Introduction
Seeds do not usually germinate during development on the parent plant, but undergo a process of maturation, generally including desiccation, before being shed (Bewley and Black, Reference Bewley and Black1994). In contrast, embryos excised from developing seeds of many species can germinate if nutrition is provided. However, germination of an excised embryo will not occur before the embryo reaches a certain stage of development. Arabidopsis embryos at early developmental stages, such as the heart and torpedo stage, do not germinate when they are excised from seeds and incubated in nutritionally enriched media (Raz et al., Reference Raz, Bergervoet and Koornneef2001). It is possible to induce vivipary by growing Arabidopsis siliques containing heart or torpedo embryos in the presence of supplements. In this case, immature embryos are also unable to germinate before reaching morphological maturity (Raghavan, Reference Raghavan2002). Likewise, the radicle does not emerge from the rudimentary embryo in a mature imbibed carrot seed until the embryo reaches approximately two-thirds of the length of the seed. Carrot embryos excised from imbibed seeds before they reach this size also do not exhibit radicle elongation even in the presence of nutrition (Homrichhausen et al., Reference Homrichhausen, Hewitt and Nonogaki2003). These findings indicate that a certain level of morphological maturity during the normal seed developmental programme, which is probably accompanied by physiological or cell maturation, is required to reach germination potential or the ability of the radicle to elongate.
It has been suggested that embryo body organization is of great importance for post-embryonic development (Jürgens, Reference Jürgens2001), yet little is known about what level of embryo organization is essential for germination potential. We have found an Arabidopsis embryogenesis mutant that produces shrunken seeds containing small, arrested embryos with partial desiccation tolerance. The arrested embryos excised from developing seeds were capable of elongating roots in nutritionally enriched media. The occurrence of germination potential in this mutant and wild-type embryos during normal seed developmental programme is characterized in this paper, providing insights into developmental programmes involved in determining root identity and germination potential in developing seeds.
Materials and methods
Plant materials
The seeds of Arabidopsis thaliana wild-type Columbia (Col-0) and knockout plants were obtained from the Arabidopsis Biological Resource Center, The Ohio State University, USA. Seeds were sown in soil in small pots (2.5 × 2.5 cm) and germinated at 22°C after 3 d of cold stratification at 4°C. Seedlings were grown in an incubator (22°C) under short-day conditions (8 h light/16 h dark periods), transferred to the greenhouse (22°C) with long-day conditions (16 h light/8 h dark periods) to induce flowering, and grown until seed harvest. Siliques were harvested and dried at 25°C in an incubator for 7 d. Seeds were extracted from dry siliques and stored in plastic tubes at 4°C until use.
Screening
For screening germination mutants, ten plants were grown for each knockout line. Seeds (200–300) from each knockout plant were plated on filter paper (No. 2, Whatman Inc., Clifton, New Jersey, USA) moistened with 4 ml water, given a 3 d cold stratification at 4°C and examined for germination. Multiple seed batches (15) were plated in a large plastic Petri dish (12.5 cm diameter) without replication for the preliminary screening.
Embryo excision and incubation
Dry mature seeds were imbibed for 2–3 d, and embryos were excised using fine forceps and a surgical blade under a dissection microscope. Embryos from developing wild-type and mutant seeds were excised as described above and incubated in Murashige–Skoog (MS) agar medium (Murashige and Skoog, Reference Murashige and Skoog1962) with or without 1% (w/v) sucrose.
