faunas is another important role of freshwater snails in ecosystems. Larval stages of many digenean trematodes utilize freshwater snails as their growth and development site, especially cercarial and metacercarial stages that have frequently been reported to be harboured in the snails. Common snail intermediate hosts of trematode larvae frequently belong to two major groups of gastropods, i.e. prosobranchs and pulmonates. Between these two snail groups, they have quite different biology in terms of their reproduction, growth and longevity. Prosobranchs are well known as a group of gill-breathing snails. The majority of them are dioecious and difficult to distinguish between sexes based on external morphology; nevertheless, hermaphrodites may be discovered in a few species. Growth patterns of prosobranchs might be uneven throughout the year or between years because females develop faster than males. For the longevity, it varies depending on different prosobranch species, ranging from 3 to 7 years and reaching 20 years for some species (Fretter and Graham, Reference Fretter and Graham1962). With respect to pulmonated snails, they are characterized by capability of air breathing. Generally, hermaphrodites with self-fertilization are frequently found in pulmonates; however, in some families such as Lymnaeidae, Planorbidae and Physidae they can be found with both self- and cross-fertilization. The growth rate of pulmonates was temperature-dependent and halted when the snails reached maturity. Life span of the pulmonates is shorter than the prosobranchs; they mostly inhabit for about one year and die after their spawning season. According to two gastropod groups stated above, Esch and Fernandez (Reference Esch and Fernandez1994) suggested that their biological variations in terms of reproductive biology, growth patterns and life span might contribute to their disparities in trematode community. In Thailand, more than 10 species/subspecies of freshwater snails have been reported to display their metacercarial infections, from several areas, particularly northern and northeastern parts; most of them frequently belong to prosobranch snails, including Anentome helena, Bithynia siamensis siamensis, Filopaludina doliaris, Filopaludina martensi martensi and Filopaludina sumatrensis polygramma (Chantima et al., Reference Chantima, Chai and Wongsawad2013, Reference Chantima, Suk-Ueng and Kampan2018; Noikong and Wongsawad, Reference Noikong and Wongsawad2014; Wongsawad et al., Reference Wongsawad, Intamong and Chantima2017; Chomchoei et al., Reference Chomchoei, Wongsawad and Nantarat2018; Phalee et al., Reference Phalee, Phalee and Wongsawad2018; Wiroonpan and Purivirojkul, Reference Wiroonpan and Purivirojkul2018; Bunchom et al., Reference Bunchom, Pilap, Suksavate, Vaisusuk, Suganuma, Agatsuma, Petney and Saijuntha2020; Butboonchoo et al., Reference Butboonchoo, Wongsawad, Wongsawad and Chai2020; Chantima and Rika, Reference Chantima and Rika2020; Wiroonpan and Purivirojkul, Reference Wiroonpan and Purivirojkul2021).
Some species of different trematode families have been known to utilize freshwater snails as their hosts for existence of their metacercarial stage, such as families Cyathocotylidae, Cyclocoelidae, Plagiorchiidae, Pronocephalidae, Psilostomatidae, Strigeidae and especially Echinostomatidae (Yoder and Coggins, Reference Yoder and Coggins1998; Wetzel and Shreve, Reference Wetzel and Shreve2003; Yurlova et al., Reference Yurlova, Vodyanitskaya, Serbina, Biserkov, Georgiev and Chipev2006). Previous studies have shown the metacercariae of family Echinostomatidae in freshwater snails from several countries, including metacercariae of Echinostoma infecting viviparid snails from Southeast Asian countries (Chai et al., Reference Chai, Sohn, Na and Van De2011, Reference Chai, Sohn, Cho, Jung, Chang, Lee, Khieu and Huy2021; Chantima et al., Reference Chantima, Chai and Wongsawad2013; Sohn et al., Reference Sohn, Chai, Na, Yong, Eom, Park, Min and Rim2013; Sohn and Na, Reference Sohn and Na2017; Sohn et al., Reference Sohn, Na, Lee, Eom, Yong, Chai and Min2019), metacercariae of Artyfechinostomum malayanum infecting Pila sp. (prosobranch) snails acquired from Cambodia (Sohn et al., Reference Sohn, Yong, Eom, Sinuon, Jeoung and Chai2017), metacercariae of Echinoparyphium aconiatum and Moliniella anceps infecting a great pond snail (Lymnaea stagnalis, pulmonate) from Central European countries (Faltýnková et al., Reference Faltýnková, Našincová and Kablásková2007, Reference Faltýnková, Našincová and Kablásková2008), and metacercariae of Hypoderaeum conoideum infecting lymnaeid, physid and planorbid of pulmonate snails from Spain (Toledo et al., Reference Toledo, Muñoz-Antoli and Esteban1999). In addition, the metacercariae belonging to other trematode families have also been recorded. Yoder and Coggins (Reference Yoder and Coggins1998) denoted a strigeid metacercaria of Cotylurus flabelliformis in a snail, Lymnaea stagnalis, from southeastern Wisconsin, United States, while a pronocephalid metacercaria of Macravestibulum obtusicaudum infecting a snail, Elimia livescens, was reported from Indiana, United States, by Wetzel and Shreve (Reference Wetzel and Shreve2003).
In Thailand, diversity of trematode metacercariae is relatively low for their infections in freshwater snail hosts; two groups of metacercariae, including echinostomes and thapariellids, have usually been reported from several areas (Anucherngchai and Chontananarth, Reference Anucherngchai and Chontananarth2016; Chantima et al., Reference Chantima, Suk-Ueng and Kampan2018; Phalee et al., Reference Phalee, Phalee and Wongsawad2018; Wiroonpan and Purivirojkul, Reference Wiroonpan and Purivirojkul2018, Reference Wiroonpan and Purivirojkul2021; Chantima and Rika, Reference Chantima and Rika2020). Research studies mentioning a group of echinostome metacercariae has mostly exhibited their occurrence and infection rate and categorized them depending on morphology, causing inability to clearly identify their species. Molecular methodology was implemented for species identification in a few previous studies. For instance, DNA regions of internal transcribed spacer 2 (ITS2) and NADH dehydrogenase subunit 1 (ND1) were applied to consider the species of echinostome metacercariae by Noikong et al. (Reference Noikong, Wongsawad, Chai, Saenphet and Trudgett2014) and Butboonchoo et al. (Reference Butboonchoo, Wongsawad, Wongsawad and Chai2020) while the random amplified polymorphic DNA (RAPD) was implemented in an investigation by Noikong and Wongsawad (Reference Noikong and Wongsawad2014) to evaluate genotype variations of echinostome metacercariae. For thapariellids, it has been quite rare for genetic investigations of this metacercarial group to be considered. Likewise, most of them have often been mentioned in terms of appearance and infection rate (Anucherngchai and Chontananarth, Reference Anucherngchai and Chontananarth2016; Chantima et al., Reference Chantima, Suk-Ueng and Kampan2018; Phalee et al., Reference Phalee, Phalee and Wongsawad2018; Chantima and Rika, Reference Chantima and Rika2020). Species identification of thapariellid metacercariae appears to be uncomplicated because of availability of their reproductive organs, for which the number, position and arrangement of these organs could facilitate assessing the species of many trematodes.
Bangkok is the capital and most urbanized area of Thailand. Almost all areas of Bangkok are provided with a penetration of canals and ponds because of the presence of a major river, the Chao Phraya River, settling in and dividing Bangkok into two major parts. In the realm of ecological functions, those canals and ponds could facilitate dispersion and diversity of various groups of aquatic faunas. Freshwater snails are one of the aquatic animals that are able to dwell in various microhabitats of water bodies in Bangkok. Moreover, canals and ponds in many areas of Bangkok are commonly situated as foraging areas for various vertebrate animals, such as some reptiles, aquatic birds and some mammals, that can act as definitive hosts of many digenean trematodes; a complete host component in the same ecosystem is represented, contributing to the complete life cycle and transmission success of trematode faunas. Meanwhile, Bangkok is one of the cities most visited by people from provincial areas and adjacent countries for work. Thus, it is possible that transmission of some endemic species of parasites into the Bangkok area could occur via the migration of those people. Considering a good distribution potential and an ability to harbour trematode larvae of freshwater snails, as well as a small amount of research mentioning their metacercarial infections in Bangkok, several biological aspects of the metacercariae were interesting and presented themselves to be investigated in our study, including infective situations in terms of prevalence and mean intensity, diversity, morphological features, and phylogenetic relationships.
Materials and methods
Snail specimen collection and exploration of metacercarial infections
A total of 59 locations of canals and ponds in the Bangkok area was designated as snail sampling sites. Coordinates of the global positioning system (GPS) of each site were recorded and mapped, as shown in Fig. 1. Snail specimens were randomly collected from each sampling site every two months using hand picking and a hand net following the counts per unit of the time-sampling method (Olivier and Schneiderman, Reference Olivier and Schneiderman1956) between March 2018 and February 2020, spending approximately 20 min with only one collector to collect the snails in each sampling site. The collected snails were kept within a perforated plastic bag and transported to a laboratory for temporary rearing in two-litre perforated plastic boxes with an appropriate proportion of the snail numbers per box. The shell morphological characteristics of snail specimens were mainly considered for their species/subspecies identification following the identification keys of Brandt (Reference Brandt1974) and Upatham et al. (Reference Upatham, Sormani, Kitikoon, Lohachit and Burch1983). An exploration of metacercarial infections in the snail specimens was performed based on the crushing and dissection techniques (Caron et al., Reference Caron, Rondelaud and Losson2008). Briefly, the snail shell was eliminated, and then the snail body was pressed within a pair of Petri dishes. Crushed snail tissue was brought under a stereomicroscope with high magnification to observe and search for the trematode metacercariae. The number of the snails examined and infected, as well as the numbers of metacercarial individuals, were counted and recorded for the calculations of prevalence and mean intensity of infections.

