Introduction
Lichens are complex systems involving symbiotic associations of at least two very different organisms, a heterotrophic fungus (mycobiont) and a photobiont, a unicellular to filamentous green algae (chlorobiont; Chapman & Margulis Reference Chapman and Margulis1998) and/or a cyanobacteria (cyanobiont). Lichens are found in almost all terrestrial habitats and geographical areas, demonstrating the successful nature of the symbiosis. Most mycobionts (>99%) are members of the Ascomycota, whereas lichen-forming Basidiomycota represent less than 0.9% of all lichenized fungi (Lücking et al. Reference Lücking, Forno, Moncada, Coca, Vargas-Mendoza, Aptroot, Arias, Besal, Bungartz and Cabrera-Amaya2017a). In recent years, the classification of lichen-forming Basidiomycota has been continuously and substantially modified based on molecular data. These modifications include the introduction of new taxa including orders, families, subfamilies, tribes and genera (Redhead et al. Reference Redhead, Moncalvo, Vilgalys and Lutzoni2002; Ertz et al. Reference Ertz, Lawrey, Sikaroodi, Gillevet, Fischer, Killmann and Sérusiaux2008; Lawrey et al. Reference Lawrey, Lücking, Sipman, Chaves, Redhead, Bungartz, Sikaroodi and Gillevet2009; Dal Forno et al. Reference Dal Forno, Lawrey, Sikaroodi, Bhattarai, Gillevet, Sulzbacher and Lücking2013; Hodkinson et al. Reference Hodkinson, Moncada and Lücking2014; Lücking et al. Reference Lücking, Forno, Moncada, Coca, Vargas-Mendoza, Aptroot, Arias, Besal, Bungartz and Cabrera-Amaya2017a).
Hygrophoraceae is one of 20 fungal families with the largest number of lichenized species (Lücking et al. Reference Lücking, Dal-Forno, Sikaroodi, Gillevet, Bungartz, Moncada, Yánez-Ayabaca, Chaves, Coca and Lawrey2014, Reference Lücking, Forno, Moncada, Coca, Vargas-Mendoza, Aptroot, Arias, Besal, Bungartz and Cabrera-Amaya2017a; Dal Forno et al. Reference Dal Forno, Lücking, Bungartz, Yánez-Ayabaca, Marcelli, Spielmann, Coca, Chaves, Aptroot and Sipman2016). This family includes at least 23 genera according to Lodge et al. (Reference Lodge, Padamsee, Matheny, Aime, Cantrell, Boertmann, Kovalenko, Vizzini, Dentinger and Kirk2014), six of which are lichenized, namely Acantholichen, Cora, Corella, Cyphellostereum, Dictyonema and Lichenomphalia. While the first five genera are mainly tropical to tropical montane, Lichenomphalia species have traditionally been considered to have an arctic-alpine distribution, with the exception of L. umbellifera which is also common in northern forests (Redhead & Kuyper Reference Redhead, Kuyper, Laursen, Ammirati and Redhead1987; Kranner & Lutzoni Reference Kranner, Lutzoni and Lerner1999; Redhead et al. Reference Redhead, Moncalvo, Vilgalys and Lutzoni2002; Lawrey et al. Reference Lawrey, Lücking, Sipman, Chaves, Redhead, Bungartz, Sikaroodi and Gillevet2009; Geml et al. Reference Geml, Kauff, Brochmann, Lutzoni, Laursen, Redhead and Taylor2012).
Lichenomphalia species have frequently been reported from central and northern Europe, but only occasionally from southern Europe (Nimis Reference Nimis1993; Suppan et al. Reference Suppan, Prügger and Mayrhofer2000). In the Iberian Peninsula, at least four Lichenomphalia species have been found (Barrasa & Rico Reference Barrasa and Rico2001): L. hudsoniana, L. meridionalis, L. velutina and L. umbellifera (previously known as Omphalina ericetorum). All have a thallus of hyphal globules (Botrydina-type) except L. hudsoniana, which has a squamulose vegetative thallus (Coriscium-type) (Redhead & Kuyper Reference Redhead, Kuyper, Laursen, Ammirati and Redhead1987). The four species present different ecogeographical adaptations to specific regions: L. meridionalis seems to be restricted to the Mediterranean region, inhabiting more or less humid sites on the supra-Mediterranean belt, whereas L. hudsoniana is known only from the montane belt of the Eurosiberian region and the other two species, L. umbellifera and L. velutina, are widespread.