Scanning electron microscopy
Excised embryos were fixed overnight in 10 mM potassium phosphate buffer, pH 7.0, containing 4% (w/v) paraformaldehyde, and then dehydrated in an increasing ethanol series [30–100% (v/v)]. Specimens in 100% ethanol were critical point dried with carbon dioxide in a Balzer CPD-020 dryer (Balzers Union, Ltd, Balzers, Liechtenstein) according to Anderson (Reference Anderson1951). The dried specimens were mounted on an aluminium planchette and coated with c. 10 nm of 60/40% Au/Pd using an EDWARDS S150B sputter coater (Edwards High Vacuum, Ltd, West Sussex, England) operating at 1 × 10− 2 torr, 5 mbar argon pressure and 1.5 kV, 20 mA plasma current, for 60 s. Examination was performed using an AmRAY 3300FE scanning electron microscope (AmRay, Bedford, Massachusetts, USA) at the Electron Microscope Facility, Department of Botany and Plant Pathology, Oregon State University.
Tetrazolium staining
Siliques containing wild-type and embryo ball (eb) mutant seeds at different stages of development were desiccated at 25°C in an incubator for 2 weeks and rehydrated. Embryos were excised and subjected to staining with 0.5% (w/v) 2,3,5-triphenyl tetrazolium chloride solution.
Differential interference contrast (DIC) microscopy
Seeds were excised from the wild-type and mutant siliques at different developmental stages and cleared with chloral hydrate solution [3.5 ml water, 0.5 g glycerol, 10 g chloral hydrate (#C-8383, Sigma, St. Louis, Missouri, USA)] overnight, according to the methods described at the SeedGenes Project website (http://www.seedgenes.org:8081/Tutorial.html#Nomarski%20Optics). Samples were mounted between a microscope slide and a cover slip with Hoyer's solution (7.5 ml water, 1.3 g glycerol, 1.9 g gum arabic and 25 g chloral hydrate) and observed with an Axioskop 2 Plus microscope (Zeiss, Jena, Germany). The DIC optics, coupled to a Pixera camera (Model #PVC 100C, Pixera Corporation, Los Gatos, California, USA), were used to capture images, which were then processed with Pixera Visual Communication Suite software.
Results
Isolation of the embryo ball (eb) mutant
To obtain insight into transcriptional regulation during seed germination, Arabidopsis Biological Resource Center (ABRC) SALK T-DNA knockout mutants for ten gibberellin (GA)-inducible transcription factors (Ogawa et al., Reference Ogawa, Hanada, Yamauchi, Kuwahara, Kamiya and Yamaguchi2003) were examined in our laboratory. While most lines germinated normally without any apparent defects, one line [SALK_045674 (At2g41940, ZFP8, C2H2 zinc finger protein)] showed partially reduced seed germination percentages in four individual seed lots (69%, 70%, 75% and 78%). In a fraction of each seed lot, about one-fourth (24%) of the seeds were shrunken (Fig. 1A) and exhibited delayed imbibition compared with wild-type seeds (Fig. 1B, C and D). An embryo-like structure was seen inside the fully imbibed knockout seeds (Fig. 1E). Excised mutant embryos were small, irregular in shape and lacked an apparent radicle and cotyledons compared to wild-type (Fig. 1F and G). Eighty-eight percent (87/99) of arrested embryos exhibited a small ball-like structure (Fig. 2A, B and C), and therefore, the mutant was named as embryo ball (eb). The remaining mutant embryos exhibited symmetrical or asymmetrical cotyledon-like structures (Fig. 2D, E, F and G).
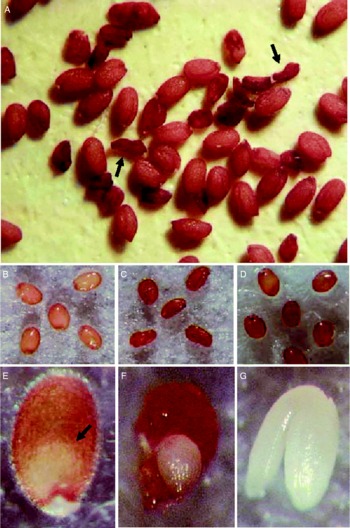
Figure 1 Characteristics of embryo ball (eb) mutant seeds found during screening of germination-associated gene knockouts of Arabidopsis thaliana, Columbia (Col-0). (A) Dry seeds of wild type (filled with full embryos) and the eb mutant (shrunken, arrows). (B) Wild-type seeds imbibed for 2 d; (C) and (D) mutant seeds imbibed for 2 and 3 d respectively. (E) Mutant seed containing a small embryo-like structure inside; (F) embryo-like structure excised from the mutant seed; (G) mature embryo excised from wild-type seed.

