Introduction
The Silurian Waukesha Biota of Wisconsin represents a rare occurrence of a Silurian Konservat-Lagerstätte, including preservation of lightly sclerotized and unsclerotized organisms—dominantly arthropods, but including organisms such as worms and a Panderodus conodont animal (Mikulic et al., Reference Mikulic, Briggs and Kluessendorf1985a, Reference Mikulic, Briggs and Kluessendorfb). Despite the rarity of exceptionally preserved Silurian biotas, little has been published on the systematics and taphonomy of the organisms contained therein. Systematic work on the Waukesha Biota has thus far been limited to descriptions of a non-calcified dasycladalean alga (LoDuca et al., Reference LoDuca, Kluessendor and Mikulic2003); a rare synziphosurine chelicerate (Moore et al., Reference Moore, Briggs, Braddy, Anderson, Mikulic and Kluessendorf2005); the oldest thylacocephalan crustacean (Haug et al., Reference Haug, Briggs, Mikulic, Kluessendorf and Haug2014); and initial assessments of the biota as a whole (Mikulic et al., Reference Mikulic, Briggs and Kluessendorf1985a, Reference Mikulic, Briggs and Kluessendorfb).
The Waukesha Biota occurs in an approximately 120 mm-thick interval of finely laminated, interbedded mudstone and dolomite 2 m from the bottom of a locally extensive paleoescarpment in the Brandon Bridge Formation (Mikulic et al., Reference Mikulic, Briggs and Kluessendorf1985a, Reference Mikulic, Briggs and Kluessendorfb). This interval contains a moderately diverse assemblage of soft-bodied organisms, many of which exhibit a remarkable state of preservation (Mikulic et al., Reference Mikulic, Briggs and Kluessendorf1985a, Reference Mikulic, Briggs and Kluessendorfb). The lower portion of the escarpment is overlain by a thin conglomerate of chert and rare silicified fossils in a glauconitic clay matrix, indicating a period of depositional hiatus (Mikulic et al., Reference Mikulic, Briggs and Kluessendorf1985a). The graptolite Monograptus spiralis (Geinitz, Reference Geinitz1842) and conodonts near the top of the Brandon Bridge strata at Waukesha suggest a late Llandovery to early Wenlock age (Mikulic et al., Reference Mikulic, Briggs and Kluessendorf1985a).
Three phyllocarid species, Ceratiocaris macroura Collette and Rudkin, Reference Collette and Rudkin2010; C. papilio Salter in Murchison, Reference Salter1859; and C. pusilla Matthew, Reference Matthew1889, occur in the Waukesha Biota. Ceratiocaris macroura and C. papilio characteristically occur in relatively nearshore facies (Salter in Murchison, Reference Salter1859; Salter, Reference Salter1860; Woodward, Reference Woodward1865; Rolfe and Burnaby, Reference Rolfe and Burnaby1961; Rolfe, Reference Rolfe1962; Mikulic et al., 1985 Reference Mikulic, Briggs and Kluessendorfa, Reference Mikulic, Briggs and Kluessendorfb; Collette and Rudkin, Reference Collette and Rudkin2010; Collette and Hagadorn, Reference Collette and Hagadorn2010), whereas C. pusilla, until now, was only known to occur in turbidite facies of the Jones Creek Formation, New Brunswick, which have been interpreted to possibly represent sediments transported into a marine trough by sediment gravity flows (Matthew, Reference Matthew1889; Copeland, Reference Copeland1957; Copeland, Reference Copeland1977). As such, it appears that the Waukesha phyllocarid assemblage may represent a transported assemblage, rather than all or part of a true biota, or that C. pusilla from the Jones Creek Formation represents a nearshore faunal element that was transported offshore by sediment gravity flows. The presence of nearly complete specimens, specimens with evidence of Salter’s position, and specimens represented by isolated pleomeres and furcae suggests that both corpses and molts are present in the assemblage.
Occurrence of more than one Ceratiocaris species in the Waukesha Biota is not unexpected, as both C. macroura and C. papilio are known to occur in the Eramosa Biota of Ontario; and C. acuminata Hall, Reference Hall1859, and C. maccoyanus Hall, Reference Hall1859, are known to occur in the Upper Silurian Bertie Group of New York (Collette and Hagadorn, Reference Collette and Hagadorn2010). The three species recognized herein are differentiated on the basis of the telson/furca length ratio, carapace length/depth ratio, details of the pleon and carapace cuticle ornamentation, and the number of pleonites protruding posteriorly from the carapace. Distinguishing characteristics of the species examined herein were reviewed by Collette and Hagadorn (Reference Collette and Hagadorn2010) and Collette and Rudkin (Reference Collette and Rudkin2010). New insight into the carapace cuticle ornamentation of C. macroura is discussed below.
Materials and methods
Ceratiocaris papilio specimens UWGM 1902 and 1904 were carbon coated and analyzed using an AMRAY model 1600 scanning electron microscope equipped with EDAX brand electron dispersive spectroscopy (EDX). A thin section of the matrix surrounding UWGM 1902 was also prepared and analyzed using a petrographic microscope. Dark brown inner cuticle, blue-gray outer cuticle, and surrounding matrix were analyzed and elemental maps were produced using EDX. Images were captured using EDAX elemental-mapping software. Specimens were also evaluated in hand sample and using a binocular reflected-light microscope.
Systematic paleontology
Repository information
UWGM, University of Wisconsin at Madison Geology Museum, Madison, Wisconsin; ROM, Royal Ontario Museum, Toronto, Ontario; GSC, Geological Survey of Canada, Ottawa, Ontario.
Class Malacostraca Latreille, 1802
Subclass Phyllocarida Packard, Reference Packard1879
Order Archaeostraca Claus, Reference Claus1888
Suborder Ceratiocaridina Clarke in Zittel, Reference Zittel1900
Family Ceratiocarididae Salter, Reference Salter1860
Genus Ceratiocaris M’Coy, Reference M’Coy1849
Type species
Ceratiocaris solenoides M’Coy, Reference M’Coy1849, by original designation.
Ceratiocaris macroura Collette and Rudkin, Reference Collette and Rudkin2010

Figure 1 Ceratiocaris macroura Collette and Rudkin, Reference Collette and Rudkin2010. (1, 2) UWGM 1923 part, counterpart, with explanatory line drawing, scale=10 mm; (3) close-up of anterior carapace and mandible, with explanatory line drawing, scale=3 mm; (4, 5) UWGM 1916 part, counterpart, scale=3 mm; (6) UWGM 1906, scale=10 mm; (7) UWGM 1906, close-up of anterior carapace and partial antenna; An=antenna, CF=furca, LCV=left carapace valve, RCV=right carapace valve, Te=telson, Th=thorax, Ro=rostrum, IP=incisor process, De=denticulation, ACM=anterior carapace margin.

