Introduction
Seed quality with respect to germination and seedling establishment is important for seed producers and farmers. This quality is influenced by various factors, including seed production conditions, harvesting, cleaning, sorting, seed treatments and seed health. In this paper we use the term ‘quality’ to refer to germination characteristics including the percentage or speed of germination, and the percentage of normal seedlings.
Premature harvest of seeds is known to have a negative effect on seed germination and tolerance to adverse conditions, e.g. hot water treatments (Jalink et al., Reference Jalink, van der Schoor, Frandas, van Pijlen and Bino1998; Groot et al., Reference Groot, Birnbaum, Rop, Jalink, Forsberg, Kromphardt, Werner and Koch2006). There is a need for a fast test to determine the quality of seeds to optimize the settings for seed sorting based on maturity or hot water treatments in the seed industry.
Seeds may accumulate damage during storage or treatments. The degree of the deterioration varies through the amounts of stress imposed by these processes. This deterioration may result in reduced germination and seedling emergence, which is not necessarily easy to predict. Matthews and Khajeh-Hosseini (Reference Matthews and Khajeh-Hosseini2006, Reference Matthews and Khajeh-Hosseini2007) showed a clear negative correlation between the mean time required for radicle protrusion and the average, final shoot length or the mean time for emergence from soil, by comparing ten maize seed lots. These results were explained by possible, prolonged periods of metabolic repair of the damage. Analysis of the degree of damage might predict germination performance of a seed lot. Mitochondria are known to be among the primary sites of deterioration, for instance during seed ageing (Bewley and Black, Reference Bewley and Black1994).
Upon imbibition metabolic processes are re-initiated, including transcription, translation and enzyme activation. All these processes require the production of energy, generated by aerobic or anaerobic respiration. During aerobic respiration pyruvate is catabolized in the citric acid cycle, which proceeds in the mitochondria. This citric acid cycle generates NADH and FADH2 which are oxidized in the electron transport chain. During electron transport a proton gradient is established over the inner mitochondrial membrane, which drives the production of ATP via ATPase. Glycolysis, which takes place in the cytosol, is the primary source of ATP in the absence of functional mitochondria. During glycolysis glucose is converted into two pyruvate molecules and NAD+ is reduced to NADH which is oxidized to NAD+ in functional mitochondria (Fan et al., Reference Fan, Higashi, Frenkiel and Lane1997; Rasmusson et al., Reference Rasmusson, Soole and Elthon2004).
Although lack of oxygen (e.g. due to submergence) is often the major reason for fermentation to occur, it may also take place under aerobic conditions. For example, during pollen tube growth both aerobic respiration and fermentation occur (Bucher et al., Reference Bucher, Brander, Sbicego, Mandel and Kuhlemeier1995).
During imbibition in pea seeds, it takes some time for the mitochondria to reconstitute to a morphologically ‘mature’ structure with a folded inner membrane (Benamar et al., Reference Benamar, Tallon and Macherel2003). When mitochondria are not functional, an alternative mechanism of regenerating NAD+ is needed. This is provided by the conversion of pyruvate to ethanol or lactic acid during fermentation (Smith and Rees, Reference Smith and Rees1979; Feireira de Sousa and Sodek, Reference Feireira de Sousa and Sodek2002).
Many plant species are known to have the ability to produce ethanol (Cossins and Beevers, Reference Cossins and Beevers1963). Elevated levels of ethanol and its precursor, acetaldehyde, were detected in soybean seeds 30 min after the beginning of imbibition (Woodstock and Taylorson, Reference Woodstock and Taylorson1981). The soybean seeds were thought unable to generate adequate energy (ATP) via aerobic respiration early in imbibition and employed anaerobic respiration, or fermentation, as an alternative mechanism of generating energy.