Figure 2 Scanning electron microscopy of eb mutant embryos excised from imbibed shrunken seeds of Arabidopsis thaliana. (A), (B) and (C) Small ball-like structures that were the majority (88%) of the mutant embryos. (D), (E), (F) and (G) Relatively larger, irregular-shape mutant embryos with symmetrical or asymmetrical cotyledon-like structures. Bar, 100 μm.
While the eb embryos had apparent defects in terms of morphology and the intact eb seeds did not geminate following full imbibition, the tissues in excised embryos showed no sign of decay, as is often seen in non-viable embryos. When eb embryos were excised from rehydrated seeds and stained with tetrazolium (TZ) to test for viability, the relatively smaller eb embryos were not stained while the larger eb embryos exhibited partial (10% in the population) or full staining (5%) by TZ (Fig. 3A). To determine when desiccation tolerance occurred in developing wild-type Arabidopsis seeds, siliques at different stages of development were harvested, desiccated for 2 weeks and rehydrated. Embryos were excised from the seeds and stained for viability. Tetrazolium staining of excised embryos at different developmental stages clearly indicated that Arabidopsis embryos at walking stick, torpedo or earlier stages were not desiccation tolerant (Fig. 3B). Although localized TZ staining was observed in walking-stick stage embryos, only morphologically mature embryos exhibited full staining by TZ (Fig. 3C).

Figure 3 Desiccation tolerance of wild-type and embryo ball (eb) mutant embryos of Arabidopsis thaliana. Tetrazolium (TZ) staining of eb mutant (A) and wild-type (B and C) embryos. Developing siliques containing different stages of wild-type and mutant embryos were dried for 2 weeks, and seeds were extracted and imbibed for 1 d at 22°C. Embryos were excised and stained by 0.5% (w/v) 2,3,5-triphenyl tetrazolium chloride solution. Note that a mutant embryo with severe morphological defects exhibited strong staining by TZ, while only mature embryos were fully stained by TZ in the wild type.
Developmental defects in the embryo ball (eb) mutant
In the eb lines, 24% of mature seeds were shrunken. The remaining viable seeds produced wild-type plants (35%) and plants carrying mutant seeds (65%). The eb siliques contained both wild-type seeds with normal embryos and colourless mutant embryos (Fig. 4A, B and D). The mutant seeds became shrunken at maturity (Fig. 4C).
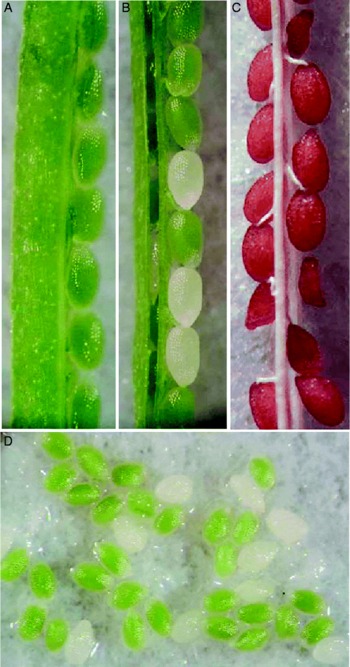
Figure 4 Colourless seed and shrunken testa phenotypes of the embryo ball (eb) mutant of Arabidopsis thaliana. (A) Developing seeds in a wild-type silique; (B) and (C) Developing and mature seeds in heterozygous eb mutant siliques. (D) Green wild-type and colourless mutant seeds excised from a heterozygous eb mutant silique. Note that about one-fourth of the seeds exhibit the mutant colourless phenotype.