Fig. 1. Bangkok map showing 59 localities of snail sampling sites.
Morphological observations
Descriptive features of the investigated metacercariae were considered based on both live and fixed specimens. For the fixing process, 10% neutral-buffered formalin (NBF) was employed as a fixative reagent for preparing the metacercaria specimens to be in a relaxed posture that facilitated the evaluation of measurement characteristics. Live and fixed metacercarial specimens were observed for their thoroughly morphological features via a compound light microscope and then individually photographed using an Olympus BX51 compound light microscope with a DP70 camera (Olympus Corporation, Japan). Measurement characteristics of the metacercaria morphology were measured from the photomicrographs using an ImageJ program (Abramoff et al., Reference Abramoff, Magalhaes and Ram2004) and were shown in micrometre units (μm) as minimum–maximum (range), followed by the arithmetic mean within parentheses. Morphological measurements of each metacercaria's organs would be denoted in two dimensions, except for the prepharynx, esophagus and intestinal caeca, which are represented only in length.
Molecular studies
After obtaining metacercarial specimens from snail host's tissue, about 10–15 individuals of live metacercariae were suddenly preserved in a microcentrifuge tube provided with absolute ethanol using a glass dropper. Before DNA extraction, preserved metacercariae were rinsed twice using ultrapure water with centrifuging at 10 000 rpm for 1 min. Genomic DNA of the metacercariae was extracted using a GF-1 Tissue DNA Extraction Kit (Vivantis, Malaysia) following the manufacturer's protocol. To maintain DNA integrity, the extracted genomic DNA samples were kept at −20°C.
Using polymerase chain reaction (PCR), a region of ITS1-5.8S-ITS2 was expected to amplify from the metacercarial genomic DNA samples using a pair of BD1 as a forward primer (5′-GTC GTA ACA AGG TTT CCG TA-3′) and BD2 as a reverse primer (5′-TAT GCT TAA ATT CAG CGG GT-3′) following Morgan and Blair (Reference Morgan and Blair1995). A total volume of 25 μL for each reaction was carried out for the PCR amplification process, comprising 1× PCR buffer, 2 mm MgCl2, 0.4 μ m of each primer, 100 μ m dNTPs mixture, 2 units μL−1 Taq polymerase (Vivantis, Malaysia) and 3 μL of the metacercarial DNA template. PCR conditions were defined in a thermal cycler (Mastercycler Pro, Eppendorf, Germany) as follows: 94°C for 3 min of predenaturation, 40 cycles of denaturation at 94°C for 40 s, annealing at 60°C for 45 s and extension at 72°C for 1 min, and 72°C for 10 min for final extension. Each PCR product was separated for considering an expected size of the ITS1-5.8S-ITS2 region using gel electrophoresis, conducting with 1.5% agarose gel stained with SYBR safe (Invitrogen). Visualization of bands of the expected metacercarial DNA region within the gel was accomplished via Gel Doc™ XR+ imaging system (Bio-Rad Laboratories, USA). DNA purification and sequencing of the PCR products were carried out by Macrogen, South Korea; the same primer set used in the PCR was also applied for DNA sequencing via the Sanger sequencing method.
Regarding phylogenetic assessments of the metacercariae investigated, phylogenetic tree based on a partial sequence data of ITS1-5.8S-ITS2 region was constructed from eight sequences of the current metacercariae (~570–1030 base pairs) and 29 sequences of related digenean trematodes acquired from the GenBank database using the Bayesian Inference analyses; a sequence of an Aspidogastrea, Aspidogaster ijimai, was placed as an outgroup (Table 1). Before the construction, all novel sequences were trimmed for discarding the primer binding regions. Of each metacercarial sample, a couple of sequences obtained from the forward and reverse primers was assembled as a contig (a set of contiguous sequences) using CAP3 sequence assembly program (Huang and Madan, Reference Huang and Madan1999). Confirmation of the presence of an expected organism and ITS1-5.8S-ITS2 region of the metacercarial sequences assembled was considered using the megablast algorithm in the standard nucleotide basic local alignment search tool (BLAST) from the NCBI database. Sequence dataset of the current metacercariae and 29 related digenean trematodes, as well as an outgroup, was aligned together using ClustalW (Thompson et al., Reference Thompson, Higgins and Gibson1994). The best-fitting of nucleotide substitution model was evaluated based on the Akaike Information Criterion (AIC) via jModelTest version 2.1.10 (Guindon and Gascuel, Reference Guindon and Gascuel2003; Darriba et al., Reference Darriba, Taboada, Doallo and Posada2012). Bayesian Inference method was analysed via MrBayes version 3.2.7 software (Ronquist et al., Reference Ronquist, Teslenko, van der Mark, Ayres, Darling, Höhna, Larget, Liu, Suchard and Huelsenbeck2012) with 10 million generations of Markov chain Monte Carlo and every 1000 generations of a tree sample frequency. First 2500 trees would be eliminated as burn-in phase of 25%. The phylogenetic tree was viewed using FigTree version 1.4.4 and then decorated via MEGA X software (Kumar et al., Reference Kumar, Stecher, Li, Knyaz and Tamura2018). Genetic divergence between sequences was evaluated based on p-distance method of a pairwise distance analysis using MEGA X software (Kumar et al., Reference Kumar, Stecher, Li, Knyaz and Tamura2018). Novel sequences of metacercariae from our study were submitted to GenBank database (Table 1).
Table 1. Sequence data of ITS1-5.8S-ITS2 region of the current metacercariae and related trematodes acquired from GenBank database for the phylogenetic analyses

Results
Infective situations of metacercariae in freshwater gastropods
Our investigation of the metacercarial infection in freshwater gastropods collected in almost all areas of Bangkok with 59 sampling localities was accomplished based on a total of 21 707 snail individuals. Of those snails, infections with the metacercarial stage of digenean trematodes were observed in 3173 snails, demonstrating an overall infective prevalence of 14.62% (Table 2). The infected snails belonged to nine families, 12 genera and 14 species/subspecies, comprising nine species/subspecies of prosobranchs and five species of pulmonates (Table 2, Fig. 2). The highest prevalence of the infections was observed in a group of viviparid snails, with the highest infection in snail Idiopoma umbilicata (82.18%), followed by Filopaludina sumatrensis polygramma (31.69%) and Filopaludina sumatrensis speciosa (29.41%). In contrast, the lowest prevalence of the infection was revealed in snail Physella acuta (0.48%), followed by Tarebia granifera (0.65%) and Sulcospira housei (0.70%). Five freshwater snail species/subspecies were observed with no infection of the metacercariae: Cyclotropis carinata, Filopaludina martensi cambodiensis, Melanoides tuberculata, Sermyla riqueti and Thiara scabra (Table 2, Fig. 2). Regarding the larval trematodes investigated, seven groups of metacercariae were recorded to infect freshwater snails, including echinostome A, echinostome B, echinostome C, echinostome D, Opisthorchiata-like, renicolid and thapariellid. Infective prevalence was demonstrated with the highest for echinostome A (7.15%) and the lowest for echinostome D (0.01%) (Table 2). To consider the common intermediate host snails of the metacercariae currently investigated, two viviparid snails, Filopaludina martensi martensi and Filopaludina sumatrensis polygramma, were noted to carry the highest diversity of metacercariae; six out of all seven metacercarial groups were observed in each of these two snail taxa (Table 2). Concerning the infections between groups of prosobranch and pulmonate snails, four metacercariae (echinostome A, echinostome B, echinostome C and Opisthorchiata-like) were found to infect both prosobranchs and pulmonates while the remaining three groups (echinostome D, renicolid and thapariellid) infected only prosobranchs (Table 2).