Among many factors affecting seed performance is the damage that accumulates in the seed during drying and storage, for instance that to DNA and cell, including mitochondrial, membranes (Bewley and Black, Reference Bewley and Black1994). When mitochondria are non-functional due to the damage, initiation of anaerobic respiration is expected to deliver the energy for metabolic processes, at least for repair. Indeed, a relation between ethanol production and seed deterioration, as evidenced by a decline in germination or seedling growth, has been suggested (Perry, Reference Perry1978; Woodstock and Taylorson, Reference Woodstock and Taylorson1981; Taylor et al., Reference Taylor, Johnson and Kataki1999; Kataki and Taylor, Reference Kataki and Taylor2001; Bicanic et al., Reference Bicanic, Persijn, Taylor, Cozijnsen, van Veldhuyzen, Lenssen and Wegh2003; Akimoto et al., Reference Akimoto, Cho, Yoshida, Furuta and Esashi2004; Rutzke et al., Reference Rutzke, Taylor and Obendorf2008). In those studies seed deterioration was imposed by natural or conditional ageing of the seeds and ethanol production was measured using gas chromatography, immobilized enzyme technology or laser-based photo-acoustic spectroscopy. For routine analyses of multiple samples, these analytical methods are relatively slow and costly. Buckley and co-workers (Buckley et al., Reference Buckley, Irvine, Buckley and Elliott2006; Buckley and Huang, Reference Buckley and Huang2011) introduced a simplified method of measuring seed-produced ethanol with a modified breath analyser. Using the latter method, we studied ethanol production by imbibed cabbage seeds of different maturity, after hot water treatments or natural ageing in the laboratory.
Materials and methods
Seed samples
Seeds of cabbage (Brassica oleracea L.) from a recently harvested commercial seed lot (cv. Stanton F1, Savoy winter cabbage) and an approximately 15-year-old, commercial seed lot (cv. Bartolo F1, white cabbage) were sorted into six fractions differing in maturity based on chlorophyll fluorescence (ScanMaster I, Satake, Houston, Texas, USA) (Jalink et al., Reference Jalink, van der Schoor, Frandas, van Pijlen and Bino1998). To test the effect of hot water treatments on seed germination and ethanol production, seeds of the highest maturity fraction (Bartolo, maturity fraction 6) were submerged for different periods of time (15, 30, 60 and 90 min) in tap water at 55°C. The seeds were then cooled with running tap water, blotted on filter paper and dried to 6% seed moisture (fresh weight basis) by incubating them for at least 3 d under circulating air at 32% relative humidity (RH) and 20°C. A relative humidity of 32% was maintained with a saturated solution of CaCl2. Seeds that were rinsed under tap water and dried, but not incubated in water at 55°C were used as a control.
Natural ageing was also investigated. A 9-month-old cabbage seed lot (cv. Amtrak F1, white cabbage) was stored under ambient conditions on a laboratory bench at about 20°C and 40–50% RH. At various times, subsamples were transferred to − 20°C to suspend ageing. About 31 months after the start of the experiment the seeds were retrieved from the − 20°C storage and subjected to a germination test and analysis of ethanol production.
Germination tests
Germination tests were performed using a Jacobsen germination table (Karma, Reference Karma1968) with two or four replicates of 50 seeds on filter paper. To maintain high humidity around the seeds an inverted plastic funnel with a hole in the conical end was placed over each filter paper with seeds. Two different germination assays were performed. In assay 1, seeds were incubated at 20°C with 8 h light (40–65 μmol m− 2 s− 1)/16 h dark. Seeds were scored as germinated when the radicle had protruded. Radicle emergence was counted daily to determine the speed of germination. Using GERMINATOR software (Joosen et al., Reference Joosen, Kodde, Willems, Ligterink, Van der Plas and Hilhorst2010), a curve-fitting program designed for the analysis of germination data, time required to reach 50% of the final germination (T 50) was calculated. The germination data were fitted to a four-parameter Hill function by iteration using the Solver tool (Microsoft Excel). The resulting curve formula was used to calculate germination parameters including the final percentage, speed and uniformity of germination. After 30 d, seedling quality, total germination percentage and percentage of normal seedlings were recorded. Normal seedlings were evaluated as described in the rulebook of the International Seed Testing Association (ISTA, 1999). In assay 2, seeds were germinated under continuous light (40–65 μmol m− 2 s− 1) with an alternating temperature of 20°C (16 h) and 30°C (8 h). As an indicator of seed quality, the number of normal seedlings established after 5 d was also counted. Final germination was determined after 7 d in this assay.
