Introduction
Zinc, along with copper, is one of the predominant metals in arc volcanic emissions (Edmonds et al., Reference Edmonds, Mather and Liu2018). Fumarolic mineral assemblages enriched in zinc have been described from many localities in the world, as in Vulcano (Bernauer, Reference Bernauer1936) and Santiaguito (Stoiber and Rose, Reference Stoiber and Rose1974). Additionally, zinc oxide and zinc silicate condensate particles were detected in considerable amounts in the ashes of Mt St. Helens (Thomas et al., Reference Thomas, Varekamp and Buseck1982).
Anhydrous sulfate minerals with transition metal cations are dominant in the fumaroles of the Great Tolbachik Fissure eruption, occurring in 1975–1976 and the Tolbachik Fissure eruption of 2012–2013 (Vergasova and Filatov, Reference Vergasova and Filatov2012; Siidra et al., Reference Siidra, Nazarchuk, Zaitsev, Lukina, Avdontseva, Vergasova, Vlasenko, Filatov, Turner and Karpov2017; Pekov et al., Reference Pekov, Koshlyakova, Zubkova, Lykova, Britvin, Yapaskurt, Agakhanov, Shchipalkina, Turchkova and Sidorov2018a; Siidra et al., Reference Siidra, Borisov, Lukina, Depmeier, Platonova, Colmont and Nekrasova2019). A zinc admixture is very common for many exhalative Cu sulfate minerals (Pekov et al., Reference Pekov, Zubkova and Pushcharovsky2018b). Surprisingly, until recently (Siidra et al., Reference Siidra, Nazarchuk, Agakhanov, Lukina, Zaitsev, Turner, Filatov, Pekov, Karpov and Yapaskurt2018a,Reference Siidra, Nazarchuk, Lukina, Zaitsev and Shilovskikhb; Siidra et al., Reference Siidra, Nazarchuk, Zaitsev and Shilovskikh2020), no anhydrous sulfate minerals with Zn-dominated sites in the structure were known and the occurrence of zinkosite, ZnSO4, is doubtful to date (Wildner and Giester, Reference Wildner and Giester1988).
Herein we report on the chemical composition, structure and properties of glikinite (Cyrillic: гликинит), Zn3O(SO4)2. Glikinite is named in honour of Prof Arkady Glikin (Аркадий Эдуардович Гликин) (1943–2012). Arkady Glikin was professor at the Department of Crystallography, Saint-Petersburg State University. The scientific interests of Arkady Glikin were devoted to crystal growth and mineral forming processes. A.E. Glikin was an author of numerous publications in scientific journals, and in Reference Glikin2009 he published a book Polymineral-Metasomatic Crystallogenesis (Glikin, Reference Glikin2009). Arkady Glikin was one of the co-authors of the development of the method for obtaining the first synthetic analogue of malachite with jewellery properties. Both the mineral and the mineral name were approved by the Commission on New Minerals, Nomenclature and Classification of the International Mineralogical Association (IMA2018-119, Nazarchuk et al., Reference Nazarchuk, Siidra, Nekrasova, Borisov and Shilovskikh2019). Type material is deposited at the Mineralogical Museum, St. Petersburg State University, St. Petersburg, Russia (catalogue no. 1/19693).
Occurrence and association
Glikinite is a fumarolic mineral that is deposited directly from volcanic gas emissions as a sublimate. It was found in September, 2017 in the Arsenatnaya fumarole, Second scoria cone, Northern Breakthrough (North Breach), Great Fissure eruption, Tolbachik volcano, Kamchatka, Russia. The Second Scoria Cone is located ~18 km SSW of the active shield volcano Ploskiy Tolbachik (Fedotov and Markhinin, Reference Fedotov and Markhinin1983). Associated minerals are langbeinite, lammerite-β, bradaczekite, euchlorine, anhydrite, chalcocyanite and tenorite (Fig. 1). The temperature of gases at the sampling location was ~250°C. All the recovered samples were packed and isolated when collected to avoid any contact with the external atmosphere.