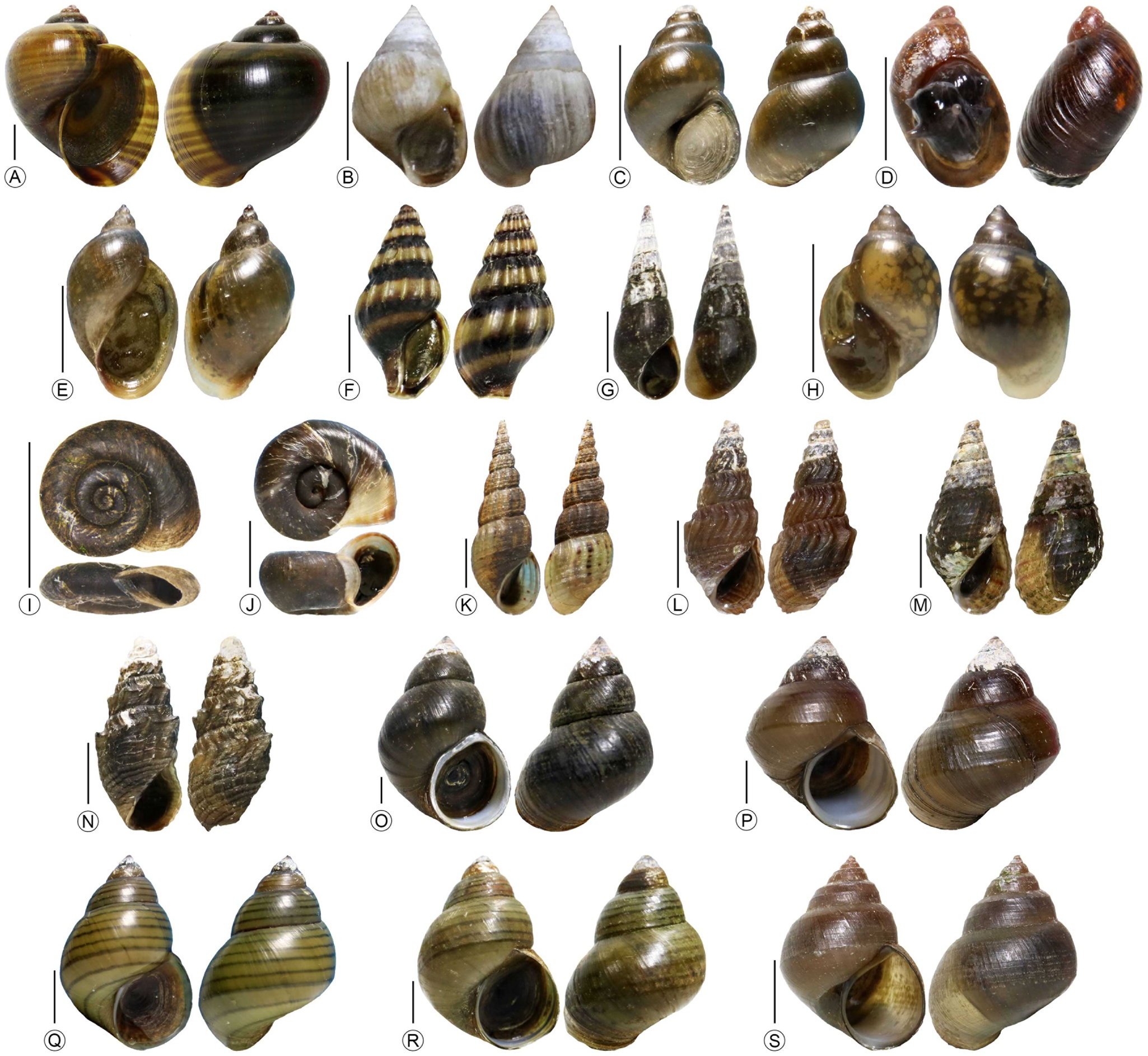
Fig. 2. All examined freshwater gastropods from Bangkok areas with 19 species/subspecies. (A) Pomacea canaliculata (B) Cyclotropis carinata (C) Bithynia siamensis siamensis (D) Austropeplea viridis (E) Radix auricularia (F) Anentome helena (G) Sulcospira housei (H) Physella acuta (I) Gyraulus siamensis (J) Indoplanorbis exustus (K) Melanoides tuberculata (L) Sermyla riqueti (M) Tarebia granifera (N) Thiara scabra (O) Filopaludina martensi cambodiensis (P) Filopaludina martensi martensi (Q) Filopaludina sumatrensis polygramma (R) Filopaludina sumatrensis speciosa (S) Idiopoma umbilicata. Scale bars: 5 mm.
Table 2. Freshwater snails investigated and their prevalence and mean intensity of the metacercarial infections

Numbers of infected snails and metacercarial individuals of each metacercaria group were represented within parentheses following the prevalence and mean intensity, respectively.
In the cases of synchronous infections, occurrences of the double and triple metacercarial infections were revealed from 395 snail individuals, demonstrating the infective prevalence of 1.82% (395/21 707); almost all of those snails were viviparid snails (Table 3). For double infections, a total of 360 snails were observed to be infected with two groups of the metacercariae at the same time. Twelve couples of the metacercariae were observed (Table 3); a couple of renicolid and echinostome A demonstrated the highest occurrence with 154 individuals of three snail taxa (26 of Filopaludina martensi martensi, 109 of Filopaludina sumatrensis polygramma and 19 of Idiopoma umbilicata), while a couple of echinostome D and echinostome A occurred in only two individuals of Filopaludina sumatrensis polygramma. Regarding triple infections, the concurrent infections of three metacercarial groups were recorded in 35 snail individuals. Five sets of the triple infections were currently categorized (Table 3). The highest occurrence was displayed in a set of Opisthorchiata-like, renicolid, and echinostome A with 19 snail individuals of four taxa (two of Filopaludina martensi martensi, ten of Filopaludina sumatrensis polygramma, two of Filopaludina sumatrensis speciosa and five of Idiopoma umbilicata). In contrast, two sets of the metacercariae, including a set of echinostome B, renicolid and echinostome A, and a set of Opisthorchiata-like, renicolid and thapariellid, were individually found in each of two snail individuals of Filopaludina sumatrensis polygramma and Filopaludina martensi martensi, respectively.
Table 3. Concurrent infections of the metacercariae in freshwater snails with the number of snails infected

Morphological descriptions of each metacercaria
Seven groups of trematodes metacercariae were discovered herein, categorized based on their morphological features. Of those, the investigated metacercariae were roughly categorized into two major groups, relying on an appearance of an oral sucker surrounded by the collar spines [i.e. collar-spined metacercariae (Fig. 3) and non-collar-spined metacercariae (Fig. 4)]. Echinostome was named for a group of the metacercaria specimens provided with the collar spines; four subgroups of them were characterized with their differently morphological characteristics and each subgroup was simply named and suffixed by the alphabet for convenience, namely echinostome A, echinostome B, echinostome C and echinostome D. Regarding another major group, three subgroups of the metacercariae were observed without an existence of the collar spines: Opisthorchiata-like, renicolid and thapariellid. Each of the seven metacercarial groups would be further detailed for their several biological features below, such as general morphology of both descriptive and measured characteristics, snail host range and prevalence and mean intensity of the infection. The prevalence and mean intensity of the infections of each metacercaria would respectively be placed within parentheses that followed each infected snail's specific name.

Fig. 3. General morphology of four groups of the collar-spined metacercariae based on living specimens. Echinostome A with (A) the metacercarial individual and (B) showing infections within snail's heart tissue, (C) echinostome B, (D) echinostome C, and echinostome D with (E) the metacercarial individual and (F) showing an existence of the metacercarial stage inside their redial stage. Abbreviations are as follows: C, caecum; CS, collar spine; CW, cyst wall; E, esophagus; EB, excretory bladder; EG, excretory granule; M, metacercaria; MCT, main collecting tube; OS, oral sucker; P, pharynx; PP, prepharynx and VS, ventral sucker.
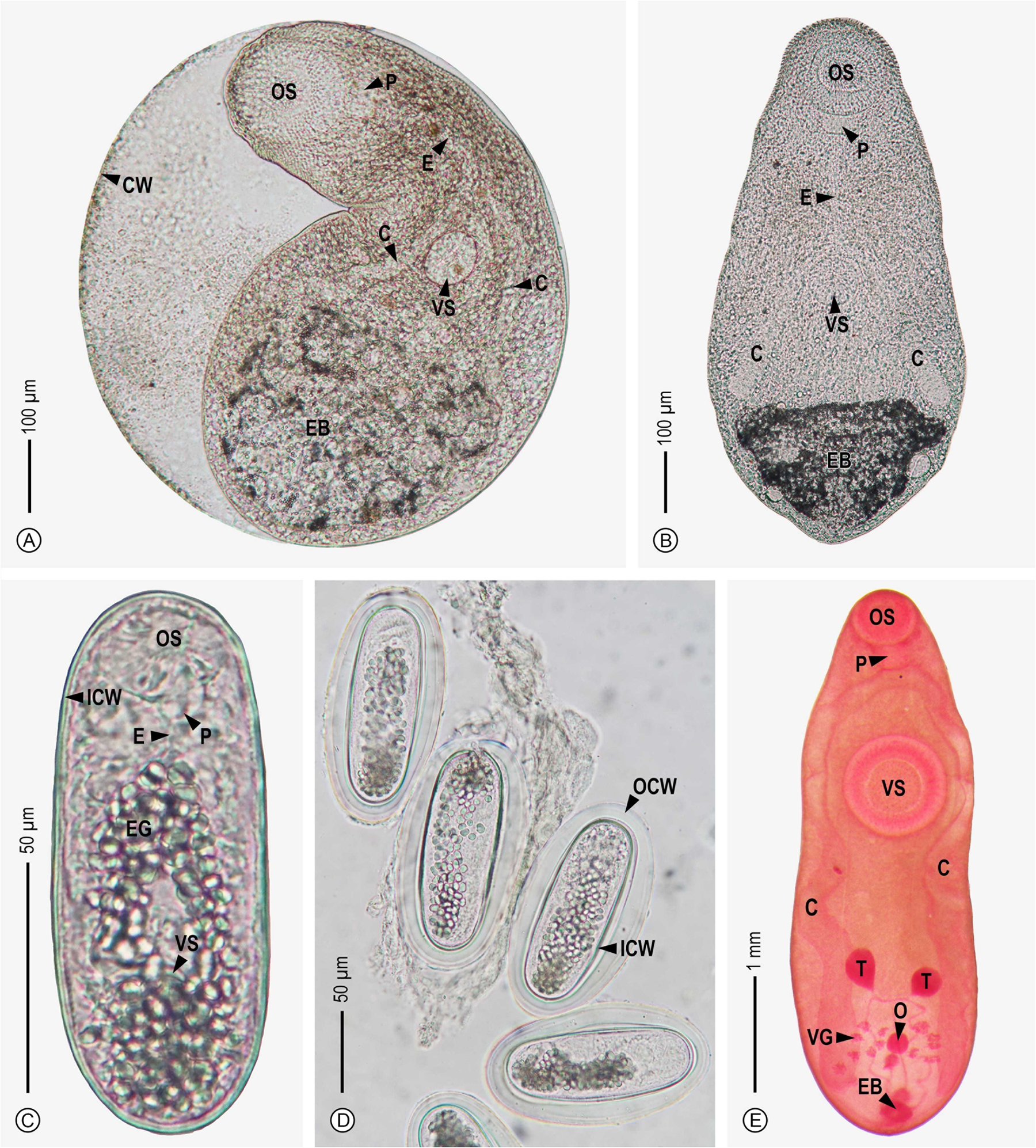
Fig. 4. General morphology of three groups of the non-collar-spined metacercariae based on living specimens, excluding thaparielid. Opisthochiata-like with (A) encysted and (B) excysted forms, renicolid with (C) the metacercarial individuals and (D) showing the cysts enveloped by the outer wall and (E) a stained specimen of thapariellid. Abbreviations are as follows: C, caecum; CW, cyst wall; E, esophagus; EB, excretory bladder; EG, excretory granule; ICW, inner cyst wall; O, ovary; OCW, outer cyst wall; OS, oral sucker; P, pharynx; T, testis; VG, vitelline glands and VS, ventral sucker.
