Introduction
Body size is a key determinant of individual fitness with potential effects on survival, resource acquisition, mate choice, reproductive success, longevity, and other important life-history traits (Roff, Reference Roff1992; Stearns, Reference Stearns1992). For example, Honek (Reference Honek1993) used data from 68 insect species in ten families to characterize a general relationship between female body size and fecundity and found a regression slope close to one, which would imply that female size is a principle evolutionary constraint on potential fecundity. Similarly, Nedved and Honek (Reference Nedved, Honek, Hodek, van Emden and Honek2012), used data for 119 species of Coccinellidae to identify a power function that described the change in body mass with body length and observed that ovariole number was almost linearly correlated with this function. However, the large size can also incur various costs, and many extrinsic factors act as constraints on body size in evolutionary time; interactions between these intrinsic and extrinsic factors generate the typical median body size observed in any population or species and govern the range of variation around it. In insects and other arthropods, male fitness tends to be less sensitive to body size than female, given the energetic demands of egg production (but see Pincheira-Donoso and Hunt, Reference Pincheira-Donoso and Hunt2017); thus males tend to be generally smaller than females unless large size provides males with an advantage in mating or acquiring females (e.g. Grossi et al., Reference Grossi, Solis, Veloso and Canals2016; Rohner et al., Reference Rohner, Blanckenhorn and Puniamoorthy2016). Despite the costs, the largest individuals in any given insect population are generally the most fit, not only because they have accumulated greater reproductive ‘capital’ during larval development, but also because their larger size enables them to consume more resources as adults, which can translate into greater ‘income’ during reproduction (Jonsson, Reference Jonsson1997; Vargas et al., Reference Vargas, Michaud and Nechols2013). The provisioning of insects with ad libitum food is the norm in laboratory cultures but is not representative of the natural situation in insect populations, where many adults at the small end of the spectrum fail to achieve their genetic size potential as a result of food deprivation in immature stages.
Beyond advantages in reproductive success, large parental body size can also confer more subtle advantages to offspring via condition-dependent parental effects that can impact progeny fitness and survival probability (Fox and Mousseau, Reference Fox, Mouseau, Mousseau and Fox1998). For example, in the large rhinocerus beetle, Trypoxylus dichotomous (L), maternal body size has been shown to affect offspring body size via effects on egg size (Kojima, Reference Kojima2015). Dixon (Reference Dixon2000) showed that the fecundity of coccinellid beetles generally correlates quite well with both adult weight and longevity. In coccinellids as in other insects, the large body size is generally associated with a good physical condition, but can only be achieved through the acquisition of adequate larval resources (Vargas et al., Reference Vargas, Michaud and Nechols2013). Consequently, entire cohorts that mature under suboptimal conditions, such as a season in which food is scarce, may consist of individuals that are below the long term average for body size. In the Coccinellidae, a cohort of small beetles will produce smaller than usual cohort of offspring, because they produce small egg clusters containing fewer eggs while maintaining egg size relatively constant (Dixon and Guo, Reference Dixon and Guo1993).
Whenever condition-dependent parental effects are sensitive to body size, such size-disadvantaged cohorts could bequeath various transgenerational constraints on the fitness of subsequent generations, with additional consequences for population dynamics. For example, Kendall et al. (Reference Kendall, Ellner, McCauley, Wood, Briggs, Murdoch and Turchin2005) tested three mechanistic models to explain long term population cycles of the pine looper moth, Bupalus piniarius (Lepidoptera: Geometridae) and obtained the best fit with a model based on maternal effects associated with the pupal size. Similarly, Gibbs and van Dyck (Reference Gibbs and van Dyck2009), in exploring the consequences of habitat fragmentation for population dynamics, concluded that maternal effects may be important, although largely unexplored, mechanism whereby environmental conditions can have transgenerational consequences. Unfortunately, very few studies have explicitly tested the impact of body size on parental effects in insect species, despite considerable attention its direct effects on fitness (but see Najafpour et al., Reference Najafpour, Rasekh and Esfandiari2018).