Ethanol assay
Five hundred milligrams of seeds (treated, sorted or control) equilibrated at 32% RH were placed in a 20 ml glass vial. Water was added to achieve the desired seed moisture percentage, taking into account the initial seed moisture content of 6%. The vials were sealed with aluminium crimp caps with 3-mm-thick butyl rubber and Teflon septa immediately after adding water. The vials containing wetted seeds were incubated at either 20°C or 40°C. Ethanol concentration in the headspace was measured at different time points using the modified breath analyser (Alcotest 6810, Dräger safety AG & Co. KGaA, Lübeck, Germany). To avoid temporary cooling, sample measurement outside the incubator was performed within 5 s.
The original breath analyser (Dräger 6810) was modified by replacing the breath sampling port with a Luer fitting and by making adjustments to the software. A disposable hypodermic needle (18 gauge, 5 cm long) was used to collect gas samples from the vials. The needle was shortened to approximately 2.5 cm and cut half through approximately 1.5 cm from the Luer hub to allow for pressure equilibration in the vial during its insertion. The device collected a subsample of approximately 0.3 ml from the headspace for every measurement. The analytical range of the breath analyser was 10–2500 μg ethanol per litre of gas mixture. The breath analyser was calibrated annually by the supplier.
Oxygen and carbon dioxide measurements
In a separate experiment, oxygen and carbon dioxide were measured in the headspace of 20 ml vials containing 500 mg of either the most mature or the least mature fractions of the Bartolo and Stanton seed lots. At the start of the experiment water was added to a final moisture content of 30%. The vials were then incubated at 40°C. After 6 h, oxygen and carbon dioxide were determined in 1 ml samples of headspace atmosphere by gas chromatography (Chrompack CP-2002 Micro-GC, Chrompack, Middelburg, The Netherlands). A Molsieve 5A PLOT column (Restec, Bellefonte, Pennsylvania, USA) was used for oxygen and a HayeSep A column (Valco Instruments Company Inc., Houston, Texas, USA) for carbon dioxide. The data were analysed using the Maestro II software (Gerstel GmbH & Co. KG, Mülheim an der Ruhr, Germany).
Results
Hot water treatments
To optimize the ethanol assay, seed samples of different quality were produced using four durations (15, 30, 60 and 90 min) of a hot water treatment at 55°C. The hot water treatments were applied to mature Bartolo seeds and the subsequent effect on seed quality was determined using germination assay 1 with four replicates. Hot water treatment for 15 min delayed germination (i.e. increased T 50), but did not affect total germination of maturity fraction 6 (the most mature) of the Bartolo seed lot (Fig. 1). However, the frequency of normal seedlings declined. Longer hot water treatments decreased total radicle emergence, increased T 50 and strongly decreased the frequency of normal seedlings. After treatment for 1 h radicle protrusion failed in all seeds.

Figure 1 Effect of hot water treatments (55°C; 15, 30, 60 and 90 min) on germination performance of cv. Bartolo seeds (fraction 6: the most mature). Total germination percentage after 30 d (□), the percentage of normal seedlings (■) and the time to reach 50% of the final germination percentage (T 50,●) are shown (mean ± SE, n = 4).
Ethanol production from the hot water treated, deteriorated seeds was analysed after equilibrating them to five different seed moisture contents (20, 30, 40, 50 and 60%) and incubating them at 20°C (24 h) or 40°C (6 h). The samples showed a marked variation in ethanol production (Fig. 2a). Control seeds (only kept under running tap water for a short time) and seeds treated with hot water for 15 min did not produce ethanol at 20°C, whereas 30–90 min hot water treatments resulted in ethanol production (Fig. 2a). The highest ethanol production at 20°C was observed from the seeds treated with hot water for 60 min and incubated at 40% moisture at both 20°C and 40°C. Headspace ethanol concentrations were much higher in seed samples incubated at 40°C than those at 20°C (Fig. 2a and b). When no (control: 0 min) or only a short (15 min) hot water treatment was given, less ethanol was produced from seeds with 40% moisture than from those with 30, 50 or 60% moisture. This local minimum was reproducible in repetitive experiments (data not shown). Tests at other incubation temperatures (30, 35, 45, 50 and 60°C) confirmed that 40°C was the most efficient temperature to detect differential ethanol production from hot water treated cabbage seeds.