Figure 2 Ceratiocaris macroura Collette and Rudkin, Reference Collette and Rudkin2010. (1, 2) UWGM 1907 part, counterpart, arrow indicates the same backfilled burrow as in 1.5, scale=10 mm; (3) close-up of carapace cuticle showing gradation from fine striae to nested scale-like pits, scale=1 mm; (4) close-up of rostrum showing coarse striae, scale=1 mm; (5) close-up of carapace cuticle showing gradation of ornamentation and crosscutting backfilled burrows, corresponding to 1.2, arrow indicates position of backfilled burrow; (6) close-up of carapace cuticle showing detail of nested scale-like pits, corresponding to 1.2; (7) close-up of eye and ocular peduncle, corresponding to 1.1, scale=1 mm; (8) close-up of exceptionally preserved portion of eye, ommatidial lenses indicated by arrows, scale=1 mm.
2001 Ceratiocaris sp. Tetrault, p. 136, fig. 44.
2010 Ceratiocaris macroura Collette and Rudkin, p. 119, fig. 1.1–1.6.
2010 Ceratiocaris macroura; Collette and Hagadorn, p. 804, fig. 3.
Type specimens
Holotype ROM 49551, paratype ROM 58940.
Diagnosis
Ceratiocaris species distinguished by exceptionally long, styliform telson. Two lateral furcal rami present; ratio of telson length to furca length approximately 4.0:1.0. Anastamosing ridges present on dorsal carapace surface, increasing in sinuosity ventrally and grading into nested scale-like pits. Length-to-height ratio of carapace is approximately 1.7:1.0. Abdominal somites ornamented with raised, sub-parallel longitudinal striae that occasionally anastomose.
Remarks
The diagnosis was emended after Collette and Hagadorn (Reference Collette and Hagadorn2010) based on new insight into the carapace cuticle ornamentation, discussed below.
Occurrence
Wenlock, upper Eramosa Formation, Ontario; late Llandovery to early Wenlock, Brandon Bridge Formation, Wisconsin.
Material
UWGM 1903, 1907, 1916, 1923.
Description
The part of specimen UWGM 1923 includes the right and left mandibles (Fig. 1.1, 1.3) and the part and counterpart of UWGM 1907 include an exceptionally preserved eye (Fig. 2.1, 2.2, 2.7, 2.8). A partial posteriorly directed antenna is preserved on UWGM 1906 (Fig. 1.6, 1.7). However, little in the way of other morphology can be discerned. Antennal morphology of C. macroura was described in detail by Jones et al. (Reference Jones, Feldmann and Mikulic2015).
The mandibles of UWGM 1923 occupy greater than half of the cephalic region (Fig. 1.1, 1.3). Both mandibles are preserved, the right in lateral aspect and the left roughly corresponding to a cross section with the occlusal surface visible. The right mandible articulates laterally with the cephalon, although the exact nature of the articulation could not be observed. The corpus mandibulae is roughly quadrangular in outline and the articular surface appears to be quite large. The palp foramen could not be identified. The gnathal lobe is roughly triangular, with pronounced anterior concavity and equally pronounced posterior convexity. The incisor process is long and distinctly acute. The molar process was either not preserved or was absent. Elongate, sinuous, and apparently quite sharp denticles are present on the incisor process, and the long axis is oriented with the long axis of the gnathal lobe.
A pedunculate eye is preserved on the part and counterpart of UWGM 1907. The ocular peduncle consists of at least two segments, and appears to have been situated in a recessed notch in the carapace positioned just ventral to the rostrum (Fig. 2.1, 2.2, 2. 7, 2.8). In UWGM 1907, the eye has been bent toward the venter and is not situated in the notch. Before biostratinomic alteration, the eye is postulated to have protruded anteriorly from underneath the carapace. Articulating with the ocular peduncle is a large, round compound eye. Individual ommatidia are preserved on a small portion of the eye of the part (Fig. 2.7, 2.8). Lenses appear to be rounded to slightly polygonal, and are minute and closely spaced. Due to preservation, ommatidial angles could not be measured. However, the small size and close ommatidial spacing suggests that the angle was small.
Thoracic somites are preserved in UWGM 1913 and UWGM 1923 (Fig. 1.1, 1.2). The carapace and rostrum are preserved in UWGM 1907, 1913, and 1923. Both carapace morphologies, the rounded type in large specimens and the quadrangular type in small specimens, as described from the Eramosa Formation (Collette and Rudkin, Reference Collette and Rudkin2010), are present in the Waukesha Biota. Waukesha specimens are generally consistent in carapace morphology with those described by Collette and Rudkin (Reference Collette and Rudkin2010), except that the carapace cuticle of Waukesha specimens is much better preserved than that of described Eramosa Formation specimens.
In previously figured specimens of C. macroura, the rostral ornamentation consisted of fine, evenly spaced striae paralleling the margin of the rostral plate (Collette and Rudkin, Reference Collette and Rudkin2010). In UWGM 1907, the rostral ornamentation is exceptionally well preserved and comprises evenly spaced striae paralleling the rostral plate margin; however, the striae are substantially more robust than those previously figured (Collette and Rudkin, Reference Collette and Rudkin2010) (Fig. 2.4). The striae become finer medially and posteriorly and eventually disappear (Fig. 2.4).
The carapace ornamentation of UWGM 1907 is well preserved (Fig. 2.3, 2.5, 2.6). Previously described carapace ornamentation consisted of raised, slightly irregularly spaced anastomosing ridges oriented nearly parallel to the ventral margin of the carapace (Collette and Rudkin, Reference Collette and Rudkin2010). A large specimen from the Eramosa Formation also exhibited anastomosing ridges produced into raised scales adjacent to the anterior, ventral, and posterior ridges of the carapace margin (Collette and Rudkin, Reference Collette and Rudkin2010).
The dorsal carapace ornamentation consists of evenly spaced, anastomosing ridges (Fig. 2.3, 2.5). Toward the venter, the ridges become more robust, exhibit more relief, and increase in sinuosity, grading into a distinctly anastomosing morphology in which the ridges begin to intersect and form scale-like pits (Fig. 2.3, 2.5, 2.6). Ventrally, this morphology grades into a series of interlocking, imbricated scale-like pits, generally becoming rounder with greater relief (Fig. 2.3, 2.6). The pits are variable in size, with smaller, rounder pits occupying spaces between larger, more axially elongated pits. The pits exhibit a fractal pattern, the larger pits containing ever-smaller pits of similar shape and arrangement. Scale-like pits are better preserved on the part of UWGM 1907, which is almost completely covered with blue-gray, phosphatized outer cuticle, suggesting that this ornamentation pattern is more consistent with the outer ?exocuticle than the inner ?endocuticle.