Since all seeds are colourless at the early stages of embryogenesis, it was hard to distinguish mutant from wild-type seeds. Later, when wild-type embryos reached the heart/torpedo stages and became pale green, mutant seeds lacked colour and could be distinguished from wild-type seeds. In Fig. 5A and B, a torpedo embryo from a wild-type pale green seed is compared to an arrested embryo found in the colourless seed, both obtained from the same silique. The mutant embryo phenotype was not observed during early globular stages (Fig. 5C and D). However, at later stages, irregularly shaped globular embryos exhibiting slight surface protuberance were observed (Fig. 5F), as well as globular embryos with normal appearance (Fig. 5E). In siliques containing normal heart-stage embryos (Fig. 5G), small arrested globular embryos were also found (Fig. 5G, inset). The delayed globular embryos did not show apparent defects at this stage and contained normal suspensor cells and hypophysis-derivative cells, including a lens-shaped cell (Fig. 5J and K). Although mutant embryos continue to grow larger than normal globular embryos, they did not differentiate into regular heart or torpedo embryos. The cell organization in transition/heart-like mutant embryos was aberrant (Fig. 5H and I).

Figure 5 Embryo arrest in embryo ball (eb) seeds of Arabidopsis thaliana. (A) and (B) Sections of pale green wild-type seed and colourless eb seed respectively, excised from a single silique. Seeds were sectioned, stained with toluidine blue and observed with a light microscope. (C), (D) and (E) Different stages of globular embryos with normal morphology found in the early stages of eb siliques. (F) Irregularly shaped globular eb embryo with slight protuberance on the surface. (G) Normal heart-stage embryo found in a pale green wild-type seed. Inset, mutant embryo arrested at the globular stage found in the same silique. (H) and (I) Close-up views of wild-type and eb transition/heart embryos respectively. Note the aberrant cell organization in the mutant embryo. (J) and (K) Close-up view and schematic representation of the delayed globular embryo [G] shown in panel (G), inset. Note that normal suspensor cells [S] and hypophysis derivative cells (highlighted in grey), including a lens-shaped cell [LSC] are observed. (C) to (I), differential interference contrast (DIC) images.
Germination potential and loss of cotyledon identity in the embryo ball (eb) mutant
Although eb embryos were larger (c. 100–250 μm) than normal globular/transition embryos ( < 80 μm), they were far smaller than mature wild-type embryos (c. 400–450 μm) and lacked normal embryonic organs (Fig. 1F and G). However, TZ staining showed that some eb embryos developed desiccation tolerance, which is typically associated with the late maturation stage, despite their impaired morphology (Fig. 3). To test whether eb embryos in developing seeds were capable of growing, they were isolated and incubated in MS media containing 1% (w/v) sucrose (Fig. 6A). While 23% of mutant embryos failed to grow, the remaining embryos exhibited apparent growth in both apical and basal regions without exogenous hormones. Surprisingly, the apical part of eb embryos initiated root-hair-like structures after 2–5 d of incubation (Fig. 6B and C). The basal part elongated to a long primary root with many root hairs, which was visually indistinguishable from normal roots observed in germinated seeds (Fig. 6D). Even though the hypocotyl and cotyledons were missing in eb embryos, leaf-like structures emerged from the apical portion of the embryo following root elongation (Fig. 6E). The leaf-like structures exhibited trichomes, indicating adult or vegetative leaf identity (Fig. 6F). However, none of eb embryos grew into adult plants. Tissue sectioning of eb embryos excised from imbibed mature seeds showed that tissue organization was impaired in the mutant embryos, although differentiation of elongated, vascular-like cells, which are normally observed in the procambium of wild-type embryos, was observed at the basal part of eb embryo (Fig. 6G and H).