Fig. 1. Glikinite (white with bluish tint prismatic crystals in the centre) associating with lammerite-β (sky blue), langbeinite (white matrix), euchlorine (grass green) and tenorite (black). Specimen destroyed during analysis, see text.
Physical properties
Glikinite crystals are colourless and transparent. The streak is white. The lustre is vitreous. The mineral is non-fluorescent under UV light or an electron beam. Glikinite is brittle. No cleavage and parting were observed, the fracture is uneven. Hardness corresponds to 2–3 on the Mohs’ scale. The density could not be measured due to the small sample size. The density calculated from the empirical formula is 3.98 g cm-3.
Glikinite is optically biaxial (+), α = 1.737(2), β = 1.686(2), γ (calc.) = 1.671(2) (589 nm) and 2Vcalc = 54°. Dispersion of optical axes is distinct, r > v. In plane-polarised light, the mineral is non-pleochroic, colourless or with a slight bluish hue.
Chemical composition
One crystal (290 μm × 100 μm in size) was mounted in epoxy resin and polished with successively decreasing oil suspensions of diamond powders with the finishing size of 0.25 μm. The mineral was then analysed by energy-dispersive (EDS) and wavelength-dispersive (WDS) spectrometry. The analyses were obtained using a Hitachi S-3400N scanning electron microscope equipped with an Oxford Instruments X-Max 20 Energy Dispersive Spectrometer (ED, n = 8), and Oxford Instruments Inca Wave 500 Wavelength Dispersive Spectrometer (WD, n = 4). The ED spectra were obtained under following conditions: 20 kV accelerating voltage, 1.8 nA beam current; defocused beam (10 μm spot size); and acquisition time 30 seconds per spectrum. The spectra were processed automatically using the AzTecEnergy software package using the TrueQ technique. The WD spectra were obtained under following conditions: 20 kV accelerating voltage; 4 nA beam current; 60 seconds per element acquisition time; and matrix correction using the XPP method. Synthetic CaSO4 (SKα), Cu metal (CuKα), Zn metal (ZnKα) and Mo metal (MoLα) were used as standards for both EDS and WDS analyses. The mineral is stable under the electron beam; no surface damage was observed after analysis.
The empirical formula calculated on the basis of O = 9 apfu is Zn2.07Cu0.97S1.98O9 (Table 1). The simplified formula is (Zn,Cu)3O(SO4)2 or Zn3O(SO4)2, which requires ZnO = 60.40 and SO3 = 39.60, total 100.00 wt.%.
Table 1. Analytical data (wt.%) (n = 12) for glikinite.

X-ray crystallography
Powder X-ray studies were carried-out using a Rigaku R-Axis Rapid II diffractometer with a cylindrical image plate detector, using CoKα radiation. For the powder-diffraction study, a Gandolfi-like motion on the φ and ω axes was used to randomise the sample and observed d values and intensities were derived using osc2xrd software (Britvin et al., Reference Britvin, Dolivo-Dobrovolsky and Krzhizhanovskaya2017). The powder data are presented in Table 2. Unit-cell parameters refined from the powder data are: a = 7.287(7) Å, b = 6.595(8) Å, c = 7.841(7) Å, β = 117.24(9)°, V = 335.0(5) Å3 and Z = 2.
Table 2. Powder X-ray diffraction data for glikinite.
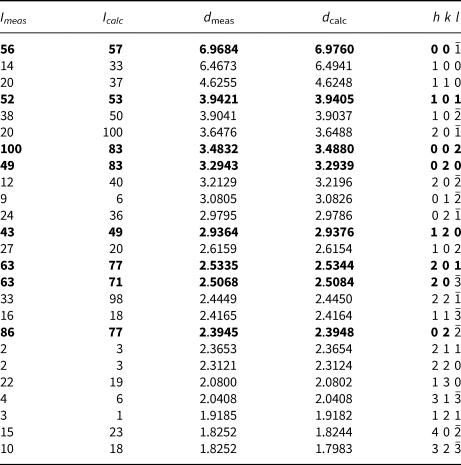
The strongest lines are given in bold.
The specimen shown in Fig. 1 was destroyed for single-crystal XRD analysis. A prismatic crystal of glikinite was crushed under the microscope into separate thin single-crystal fragments. A thin needle (0.03 × 0.03 × 0.15 mm) was used for diffraction measurements performed on a Bruker Smart Apex II DUO diffractometer with a micro-focus X-ray tube equipped with a CCD detector (MoKα radiation). The data were integrated and corrected for absorption using a multi-scan type model implemented in the Bruker programs APEX and SADABS (Bruker-AXS, 2014). More than a hemisphere of X-ray diffraction data was collected. The structure was solved by direct methods and was refined using SHELXL software (Sheldrick, Reference Sheldrick2015). All of the atoms were refined anisotropically. The crystal studied was twinned, and the twinning matrix [1 0 0, 0 $\bar{1}$ 0,
$\bar{1}$ 0
$\bar{1}$] was employed during the crystal structure refinement. The structure of glikinite was successfully refined using the SHELX software package on the basis of F 2 for 518 unique observed reflections with F 2 ≥ 2σ(F 2) to R 1 = 0.046 (Table 3). The unit cell of glikinite is similar to that reported for the (apparently isomorphous) synthetic phase Zn3O(SO4)2 by Bald and Grühn (Reference Bald and Grühn1981) (P21/m, a = 7.937(2) Å, b = 6.690(2) Å, c = 7.851(2) Å, β = 124.39(1)°, V = 344.01 Å3 and R 1 = 0.085). There is a significant difference in both the a-dimension and the monoclinic angle which presumably relates to the significant Cu content in glikinite (Table 1). Atom coordinates and thermal displacement parameters are given in Table 4 and selected interatomic distances in Table 5. The crystallographic information files have been deposited with the Principal Editor of Mineralogical Magazine and are available as Supplementary material (see below).
Table 3. Crystallographic data and refinement parameters for glikinite.