Echinostome A
Specimens of the echinostome A metacercaria were usually observed as globular-shaped, and their cyst walls consisted of two layers (Fig. 3A and B). Oral and ventral suckers are well developed. The oral sucker is positioned at the subterminal region of the anterior body. Collar spines were distinctly observed surrounding the oral sucker; 37 spines were counted, with clumping of five spines at terminal ends. The prepharynx was observed with short length. The pharynx is quite large and vertically sub-elongated. The ventral sucker is placed at around the one-third region, measured from the posterior body, and is distinctively larger than the oral sucker. The main collecting tubes of the excretory system are situated along lateral body sides from the level of the oral sucker to the posterior body, filled with circular and rather large excretory granules near the part of the posterior body. Concerning the infected organ, echinostome A was usually found in the heart tissue of snail hosts (Fig. 3B). Several morphological characteristics measurements were carried out based on 21 specimens, as follows: 170–232 (206) μm × 171–234 (205) μm of cyst, 24–64 (42) μm × 30–69 (48) μm of oral sucker, 37–82 (60) μm × 36–88 (62) μm of ventral sucker, 24–25 (25) μm of prepharynx length, 13–41 (28) μm × 15–28 (20) μm of pharynx and 52–74 (63) μm of esophagus length. The following 13 freshwater snails were parasitized by the echinostome A metacercaria, namely Anentome helena (6.24%, 8.03), Austropeplea viridis (9.38%, 2.00), Bithynia siamensis siamensis (2.83%, 7.03), Filopaludina martensi martensi (5.84%, 7.37), Filopaludina sumatrensis polygramma (20.11%, 14.58), Filopaludina sumatrensis speciosa (9.80%, 13.20), Gyraulus siamensis (2.58%, 12.38), Idiopoma umbilicata (60.40%, 14.93), Physella acuta (0.36%, 2.00), Pomacea canaliculata (1.78%, 2.96), Radix auricularia (7.20%, 5.96), Sulcospira housei (0.70%, 1.00) and Tarebia granifera (0.41%, 8.90) (Table 2).
Echinostome B
Cysts of the echinostome B metacercaria were observed as spherical, with a thin wall (Fig. 3C). Muscular oral and ventral suckers were observed; the oral sucker is placed at the subterminal region of the anterior body and is prominently larger than the ventral sucker. Ambiguous collar spines were observed and are positioned surrounding the oral sucker. A prepharynx was observed. The pharynx is quite large and vertically oval. A relatively long esophagus is apparent. The main collecting tubes of the excretory system were obvious, filled with circular excretory granules and ranging from the oral sucker level to terminate near the excretory bladder that is situated at the posterior body. The measured characteristics were performed using 14 specimens and showed as follows: 102–140 (125) μm × 103–138 (122) μm of cyst, 28–62 (43) μm × 40–58 (48) μm of oral sucker, 21–46 (35) μm × 24–48 (41) μm of ventral sucker, 14–21 (17) μm of prepharynx length, 12–23 (18) μm × 9–20 (14) μm of pharynx, and 44–45 (44) μm of esophagus length. The metacercariae of echinostome B were found to exist in six freshwater snails [i.e. Anentome helena (0.43%, 1.50), Bithynia siamensis siamensis (0.20%, 9.00), Filopaludina martensi martensi (3.05%, 4.81), Filopaludina sumatrensis polygramma (3.81%, 8.87), Pomacea canaliculata (0.22%, 4.67) and Radix auricularia (0.22%, 30.67) (Table 2)].
Echinostome C
The shape of the echinostome C metacercaria was usually observed as subglobular to oval (Fig. 3D). Slightly different sizes between the oral and ventral suckers were observed. The oral sucker is situated at the subterminal region of the anterior body and surrounded by the collar spines. The pharynx is quite large and vertically elliptical. A long esophagus was observed to branch into two intestinal caeca. The ventral sucker is placed near the middle of the body length. The main collecting tubes were distinctly observed to sinuously lay from the oral sucker level to reach the excretory bladder, containing circular excretory granules and relatively sizable oval excretory granules. The excretory bladder is triangular and located at the posterior end of the body. Measured characteristics of the metacercaria morphology were evaluated based on 15 specimens and listed as follows: 156–185 (167) μm × 115–155 (141) μm of cyst, 30–45 (36) μm × 42–67 (48) μm of oral sucker, 27–49 (39) μm × 40–64 (50) μm of ventral sucker, 16–27 (21) μm × 12–22 (18) μm of pharynx, 16–67 (32) μm of esophagus length, 57–121 (87) μm of caecum length and 17–38 (23) μm × 22–71 (45) μm of excretory bladder. An infection of this metacercarial group was revealed in the six following freshwater snails: Anentome helena (0.65%, 10.67), Bithynia siamensis siamensis (0.26%, 15.75), Filopaludina martensi martensi (0.08%, 19.67), Indoplanorbis exustus (14.16%, 41.10), Radix auricularia (5.07%, 20.29) and Tarebia granifera (0.25%, 33.33) (Table 2).
Echinostome D
Based on our laboratory experience, metacercariae of the echinostome D (Fig. 3E and F) were always found to exist within the redial stage (Fig. 3F) and their shape is sub-elongated or elliptical. The appearance of both oral and ventral suckers was observed. The oral sucker is surrounded by collar spines and placed at the subterminal region of the anterior body. Notably, two terminal corners of the collar spines are clumped by the four sizable spines. The prepharynx is relatively long. The pharynx is vertically oval. The esophagus is long and separated into two intestinal caeca. The main collecting tubes of the excretory system are situated along the lateral body sides from the oral sucker level to reach an excretory bladder that is placed at the posterior body; those tubes contain circular excretory granules, especially the two-thirds and third parts of the body measured from the anterior body. The following measured characteristics of the metacercarial morphology were carried out on six specimens: 152–162 (156) μm × 88–99 (95) μm of cyst, 20–30 (24) μm × 30–43 (36) μm of oral sucker, 21–45 (31) μm × 23–47 (34) μm of ventral sucker, 15–32 (22) μm of prepharynx length, 14–21 (17) μm × 10–17 (13) μm of pharynx, 29–44 (37) μm of esophagus length and 49–52 (50) μm of caecum length. There is only one taxon of snails, Filopaludina sumatrensis polygramma that was infected by this metacercarial group, with 0.06% of an infective prevalence (Table 2).
Opisthorchiata-like
The specimens of this metacercarial group are large in size compared with other encystment metacercariae investigated herein (Fig. 4A and B). A thin and weak cyst wall was observed; the cyst shape was globular to elliptical. The larval trematode body is loosely contained inside the cyst (Fig. 4A). The body of the larvae is elliptical and rather obtuse at the posterior body, covered with triangular spines, especially the part of the anterior body (Fig. 4B). Oral and ventral suckers are available; the oral sucker is situated at the subterminal region of the anterior body and is obviously larger than the ventral sucker that is placed at the middle of the body length. A prepharynx was not observed. The pharynx is quite large and horizontally oval. The long esophagus is bifurcated into two intestinal caeca that extend to terminate near the excretory bladder. The excretory bladder is triangular or semicircular in shape, distinctively large in size, positioned at the posterior body, and filled with fine, dark excretory granules. Several measured morphological characteristics were evaluated based on eight specimens and are listed as follows: 737–774 (751) μm × 623–724 (685) μm of cyst, 690–1015 (859) μm × 246–477 (357) μm of body of excysted specimens, 114–166 (136) μm × 112–215 (156) μm of oral sucker, 63–120 (85) μm × 65–152 (93) μm of ventral sucker, 31–59 (43) μm × 34–66 (52) μm of pharynx, 103–244 (171) μm of esophagus length, 251–411 (331) μm of caecum length, and 115–267 (190) μm × 168–412 (284) μm of excretory bladder. The Opisthorchiata-like metacercaria was recorded to parasitize in eight freshwater snails: Anentome helena (1.08%, 1.00), Bithynia siamensis siamensis (0.07%, 1.00), Filopaludina martensi martensi (10.75%, 2.91), Filopaludina sumatrensis polygramma (4.26%, 1.80), Filopaludina sumatrensis speciosa (12.75%, 1.77), Idiopoma umbilicata (17.82%, 1.78), Physella acuta (0.12%, 2.50) and Pomacea canaliculata (0.15%, 1.00) (Table 2).
Renicolid
Metacercariae of the renicolid (Fig. 4C and D) are rod-shaped or elongated, and their cyst walls consist of two layers; the outer layer is thin, weak and flexible and thick-spaced (Fig. 4D). Oral and ventral suckers are present; the oral sucker is located at the subterminal region of the anterior body, while the ventral sucker is near the third part of the body, measured from the anterior body. No prepharynx was observed. The pharynx is relatively large. The esophagus displays with short length and is branched into two intestinal caeca. Relatively large excretory granules with spherical to oval shapes within the main collecting tubes are densely scattered in the two-thirds part and the third part of the body measured from the anterior. However, several organs, including the esophagus, intestinal caeca, ventral sucker and excretory bladder of those metacercarial specimens, were usually difficult to observe because of a covering of dense excretory granules. The following measurement characteristics were demonstrated using 39 specimens: 128–164 (142) μm × 62–91 (76) μm of cyst (outer thick layer included), 110–133 (118) μm × 37–52 (45) μm of cyst (the outer excluded), 10–26 (19) μm × 16–31 (24) μm of oral sucker, 14–29 (17) μm × 12–28 (18) μm of ventral sucker, and 5–9 (8) μm × 5–10 (8) μm of pharynx. Metacercarial specimens of the echinostome D were only discovered to infect a group of viviparid snails, namely Filopaludina martensi martensi (4.19%, 137.94), Filopaludina sumatrensis polygramma (7.27%, 63.70), Filopaludina sumatrensis speciosa (10.78%, 13.55) and Idiopoma umbilicata (44.55%, 20.38) (Table 2).