Lichenomphalia meridionalis (Contu & La Rocca) P.-A. Moreau & Courtec. has been reported only in the Mediterranean region, in Sardinia (Italy) (Contu & La Rocca Reference Contu and La1999), where it was originally found, and Spain (Barrasa & Rico Reference Barrasa and Rico2001). The aim of the present study is to increase our knowledge of the poorly studied Mediterranean basidiolichens by answering two questions: 1) is L. meridionalis distinguishable from other closely related Lichenomphalia species on the basis of molecular data, and 2) are the chlorobionts of L. meridionalis different from those of other Lichenomphalia species? Microscopic observations and molecular markers were used to investigate symbiont composition in specimens collected at different altitudes in several localities on the Iberian Peninsula.
Materials and Methods
Taxon sampling and datasets
We analyzed L. meridionalis samples, including both vegetative thalli and basidiomata, collected in natural habitats from nine localities in Spain at altitudes ranging from 533 to 2200 m (Table 1). Specimens are preserved in the University of Alcalá Herbarium (AH).
Table 1. Samples of Lichenomphalia meridionalis with details of voucher information (AH = Alcalá Herbarium), origin in Spain (including altitude (m above sea level)) and collection dates.

Morphological examination of ‘in thallus’ and isolated lichen chlorobionts
We examined a total of fifteen specimens of L. meridionalis thalli. A macroscopic photograph of basidiomata was taken using an Olympus E-510 camera. Chlorobionts of L. meridionalis were isolated from a fresh lichen thallus (sample Lms12) according to Gasulla et al. (Reference Gasulla, Guéra and Barreno2010). The isolated chlorobionts were axenically cultured on small nylon square membranes in semi-solid Bold 3N medium (Bold & Parker Reference Bold and Parker1962). They were incubated in a growth chamber maintained at a continuous temperature of 15 °C, with light/dark cycles of 14/10 h. Micrographs were made with an Olympus BX50 microscope using bright field.
DNA isolation, amplification and sequencing, and phylogenetic and statistical analyses
Total DNA was isolated from basidiomata and thallus fragments following the protocol described by Reynolds & Williams (Reference Reynolds and Williams2004). Basidioma DNA was used to obtain the sequences of the fungal nuclear large ribosomal subunit gene (LSU) and the internal transcribed spacers of the rRNA operon (ITS), using specific primers (see Supplementary Material Table S1, available online). The DNA from thallus fragments was used to obtain algal sequences. The internal transcribed spacers of the rRNA operon (ITS), the chloroplast psbB gene encoding for the protein CP-47 of photosystem II, the 23S rDNA encoding the chloroplast large subunit rRNA and the nuclear gene encoding for the L10A protein of the 60S ribosome (RPL10A) were sequenced for algal cells using specific primers (Supplementary Material Table S1). Since thallus fragments were directly used for DNA extraction, we designed specific primers based on the sequence of Coccomyxa subellipsoidea (SAG 216-13) to avoid amplification of DNA from other inhabiting organisms (Supplementary Material Table S1). Amplification reactions were carried out by PCR performed in a 25 μl reaction volume containing a PuReTaqTM Ready-To-GoTM PCR bead (GE Healthcare, Buckinghamshire, UK). Cycling conditions for all PCRs were: one cycle at 95 °C for 5 min and 35 cycles of 95 °C for 30 s, 55 °C for 30 s and 72 °C for 1 min. These cycles were followed by a final extension at 72 °C for 10 min. Amplified products were electrophoresed in 1.0% agarose gel and stained with SYBR® Safe (Invitrogen, Eugene, OR, USA) for visualization of the bands. PCR products were purified directly using the QIAquick PCR Purification Kit (Qiagen, Valencia, CA, USA). Purified amplicons were sequenced using the BigDyeTM Terminator Cycle Sequence Ready Reaction Kit II (Applied Biosystems, Foster City, CA, USA), separated by automated multicapillary electrophoresis, and further analyzed on an ABI Prism 3730 Genetic Analyzer (Applied Biosystems). Each sample was sequenced in both directions with the same primers as those used for PCR. All the obtained sequences are available in GenBank with the Accession numbers indicated in Table S2 (Supplementary Material, available online).