Figure 6 Germination potential in the arrested embryo ball (eb) embryos of Arabidopsis thaliana. (A) Isolated eb embryo. (B) and (C) eb embryos with root-hair-like structures. (D) Radicle elongation from eb embryos. (E) Leaf-like structures emerged from the apical part of an eb embryo. (F) Trichome on the surface of a leaf-like structure. Bars, 250 μm. (G) and (H) Tissue sections of eb and wild-type embryos respectively, dissected from mature imbibed seeds.
Radicle emergence from intact eb seeds was also examined prior to drying. Seventy-seven percent (24/31) of intact mutant seeds germinated when excised from siliques prior to desiccation. In contrast, none of the wild-type embryos examined at heart (15), late heart (15), torpedo (32) and walking stick (28) stages germinated (Fig. 7A, stages A1–A4). Only partial germination potential was observed in relatively young, morphologically mature embryos (Fig. 7A, stage A5). A more detailed analysis of the induction of germination potential was performed using the green, morphologically mature wild-type embryos in successively developing siliques (Fig. 7B, B1–B7). While embryos from these siliques were morphologically mature and indistinguishable from each other (Fig. 7B, top panels), they exhibited different responses when they were tested for germination potential in media (without desiccation) (Fig. 7B). The relatively younger embryos (B1–B5) did not exhibit full germination potential, while the later-stage embryos (B6 and B7) germinated perfectly (Fig. 7B, germination data). The timing of morphological maturation did not match the timing of the induction of germination potential. During germination tests, the B1- to B5-stage embryos lost green colour in the axis, cotyledons or whole embryos, while B6 and B7 germinable embryos retained their green colour during this period (Fig. 7B, bottom panels) and produced seedlings (data not shown).

Figure 7 Germination potential in wild-type embryos of Arabidopsis thaliana, excised from seeds at different developmental stages. (A) Examination of broad embryogenesis stages. Siliques at the A1–A7 positions in the schematic representation (left) were harvested from a fully developed inflorescence exhibiting the oldest yellowish silique at its base. Seed (top row photos) and embryo (bottom row photos) morphology was examined by dissection and differential interference contrast (DIC) microscopy respectively. Excised embryos (middle row photos) were incubated in Murashige–Skoog medium containing 1% (w/v) sucrose for 4 d at 22°C. The numbers of embryos germinated/tested are shown below the panels. Note that germination potential of embryos does not occur before morphological maturation. (B) Detailed analysis of the timing of the induction of germination potential in morphologically mature embryos. Successive siliques containing mature embryos (positions B1–B7 in the schematic representation) were harvested, embryos were excised from seeds (top row photos) and incubated as described above (bottom row photos), and germination (seedling development) was recorded. Similar experiments were repeated four times. Representative germination data are shown (middle). Colour depletion in the axis and cotyledons (cot) are indicated by arrows in the bottom row panels. Embryos before starting extensive growth are shown. T, torpedo; W, walking-stick.