Table 4. Coordinates, isotropic and anisotropic displacement parameters (Å2) of atoms in glikinite.
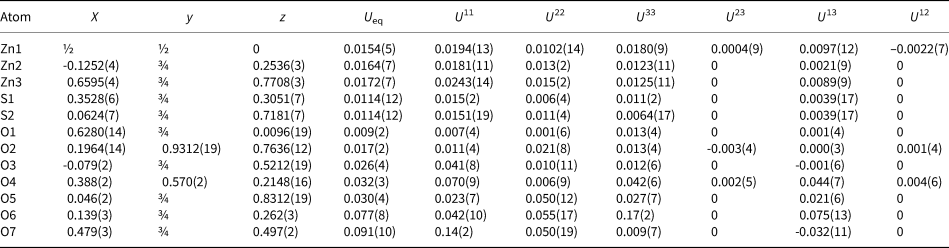
Table 5. Selected interatomic distances (in Å) in glikinite.
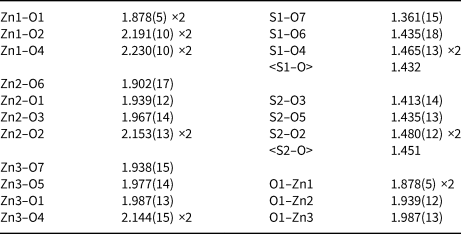
Discussion
The crystal structure of glikinite contains three symmetrically independent Zn sites with two types of different coordination environments (Fig. 2). The Zn1 site is coordinated by six oxygen ligands thus forming ZnO6 distorted octahedra. The Zn2 and Zn3 sites have a strongly distorted trigonal bipyramidal coordination environment consisting of five oxygen atoms each. There are two symmetrically independent S sites in the crystal structure of glikinite. The S6+ cations have typical but distorted tetrahedral coordination. Generally, coordination environments of atoms in glikinite are very similar to those in synthetic Zn3O(SO4)2 (Bald and Grühn, Reference Bald and Grühn1981). The significant amount of copper determined by microprobe in the structure of glikinite does not significantly influence the bond length and angle values in comparison with the structure of synthetic Zn3O(SO4)2. The crystal structure of glikinite is an example of a quenched high-temperature structure. Equal distribution of Cu2+ over three symmetrically independent Zn sites in glikinite can be hypothesised. Thus glikinite is a Zn,Cu analogue of synthetic Zn3O(SO4)2 (Table 6) (Bald and Grühn, Reference Bald and Grühn1981).

Fig. 2. Coordination of atoms in glikinite.
Table 6. Crystal chemical data for glikinite, the related synthetic compound, and vergasovaite.
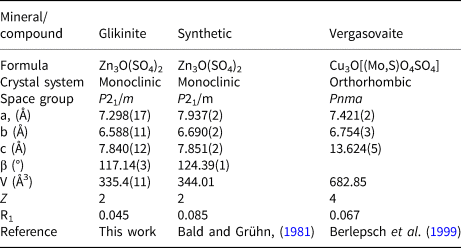
The structure of glikinite contains seven O2– anions. The O2–O7 atoms belong to the sulfate groups. The O1 atoms are tetrahedrally (Fig. 2) coordinated by four Zn atoms forming short and strong O–Zn bonds. From the viewpoint of the bond-valence theory, these bonds are the strongest in the structure and thus it makes sense to consider the O2– anions as coordination centres for oxocentred O1Zn4 tetrahedra (Krivovichev et al., Reference Krivovichev, Mentré, Siidra, Colmont and Filatov2013). OZn4 tetrahedra share common corners thus forming [Zn3O]4+ chains (Fig. 3). A similar topology [Cu3O]4+ of chains was described previously in the structures of vergasovaite Cu3O[(Mo,S)O4SO4] (Berlepsch et al., Reference Berlepsch, Armbruster, Brugger, Bykova and Kartashov1999) and kamchatkite KCu3OCl(SO4)2 (Siidra et al., Reference Siidra, Nazarchuk, Zaitsev, Lukina, Avdontseva, Vergasova, Vlasenko, Filatov, Turner and Karpov2017). Sulfate groups interconnect [Zn3O]4+ chains into 3D framework (Fig. 4) in glikinite.

Fig. 3. [Zn3O]4+ chain in the structure of glikinite.

Fig. 4. General projection of the crystal structure of glikinite along the b axis. OZn4 polyhedra are added on the right (SO4 = yellow and OZn4 = red).
Supplementary material
To view supplementary material for this article, please visit https://doi.org/10.1180/mgm.2020.33
Acknowledgements
We are grateful to an anonymous reviewer, Ian Graham, Stuart Mills and Peter Leverett for valuable comments. This work was financially supported by the Russian Science Foundation, grant no. 16-17-10085. Technical support by the SPbSU X-ray Diffraction and Geomodel Resource Centres is gratefully acknowledged.