Given that the fitness consequences of body size will diverge between males and females whenever the net effects of natural and sexual selection differ between the sexes (Rohner et al., Reference Rohner, Blanckenhorn and Puniamoorthy2016), the parental effects of body size may also diverge between mothers and fathers. Previous work on the Nearctic lady beetles Coleomegilla maculata DeGeer and Hippodamia convergens Guerin-Meneville (Coccinellidae) has demonstrated parental effects of body size on both egg size and progeny developmental phenotypes (Vargas et al., Reference Vargas, Michaud and Nechols2012a,Reference Vargas, Michaud and Necholsb,Reference Vargas, Michaud and Necholsc). However, male and female body size was manipulated in parallel within treatments in these experiments, with no attempt to resolve gender-specific impacts of body size on maternal vs. paternal effects.
The multicolored Asian ladybeetle, Harmonia axyridis Pallas (Coleoptera: Coccinellidae), is a voracious arthropod predator that has successfully invaded various agricultural ecosystems across Europe, Africa, and North and South America (Michaud, Reference Michaud2002; Brown et al., Reference Brown, Thomas, Lombaert, Jeffries, Estoup and Handley2011; Ukrainsky and Orlova-Bienkowskaja Reference Ukrainsky and Orlova-Bienkowskaja2014; Lombaert et al., Reference Lombaert, Guillemaud, Lundgren, Koch, Facon, Grez, Loomans, Malausa, Nedved, Rhule, Staverlokk, Steenberg and Estoup2014; Grez et al., Reference Grez, Zaviezo, Roy, Brown and Bizama2016). A high degree of phenotypic plasticity is among the traits often inferred to contribute to the success of H. axyridis as an invasive species (Roy and Brown, Reference Roy and Brown2015), and this includes an ability to mature at a wide range of adult body sizes. As in most coccinellids, H. axyridis females tend to be larger than males, likely because of the correlation between body size and female fecundity (Honek, Reference Honek1993, Reference Honek, Hodek and Honek1996). However, male H. axyridis invest heavily in seminal fluid proteins that have allomonal effects on both female fertility and progeny developmental phenotypes (Wang et al., Reference Wang, Michaud, Tan, Murray and Zhang2013; Michaud et al., Reference Michaud, Abdelwahab, Canassa and Bain2018). In the present study, we used H. axyridis as a model species to investigate the gender-specific impacts of adult body size, and hence overall physical condition, on the parental effects that shape progeny developmental phenotypes. We hypothesized that offspring of smaller parents would suffer fitness costs in the form of reduced final body size, even when reared on ad libitum food. Second, we hypothesized that offspring would respond more strongly to epigenetic signals from parents of the same sex, given that parental effects result from differential patterns of gene expression, which could be gender-specific across generations just as they are within generations.
Material and methods
Insect colony
A colony of H. axyridis was established from adult beetles collected from a sorghum field at the Agricultural Research Center-Hays, Hays, Kansas, USA, in June 2017. The adults were held in 1 L glass mason jars, ca. 150–160 beetles per jar, filled with strips of wax paper to serve as harborage and provisioned daily with frozen eggs of the Mediterranean flour moth, Ephestia kuehniella Zeller (Lepidoptera: Pyralidae), with water provided on a cotton wick. Jars were kept in a growth chamber set to 18 ± 1 °C, 50–60% RH and 12:12 L:D photoperiod. Previous work has shown these conditions are ideal for maintaining beetles in reproductive diapause for extended periods (Michaud and Qureshi, Reference Michaud and Qureshi2006).
Experimental beetles
In order to produce parental beetles for the experiment, a series of 30 adult females were removed from the stock colony, isolated in plastic Petri dishes (5.5 cm diam), and provisioned with frozen eggs of E. kuehniella with water provided on a small cube of sponge, both refreshed daily. The dishes were held in a climate-controlled growth chamber set to 23 ± 1 °C, 50–60% RH, and a 16:8 L:D day length. All life stages of experimental insects were held under these physical conditions. Eggs were collected daily from ovipositing females by transferring the beetles to clean Petri dishes. Eggs were dislodged from adhesion to the dishes using a fine camel hair brush wetted with a dilute (1.0%) solution of Clorox® bleach; dislodged eggs were then transferred individually to a piece of filter paper. For both experiments, neonate larvae were isolated in Petri dishes (5.5 cm diam) upon eclosion, labeled according to their maternal lineage to prevent the subsequent pairing of siblings, and then assigned to one of two treatments, ensuring that equal numbers of larvae from each clutch were assigned to each treatment.