All eight thoracomeres are preserved on UWGM 1923. The thoracomeres are axially shortened compared to the pleomeres, decrease in dorsoventral depth posteriorly from thoracomere I, then increase in dorsoventral depth posteriorly from thoracomeres VI–VIII moving toward the pleon. No thoracopods are preserved in Waukesha C. macroura specimens.
Pleonal features in Waukesha C. macroura specimens are consistent with those previously described (Collette and Rudkin, Reference Collette and Rudkin2010). Partial pleopodal bases are preserved on pleomeres III and IV of the part of UWGM 1923 (Fig. 1.1).
The telson and furcae are preserved on UWGM 1923 and UWGM 1916 (Fig. 1.1, 1.2, 1.4, 1.5). Consistent with specimens described by Collette and Rudkin (Reference Collette and Rudkin2010), the telson/furca ratio of UWGM 1916, the only Waukesha C. macroura specimen with a nearly complete telson, is approximately 4.0:1.0. The telson of UWGM 1923, a nearly complete specimen exhibiting the quadrangular carapace morphotype, is much shorter than that of ROM 58940 (Collette and Rudkin, Reference Collette and Rudkin2010). The telson of UWGM 1923 appears to be incomplete (Fig. 1.1, 1.2).
Remarks
Of the C. macroura specimens examined, UWGM 1923 part and counterpart is nearly complete, including the carapace, cephalic, thoracic, and pleonal regions, as well as the telson and furcae. UWGM 1903 and UWGM 1907 include the carapace without the pleon, telson, or furcae. UWGM 1916 includes pleomeres IV—VII with the telson and furcae. None of the specimens examined appear to be in Salter’s position, a condition characteristic of crustacean exuviae, characterized by partial separation of the carapace and pleon, dorsal flexure of the carapace, and anteroventral flexure of the pleon (Glaessner, Reference Glaessner1969; Feldmann and Tshudy, Reference Feldmann and Tshudy1987).
Differentiating corpses and molts is based largely on comparisons with exuviae of other crustacean groups, and some degree of conjecture. Despite this, it seems unlikely that complete specimens, including the carapace, thoracic somites, and pleon, without evidence of dorsal carapace flexure or anteroventral pleonal flexure represent molts. Specimens including just the carapace, or just the pleon or posterior pleonites may represent molts. As such, at least one Waukesha C. macroura specimen (UWGM 1923) appears to represent a corpse. UWGM 1903, 1907, and 1916 might represent molts.
In arthropod compound eyes, a trade-off in visual acuity exists between sensitivity and resolution (Snyder, Reference Snyder1977; Land and Nilsson, Reference Land and Nilsson2006). This trade-off is essentially a function of the ommatidial acceptance angle, a smaller angle allowing for higher resolution but decreased sensitivity and a higher angle allowing for greater sensitivity but lower resolution (Snyder, Reference Snyder1977; Land and Nilsson, Reference Land and Nilsson2006). As such, organisms living well within the photic zone are able to benefit from the higher resolution afforded by a smaller ommatidial acceptance angle, whereas arthropods inhabiting deeper-water environments require a greater ommatidial acceptance angle, and, as a result see in lower resolution. The interpreted low ommatidial acceptance angle of the eyes of C. macroura suggests that it was capable of seeing in relatively high resolution, but that its eyes would not have been sensitive enough to see in deep-water, low-light environments.
Previously proposed generalized feeding strategies for Ceratiocaris species, included deposit feeding using the thoracopods to lift sediment into suspension, or carrion feeding (Rolfe and Beckett, Reference Rolfe and Beckett1984). It has been noted that the molar process in archaeostracan phyllocarids is often undeveloped, as in carnivorous euphausiaceans (Dzik, Reference Dzik1980; Rolfe and Beckett, Reference Rolfe and Beckett1984). This suggests that some archaeostracans may have been rapacious.
Well-preserved mandibles are known from Ceratiocaris papilio Salter in Murchison, Reference Salter1859 and C. monroei (Whitfield, Reference Whitfield1896) (Woodward, Reference Woodward1865; Rolfe, Reference Rolfe1962), as well as from the rhinocarid genera Dithyriocaris and cf. Hebertocaris (Woodward, Reference Woodward1865; Stumm and Chilman, Reference Stumm and Chilman1969). The mandibles of C. papilio, Dithyriocaris spp., and cf. Hebertocaris spp. exhibit a relatively enlarged gnathal lobe and transversely oriented denticles, and in Dithyriocaris spp. the denticles exhibit c-shaped cusps (Woodward, Reference Woodward1865; Rolfe, Reference Rolfe1962; Stumm and Chilman, Reference Stumm and Chilman1969). This denticle morphology is grossly similar to that of nebaliid leptostracans (e.g., Martin et al., Reference Martin, Vetter and Cash-Clark1996), which are generally detritivores (e.g., Vannier et al., Reference Vannier, Boissy and Racheboeuf1997), although the denticulation in nebaliids is present on the molar process, rather than the gnathal lobe. This suggests that gnathal lobe denticulation in Dithyriocaris and cf. Hebertocaris was convergent on the molar process denticulation of nebaliids, possibly due to similar feeding strategies.
Denticles of the gnathal edge of the mandible in the rapacious anostracan branchiopod Branchinecta raptor Rogers, Quinney, Weaver, and Olesen, Reference Rogers, Quinney, Weaver and Olesen2006 bear some similarity to those of C. macroura in being longitudinally oriented and apparently quite blade-like (Rogers et al., Reference Rogers, Quinney, Weaver and Olesen2006). The incisor process of C. macroura also bears similarity to that of B. raptor and the rapacious decapod Procaris ascensionis Chace and Manning, Reference Chace and Manning1972, in being relatively pronounced, sharp, and blade-like (Chace and Manning, Reference Chace and Manning1972; Rogers et al., Reference Rogers, Quinney, Weaver and Olesen2006). The morphology and remarkable size of the mandible of C. macroura suggests that it may have been a predatory species.