Thapariellid
Metacercariae of this group can be identified to a species level as Thapariella anastomusa based on their morphological characteristics (Fig. 4E). The metacercarial specimens were observed as a non-encysted form with a fusiform shape. Live specimens of this metacercaria can be observed by the naked eye as brownish red. Oral and ventral suckers appear as spherical shaped. The oral sucker is located at the subterminal region of the anterior body. A prepharynx and esophagus are absent. The pharynx is large and muscular. Bifurcated intestinal caeca are placed along the lateral body sides from the pharynx level to near the posterior end of the body. The ventral sucker is prominently larger than the oral sucker and is situated near the one-third part measured from the anterior body. Concerning the posterior body, several reproductive organs were observed. The testes are spherical to oval and positioned in front of the ovary and vitelline glands. The ovary is circular and surrounded by groups of vitelline glands. These vitelline glands are clumped together in several groups that display as polygonal in shape. The excretory bladder is elliptical and situated at the posterior end of the body and appears to be small in size. All measured morphological characteristics were evaluated based on 16 specimens, as follows: 2349–6099 (3851) μm × 772–2073 (1356) μm of body, 321–580 (445) μm × 362–649 (501) μm of oral sucker, 488–999 (753) μm × 467–966 (747) μm of ventral sucker, 160–361 (246) μm × 194–390 (284) μm of pharynx, 1690–5354 (3213) μm of caecum length, 136–461 (285) μm × 95–341 (226) μm of testis, 78–253 (168) μm × 61–214 (147) μm of ovary, and 103–328 (191) μm × 30–260 (113) μm of excretory bladder. Three viviparid snails were found to be infected by this metacercarial group: Filopaludina martensi martensi (5.09%, 2.30), Filopaludina sumatrensis polygramma (1.47%, 1.75) and Idiopoma umbilicata (1.98%, 2.50) (Table 2).
Quick identification key of the metacercariae investigated
A quick dichotomous identification key of the metacercariae investigated was constructed and demonstrated as below for showing overall discrimination in determining the prominent features of each metacercarial group.

Phylogenetic relationships and molecular identification
From our seven groups of the metacercariae that are morphologically categorized, five of them were successfully amplified and sequenced for obtaining their DNA sequences of the ITS1-5.8S-ITS2 region, excluding two groups of echinostome D and thapariellid. Following the phylogenetic tree (Fig. 5) based on the ITS1-5.8S-ITS2 region of the current metacercariae and their related trematodes using Bayesian inference analyses, general topology of the phylogram displayed that eight novel sequences from the five metacercarial groups investigated were clustered together as a monophyletic group by applying a sequence of Aspidogastrea, Aspidogaster ijimai, as an outgroup. A sequence data set of the current eight metacercariae and 29 related digenean trematodes was considered to be grouped into the three following major clades: a clade of superfamily Microphalloidea, a clade of suborder Opisthorchiata and a clade of superfamily Echinostomatoidea. A sequence of renicolid metacercaria (32.2KSW1 obtained from Filopaludina sumatrensis polygramma snail) was closely grouped with a clade of two sequences of the family Renicolidae (clade A). For the group of Opisthorchiata-like metacercaria, two current sequences (8KS1 and 10KS1 from the same snail species, Filopaludina martensi martensi) were clustered together with a high posterior probability value, and this clade was arranged to closely relate with a clade of the suborder Opisthorchiata; those two current sequences showed an identical sequence with 0.0% of genetic divergence. Five sequences of the current echinostome metacercariae, subdivided into three morphological groups, displayed an arrangement of the relationship with other echinostomatid species in three different patterns (clade E). Two current sequences of the echinostome A metacercaria (63NC1 and 12NC3 from the same snail species, Filopaludina sumatrensis polygramma) were clustered together and displayed the identical sequences with 0.0% genetic divergence. A clade of 63NC1 and 12NC3 was closely correlated with a sequence of Echinostoma mekongi (MT409016.1), with a high support value of posterior probability. The genetic divergences between sequences of a couple of 63NC1 and Echinostoma mekongi (MT409016.1) and a couple of 12NC3 and Echinostoma mekongi (MT409016.1) were 0.7 and 0.8%, respectively. Regarding echinostome B metacercaria (46TLC2 and 12TK2 from the same snail species, Filopaludina sumatrensis polygramma), two sequences of 46TLC2 and 12TK2 were grouped together; 0.0% genetic divergence revealed the identical sequences between 46TLC2 and 12TK2. A clade of these two sequences was closely related to a sequence of Euparyphium albuferensis (AJ564384.1) with high probability support. The genetic divergence between 46TLC2 and Euparyphium albuferensis (AJ564384.1) was 0.9%, while divergence between a pair of 12TK2 and Euparyphium albuferensis (AJ564384.1) was 0.0%. For the echinostome C metacercaria, a sequence of 6.2DM2 (from Indoplanorbis exustus) was arranged to highly relate with a clade of genus Echinostoma, demonstrating no close relationship to specific digenean trematode species.

Fig. 5. Phylogenetic tree of ITS1-5.8S-ITS2 region of the current metacercariae (bold and colored taxa) and relevant trematodes using Bayesian Inference analyses. A support value of posterior probability was attached at each node. Scale bar measured the branch length as expected number of substitutions per site. Alphabets showing actual trematode families, as follows: A: Renicolidae; B: Cryptogonimidae; C: Heterophyidae; D: Opisthorchidae; E: Echinostomatidae and F: Echinochasmidae.
Discussion
Investigations of trematode metacercariae infecting freshwater animals in Thailand have usually been performed in fish hosts (Wongsawad et al., Reference Wongsawad, Rojanapaibul, Mhad-arehin, Pachanawan, Marayong, Suwattanacoupt, Rojtinnakorn, Wongsawad, Kumchoo and Nichapu2000; Mard-arhin et al., Reference Mard-arhin, Prawang and Wongsawad2001; Nithiuthai et al., Reference Nithiuthai, Suwansaksri, Wiwanitkit and Chaengphukeaw2002; Krailas et al., Reference Krailas, Janecharat, Ukong, Junhom, Klamkhlai, Notesiri and Ratanathai2004, Reference Krailas, Veeravechsukij, Chuanprasit, Boonmekam and Namchote2016; Kumchoo et al., Reference Kumchoo, Wongsawad, Chai, Vanittanakom and Rojanapaibul2005; Pinlaor et al., Reference Pinlaor, Onsurathum, Boonmars, Pinlaor, Hongsrichan, Chaidee, Haonon, Limviroj, Tesana, Kaewkes and Sithithaworn2013; Suanyuk et al., Reference Suanyuk, Mankhakhet, Soliman, Saleh and El-Matbouli2013; Namsanor et al., Reference Namsanor, Kiatsopit, Laha, Andrews, Petney and Sithithaworn2020; Patarwut et al., Reference Patarwut, Chontananarth, Chai and Purivirojkul2020; Charoensuk et al., Reference Charoensuk, Ribas, Chedtabud and Prakobwong2022); on the other hand, freshwater snails have occasionally been mentioned as intermediate hosts of the metacercariae (Chantima et al., Reference Chantima, Chai and Wongsawad2013, Reference Chantima, Suk-Ueng and Kampan2018; Noikong et al., Reference Noikong, Wongsawad, Chai, Saenphet and Trudgett2014; Phalee et al., Reference Phalee, Phalee and Wongsawad2018; Bunchom et al., Reference Bunchom, Pilap, Suksavate, Vaisusuk, Suganuma, Agatsuma, Petney and Saijuntha2020). In terms of biodiversity, the current study demonstrated a variety of metacercarial trematode larvae infecting snail intermediate hosts in Bangkok areas, as well as a variety of gastropod faunas distributed in freshwater habitats in Bangkok. A relatively high overall prevalence of the metacercariae was demonstrated in our study (14.62%) in comparison to previous studies that explored larval trematode infections in various freshwater snails from different parts of Thailand; for example, Bunchom et al. (Reference Bunchom, Pilap, Suksavate, Vaisusuk, Suganuma, Agatsuma, Petney and Saijuntha2020) denoted prevalence of the metacercarial infections in six snail species with 6.63% (249/3757) from canals, ponds and paddy fields in Maha Sarakham Province (northeastern Thailand), while Chantima et al. (Reference Chantima, Suk-Ueng and Kampan2018) showed 8.22% (138/1679) from their exploration in 11 snail species/subspecies from Mae Lao agricultural areas of Chiang Rai Province (northern Thailand). It is interesting that a higher infective situation was observed in the residential or urbanized areas, as characterized in this study. Changes in some parameters of water quality in urbanized habitats that promote colonization of freshwater snails are a possible reason for this result. A study by VanAcker et al. (Reference VanAcker, Lambert, Schmitz and Skelly2019) showed that parasitism of larval echinostome trematodes in snails can be increased with a gradient of residential or suburban land use, and mentioned that the infective rate was positively correlated with some water-quality parameters, such as total phosphorus that has been known to enhance the survivorship and reproduction of snails. Therefore, the good colonization of the snail faunas based on some water-quality parameters of water bodies in residential or urbanized areas probably resulted in an increase in the chance of encounters between the snails and parasites, leading to the high infection that was observed.