The phylogenetic relationships of the L. meridionalis mycobiont within that genus were inferred on the basis of the alignment of 15 sequences of the fungal LSU rDNA. Sequences from Arrhenia epichysium and A. lobata were used as out-group. The identity of the chlorobiont of L. meridionalis was determined from the sequences of the four molecular markers: the chloroplast psbB and 23S rDNA, and the nuclear ITS and RPL10A. The algal sequences were arranged into multiple sequence alignments (MSA) using MAFFT version 7 (Katoh & Standley Reference Katoh and Standley2013) on the Guidance web server, available at http://guidance.tau.ac.il (Penn et al. Reference Penn, Privman, Landan, Graur and Pupko2010a, Reference Penn, Privman, Ashkenazy, Landan, Graur and Pupkob). The GUIDANCE2 algorithm was used to create a SuperMSA by concatenating the default MSA and alternative 20 MSAs. All datasets were subjected to maximum likelihood (ML) with PhyML (Guindon et al. Reference Guindon, Dufayard, Lefort, Anisimova, Hordijk and Gascuel2010) using the general time-reversible model (GTR) as a substitution model (Tavaré Reference Tavaré and Miura1986), selected as the best model with ‘Smart Model Selection’ (SMS) (Lefort et al. Reference Lefort, Longueville and Gascuel2017). Bootstrap supports (Felsenstein Reference Felsenstein1985) were calculated to estimate the robustness of the clades from 100 replicates of the data. Trees were displayed with FigTree v.1.3 (Rambaut Reference Rambaut2008). Parsimony networks of haplotypes were constructed according to a 95% parsimony interval with the program TCS 1.18 (Clement et al. Reference Clement, Posada and Crandall2000).
Results
Structure and symbiont composition of Lichenomphalia meridionalis vegetative thallus
The vegetative thalli of the Lichenomphalia meridionalis samples were of the Botrydina-type (Fig. 1B), consisting of green to dark green globules measuring c. 20–55 μm diam. with an outer covering of fungal hyphae (Fig. 1B–D). The vegetative thallus of samples Lms1, Lms2, Lms3, Lms6 and Lms 8 consisted exclusively of green globules of 20–55 μm diam. containing green algae. However, the vegetative thallus of samples Lms 4, Lms 5, Lms 7, Lms 9, Lms 10, Lms 11, Lms 12, Lms 13, Lms 14 and Lms 15 contained globules of green algal cells along with globules enclosing a cyanobacterium (see Fig. 1D). The chlorobiont of L. meridionalis isolated from a fresh lichen thallus (sample Lms12) was seen as an ellipsoidal, single-celled green alga, measuring 3–5 μm × 6–12 μm (Fig. 1E).