Discussion
The eb mutant produced colourless seeds, which have also been observed in other embryogenesis mutants (Li and Thomas, Reference Li and Thomas1998; Chen et al., Reference Chen, Ullah, Young, Sussman and Jones2001; Sparkes et al., Reference Sparkes, Brandizzi, Slocombe, El-Shami, Hawes and Baker2003). The rate of embryo lethality (24%) and the segregation between the mutant (65%) and wild-type (35%) plants generated from the viable seeds suggest that eb is a recessive mutation and that homozygous eb mutants are arrested. The eb mutant was originally isolated from SALK_045674, a T-DNA insertion line for At2g41940, a C2H2 zinc finger gene. Our analyses indicated that the mutant phenotypes did not segregate with the T-DNA insertion flanking this gene (data not shown). Therefore, the molecular mechanisms responsible for this mutation are still unknown. However, the mutant phenotypes provided multiple implications important for seed biology. First of all, it is surprising that radicle emergence can be observed from such a small ball-like structure as eb embryo in the absence of plant hormones. The induction of vigorous root growth from the arrested embryos suggests that the basal part of the eb embryo is functional at least to some extent, and that severe defects in the cotyledons and hypocotyl do not affect germination potential in the root. Loss of cotyledon and hypocotyl identity also did not stop the activity at the apical region of the embryo. Embryos excised from developing eb seeds and eb seeds with a testa were able to germinate in media, while germination potential was not observed in wild-type heart, torpedo and walking-stick embryos. There are two possibilities to explain this difference. One possible explanation is that germination potential already occurs in wild-type heart, torpedo and walking-stick embryos, but it is suppressed by some unknown mechanism that is absent in the eb embryo due to the lack of cotyledons and hypocotyl. Another possibility is that occurrence of germination potential in the Arabidopsis embryo absolutely requires physiological maturation that occurs only in the morphologically mature embryo during normal development. In this case, eb embryos must have been through the physiological maturity, at least in part of the defective embryo. The biochemical analysis using TZ supports the latter possibility. The cells in some eb embryos extracted from imbibed seeds were positively stained by TZ, indicating that they were viable after desiccation. These results suggest that, while morphological differentiation is abnormal in the eb embryos after the globular/transition stages, further maturation of the mutant embryos may continue to a certain extent. Germination potential in the mature embryo probably occurs before desiccation tolerance induction, since wild-type, morphologically mature green embryos that exhibited germination potential did not survive desiccation (data not shown).
In addition to the importance of eb mutant phenotypes in seed germination biology, this mutant provides another potentially useful tool for developmental biology. The eb phenotypes are similar to those of the gurke (Torres-Ruiz et al., Reference Torres-Ruiz, Lohner and Jürgens1996; Baud et al., Reference Baud, Bellec, Miquel, Bellini, Caboche, Lepiniec, Faure and Rochat2004; Kajiwara et al., Reference Kajiwara, Furutani, Hibara and Tasaka2004) and laterne (Treml et al., Reference Treml, Winderl, Radykewicz, Herz, Schweizer, Hutzler, Glawischnig and Ruiz2005) mutants that are used in developmental biology research. The gurke embryos also lack cotyledons and hypocotyls and develop root-hair-like structures and vegetative leaves at the apical region when germinated in media (Torres-Ruiz et al., Reference Torres-Ruiz, Lohner and Jürgens1996; Baud et al., Reference Baud, Bellec, Miquel, Bellini, Caboche, Lepiniec, Faure and Rochat2004; Kajiwara et al., Reference Kajiwara, Furutani, Hibara and Tasaka2004). However, cell organization in the basal part of the gurke embryo is very similar to that of normal embryos, including the radial cell distribution patterns and the appearance of procambium (Torres-Ruiz et al., Reference Torres-Ruiz, Lohner and Jürgens1996). Furthermore, gurke seeds are filled with a larger cylindrical embryo and therefore do not exhibit a shrunken testa. In the laterne mutants, which exhibit phenotypes similar to those observed in eb, cell organization in the basal region of the embryo is normal. In laterne, a normal hypocotyl is present; only cotyledons are deleted. Although laterne seeds are thinner than wild-type and gurke seeds, they are both filled with the substantial volume of the embryos (Treml et al., Reference Treml, Winderl, Radykewicz, Herz, Schweizer, Hutzler, Glawischnig and Ruiz2005), which is quite different from eb seeds. Thus, eb embryos exhibit a more severe phenotype than those found in gurke and laterne. While it is possible that eb is a different allele of a known mutation, the most intriguing feature of the eb embryo is its germination potential during seed development. This mutant may provide an excellent tool for developmental biology and seed germination research.
Acknowledgements
We are grateful to the Arabidopsis Biological Resource Center, The Ohio State University, USA for providing the knockout lines for our research. We also thank Sabry Elias, Seed Laboratory, Oregon State University, USA for providing the tetrazolium solution. This work was supported by the National Science Foundation grant IBN-0237562 to H.N. and American Seed Research Foundation grant to H.N. and R.C.M.