Regarding the investigated infected snails, all of them belonged to 14 species/subspecies and represented a high number of infected snail taxa when compared with previous studies that reported metacercarial infections in freshwater snails from Thailand (Chantima et al., Reference Chantima, Chai and Wongsawad2013, Reference Chantima, Suk-Ueng and Kampan2018; Noikong and Wongsawad, Reference Noikong and Wongsawad2014; Wongsawad et al., Reference Wongsawad, Intamong and Chantima2017; Chomchoei et al., Reference Chomchoei, Wongsawad and Nantarat2018; Phalee et al., Reference Phalee, Phalee and Wongsawad2018; Wiroonpan and Purivirojkul, Reference Wiroonpan and Purivirojkul2018; Bunchom et al., Reference Bunchom, Pilap, Suksavate, Vaisusuk, Suganuma, Agatsuma, Petney and Saijuntha2020; Butboonchoo et al., Reference Butboonchoo, Wongsawad, Wongsawad and Chai2020; Chantima and Rika, Reference Chantima and Rika2020; Wiroonpan and Purivirojkul, Reference Wiroonpan and Purivirojkul2021). Of the 14, the nine following taxa have never been known to be infected by metacercarial trematodes from those previous reports and can be addressed as the new host record of the metacercarial infections in Thailand: Austropeplea viridis, Filopaludina sumatrensis speciosa, Gyraulus siamensis, Indoplanorbis exustus, Physella acuta, Pomacea canaliculata, Radix auricularia, Sulcospira housei and Tarebia granifera; this finding expanded an infective database in terms of the snail host spectrum and indicated an important role and involvement of the snail faunas in trematode transmissions among host organisms in ecosystems. In terms of overall metacercarial infections among all infected snails, a group of viviparid snails (Idiopoma umbilicata, Filopaludina sumatrensis polygramma, Filopaludina sumatrensis speciosa and Filopaludina martensi martensi) exhibited a highly infective prevalence (26.10–82.18%) in comparison to the remaining groups of infected snails (0.48–14.16%). The high diversity and wide dispersion of viviparids in our research areas (five species/subspecies obtained from 49 of all 59 sampling localities) may have contributed to trematode transmission success by increasing the possibility of encounters between the trematode larvae and their potential snail hosts. Species diversity with existence ability in various habitat types of the Asian viviparids based on their phenotypic divergence has been suggested by Hirano et al. (Reference Hirano, Saito, Tsunamoto, Koseki, Prozorova, Do, Matsuoka, Nakai, Suyama and Chiba2019). Nevertheless, insightful information in terms of parasitic preference and host–parasite relationship of the viviparids remains scarce, and restricted our clear conclusion, although the viviparid snails have frequently been mentioned for their infections with trematode metacercariae. Based on previous observations considered in several Asian countries (Thailand, Vietnam, Lao PDR, Cambodia and South Korea), a group of viviparid snails could be addressed as a common intermediate host of the metacercariae, particularly the three following snails: Filopaludina martensi martensi, Filopaludina sumatrensis polygramma and Cipangopaludina spp. (Chai et al., Reference Chai, Sohn, Na and Van De2011; Chantima et al., Reference Chantima, Chai and Wongsawad2013, Reference Chantima, Suk-Ueng and Kampan2018; Sohn et al., Reference Sohn, Chai, Na, Yong, Eom, Park, Min and Rim2013; Sohn and Na, Reference Sohn and Na2017; Phalee et al., Reference Phalee, Phalee and Wongsawad2018; Sohn et al., Reference Sohn, Na, Lee, Eom, Yong, Chai and Min2019; Butboonchoo et al., Reference Butboonchoo, Wongsawad, Wongsawad and Chai2020; Chai et al., Reference Chai, Sohn, Cho, Jung, Chang, Lee, Khieu and Huy2021). Our results also represented Filopaludina martensi martensi and Filopaludina sumatrensis polygramma as a common intermediate host of metacercariae based on their carrying capability for diverse metacercarial groups; six different groups of all seven metacercarial groups investigated were observed to be harboured in these two viviparids individually. On the other hand, the lowest infective prevalence was displayed in a pulmonate snail, Physella acuta; the living behaviour of this snail species could contribute to a low chance of encounter between them and an infective stage of digenean trematodes due to their microhabitat preference of existing by attaching to floating vegetations near the water surface, a phenomenon that was usually observed in our field survey, bringing about the low observed infection rate. Furthermore, the snail Physella acuta has been known as an invasive species, and they are well distributed in various microhabitats in Thailand (Ng et al., Reference Ng, Limpanont, Chusongsang, Chusongsang and Panha2018). Invading properties of this snail species in their non-native region could also be a possible reason to describe their low parasitic infection based on the ‘enemy-release hypothesis’ – great distribution and abundance of invasive species are considered as the results of decreasing regulation by predators, parasites and other natural enemies in their invasive region (Keane and Crawley, Reference Keane and Crawley2002; Torchin et al., Reference Torchin, Lafferty, Dobson, McKenzie and Kuris2003).
Among the five snails with no metacercarial infection that were investigated, two of them, Cyclotropis carinata and Filopaludina martensi cambodiensis, are probably restricted by a lack of their distribution and abundance in the current study areas; of all 59 snail sampling sites, one sampling site with only one individual was observed with an appearance of Cyclotropis carinata, while Filopaludina martensi cambodiensis with four individuals was sampled from three sampling sites. Moreover, the habitat preference of the snail Cyclotropis carinata has been known as the tidal zone of slight brackish water (Brandt, Reference Brandt1974) and, based on our field work, this snail individual was collected from the water body with 0.48 ppt of an average salinity recorded (standard range of brackish water: 0.5–30.0 ppt). Therefore, this habitat type with the relatively brackish condition could be unsuitable as the foraging and habitation areas of some normal animal hosts of the local trematodes, leading to an unsuccessful transmission of the trematode faunas. The remaining three of all five uninfected snails were considered as members of the family Thiaridae, including Melanoides tuberculata, Sermyla riqueti and Thiara scabra with 297, 134 and 154 examined individuals, respectively. According to previous studies, the thiarid snails have usually been reported for the cercarial infections, especially a group of heterophyid cercariae (Dechruksa et al., Reference Dechruksa, Krailas, Ukong, Inkapatanakul and Koonchornboon2007; Pinto and De Melo, Reference Pinto and De Melo2011; Krailas et al., Reference Krailas, Namchote, Koonchornboon, Dechruksa and Boonmekam2014; Chontananarth and Wongsawad, Reference Chontananarth and Wongsawad2017; Veeravechsukij et al., Reference Veeravechsukij, Namchote, Neiber, Glaubrecht and Krailas2018; Apiraksena et al., Reference Apiraksena, Namchote, Komsuwan, Dechraksa, Tharapoom, Veeravechsukij, Glaubrecht and Krailas2020; Wiroonpan et al., Reference Wiroonpan, Chontananarth and Purivirojkul2021); evidence of their metacercarial infections remains scarce, although the relatively low infective prevalence of some trematode metacercariae has been known from two thiarid snails, including Tarebia granifera from our study and Melanoides tuberculata from a study by Bunchom et al. (Reference Bunchom, Pilap, Suksavate, Vaisusuk, Suganuma, Agatsuma, Petney and Saijuntha2020). Thus, it probably suggests the aspect that thiarid faunas are possibly nontarget hosts and unsusceptible to metacercarial infections in comparison to infections by cercariae. Compatibility and susceptibility of thiarid snails and which larval stages of trematodes can be noted are interesting questions and should be investigated in the future for more understanding.
Between two primary groups of the snail hosts, this study discovered that prosobranchs had a higher potential host of metacercariae than pulmonates based on their ability to harbour metacercariae (prosobranchs carrying all seven metacercarial groups currently investigated and pulmonates carrying four of all seven groups). Because they were found solely in prosobranchs, three groups of metacercariae, comprising echinostome D, renicolid and thapriellid, may be distinguished from the others by their snail host group. The different potentiality of prosobranchs and pulmonates to be hosts of metacercariae was quite difficult to interpret based on biological differences (reproductive biology, growth patterns and life span) of the two snails as recommended by Esch and Fernandez (Reference Esch and Fernandez1994) due to scarce information about comparative biology between these two snail groups related to trematode infections. However, microhabitat preference of pulmonates to inhabit near the water's surface level (Cheatum, Reference Cheatum1934) may contribute to a low confronting chance between the snails and cercarial stage of some trematode species residing in deeper water levels (Loy et al., Reference Loy, Motzel and Haas2001; Haas et al., Reference Haas, Beran and Loy2008), probably limiting the ability of some trematodes to parasitize pulmonate snails.
Concerning concurrent infections, a quite low proportion, with 1.82% of all examined freshwater snails, exhibited the individual infection with two or three metacercarial groups at the same time (double or triple infections). Of those infected snails, almost a hundred percent belonged to a group of viviparid snails with four taxa, namely Filopaludina martensi martensi, Filopaludina sumatrensis polygramma, Filopaludina sumatrensis speciosa, and Idiopoma umbilicata. These viviparid snails are well known in many areas of Thailand for their popularity as a human food source and, meanwhile, they can carry the infective stage of various digenean trematodes, including a group of human flukes. Thus, it is fascinating to note the pathogenic severity in humans when they consume uncooked or semi-cooked dishes of the metacercaria-carrying snails (in the case of those mixing metacercariae that can cause disease together within their final hosts). Our finding is consistent with previous studies (Sewell, Reference Sewell1922; Devkota et al., Reference Devkota, Budha and Gupta2011) on the two following points: (1) a low infective proportion observed and (2) the occurrence of concurrent infections that are commonly found in the snails that can serve as the host of various species of digenean trematodes (as mentioned above, our results showed six of all seven metacercarial groups harboured in viviparid snails). However, these two previous studies were based on the investigations of cercarial infections.