Although thoracopods of C. macroura are unknown, absence of obvious raptorial thoracopods (e.g., Rolfe and Beckett, Reference Rolfe and Beckett1984) should not be used as evidence to argue against a predatory lifestyle in any fossil group. For example, Procaris ascensionis uses its pereiopods, which would not likely be interpreted as raptorial appendages in a fossil of that species, to grasp prey and the third maxilliped, unlikely to be well-preserved in a fossil, to hold prey against the gnathothorax (Abele and Felgenhauer, Reference Abele and Felgenhauer1985).
The presence of both C. macroura morphotypes, sensu Collette and Rudkin (Reference Collette and Rudkin2010), suggested to represent ontogenetic stages (Collette and Rudkin, Reference Collette and Rudkin2010) in the Waukesha Biota, supports the idea that the two morphotypes represent the same species. It must be considered that the variation may represent sexual dimorphism, rather than ontogenetic variation. In Nebalia spp. (e.g., N. hessleri), sexual dimorphism is reflected in both carapace morphology and antennal morphology, males tending to possess long, multiarticulate, anteriorly directed antennal flagella and much shallower lateral carapace margins than females (e.g., Martin et al., Reference Martin, Vetter and Cash-Clark1996). As such, variation in carapace morphology of C. macroura might represent sexual dimorphism, rather than ontogenetic variation.
Ceratiocaris papilio Salter in Murchison, Reference Salter1859

Figure 3 Ceratiocaris papilio Salter in Murchison, Reference Salter1859. (1) UWGM 1926, with explanatory line drawing, scale=1 mm; (2) UWGM 741 with explanatory line drawing, counterpart UWGM 1905 not figured, scale=10 mm; (3, 4) UWGM 1917, scale=1 mm, MaP=mandibular palp, Pp=pleopod, ThP=thoracopod.

Figure 4 Ceratiocaris papilio Salter in Murchison, Reference Salter1859. (1) UWGM 741, close-up of pleonite VI showing ornamentation, scale=1 mm; (2) UWGM 1910, counterpart not figured, scale=10 mm; (3) UWGM 1926, close-up of ornamentation on pleonite VII, scale=1 mm; (4) UWGM 1924, scale=2 mm; (5) UWGM, 1925, counterpart, UWGM 1922 not figured, showing dorsal striae, scale=2 mm.
1859 Ceratiocaris papilio Salter in Murchison, p. 252, fig. 1.
1860 Ceratiocaris papilio; Salter, p. 155, fig. 1.
1865 Ceratiocaris papilio; Woodward, p. 401, pl. 11, figs. 1–2.
1962 Ceratiocaris papilio; Rolfe, p. 912, pl. 129, figs. 1, 3–10.
2010 Ceratiocaris papilio; Budil et al., p. 551, figs. 4–9.
2010 Ceratiocaris papilio; Collette and Hagadorn, p. 804, fig. 3.
2010 Ceratiocaris papilio; Collette and Rudkin, p. 121, figs. 2–3.
Neotype
BGS GSM-7479, designated by Rolfe and Burnaby (Reference Rolfe and Burnaby1961).
Diagnosis
See Collette and Hagadorn (Reference Collette and Hagadorn2010).
Occurrence
Wenlock, Lanarkshire, Scotland; Wenlock, upper Eramosa Formation, Ontario; lower Ludfordian, Kopanina Formation, Všeradice, Czech Republic; late Llandovery to early Wenlock, Brandon Bridge Formation, Wisconsin.
Material
UWGM 741/1905, 1902, 1904, 1908, 1910, 1914, 1917, 1919, 1920, 1921, 1922, 1925, 1926, 1930.
Description
The cephalon is preserved in most specimens (Fig. 3.1, 3.2, 3.3, 3.4). The mandible is present on UWGM 1917 and 1926 (Fig. 3.1, 3.3, 3.4). A structure reminiscent of the mandibular palp is preserved in both specimens.
The thoracic region and carapace are preserved on nearly all specimens (Fig. 3.1–3.6). The rostral plate was readily identified on UWGM 741/1905 and 1926 (Fig. 3.1, 3.2). Rostral plate ornamentation was not as well preserved as on previously described specimens (Rolfe, Reference Rolfe1962). However, rostral plate morphology of Waukesha C. papilio specimens is consistent with that of specimens described from Scotland (Rolfe, Reference Rolfe1962). Waukesha C. papilio specimens lack evidence of the dorsal hinge nodes described from Scottish specimens (Rolfe Reference Rolfe1962; Collette and Rudkin, Reference Collette and Rudkin2010; Collette and Hagadorn, Reference Collette and Hagadorn2010).
The “optic node” was observed on UWGM 741/1905 (Fig. 3.1) and, consistent with previous observations, appears to correspond to the position of the mandible (Salter, Reference Salter1860; Rolfe, Reference Rolfe1962; Collette and Hagadorn, Reference Collette and Hagadorn2010; Collette and Rudkin, Reference Collette and Rudkin2010). Consistent with previous assertions, Waukesha C. papilio specimens exhibit thin carapace cuticle, and the optic node appears to represent compression of the cuticle around the mandible (Rolfe, Reference Rolfe1962; Budil et al., Reference Budil, Collette and Manda2010; Collette and Rudkin, Reference Collette and Rudkin2010). Carapace ornamentation on Waukesha C. papilio specimens consists of faint, parallel to subparallel striae.
Thoracomeres were identified on three specimens (Fig. 3.1, 3.2, 3.2). Thoracomeres are relatively well preserved, and exhibit the same aforementioned pattern of dorsoventral expansion and reduction as C. macroura. Partial thoracopods are preserved in all specimens in which thoracomeres are identifiable.
Well-preserved pleomeres occur in many specimens (Fig. 3.1–3.4). Pleonal cuticle ornamentation in C. papilio comprises elevated trigonal, or chevron-shaped scales and a finer pattern of subparallel striae that are deflected moving posteroventrally toward the long axis of the pleon (Jones and Woodward, Reference Jones and Woodward1888; Rolfe, Reference Rolfe1962; Collette and Rudkin, Reference Collette and Rudkin2010). A finer trigonal scale pattern is superimposed over the coarse trigonal scale ornamentation (Collette and Rudkin, Reference Collette and Rudkin2010). All of these pleonal ornamentation features were observed in Waukesha C. papilio specimens (Fig. 4.1, 4.3). The pattern of fine striae that has been previously noted (Jones and Woodward, Reference Jones and Woodward1888; Rolfe, Reference Rolfe1962) appears to result from the arrangement of the coarser trigonal scales, the scales being offset in such a way that fine striae punctuate the coarser ornamentation pattern and are deflected until they are subparallel to the long axis of the body along the ventral margin (Fig. 4.3).