Figure 2 Effect of duration of hot water (55°C) treatment and seed moisture content (%) on headspace ethanol levels above 500 mg samples of cv. Bartolo seeds in 20 ml vials. Ethanol was measured after 24 h incubation at 20°C (a) or 6 h incubation at 40°C (b).
Seed maturity
The second set of experiments was performed on cabbage seeds varying in maturity. The seed quality of six maturity fractions, which were sorted on the basis of their chlorophyll fluorescence (i.e. decreasing chlorophyll indicating increasing maturity), was analysed using germination assay 1, with four replicates. The frequency of normal seedlings gradually increased from fraction 1 (the least mature, 69%) to fraction 6 (the most mature, 93%) (Fig. 3), while total germination was affected only in fraction 1 (the least mature seeds). After incubation at 20°C (30% moisture) for 24 h, headspace ethanol was detectable only from fraction 1 (the least mature) (Fig. 3). When seeds were incubated at 40°C for 6 h, much higher ethanol levels were found for seeds across all maturity fractions. Headspace ethanol levels decreased with increasing seed maturity and showed a negative correlation with the frequency of normal seedlings (r = − 0.97).
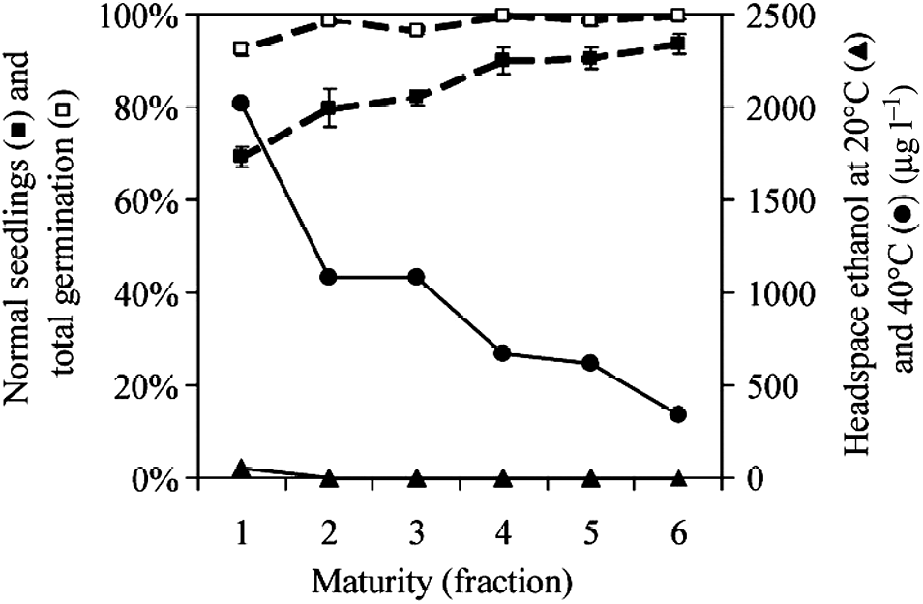
Figure 3 Seeds (cv. Bartolo) were sorted into six fractions differing in maturity based on their chlorophyll content, with fraction 1 (the highest chlorophyll content, i.e. the least mature) through fraction 6 (the lowest chlorophyll content, i.e. the most mature). The percentages of total germination and normal seedlings (mean ± SE, n = 4) were determined according to germination analysis 1. Headspace ethanol levels (500 mg samples, 20 ml vials and 30% final seed moisture) were measured after 24 h incubation at 20°C (▲) or 6 h incubation at 40°C (●).