Of all seven metacercariae currently observed, only two of them, echinostome A and thapriellid, have usually been reported for their infections in freshwater snail faunas from several areas of Thailand (Anucherngchai and Chontananarth, Reference Anucherngchai and Chontananarth2016; Chantima et al., Reference Chantima, Suk-Ueng and Kampan2018; Phalee et al., Reference Phalee, Phalee and Wongsawad2018; Wiroonpan and Purivirojkul, Reference Wiroonpan and Purivirojkul2018; Chantima and Rika, Reference Chantima and Rika2020), representing that a quite high diversity of metacercariae could be established for our study. The remaining five groups (echinostome B, echinostome C, echinostome D, Opisthorchiata-like and renicolid) still had no reports of their infections in snail hosts and can be addressed as the new records in Thailand; this finding provides an additional range of larval trematode diversity and indicates an important role and involvement of freshwater snails in trematode transmissions in ecosystems. However, these five metacercariae seem to be difficult to discuss with any previous data due to an absence of information when compared with those two metacercariae that are frequently documented. Based on both morphological and molecular assessments, five morphological groups of the metacercariae, excluding echinostome D and thapariellid due to their unsuccessful DNA amplifications, can be molecularly categorized into different taxonomic levels. Sequence data of two metacercariae, echinostome A and echinostome B, displayed a close relationship with a specific trematode species and can be identified into the species level. Meanwhile, sequence data of echinostome C and renicolid metacercariae can be molecularly identified into the genus and family levels, respectively. Unfortunately, sequence data of Opisthorchiata-like metacercaria cannot be stated in the narrowly taxonomic level based on molecular evidence. Regarding the DNA region evaluated, our phylogram represented an appropriate region of the ITS1-5.8S-ITS2 for the phylogenetic investigations among distant species of trematodes, according to a suggestion of Duflot et al. (Reference Duflot, Setbon, Midelet, Brauge and Gay2021), because its topology showed no difference in a grouping of evolutionary relationships when compared to previous research in terms of broader clades. The phylogenetic trend of major clades from our results was also congruent with a study by Olson et al. (Reference Olson, Cribb, Tkach, Bray and Littlewood2003) that used different genes [i.e. complete small subunit ribosomal RNA (ssrDNA) and partial large subunit ribosomal RNA (lsrDNA)] to consider the phylogeny and classification of digenean trematodes. Considering only the region of ITS1-5.8S-ITS2 seems to be inadequate for species discrimination due to its relatively conserved region (Duflot et al., Reference Duflot, Setbon, Midelet, Brauge and Gay2021). For additional study, a mitochondrial gene, cytochrome c oxidase subunit 1 (cox1), should be included to evaluate the species discrimination because of the high mutation rate of its region (Duflot et al., Reference Duflot, Setbon, Midelet, Brauge and Gay2021); moreover, this gene was mentioned as a reliable DNA region for species identification of many species (Dawnay et al., Reference Dawnay, Ogden, McEwing, Carvalho and Thorpe2007). Nevertheless, unavailability of involved DNA sequence data of some current metacercariae on the GenBank database could limit the accessibility of taxonomic identification to a narrow level when using molecular approaches.
With discussion below, each group of the investigated metacercariae is presented with more details of their various biological aspects, such as morphological features, infective situation, snail host range, important roles in ecosystems, medical and livestock impacts, etc.
For metacercariae of echinostome A, their common morphological characters were congruent with the 37-collar-spined Echinostoma spp. (group) (or simply called as ‘revolutum’ group) (Chai et al., Reference Chai, Cho, Chang, Jung and Sohn2020) in several similar features, such as appearances of a bilayered cyst wall, a head collar with 37 collar spines, muscular oral and ventral suckers, and prominent excretory granules inside the excretory system's main collecting tubes. However, morphological characters of this metacercarial group cannot be utilized alone to clearly identify them into a species level, although the number and arrangement of their collar spines (important characteristics for the echinostome trematode identification) were included for consideration. According to our phylogenetic assessments, the phylogram showed a close relationship between our sequence data of this metacercaria and a sequence of Echinostoma mekongi (MT409016.1), with a high posterior probability value and low genetic distance between sequences, indicating that our echinostome A metacercariae were certainly conspecific with Echinostoma mekongi. The adult stage of Echinostoma mekongi was initially detected in riparian people around the Mekong River in Cambodia (Cho et al., Reference Cho, Jung, Chang, Sohn, Sinuon and Chai2020), and then its metacercarial stage was discovered in a viviparid snail, Filopaludina martensi cambodiensis, acquired near the Tonle Sap Lake of Cambodia (Chai et al., Reference Chai, Sohn, Cho, Jung, Chang, Lee, Khieu and Huy2021). The Echinostoma mekongi has never been documented for its distribution in Thailand, hence the current study can be considered a novel record at the metacercarial stage. This echinostomatid species is one of serious parasites for human health as mentioned above that they were originally discovered to infect Cambodian people. Our results represented a high variety of freshwater snails that were infected with the echninostome A (Echinostoma mekongi) metacercariae; 13 of all 19 examined snail species/subspecies harboured this metacercarial group. All thirteen taxa belonged to nine of prosobranchs (Pomacea canaliculata, Bithynia siamensis siamensis, Anentome helena, Sulcospira housei, Tarebia granifera, Filopaludina martensi martensi, Filopaludina sumatrensis polygramma, Filopaludina sumatrensis speciosa and Idiopoma umbilicata) and four of pulmonates (Austropeplea viridis, Radix auricularia, Physella acuta and Gyraulus siamensis); all of them can be considered as additional second intermediate hosts of the Echinostoma mekongi metacercariae. Our investigation did not uncover this metacercaria in the snail Filopaludina martensi cambodiensis, as described by Chai et al. (Reference Chai, Sohn, Cho, Jung, Chang, Lee, Khieu and Huy2021) from Cambodia; this might be owing to the snail's scarce distribution in our research locations (only six individuals were examined throughout the study). Concerning the prevalence and mean intensity of echinostome A metacercariae, the infection in freshwater snails tended to be high in a group of viviparid snails. Nonetheless, understanding about the metacercaria's host preference in viviparid snails remains sparse in Thailand and for all of Southeast Asia. Availability of this metacercaria in the snail faunas may raise awareness concerning the consumption behaviours of humans, to avoid ingesting the snails carrying metacercaria, particularly viviparid snails that commonly harbour this metacercarial group and are popularly consumed by humans.
Regarding metacercariae of echinostome B, their general morphological features were quite similar to the morphology of echinostome A, such as elliptical cyst and prominent excretory granules inside main collecting tubes; moreover, those two groups were usually found to infect the same organ, heart tissue, of snail hosts. However, the echinostome B can be discriminated from echinostome A by the following characteristics: indistinctiveness of collar spines, an oral sucker larger than the ventral sucker, and relatively small cyst. Following our phylogenetic assessments, this metacercarial group can be identified as conspecific with an echinostomatid trematode Euparyphium albuferensis, based on the low genetic divergences and the high posterior probability value between our sequence data (46TLC2 and 12TK2) and a sequence of Euparyphium albuferensis (AJ564384.1). The Euparyphium albuferensis is scarcely mentioned in Thailand and worldwide for its general biology of any developing stages. This species was discovered as a new species from Spain by Esteban et al. (Reference Esteban, Toledo, Sánchez and Muñoz-Antolí1997) that they found in its adult stage in brown rat (Rattus norvegicus) and black rat (Rattus rattus) for natural infection; albino rats, mice and golden hamsters have also been known to be able to serve as final hosts of this trematode species, based on an experimental infection. Esteban et al. (Reference Esteban, Toledo, Sánchez and Muñoz-Antolí1997) also mentioned the metacercarial stage of this trematode species and described the shape and size of cyst (i.e. spherical shaped and size of 132–146 μm for its diameter); our finding of echinostome B morphology was according to the descriptions of this previous study, with the same shape and relatively similar range of the cyst diameter (102–140 μm). Considering the common characters of genus Euparyphium, some morphological features of echinostome B metacercariae were similar to the adult Euparyphium following the descriptions of Kostadinova and Gibson (Reference Kostadinova and Gibson2002) and Jones et al. (Reference Jones, Bray and Gibson2005), such as relatively small collar spines, a quite small ventral sucker, a short prepharynx and esophagus, and an elongated oval pharynx. Members of Euparyphium displayed inconsistence and a varying number of collar spines among different species; 27, 45 and 55 spines were documented (Kostadinova and Gibson, Reference Kostadinova and Gibson2002). An infective situation and a snail intermediate host range of the echinostome B could not be clearly discussed with previous data due to the lack of infective evidence of this metacercarial group.
With respect to echinostome C metacercariae, among all collar-spined metacercariae currently investigated, the metacercariae of echinostome C can be separated from the remaining by the differences of their elliptical cyst shape and their relatively large and elliptical excretory granules. Collar spines of the echinostome C were rather morphologically similar to the echinostome A and echinostome B, but they were quite shorter than the echinostome A and more prominent than the echinostome B. According to the phylogenetic analyses, sequence data of echinostome C exhibited an intimate relationship with a clade of genus Echinostoma, no specific species closely related, with a high support value of posterior probability. Thus, at least a genus level of trematode faunas could be scoped and identified for this metacercarial group. Remarkably, this metacercarial group was frequently observed to infect together with the echinostome cercaria within the same snail individuals. Our previous study (Wiroonpan et al., Reference Wiroonpan, Chontananarth and Purivirojkul2021) considered the species based on genetic evidence of the echinostome cercaria that infected together with this echinostome C metacercaria and revealed that the echinostome cercaria was closely related to an echinostomatid Petasiger exaeretus; meanwhile, our current study suggested that the echinostome C metacercaria belonged to the genus Echinostoma as mentioned above. Thus, this phenomenon of concurrent infections does not appear to be interpreted as the cercarial stage reinfecting the same snail host to develop its metacercarial stage; also, it does not suggest the same trematode species of these cercarial and metacercarial stages infected the same snail at the same time.