Pleopods are preserved on UWGM 741/1905, 1917, 1921, and 1926. In Waukesha specimens, only the bases of pleopods I–IV are preserved (Fig. 3.1, 3.2, 3.3, 3.4). Pleopods of C. papilio are composed of a long, robust basis, from which two relatively slender rami, the endopod and exopod, emanate (Rolfe, Reference Rolfe1962). This is grossly similar to pleopod morphology in leptostracan phyllocarids, which retain relatively robust pleopods in pleomeres I–VI, and rather similar to pleopods of most eumalacostracans, which generally retain pleopods I–V. Endopodal and exopodal rami are not preserved in any Waukesha C. papilio specimens.
The telson and furcae are present in UWGM 741/1905, 1902, 1917, 1922, 1924, 1925, and 1926, and are generally present in unbroken Waukesha C. papilio specimens. UWGM 1924, 1925, and 1926 represent isolated occurrences of the telson and furcae that occur on unbroken rock slabs, indicating that separation of the telson from the pleon was either biostratinomic, or before death of the organism, perhaps during ecdysis (Fig. 4.4, 4.5). Attribution of UWGM 1924, 1925, and 1926 to C. papilio, rather than C. macroura is based on the telson/furca length ratio of approximately 1.9:1.0, which is more consistent with that of C. papilio than that of C. macroura, which is approximately 4.0:1.0 (Collette and Rudkin, Reference Collette and Rudkin2010; Collette and Hagadorn, Reference Collette and Hagadorn2010). Both species exhibit longitudinally striated furcae and a telson with lateral spine rows (Fig. 4.4, 4.5).
Relatively complete Waukesha C. papilio specimens preserving the telson and furcae tend to preserve little in the way of fine detail in the caudal region, with the exception of faintly preserved, minute lateral spines. Isolated occurrences of the telson and furcae, in contrast, preserved much surficial morphology. The presence of striae on the dorsal and ventral surfaces of furcae of C. papilio individuals has been noted (Rolfe, Reference Rolfe1962; Collette and Rudkin, Reference Collette and Rudkin2010).
Waukesha specimens of isolated telsons and furcae, in contrast to previous assertions, bear robust, widely spaced striae on the dorsal surface, and only a single medial striation on the ventral surface, which appears to have been impressed through the venter from the dorsum (Fig. 4.4, 4.5). Differences in telson and furca striations in Waukesha specimens may be taphonomic, as was proposed for Eramosa and Scottish specimens (Collette and Hagadorn, Reference Collette and Hagadorn2010). More evidence is needed to form a well-reasoned hypothesis. Worthy of note is that the isolated telson and furca specimens, UWGM 1922 and 1925 (part and counterpart) are 21 mm long and UWGM 1924 is 19 mm long, whereas the two nearly complete specimens, UWGM 741 and 1905 (part and counterpart), and UWGM 1926 are 37 and 38 mm in total length, respectively. This will be discussed in more detail below.
Remarks
Of the C. papilio specimens examined, UWGM 1926 and UWGM 741/1905 (part and counterpart) represent nearly complete specimens, UWGM 1926 including the entire length from the anteriormost rostrum to posteriormost telson, and UWGM 741/1905 missing the posteriormost portion of the telson. UWGM 1922 and 1925 (part and counterpart) represent isolated occurrences of the telson and furcae. Other specimens include the posterior pleomeres, telson, and furcae, or carapace including pleomeres I–IV. These include specimens in which the carapace region and posterior pleomeres were broken from the specimens, and those in which separation appears to have been biostratinomic.
The paucity of complete specimens precluded detailed size range comparison of C. papilio specimens; however, two generalizations can be made. Ceratiocaris papilio specimens from Waukesha appear to have been much smaller than examples from Scotland, projected to reach up to 600 mm total length (Rolfe, Reference Rolfe1962), and Ontario, ranging from 46 to 88 mm in length (Collette and Rudkin, Reference Collette and Rudkin2010). Although relatively large individuals are represented in Waukesha and Eramosa by isolated pleons and furcae (Collette and Rudkin, Reference Collette and Rudkin2010), complete specimens of large individuals are absent. What this size disparity in complete and incomplete individuals represents is unclear. If the incomplete specimens represent exuviae, it suggests that large individuals may have been transient inhabitants of the environmental settings represented by those strata.
UWGM 741/1905, 1904, and 1926, being complete specimens with evidence of neither dorsal carapace flexure nor ventral pleonal flexure, most likely represent corpses. Fragmentary specimens, such as those including only the posterior pleomeres and furcae, or telson and furcae, are more likely to represent molts. Ceratiocaris papilio specimens figured from Scotland (Salter, Reference Salter1860) exhibited pronounced ventral pleonal flexure and relative dorsal carapace flexure resulting in a curious misinterpretation of the relative position of the carapace and pleon (Salter, Reference Salter1860). These specimens are likely to have been in Salter’s position and probably represent exuviae.
The absence of dorsal hinge nodes from Waukesha C. papilio specimens is not problematic from a diagnostic perspective, as specimens from Waukesha exhibit all other diagnostic criteria of C. papilio. Dorsal hinge nodes have only been documented on the right carapace valve, with corresponding “shallow embayments to receive the right valve process” on the left valve (Rolfe, Reference Rolfe1962, p. 917). Dorsal hinge nodes have been interpreted either to represent a clasping mechanism to stabilize the valves (Rolfe, Reference Rolfe1962), or to be sensory organs (Crasquin et al., Reference Crasquin, Racheboeuf and Bergström2009).
Ceratiocaris pusilla Matthew, Reference Matthew1889

Figure 5 Ceratiocaris pusilla Matthew, Reference Matthew1889. (1, 2) UWGM 1909, part, counterpart, Pp=pleopod, scale=10 mm; (3, 4) Close-up of caudal region showing details of furca and telson, CF=furca, Te=telson, scale=10 mm; (5, 6) UWGM 1913, scale=2 mm.
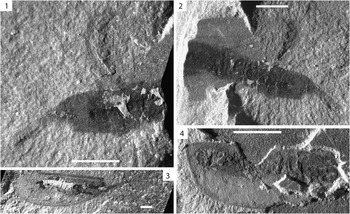
Figure 6 Ceratiocaris pusilla Matthew, Reference Matthew1889. (1) UWGM 1912 showing evidence of Salter’s position, scale=3 mm; (2) UWGM 1918 showing evidence of Salter’s position, scale=3 mm; (3) UWGM 1929, scale=3 mm; (4) UWGM 1911 showing ventrally reflexed rostrum, scale=5 mm.