Oxygen and carbon dioxide
The previous experiment was partly repeated and extended with maturity fractions 1 and 6 from the same Bartolo seed lot and from another cultivar (Stanton), using germination assay 1 with two replicates. The most mature fraction 6 performed better, in terms of the percentages of germination and normal seedlings, than the least mature fraction 1 for both seed lots (Fig. 4). Headspace ethanol, carbon dioxide and oxygen levels of fractions 1 and 6 of Bartolo or Stanton seeds were determined by gas chromatography after 6 h incubation at 40°C with a 30% moisture content. Ethanol and carbon dioxide levels were higher while oxygen was significantly lower for fraction 1 (the least mature) compared to fraction 6 (the most mature) for both cultivars (Fig. 4) (ANOVA, P = 0.02). Oxygen levels never dropped below 18% during the assay.

Figure 4 Effect of seed maturity on germination performance (germination assay 1) and respiration. Seeds of two cultivars Bartolo (B) and Stanton (S) differing in maturity were sorted based on their chlorophyll content into six fractions, fraction 1 (the highest chlorophyll content, i.e. the least mature) through fraction 6 (the lowest chlorophyll content, i.e. the most mature). The control was water. The percentages of final germination and normal seedlings are shown in (a). Headspace ethanol (b), oxygen (c) and carbon dioxide (d) levels were determined in the headspace after 6 h incubation at 30% seed moisture and 40°C. Values are means ± two data points.
Natural ageing
Quality variation was also obtained from naturally aged cabbage seeds (cv. Amtrak). Storage of the cabbage seeds at the laboratory bench resulted in a gradual decline in germination performance (germination assay 2, with two replicates) (Fig. 5). The number of normal seedlings after 5 d (germination assay 2: early evaluation) declined from 92 to 58% over a period of 31 months. The decline was less obvious (from 94 to 82%) when evaluation was performed after 7 d. When samples from these aged seeds were incubated at 20°C with 30% moisture for 24 h, headspace ethanol was detected from seeds stored for 19 months or longer (Fig. 5). Headspace ethanol levels were much higher when incubated at 40°C (3.5 h). Ethanol production was already detectable from seeds stored for 4.8 months when the 40°C incubation was used for ethanol assay. Prolonged (24 h) incubation at 40°C allowed ethanol detection from all samples, while ethanol production from seeds aged for 17 months or more reached the maximum detection limit.