Egg size experiment
Because egg size is a maternal effect possibly influenced by the parental size, a preliminary experiment was conducted to determine whether small couples, produced by an extreme restriction of larval food, would produce smaller eggs than larger couples. Ideally, we would have measured all eggs produced in the reciprocal crosses, but the collection and measuring of eggs is a very time-consuming process and time and labor constraints made this impossible in the larger experiment. Therefore, a series of 30 larvae were reared with ad libitum access to E. kuehniella eggs (more than could be completely consumed by that particular larval stage in 24 h), and another 50 were reared with only 30 min daily access to food. The larger number was reared in the latter treatment to compensate for an expected high rate of larval mortality. Both treatments had continuous access to water on a cube of sponge, which was replaced daily, and were held under the same physical conditions as the stock colony.
Adults were weighed and sexed within 24 hours of emergence, and then provisioned with ad libitum E. kuehniella eggs and water on a cube of sponge, both replaced daily. After 7–10 days post-emergence, females were paired with unrelated males from the same treatment in the same Petri dishes. Once females began ovipositing, their egg clusters were collected daily by transferring the adult beetles to clean dishes. Eggs were carefully dislodged from adhesion to the substrate using a dilute (1.0%) solution of The length and width of a subsample of five eggs from each female's daily oviposition were measured (to the nearest 0.02 mm) on a stage micrometer under a stereomicroscope at 50X magnification, until a total of 10 days' oviposition had been sampled for each female. These measurements were used to estimate egg volume based on the assumption that eggs were elipsoidal in shape (Takakura, Reference Takakura2004):

Reciprocal crosses
In order to obtain parental beetles of two different sizes for this experiment, a total of 240 neonate larvae were isolated in Petri dishes (5.5 cm diam) and labeled according to their maternal lineage to prevent the subsequent pairing of siblings. Larvae were then divided into two groups, 100 provided ad libitum food access (24 h daily), and 140 provided restricted food access (4 h daily), with the larger number allocated to the food deprivation treatment to compensate for higher expected juvenile mortality. The latter period of food access was selected based on the observation that both survival and fecundity were unacceptably low in the previous experiment when larvae were allowed only 30 min of food access daily. Individuals of both groups were each provisioned daily with a surfeit of E. kuehniella eggs (more than could be completely consumed by that particular larval stage in 24 h), plus water on a cube of sponge, but the food deprivation group had access to moth eggs for only 4 h each morning, with only water available for the rest of day. Adults of both groups were weighed and sexed within 24 h of emergence, isolated in plastic Petri dishes (5.5 cm diam) and provisioned with E. kuehniella eggs and water ad libitum, both refreshed daily.
Virgin adult beetles ranged from 7 to 10 days old when they were brought together in pairs. All beetles were paired with non-siblings in one of four reciprocal crossing treatments representing all possible male-female combinations of size. Mating treatments (n = 20 pairs per treatment) were established as follows: T1:♀L × ♂L, T2: ♀L × ♂S, T3: ♀S × ♂L, and T4: ♀S × ♂S, where ‘L’ and ‘S’ indicate large and small, respectively. Following mating, couples were again isolated in Petri dishes (5.5 cm diam) and provided with E. kuehniella eggs and water, refreshed daily. After the initial mating, males were isolated in separate Petri dishes with food and water and reunited with the same females every other day to maintain their fertility. All eggs were collected daily during a period of 21 reproductive days, tallied from the day each female laid her first clutch. All egg clusters were counted and then monitored twice daily until eclosion so that egg development times could be determined to the nearest 12 h; egg fertility (percent hatching) was recorded. Data from any couples that did not produce 21 egg clutches were discarded.
We also reared a series of ten larvae from the second clutch of each female, isolating neonates in Petri dishes and rearing them with ad libitum food as previously described. Larvae were examined twice daily and all developmental transitions were recorded in 12 h increments until they emerged as adults. Adults were sexed and weighed on an analytical balance within 24 h of emergence.
Statistical analysis
All life history parameters (developmental times, egg volume, and adult fresh weights) were analyzed by one-way ANOVA after passing tests for normality (Shapiro–Wilk test) and equality of variance (Levine's test). Whenever the main effect of treatment was significant in the reciprocal crossing experiment, means were separated by the Bonferroni test with correction for the experiment-wide error rate (α < 0.05).