1889 Ceratiocaris pusillus Matthew, p. 56, pl. 4, fig. 9.
1889 Ceratiocaris pusillus; Miller, p. 537.
1890 Ceratiocaris pusilla; Jones, p. 64.
1893 Ceratiocaris pusillus; Vodges, p. 378.
1898a Rhinocaris pusilla; Matthew in Jones, p. 344.
1898b Rhinocaris pusilla; Matthew in Jones, p. 43.
1900 Rhinocaris pusilla; Matthew in Jones, p. 404.
1915 Ceratiocaris pusilla; Bassler, p. 1390.
1934 Ceratiocaris pusilla; Van Straelen and Schmitz, p. 63.
1957 Ceratiocaris pusilla; Copeland, p. 600, pl. 67, fig. 1.
2010 Ceratiocaris pusilla; Collette and Hagadorn, p. 806, figs. 3, 5.3–5.4.
Type specimens
Holotype ROM 2701; hypotypes, GSC 13298 and 13298a.
Diagnosis
See Collette and Hagadorn (Reference Collette and Hagadorn2010).
Occurrence
Llandovery, upper Jones Creek Formation, New Brunswick; late Llandovery to early Wenlock, Brandon Bridge Formation, Wisconsin.
Material
UWGM 1911, 1912, 1913, 1918, 1928, 1929; ROM 22701; GSC 13298.
Description
The cephalic region is present in UWGM 1913, and 1929 (Figs. 5.5, 5.6, 6.3). In UWGM 1913, a structure corresponding to the probable position of the mandible is present. No other cephalic appendages were preserved.
The carapace is preserved in all Waukesha C. pusilla specimens and generally contains preserved thoracomeres (Figs. 5.1, 5.2, 5.5, 5.6, 6. 3, 6.4). Thoracopods are present in UWGM 1909, part and counterpart, but are preserved as ghosted outlines and could not be evaluated in detail (Fig. 5.1, 5.2).
General carapace form and ornamentation were described by Copeland (Reference Copeland1957, p. 601), who reconstructed the carapace as “horseshoe-shaped in dorsal view” and “elliptical to oblong in lateral view”. A high-resolution photograph of ROM 22701 and the part of GSC 13298 were examined for comparative purposes. This dorsal reconstruction was apparently based on a dorsally preserved specimen on GSC 13298, on which both carapace valves are preserved, articulating with an obviously discrete rostral plate. The holotype and other hypotypes are laterally preserved and exhibit the more characteristic “elliptical to oblong” shape (Copeland, Reference Copeland1957, p. 601).
Waukesha C. pusilla specimens are all laterally preserved and consistent with this carapace shape. Carapace ornamentation could not be discerned on most Waukesha C. pusilla specimens; however, portions of exceptionally preserved carapace cuticle are present on UWGM 1909. This ornamentation differs from the faint longitudinal striae in the diagnosis of Collette and Hagadorn (Reference Collette and Hagadorn2010) in being composed of coarse, widely spaced longitudinal striae that give well-preserved carapace cuticle the appearance of being divided into narrow quadrangular sections (Fig. 5.1, 5.2). The striae tend to be quite long, although the full length is generally not preserved. Carapace striae appear to thin and pinch out against adjacent striae at irregular intervals.
That the rostrum is slender, acutely acuminate in lateral aspect, and apparently quite delicate has not generally been mentioned. In Waukesha specimens, UWGM 1911 and 1913, the rostrum is ventrally reflexed, apparently due to biostratinomic alteration (Figs. 5.5, 5.6, 6.4).
Pleon
Individual pleomeres were discerned in UWGM 1909, 1912, 1913, 1918, and 1929. They appear to be generally dorsoventrally compressed, consistent with the morphology of the carapace. Only pleomere VII protrudes from the carapace in C. pusilla (Collette and Hagadorn, Reference Collette and Hagadorn2010).
Bases of pleopods I–IV are present on UWGM 1909 (Fig. 5.1, 5.2). The pleopodal bases are elliptical in outline laterally, relatively long, and decrease in length posteriorly (pleopod 1 length=3.2 mm, width=1.7 mm; pleopod 2 length=3.53 mm, width=1.5 mm; pleopod 3 length=2.7 mm, width=1.5 mm; pleopod 4 length=2.14 mm, width=1.5 mm). This pattern of decreasing pleopod length posteriorly is generally consistent with the pattern observed in other Ceratiocaris species (e.g., Rolfe, Reference Rolfe1962), as well as in leptostracan phyllocarids.
Interpretation of the telson and furca morphology of C. pusilla has historically been problematic (see Mathew, Reference Matthew1889; Copeland, Reference Copeland1957; Collette and Hagadorn, Reference Collette and Hagadorn2010). The reconstruction figured by Matthew (Reference Matthew1889) includes a slender, styliform telson and furcae, with the telson approximately 30 percent longer than the furcal rami. This is not morphologically consistent with the holotype, or with the hypotypes used in the reconstruction by Copeland (Reference Copeland1957). Copeland (Reference Copeland1957) reconstructed the telson as being slightly longer than the furcae, but with a relatively robust double spine row on both the telson and furcal rami.
Examination of the hypotype yielded neither evidence of robust double spine rows on the telson, nor exceptionally long furcal rami. One specimen on GSC 13298, which contains multiple specimens, is preserved with the telson in close proximity to what appears to be an isolated telson of another specimen. There is no evidence that the putative isolated telson articulates with the telson of the nearly complete specimen, nor is there evidence of preserved furcae on that specimen. The long furcal rami previously figured (Copeland, Reference Copeland1957) may have resulted from misinterpretation of isolated telsons superimposed over the telson of one or more hypotypes (Collette and Hagadorn, Reference Collette and Hagadorn2010).
UWGM 1909 includes well-preserved furcae, which appear to terminate naturally and clearly articulate with the telson head (Fig. 5.1, 5.2, 5.3, 5.4). The furcae of UWGM 1909 are short, considerably less than 10 percent of the length of the telson, roughly triangular in lateral outline, and overall bluntly acuminate (Fig. 5.1, 5.2, 5.3, 5.4). Thus, the furcae of C. pusilla are inconsistent with those previously figured (Matthew, Reference Matthew1889; Copeland, Reference Copeland1957), but are instead short and blunt. No evidence of robust double spine rows exists on telson or furcae of Waukesha specimens.
Remarks
Waukesha C. pusilla specimens are preserved as isolated carapaces or as relatively complete specimens. No Waukesha C. pusilla specimens are preserved as isolated pleomeres, or caudal elements. Ceratiocaris pusilla specimens, as a whole, tend to preserve less blue-gray exterior cuticle than other Ceratiocaris species represented in the Waukesha Biota. The reason for this is unclear, but it may be the result of thinner, more weakly sclerotized cuticle than in other species present.