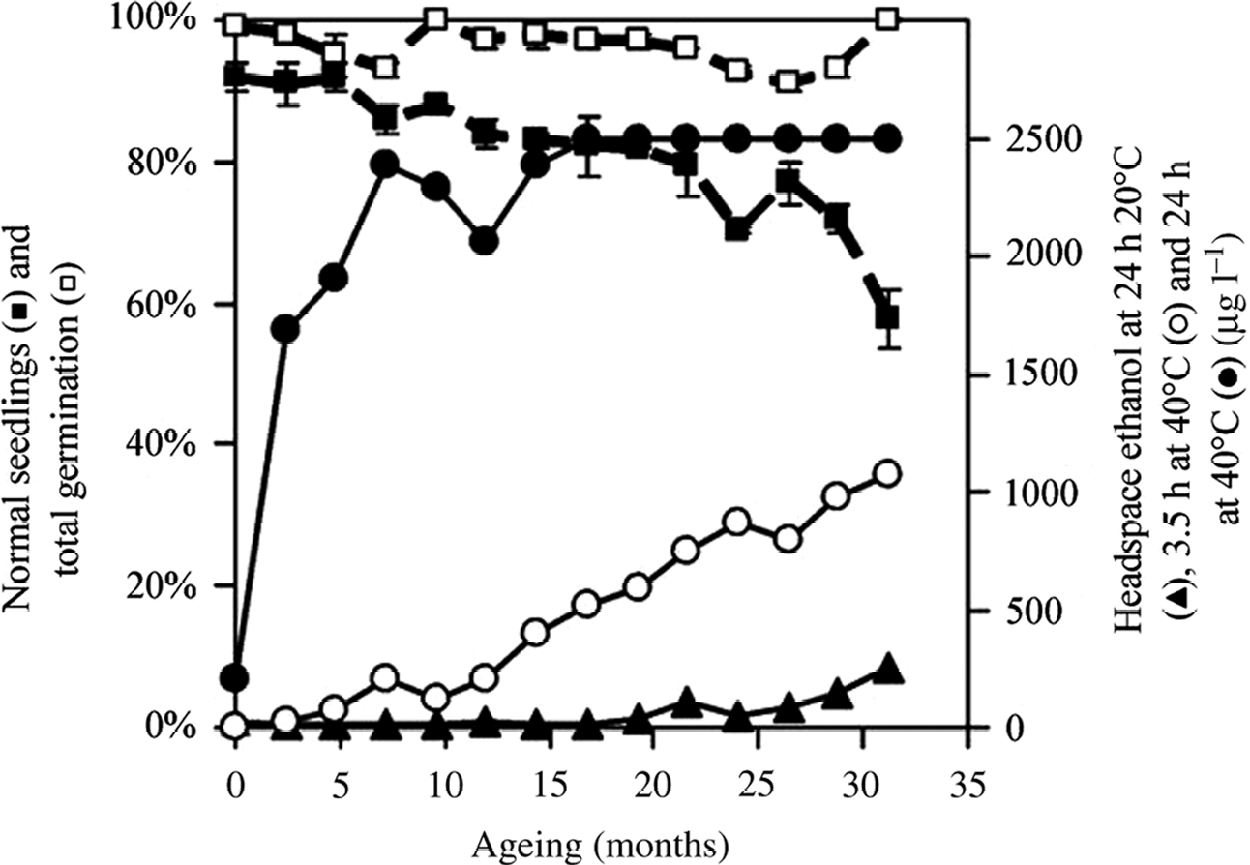
Figure 5 Effect of seed ageing on ethanol production. Cabbage seeds (cv. Amtrak) were naturally aged at about 20°C on a laboratory bench. Seed quality was assessed by the percentage of normal seedlings after 5 d (■) and total germination after 7 d (□) (mean ± two data points). Headspace ethanol was measured above 500 mg seed samples at 30% moisture in 20 ml vials after 24 h incubation (▲) at 20°C or after 3.5 h (○) or 24 h (●) incubation at 40°C.
The ethanol levels measured at 40°C after 3.5 h were negatively correlated (r = − 0.92) with the number of normal seedlings after 5 d. This correlation was slightly less (r = − 0.87) when the ethanol assay was performed at 20°C for 24 h. The amount of ethanol produced at 40°C after 3.5 h was almost linearly correlated (r = 0.98) with ageing time at the laboratory bench.
Discussion
Field emergence of seeds is an important aspect of crop establishment. Seed companies and growers are seeking reliable and rapid methods to predict field performance of their seeds. Field performance is a complex trait related to a collection of physiological and genetic factors, all of which cannot be measured in a single assay. Most likely, multiple tests will be needed to provide a comprehensive evaluation and reliable prediction of field performance.
Delouche and Baskin (Reference Delouche and Baskin1973) proposed a sequence of events during seed deterioration which leads to loss of germination and field performance. The first event in this model is membrane degradation. An ideal test, therefore, would be one that could measure membrane deterioration (McDonald, Reference McDonald1980).
As ethanol production most likely is initiated or enhanced by the loss of mitochondrial membrane integrity, an ethanol assay has good potential as a test to examine the level of deterioration in seeds. While ethanol can be quantified with research-oriented, analytical equipment, the assay has to be simple for practical purposes. In the present work we demonstrated that variation in cabbage seed quality can be analysed by measuring ethanol production with a hand-held breath analyser (Buckley et al., Reference Buckley, Irvine, Buckley and Elliott2006; Buckley and Huang, Reference Buckley and Huang2011).