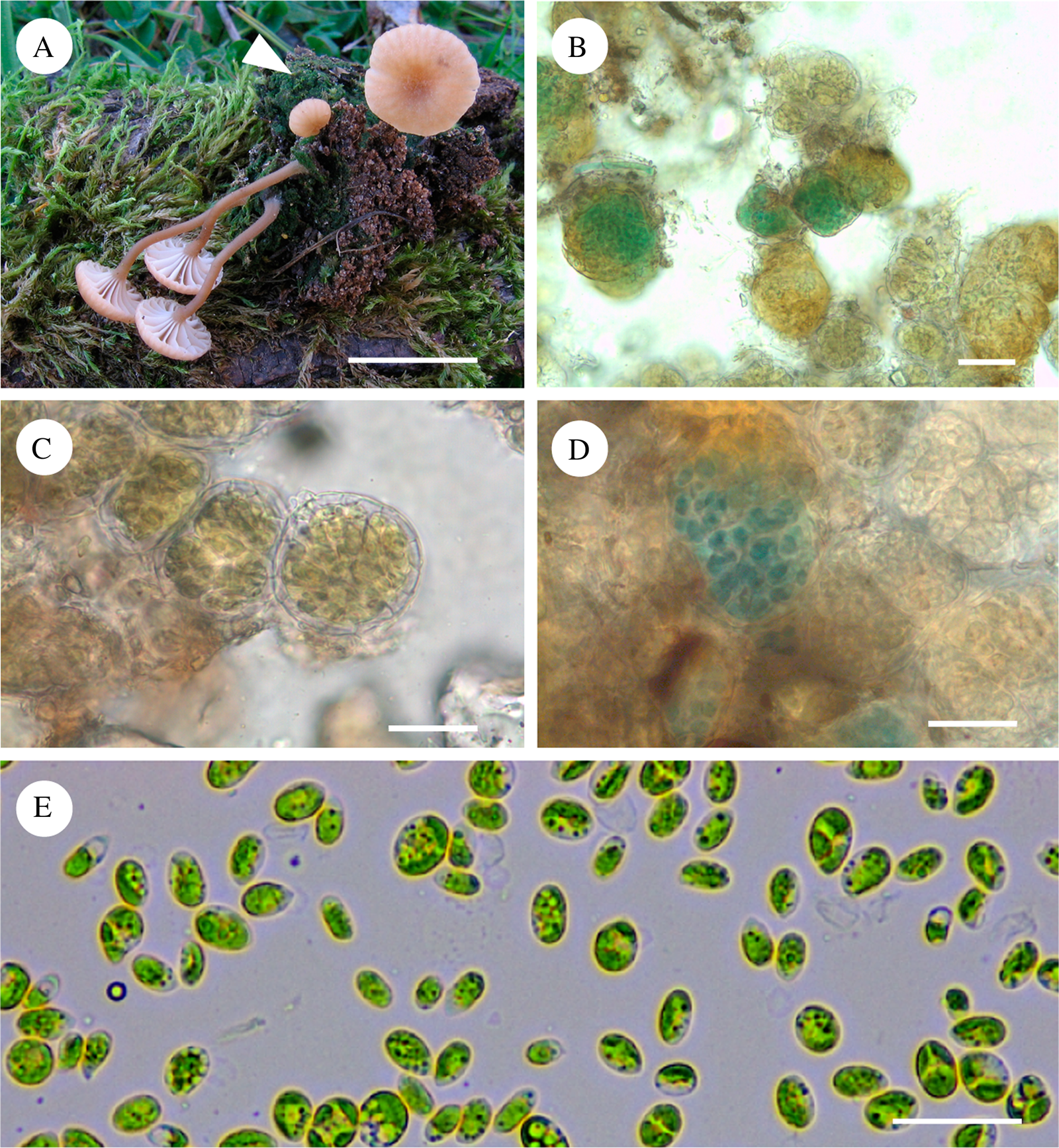
Fig. 1. Lichenomphalia meridionalis. A, basidiomata with the vegetative thallus at the base (arrowhead) (AH 32757, Lms 4); B, globules of vegetative thallus (AH 32760, Lms 11); C, vegetative thallus showing globules enclosing Coccomyxa algal cells surrounded by mycobiont hyphae (AH 32760, Lms 11); D, vegetative thallus showing globules enclosing a cyanobacterium, which appear as green-blue spots among the Coccomyxa cells inside a colourless envelope formed by fungal hyphae (AH 23937, Lms 15); E, chlorobionts isolated from L. meridionalis (AH 39243, Lms 12). Scales: A = 5 mm; B–D = 25 μm; E = 20 μm. In colour online.