Results
Egg size experiment
Only 34% of larvae survived to emerge as viable adults in the 30 min food limitation treatment, nine males and eight females, which enabled the formation of eight pairs in this treatment. In contrast, survival in the ad libitum treatment was 93.3%, and a series of eight females were randomly selected and paired with eight unrelated males. Mean fresh weight of females in the 30-min treatment was less than half that of females in the ad libitum treatment (15.4 mg vs. 31.4 mg). One pair in the 30 min treatment produced only infertile eggs that collapsed shortly after oviposition and had to be excluded from consideration; other females in this treatment died before producing 10 days of oviposition or laid fewer than five eggs in some clutches. To compensate for this, we measured more than ten clutches for some pairs until we had measured a total of 200 eggs in each treatment, representing samples of 158 clusters in total. When the mean egg volumes of each cluster were subjected to analysis, there was no significant difference in egg volume between treatments (30 min: mean ± SEM = 0.0325 ± 0.0007 mm3; ad libitum: mean ± SE = 0.0316 ± 0.0004 mm3; F 1,156 = 1.24, P = 0.268).
Reciprocal crosses
Females were significantly heavier than males in both the ad libitum feeding treatment (F 1,117 = 49.1, P < 0.001) and in the food limitation treatment (F 1,104 = 16.6, P < 0.001). Limiting food access to 4 hours daily reduced male fresh weight by 41% compared to those that had continuous access to food (mean ± SE = 16.7 ± 0.4 vs. 28.5 ± 0.5 mg; F 1,114 = 365.0, P < 0.001) and female fresh weight by 44% (mean ± SE = 18.8 ± 0.3 vs. 33.5 ± 0.5 mg; F 1,117 = 532.1, P < 0.001). The preoviposition period was unaffected by mating treatment, but 20-day fecundity was highest when both parents were large, and lowest when both parents were small, with reciprocal crosses intermediate and not significantly different from each other (Table 1). Similarly, the largest percentage of eggs hatched when both parents were large, and the smallest percentage when both parents were small, with reciprocal crosses intermediate.
Table 1. Mean (±SE) preoviposition periods, 20-day fecundities, and egg fertilities of Harmonia axyridis adults reared on two different feeding regimes (4 h daily access vs. ad libitum access to eggs of Ephestia kuehniella), with the resulting small and large females (♀S and ♀L) paired in all possible combinations with small and large males (♂S and ♂L)

Values followed by the same letter were not significantly different (ANOVA followed by Bonferroni, α = 0.05).
There was a significant effect of mating treatment on the eclosion time of eggs (F 3,619 = 21.7, P < 0.001), the duration of larval development (F 3,619 = 15.5, P < 0.001), and time spent in pupation (F 3,619 = 3.9, P = 0.009; Fig. 1). The fastest eclosion and pupation of progeny occurred when both parents were small, and the slowest when both parents were large, with other treatments intermediate. The slowest larval development occurred when small females were mated by large males, with other treatments not significantly different. The total duration of immature development (egg + larva + pupa) also differed among treatments (F 3,619 = 5.8, P = 0.001), the slowest development occurring in the progeny of small females mated to large males and the fastest when either large or small parents were paired together.