The sensitivity of the assay was improved by incubating the seeds at 40°C instead of the moderate temperatures of 20 or 25°C used in previous studies (Perry, Reference Perry1978; Woodstock and Taylorson, Reference Woodstock and Taylorson1981; Taylor et al., Reference Taylor, Johnson and Kataki1999; Kataki and Taylor, Reference Kataki and Taylor2001; Bicanic et al., Reference Bicanic, Persijn, Taylor, Cozijnsen, van Veldhuyzen, Lenssen and Wegh2003; Akimoto et al., Reference Akimoto, Cho, Yoshida, Furuta and Esashi2004; Rutzke et al., Reference Rutzke, Taylor and Obendorf2008; Buckley and Buckley, Reference Buckley and Buckley2009; Buckley and Huang, Reference Buckley and Huang2011). The advantage of using 40°C for ethanol assay was also well exemplified by the analysis of different fractions of seeds differing in maturity (Fig. 3) and the naturally aged seeds with different quality (Fig. 5).
Our results also identified some precautions necessary for the reliable application of this method. The ethanol levels at 40°C after prolonged incubation (24 h) of the aged seeds exceeded those commonly measured with the breath analyser (e.g. human breath analysis by law enforcement). This potential problem can be addressed by a newer version of the analyser, which is dedicated particularly for use in agricultural research and application. The maximum ethanol detection limit of the modified breath analyser could be 9990 μg l− 1 which exceeds the previous detection limit of 2500 μg l− 1 (Burkhard Stock, Dräger safety AG&CO KGaA, pers. comm.).
In general, incubation at 40°C yielded a substantial amount of headspace ethanol within hours and enabled the entire assay to be run within a single working day. Higher temperatures are not recommended because discrimination of differences in seed quality was not improved (data not shown).
The increase of ethanol levels in the headspace at higher temperatures can be explained, in part, by the effect of temperature on the partitioning of ethanol in an air/water system. Elevated metabolism in seeds could additionally explain higher headspace ethanol levels at 40°C compared to those at 20°C. Pyruvate decarboxylase is one of the regulatory enzymes in the conversion of pyruvate to ethanol (Davies et al., Reference Davies, Grego and Kenworthy1974). The activity of this enzyme in wheat seeds is about five times greater at 40°C than at 20°C (Singer and Pensky, Reference Singer and Pensky1952). It is also possible that incubation at 40°C induced elevated ethanol production by additional seed deterioration, because 40°C is above the maximum germination temperature for cabbage seeds (Elson et al., Reference Elson, Morse, Wolf and Vaughan1992). Furthermore, immature or deteriorated seeds may be more sensitive to high temperature stress than high-quality seeds. The elevated headspace ethanol observed at 40°C may be due to a combination of physical and biological factors. During the assay oxygen levels did not drop below 18% (Fig. 4), indicating that ethanol production was not due to lack of oxygen.
A moisture content of 30% seems optimal for determining ethanol production from cabbage seeds (Fig. 2). Also, this is below the minimal moisture content (40%) required for radicle protrusion (data not shown). This agrees with previous research (Rutzke et al., Reference Rutzke, Taylor and Obendorf2008), in which the quality differences of artificially aged cabbage seed could be resolved better at 30% than at 60% moisture.
Hot water treatment of seeds is performed to reduce seed-borne diseases (McGee, Reference McGee1995). In practice, it is important to find a balance between effectiveness against pathogens and seed damage. Seed lots may differ in their sensitivity to hot water treatments, and therefore test treatments should be performed to determine the optimal conditions (Groot et al., Reference Groot, Birnbaum, Rop, Jalink, Forsberg, Kromphardt, Werner and Koch2006, Reference Groot, Birnbaum, Kromphardt, Forsberg, Rop and Werner2008). However, test treatments are time-consuming, and a rapid assay to determine potential negative effects on seed germination performance is needed. The ethanol assay developed here may offer such a rapid assay.
Acknowledgements
Dräger safety AG&CO KGaA (Germany) is acknowledged for providing modified breath analysers. The research presented here has been funded by the Netherlands Ministry of Economic affairs, Agriculture and Innovation in the frame of supporting organic agriculture through research.