Unlike other species present in the Waukesha Biota, C. pusilla specimens (UWGM 1909, 1912, and 1918) do exhibit evidence of preservation in Salter’s position. In the aforementioned specimens, there is evidence of dorsal carapace displacement and anteroventral pleon displacement, consistent with the post-molt condition in other malacostracan groups (e.g., Feldmann and Tshudy, Reference Feldmann and Tshudy1987). UWGM 1913 and UWGM 1929, in contrast, include the carapace and preserved posterior pleomeres, but no evidence of anteroventral pleon flexure or dorsal carapace flexure. Apparent preservation in Salter’s position is not unequivocal evidence of a specimen representing a molt, nor is preservation of intact specimens with no evidence of Salter’s position evidence that specimens represent corpses (Feldmann and Tshudy, Reference Feldmann and Tshudy1987). Preservation of specimens in Salter’s position suggests that they represent molts. Intact specimens, with no evidence for Salter’s position likely represent corpses; however, both of these lines of evidence are open to interpretation. UWGM 1911 and 1928, both of which are isolated carapaces, may represent molts as well.
Pechoracaris aculicauda Dzik, Ivantsov, and Deulin, Reference Dzik, Ivantsov and Deulin2004, is similar in form to C. pusilla in exhibiting a dorsoventrally shallow carapace that covers pleonites I–VI and exceptionally short furcae (Dzik et al., Reference Dzik, Ivantsov and Deulin2004). Pechoracaris aculicauda was attributed, based on scant morphological criteria, to Hoplostraca Schram, Reference Schram1973, a taxon erected to include Sairocaris Rolfe, Reference Rolfe1963, spp. and Kellibrooksia macrogaster Schram, Reference Schram1973. Pechoracaris aculicauda bears little resemblance to Sairocaris spp. and K. macrogaster, both of which exhibit an axially shortened carapace, often not covering the entire thorax, an enlarged pleon, and thoracopods that are not enclosed laterally by the carapace. Pechoracaris aculicauda should not be included in Hoplostraca. Based on specimens figured by Dzik et al. (Reference Dzik, Ivantsov and Deulin2004, fig. 1c), it appears that P. aculicauda may have a discrete rostral plate. If so, that species should be transferred to Ceratiocaris. Based on its gross similarity to C. pusilla, P. aculicauda may be a closely related form.
Taphonomy
Preservation
Phyllocarids from the Waukesha Biota are all preserved as essentially two-dimensional compression fossils, often including part and counterpart. Specimens vary in completeness. Many specimens include the cephalic, thoracic, and pleonal regions, as well as the telson and furcae. The nature of preservation is such that the thoracomeres, anterior pleomeres, thoracopods, and basalmost pleopods are observable through the carapace wall. Specimens are rarely preserved as isolated occurrences of the telson, furcae, and pleon without the thoracomeres and carapace, or partial thorax and anterior pleomeres without the carapace. In one C. papilio specimen, nearly the entire specimen is crushed and impacted into the carapace (Fig. 4.2).
Cuticle of Waukesha Biota phyllocarids and most soft-bodied arthropods from the biota is preserved in two manners, both of which are present on nearly all specimens. Most cuticle is preserved as a dark brown carbon film, exhibiting no relief (Fig. 7). The dark brown, carbonaceous material was apparently quite thin and pliant and is frequently compressed over the topography of bedding planes. Nearly all specimens also exhibit cuticle preserved as a blue-gray material present on isolated portions of specimens in the form of apparent flaky remnants (Fig. 7). The blue-gray material in all cases overlies the brown, deformable material and exhibits observable, submillimeter-scale relief. Where blue-gray cuticle is present on parts, it is absent from counterparts and vice versa, suggesting that both cuticle layers were once present on the entire or nearly entire specimen. In UWGM 1907, both the brown cuticle and blue-gray cuticle are crosscut by backfilled burrows, apparently produced by deposit feeding or scavenging organisms during decay of the specimen (Fig. 2.4, 2.5).

Figure 7 EDAX results for UWGM 1904. (1) Entire specimen showing brown and blue-gray cuticle, and area included in the EDAX results, scale=3 mm; (2) close-up of cuticle corresponding to EDAX results, with elemental maps.
Crosscutting both the brown inner cuticle and blue-gray outer cuticle on a small percentage of specimens are aggregates of minute, interlocking pyrite crystals. The smallest grains could not be observed in detail, because specimens bearing pyrite could not be analyzed in the scanning electron microscope. However, individual crystals appear to be subhedral to euhedral pyritohedra and cubic crystals. Rarely larger (sub-millimeter-scale) euhedral cubic crystals could be observed crosscutting the cuticle. Dense accumulations of minute pyrite crystals were also present in the burrows crosscutting C. macroura specimen UWGM 1907. In that specimen, neither the burrows nor the pyrite crosscut the blue-gray outer cuticle, but instead appear to be present beneath the blue-gray cuticle, which has been compressed over both the pyrite and the burrows. In specimens containing pyrite, pyrite crystals are also present on bedding planes surrounding the specimens, but in lower concentrations than those associated with the specimens.
Composition
The matrix surrounding the specimens was found to be enriched in Al, K, Si, Mg, Na, and Fe, consistent with the composition of common clay minerals and quartzose silt grains (Fig. 7). Consistent with this, the matrix in thin section appeared to be composed dominantly of clay with a minor component of quartzose silt grains. The brown inner cuticle material did not differ noticeably in elemental composition from the surrounding matrix (Fig. 7). As such, this material was interpreted to be a carbonaceous film—the electron dispersive spectroscopy method used can only detect elements heavier than carbon. Elemental maps revealed that the blue-gray outer cuticle was enriched in both P and Ca, but depleted in Al, K, Si, Mg, Na, and Fe (Fig. 7). As such, the blue-gray cuticle is interpreted to be composed of a calcium phosphate mineral, probably apatite.
Discussion
Evidence of Salter’s position in only UWGM 1909, 1912, and 1918 suggests that those specimens represent molts. Incomplete knowledge of archaeostracan ecdysial sutures complicates this interpretation. Specimens including only the carapace, pleomeres, or telson and furcal rami may also represent molts. The common occurrence of nearly complete phyllocarid specimens with evidence of neither ventral pleonal flexure nor dorsal carapace flexure in the Waukesha Biota suggests that not all phyllocarid specimens represent molts. It has been asserted that the Waukesha Biota is dominated by arthropod exuviae and likely represents “a hydrodynamically controlled taphonomic accumulation within a localized sediment trap” (Kluessendorf, Reference Kluessendorf1994, p. 343). This may be the case given the paucity of normal-marine fauna associated with the preserved soft-bodied organisms in the Waukesha Biota, as well as the preservation of the biota near the base of a paleo-erosional surface (Mikulic et al., Reference Mikulic, Briggs and Kluessendorf1985a, Reference Mikulic, Briggs and Kluessendorfb).