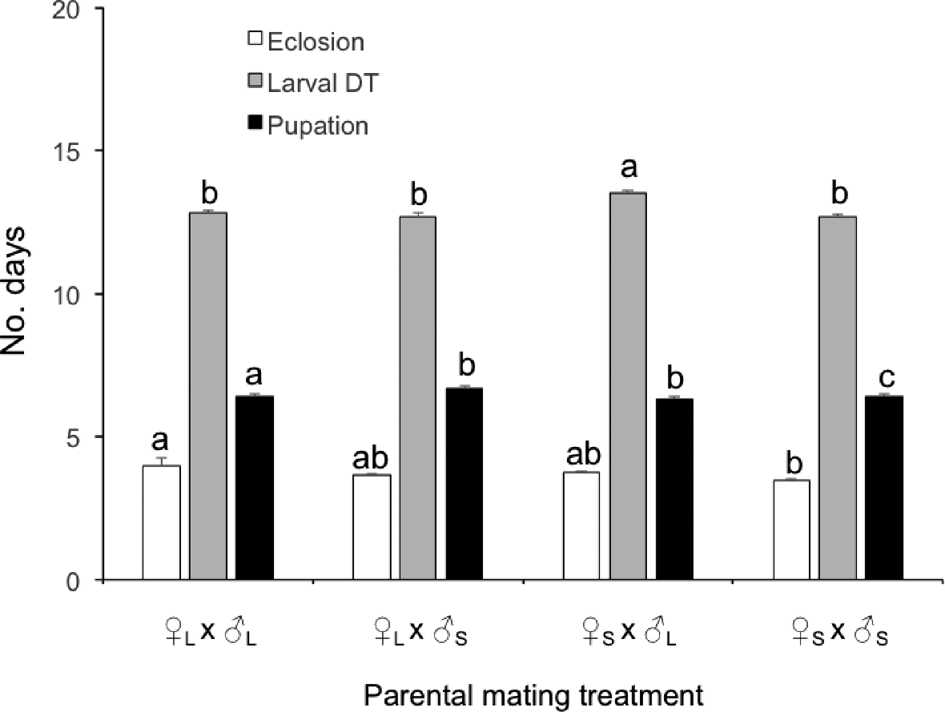
Figure 1. Mean (+SE) time (in days) required for eclosion of eggs, larval development (Larval DT) and pupation, for the progeny of small and large Harmonia axyridis females (♀S and ♀L) paired in all possible combinations with small and large males (♂S and ♂L). For a given parameter, columns bearing the same letter were not significantly different among treatments (ANOVA followed by Bonferroni, α = 0.05).
Parental mating treatment significantly affected adult fresh weight at emergence for males (F 3,301 = 3.3, P = 0.021) and females (F 3,314 = 4.5, P = 0.004). Both male and female progeny were heaviest when both parents were large, but the smallest daughters were produced by small females and the smallest sons when small fathers mated large mothers (fig. 2).

Figure 2. Mean (+SE) fresh adult weights (in mg) of male and female progeny of small and large Harmonia axyridis females (♀S and ♀L) paired in all possible combinations with small and large males (♂S and ♂L). Columns bearing the same letter were not significantly different among treatments, within genders (ANOVA followed by Bonferroni, α = 0.05).
Discussion
The larval food deprivation treatment was successful in producing a cohort of H. axyridis adults that weighed significantly less than the one reared with unlimited access to food. Although 21-day fecundities were significantly reduced only when mothers were small, egg fertility was reduced equivalently when either parent was small, and even more so when both parents were small, demonstrating substantial fitness costs of reduced body weight in both sexes. Small adults also gave rise to offspring that emerged at smaller adult sizes, even when reared on ad libitum food. Interestingly, even very small females produced by extreme larval food deprivation did not produce smaller eggs than those reared on ad libitum food, suggesting that small females do not trade off egg size in order to increase egg number, as inferred by Dixon and Guo (Reference Dixon and Guo1993), and that differences in egg size were not implicated in the other parental effects observed.
Offspring body size responded more strongly to epigenetic signals from the same-sex parent, supporting both of our initial hypotheses. Relatively few studies have explored whether parental effects may be gender-specific for progeny, despite the fact that parental effects result from differential patterns of gene expression, which could be gender-specific across generations just as they are within generations. In the eastern mosquito fish, Gambusia holbrooki Girard, age-specific maternal effects result in older mothers producing smaller daughters, but not smaller sons, whereas paternal age affects relative genital size in sons, but not body size (Vega-Trejo et al., Reference Vega-Trejo, Kruuk, Jennions and Head2018). In the neriid fly, Telostylinus angusticollis, paternal effects on body size varied between sons and daughters, whereas maternal effects did not (Bonduriansky et al., Reference Bonduriansky, Runagall-McNaull and Crean2016). Because sexual differences are largely a consequence of gender-specific patterns of gene expression, it is not surprising that epigenetic signals from parents could have gender-specific effects on gene expression in progeny.
The parental effects of body size on progeny developmental time were not so clear-cut. Large parents produced eggs that took longer to hatch than those of small parents, with reciprocal crosses intermediate, which is consistent with a symmetric effect of maternal and paternal body size on the speed of embryological development. The same was not true for larval development time, which usually contributes to the largest amount of variation in total duration of immature stages in coccinellids. Larval development was extended only in the progeny of small females when they were mated to large males. This would suggest an asymmetric interaction between maternal and paternal body size effects, but one which is difficult to interpret given that progeny of small pairs did not differ from those of large pairs in this regard. However, pupation times varied in a manner that was consistent among parental mating treatments and reflected the pattern observed for eclosion times; the progeny of large parents spent longer in pupation than those of small parents, with both reciprocal crosses intermediate.