Identification of the L. meridionalis mycobiont and its phylogenetic relationships within Lichenomphalia
Fifteen samples of L. meridionalis from different localities in Spain (Table 1) were macroscopically characterized by basidioma features. Pilei were ochraceous to yellowish red, convex to applanate or slightly depressed, 4–10 mm in diameter. Stipes 4–10 mm in height had a white mycelial patch at the base and decurrent lamellae concolorous with the pileus (Fig. 1A). We generated five nuclear LSU rDNA sequences of the mycobiont from herbarium specimens, some of them preserved for more than two decades, and these constitute the first molecular data available in the NCBI databases for L. meridionalis. These sequences were used to infer the phylogenetic relationships of L. meridionalis with other Lichenomphalia species. The obtained phylogeny (Fig. 2) showed L. umbellifera and the sister species L. altoandina occupying the basal position of the fungal LSU phylogram. All the remaining species belonged to a clade with two separate subclades: subclade I included three species, L. grisella (accession U66454 originally labelled as L. velutina), L. meridionalis and L. oreades (accession U66443 originally labelled as L .grisella), and subclade II included four Lichenomphalia species (L. alpina, L. hudsoniana, L. lobata and Lichenomphalia sp.). Additional analyses of the nrITS sequences from Lichenomphalia species closely related to L. meridionalis, such as L. grisella and