Given that a number of the Waukesha phyllocarids appear to represent corpses, it must be considered that these might represent true faunal elements of the biota. In evaluating whether the Waukesha phyllocarids represent all or part of a true biota, or a transported assemblage representing faunal elements of more than one paleoenvironment, occurrences of C. macroura, C. papilio, and C. pusilla were compiled (Table 1). Although only one previous occurrence of C. macroura has been documented, its occurrence in a relatively nearshore environment (Collette and Rudkin, Reference Collette and Rudkin2010) is consistent with previous occurrences of C. papilio, all of which have been from nearshore, or relatively nearshore environments (detailed paleoenvironmental information for C. papilio is surprisingly lacking given that the species has been studied for more than 150 years).
Table 1 Paleoenvironmental occurrences of Ceratiocaris macroura Collette and Rudkin, Reference Collette and Rudkin2010; Ceratiocaris papilio Salter in Murchison, Reference Salter1859; and Ceratiocaris pusilla Matthew, Reference Matthew1889.

In contrast to this, the only documented occurrence of C. pusilla was from more distal turbidite deposits of the Llandovery Jones Creek Formation (Matthew, Reference Matthew1889; Copeland, Reference Copeland1957). Occurrence of C. pusilla in turbidite deposits may indicate that it was a pelagic species, but is more likely to have resulted from basinward transport of nearshore faunules. Co-occurrence of Ceratiocaris species, apparently representing nearshore faunules, with C. pusilla, previously only recognized from marine trough turbidites (Copeland, Reference Copeland1977), could mean that the Waukesha phyllocarid assemblage represents a transported assemblage, rather than all or part of a biota, or that the occurrence of C. pusilla in the Jones Creek Formation resulted from basinward transport of nearshore faunules.
Waukesha Biota arthropods were previously reported as exhibiting soft-bodied preservation with infillings of diagenetic fluorapatite (Mikulic et al., Reference Mikulic, Briggs and Kluessendorf1985a). The findings presented here are not consistent with fossils being infilled with apatite, but rather exhibiting replacement of the outermost cuticle of phyllocarid specimens with apatite. Specimens exhibit little relief and generally include little evidence of infilling so much as compression over the topography of the bedding plane on which they are preserved. Furthermore, the presence of apatite on only the outermost cuticle layer suggests that replacement occurred on the exterior of specimens, the interior being shielded from remineralization and, as a result, being preserved as a carbonaceous film.
Phosphate mineralization on shrimp has been documented in decay experiments (Briggs and Kear, Reference Briggs and Kear1993; Hof and Briggs, Reference Hof and Briggs1997). Reported phosphate mineralization has generally been localized and largely restricted to soft tissues, such as muscle, and in the case of mantis shrimp, the hepatopancreas (Briggs and Kear, Reference Briggs and Kear1993; Hof and Briggs, Reference Hof and Briggs1997), both of which are quite different from preservation of Waukesha phyllocarids. Allison (Reference Allison1988) proposed a model of phosphate mineralization in fossil preservation in which low rates of burial, and prolonged residence at the oxic/anoxic interface within the sediment column concentrates available phosphate, potentially resulting in larger-scale phosphate mineralization than has been observed in laboratory experiments. This suggests that large-scale mineralization of Waukesha phyllocarid cuticle may have occurred during early diagenesis.
Pyritization of fossils, including skeletal material and soft parts, is a well-known phenomenon (Canfield and Raiswell, Reference Canfield and Raiswell1991). Sedimentary pyrite forms by reaction of detrital iron-bearing minerals with H2S produced by microbial reduction of interstitial sulfate, using organic matter as both a reductant and energy source (Berner, Reference Berner1984; Canfield and Raiswell, Reference Canfield and Raiswell1991). This process occurs under anoxic conditions, which are produced by aerobically respiring organisms, generally near the sediment-water interface.
The crosscutting relationship of pyrite to calcium phosphate in Waukesha phyllocarid cuticle clearly demonstrates that pyrite formation occurred after phosphate mineralization. The consistent preservation of the outer cuticle as calcium phosphate and the inner cuticle as a carbonaceous film that was apparently easily deformable, as evidenced by its consistent compression over the topography of bedding planes, suggests that phosphatization occurred on the outermost cuticle of the specimens during early diagenesis, most likely after shallow burial and during decay. Decaying specimens apparently remained in the sediment as deformable carbon films, encased in calcium phosphate after potentially prolonged residence near the oxic/anoxic boundary, later to be buried below the oxic/anoxic boundary, where early diagenetic pyrite crosscut both the carbonized and phosphatized cuticle. High concentrations of minute, interlocking pyrite crystals within the burrows in UWGM 1907 may have resulted from localized anoxia produced by consumption of fecal infillings by aerobic microbes.
Conclusions
Three phyllocarid species, Ceratiocaris macroura, C. papilio, and C. pusilla, are present in the late Llandovery–early Wenlock Waukesha Biota. Specimens including only posterior pleomeres and caudal elements, those including only caudal elements, and those exhibiting evidence of Salter’s position may represent molts. Nearly complete specimens likely represent corpses. Whether the Waukesha phyllocarid assemblage represents a true biota or transported assemblage is difficult to determine given the available evidence.
A complex taphonomic history is evidenced by the presence of carbonized cuticle, phosphatized cuticle, and cross-cutting, minute pyrite crystals. The consistent presence of phosphatized cuticle overlying carbonized cuticle suggests that phosphate mineralization occurred on the outermost cuticle of the specimens, likely during prolonged burial near the oxic/anoxic boundary within the sediment column. The consistent crosscutting relationship of pyrite to both carbonized cuticle and phosphatized cuticle suggests that pyrite formation occurred after phosphate mineralization, likely after burial below the oxic/anoxic boundary. Pyrite aggregates in cross-cutting burrow infills may have formed due to localized anoxia caused by decay of fecal burrow infillings.
Acknowledgments
We would like to acknowledge E. Maguire, E. Sload, and K. Kornecki for assistance with EDX analysis, as well as two anonymous reviewers, whose comments greatly improved the manuscript.