In a group of echinostome D metacercariae, the head collar with four large spines clumped at the corners and the elongated oval cyst shape can be considered as distinctively morphological characters of this metacercarial group that differ from the remaining groups of collar-spined metacercariae. Based on our observation, metacercarial specimens of echinostome D always occurred as contained within their redial stage inside the snail host tissue. An occurrence of metacercariae developing within the redial stage was previously denoted. Chérnin (Reference Chérnin1967) revealed that almost half of all echinostome rediae observed in a planorbid snail, Australorbis glabratus, were individually found to contain a few normal metacercariae, and suggested that the cercariae did not require leaving their rediae before their encystment, which is in accordance with a study by Nasir (Reference Nasir1962) as well. Meanwhile, Chérnin (Reference Chérnin1967) found that all metacercaria-contained rediae were dead, and this finding supported that ‘intra-redial encystment seems to particularly occur in echinostome larvae which are unhealthy for various reasons’. Nevertheless, the metacercaria-contained rediae represented in that previous study were observed via the transplanted rediae in a snail Australorbis glabratus, whereas the same observation in our finding was found from naturally infected Filopaludina sumatrensis polygramma snails. The echinostome D metacercariae were one of the undefined taxonomic groups in our study for their actual trematode taxa because of their limitations of the small number of specimens, indistinctively morphological features of an encysted stage, and unsuccessful molecular practices for the phylogenetic assessments and molecular identification.
With regard to Opisthorchiata-like metacercariae, specimens of this metacercarial group were prominent for their morphological features with large-sized cyst, larva loosely contained within the cyst, flexible and transparent cyst, and distinctive excretory bladder. For their infective situations in snail faunas, these metacercariae tended to display high prevalence and high mean intensity of the infection in a group of viviparid snails. It is possible to notice that this metacercarial group preferred to utilize viviparid snails as its second intermediate host; however, more thorough and comprehensive evidence in terms of host–parasite interactions needs to be carried out to prove this view. Opisthorchiata-like metacercariae were successfully accessed and evaluated for their genetic properties, but our results cannot shape their narrow-leveled taxa based on the molecular investigations. Following the phylogram, sequence data of this metacercarial group exhibited a close relationship with a clade of a broad level of actual trematode taxon (i.e. a clade of suborder Opisthorchiata, which consists of the following trematode families: Cryptogonimidae, Heterophyidae and Opisthorchidae). Adult trematodes of these three families have been known to exist in different final hosts and to be different groups of parasitic trematodes. Cryptogonimids are digenean trematodes that parasitize several fishes; heterophyids are well known as an intestinal fluke of birds and mammals; and opisthorchids commonly exist in reptiles, birds and mammals as a liver fluke (Schell, Reference Schell1970; Bray et al., Reference Bray, Gibson and Jones2008). Nevertheless, it would be better to know more about the biological features of any developing stages of the current metacercarial group, especially with an important impact on human public health problems due to a high infection rate observed in the snail faunas that are popular for human consumption.
With respect to renicolids, the metacercariae of this group differ morphologically from the others by their small rod cyst and outer cyst wall provided with thick spacing. In terms of infective areas inside the snail body, they seem to prefer parasitizing the head part of their snail host tissue, while other metacercarial groups were usually found to parasitize the internal organs or soft mantle tissue of their snail body. It is interesting to question the potential second intermediate hosts of renicolid metacercariae because they were found to be harboured only by a group of viviparid snails, based on our observation; additional considerations in cases of specificity and relationship between the metacercariae and their snail hosts could possibly provide further comprehensive evidence and more understanding about the fundamental biology of this metacercarial trematode. According to our phylogenetic investigations, at least a family level of digenean trematodes (i.e. family Renicolidae) can be categorized for the current renicolid sequence data. Adult trematodes of the family Renicolidae are commonly known as a group of parasitic faunas in kidneys and ureters of aquatic birds (Schell, Reference Schell1970; Bray et al., Reference Bray, Gibson and Jones2008), and their distribution and infective data, as well as other biological features, have scarcely been explored in Thailand.
Concerning the group of thapariellid metacercariae, the metacercarial stage of this group does not encyst and can be observed by the naked eye due to being quite large in comparison to the remaining groups of our finding. Descriptive morphological features in terms of shape, position, and arrangement of organs were not different between our specimens and the specimens previously documented from several areas of Thailand (Anucherngchai and Chontananarth, Reference Anucherngchai and Chontananarth2016; Chantima et al., Reference Chantima, Suk-Ueng and Kampan2018; Wiroonpan and Purivirojkul, Reference Wiroonpan and Purivirojkul2018, Reference Wiroonpan and Purivirojkul2021; Chantima and Rika, Reference Chantima and Rika2020), especially the descriptions in a study of first record in Thailand (Phalee et al., Reference Phalee, Phalee and Wongsawad2018). Comparing the measured characteristics of our specimens to the first record study's specimens, the sizes of the body and oral sucker between these two studies were quite similar in range. Pharynx size of our specimens was somewhat larger than that found in Phalee et al. (Reference Phalee, Phalee and Wongsawad2018). In contrast, the ventral sucker, testes, and ovary of our specimens were quite smaller than the specimens of Phalee et al. (Reference Phalee, Phalee and Wongsawad2018). These small variations between the two investigations might, however, be due to a staining procedure during specimen processing. The thapariellid metacercariae can be identified to a species level based on their morphological traits, and they belong to the trematode species Thapariella anastomusa, which is an intestinal fluke that in its adult stage regularly parasitizes aquatic birds (Schell, Reference Schell1970; Phalee et al., Reference Phalee, Phalee and Wongsawad2018). Although thapariellid metacercariae seem to be widespread in Thailand, their negative effects on human health and livestock farming have never been established. Prevalence and mean intensity of thapariellid metacercarial infection appear to differ among species/subspecies of their snail hosts in comparison to previous studies. Anucherngchai and Chontananarth (Reference Anucherngchai and Chontananarth2016) found that the snail Filopaludina sumatrensis polygramma had the greatest prevalence and mean intensity, whereas Phalee et al. (Reference Phalee, Phalee and Wongsawad2018) found the same characteristics in snail Filopaludina martensi martensi. Chantima et al. (Reference Chantima, Suk-Ueng and Kampan2018) and Chantima and Rika (Reference Chantima and Rika2020) showed the highest prevalence of the infection in snail Filopaludina doliaris, while the highest mean intensity was observed in different snails of Filopaludina doliaris (Chantima et al., Reference Chantima, Suk-Ueng and Kampan2018) and Filopaludina martensi martensi (Chantima and Rika, Reference Chantima and Rika2020). According to those prior studies and ours, the two viviparid snails (Filopaludina martensi martensi and Filopaludina sumatrensis polygramma) might be common snail intermediate hosts of the Thapariella anastomusa metacercarial stage. Interestingly, a viviparid snail, Idiopoma umbilicata, was shown to be an intermediate host of Thapariella anastomusa metacercariae for the first time in Thailand, revealing the wide range of snail hosts of this trematode species. More information regarding the molecular evidence of this metacercarial group could not be offered here, because we were unable to completely assess their genetic materials during the process of molecular investigations.
In conclusion, our investigations denoted a variety both of trematode metacercariae and their snail intermediate hosts in Bangkok, Thailand. The diversity of the trematode larvae, metacercariae, in our study can be considered to be high when compared to previous research in Thailand, and five out of all seven of these metacercariae have been revealed as new records in Thailand, increasing basic information of larval trematode diversity in freshwater snails and revealing ability and an important role of snail faunas in trematode transmission processes. Nonetheless, the taxonomic status of some metacercarial groups, such as Opisthorchiata-like and renicolids, needs to be further clarified in the future to determine their actual species and obtain better knowledge of their biology. Growing these metacercariae in their definitive hosts would be another option for obtaining important adult characteristics, particularly reproductive organ features, which are a key feature for determining the actual species of many digenean trematodes. DNA sequence comparisons between metacercariae and the experimented adults could also be included. Interestingly, a species of human intestinal fluke, Echinostoma mekongi, has been mentioned for the distribution of its infective stage (echinostome A metacercariae) in our study areas, providing additional basic information for epidemiological surveillance of this medically important fluke in Thailand. Moreover, for a better understanding of trematode transmissions among the animal hosts in various habitats, both physical and biological factors, such as habitat types, water-quality parameters, animal host composition, disturbance from human activities, etc., should be further provided to evaluate the factors that would relate and contribute to parasitic infections in ecosystems.
Acknowledgements
We appreciate our lab colleagues and friends for their help in field studies. We also thank reviewers for their valuable recommendations in this study.
Financial support
This work was supported by the Office of the Ministry of Higher Education, Science, Research and Innovation; and the Thailand Science Research and Innovation through the Kasetsart University Reinventing University Program 2021; the Center of Excellence on Biodiversity (BDC), Office of Higher Education Commission (BDC-PG2-161004); the Science Achievement Scholarship of Thailand (SAST); and the Department of Zoology, Faculty of Science, Kasetsart University.
Conflict of interest
The authors declare there are no conflicts of interest.
Ethical standards
This work was authorized by the ethics committee of Kasetsart University (approval no. ACKU61-SCI-033) to rear the snail specimens and explore their metacercarial infections.