L. oreades, revealed the existence of a microindel. This microindel must be a deletion in L. meridionalis because the other two taxa form a paraphyletic clade with respect to L. meridionalis and thus two independent, but identical insertion events would be needed to explain the observed pattern (Fig. 3A). The phylogenetic reconstruction based on a dataset of fungal nrITS sequences (Fig. 3B) showed the clustering of L. meridionalis with L. grisella (accession U66454, originally labelled as L. velutina), whereas L. oreades appeared as sister clade, including two sequences originally labelled as L. grisella (U66443, AY293949) but subsequently re-identified as L. oreades (Lücking et al. Reference Lücking, Thorn, Saar, Piercey-Normore, Moncada, Doering, Mann, Lebeuf, Voitk and Voitk2017b).
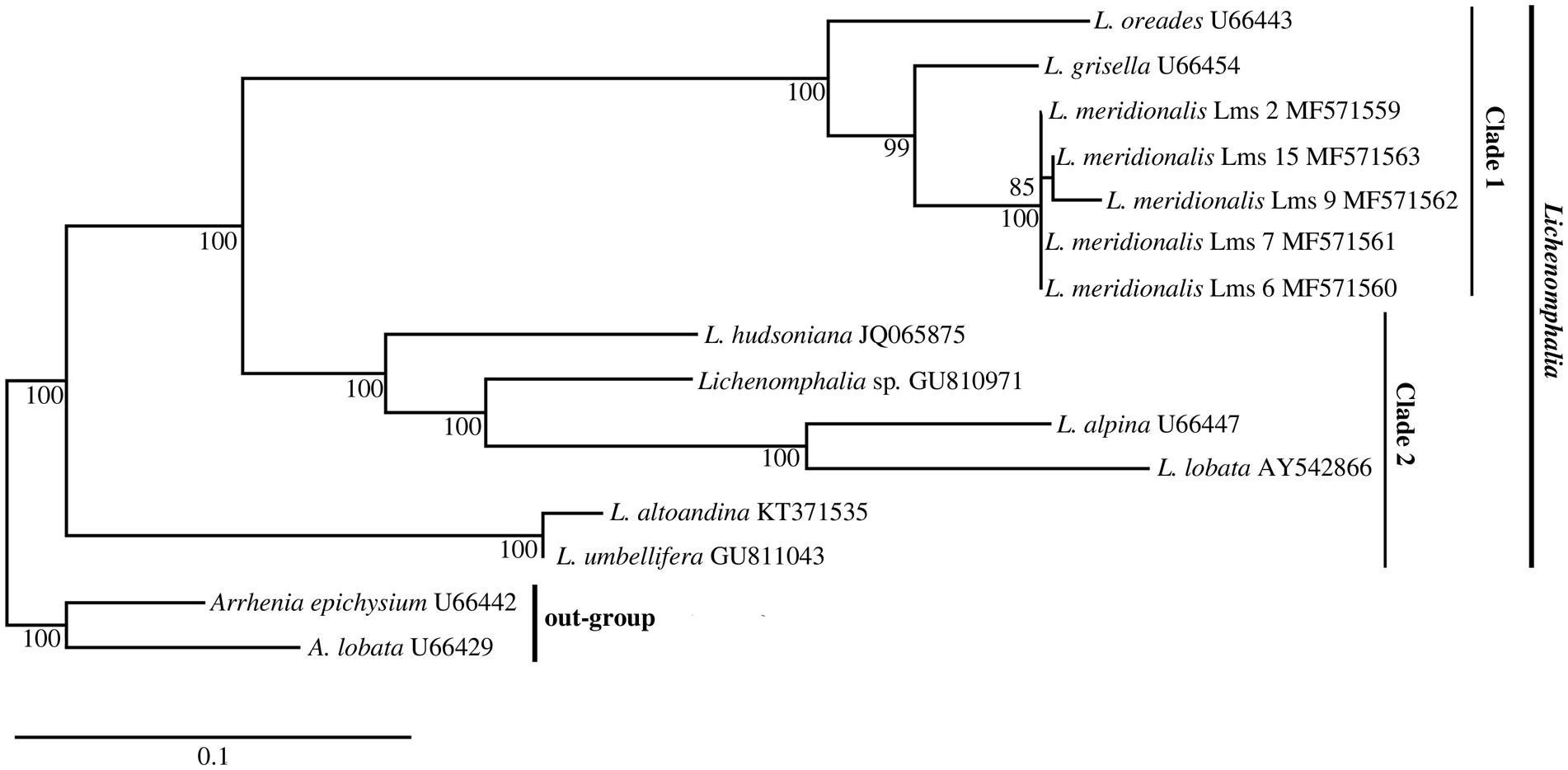
Fig. 2. Phylogram of Lichenomphalia species obtained from fungal LSU rDNA sequences. Numbers below branches are ML bootstrapping values. Vertical bars show clades representing subgenera proposed by Lodge et al. (Reference Lodge, Padamsee, Matheny, Aime, Cantrell, Boertmann, Kovalenko, Vizzini, Dentinger and Kirk2014). The phylogeny was rooted with Arrhenia spp. GenBank Accessions are shown to the right of each name. The scale bar corresponds to 0.1 nucleotide substitutions.
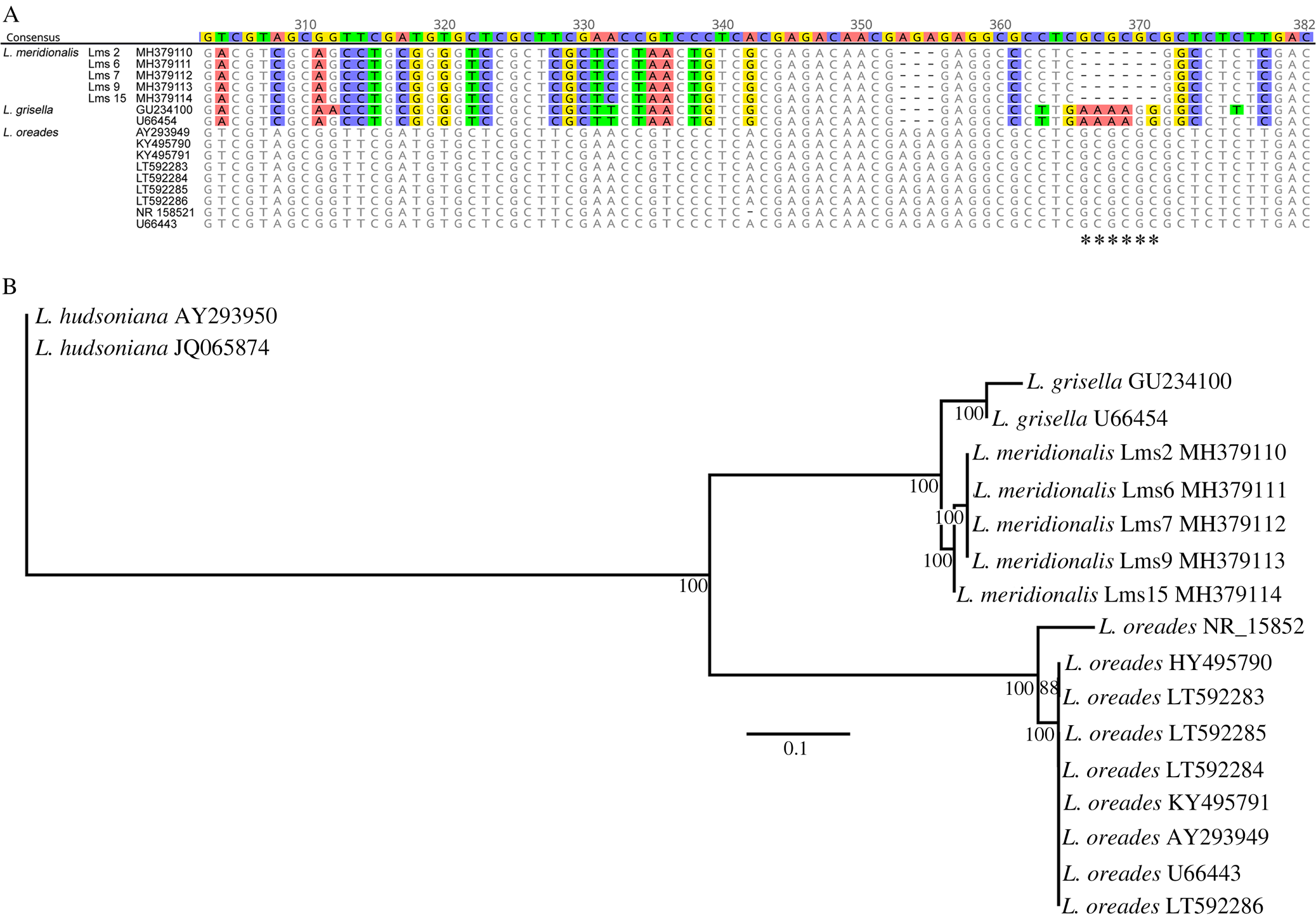