Generally, the adult body size is inversely correlated with developmental time in coccinellids, i.e., faster development is generally associated with a larger size at emergence (Honek, Reference Honek, Hodek and Honek1996; Michaud, Reference Michaud2005; Vargas et al., Reference Vargas, Michaud and Nechols2012b), and this holds true for H. axyridis also, at least when food sources vary in suitability for larval development (e.g., Michaud, Reference Michaud2000; Wolf et al., Reference Wolf, Romeis and Collatz2018). However, the present results suggest that a trade-off between body size and developmental time can emerge at the individual level; either slower embryonic and pupal development is required when parental effects cue growth to a larger adult weight or they reflect pleiotropic effects of epigenetic signals unrelated to weight gain. The relationship between developmental time and adult body weight may also change under conditions of food-limitation. For example, when subjected to severe dietary restriction, larvae of C. maculata are more likely to add or subtract larval stadia, even though supernumerary instars delay development (Vargas et al., Reference Vargas, Michaud, Nechols and Moreno2014). Moreover, there exists considerable interspecific variation in parental effects on developmental time and adult size in the Coccinellidae. In H. axyridis, offspring of later clutches mature to larger sizes than those of earlier clutches but spend significantly longer feeding as larvae (Michaud et al., Reference Michaud, Abdelwahab, Canassa and Bain2018). In contrast, larvae of later clutches develop faster to achieve larger body sizes in H. convergens (Vargas et al., Reference Vargas, Michaud and Nechols2012c), whereas there are no age-specific maternal effects on either trait in C. maculata (Vargas et al., Reference Vargas, Michaud, Nechols and Moreno2014) or Eriopis connexa (Germar) (Colares et al., Reference Colares, Michaud, Torres and Silva-Torres2015). Therefore, coccinellid species exhibit considerable variation in the ways their developmental phenotypes can be modified by parental effects, likely as a function of divergent ecologies.
The insight that food limitation in one generation can negatively impact the fitness and body size potential of subsequent generations has important implications for the conservation of biological control in field crops. In conventional industrial-scale farming, large populations of predators seasonally colonize a series of synchronous monocultures, encountering relatively uniform conditions over large areas. These findings underscore the importance of tolerating sub-economic levels of pests in these crops, as adequate prey availability is essential for preserving the fitness of predator populations across generations as they move among crops in the agricultural landscape. For example, the currently wide-spread practice of treating seeds with systemic insecticides is effective in reducing pest pressure during early stages of crop growth, but entails risks for potential negative transgenerational impacts on the fitness of entire predator populations via prey deprivation effects, apart from its direct environmental and toxicological impacts.
In the case of opportunistic and invasive species such as H. axyridis, the transgenerational effects of large body size could accelerate species evolution and ecological range expansion, for example when abundant novel prey can be successfully exploited in an invaded range (Grez et al., Reference Grez, Zaviezo, Roy, Brown and Segura2017), or when novel invasive prey species achieve outbreak population levels (Michaud, Reference Michaud2018). The resulting large populations of high-condition individuals produced by well-fed parents could have pronounced impacts on intraguild competitors, especially native coccinellid species, ultimately altering community composition (Zaviezo et al., Reference Zaviezo, Soares and Grez2019). Whether a population that achieves large adult size will give rise to another generation of large, high-condition offspring will remain contingent on the food supply they encounter, but populations of large individuals will intensify intraguild interactions with competing species, and potentially enable niche expansion by the invader. We conclude that reduced body size due to prey deprivation during development has the potential to exert transgenerational constraints on the fitness of predatory species via epigenetic effects. Conversely, outbreaks of suitable prey that produce large, well-fed predators can potentially provide an epigenetic boost to the fitness of the subsequent generation, creating a positive transgenerational effect that could enhance the ecological success of highly competitive species such as H. axyridis.
Acknowledgements
The authors are grateful to Hannah Stowe and Nagwan Ellawatty for laboratory assistance. This work was funded, in part, by agreement no. 58-3072-6-006 from the Agricultural Research Service, US Department of Agriculture and is contribution no. 20-024 of the Kansas Agricultural Experiment Station.