Fig. 3. Alignment and phylogenetic reconstruction based on the fungal nrITS sequences from Lichenomphalia meridionalis, L. grisella and L. oreades. A, alignment of a portion of fungal nrITS2 sequences from each of the three species; GenBank Accession numbers and sample codes are listed adjacent to species names; the discriminator microindel is labelled with asterisks. B, phylogenetic reconstruction based on the fungal nrITS sequences from each of the three species; numbers below branches are ML bootstrapping values, the phylogeny was rooted with L. hudsoniana, and GenBank Accession numbers are shown to the right of each name; the scale bar corresponds to 0.1 nucleotide substitutions. In colour online.
Identification of Lichenomphalia meridionalis chlorobionts
In order to determine the identity of the chlorobiont of L. meridionalis, we analyzed several molecular markers. Initially, no ITS sequence could be generated from any herbarium sample. However, a single sequence was obtained from algae isolated from a fresh Lms12 thallus (accession MN473374). BLAST searches carried out against the nucleotide NCBI databases retrieved a best score (470/471 identities) with Coccomyxa sp. C8 (accession AY293938) from Omphalina ericetorum (currently L. umbellifera). This sequence was used to infer the phylogenetic relationships of the chlorobiont from L. meridionalis together with other closely related Coccomyxa species. The obtained phylogeny (Fig. 4) showed the inclusion of the chlorobiont from Lms12 within a clade designated as C. subellipsoidea by Malavasi et al. (Reference Malavasi, Skaloud, Rindi, Tempesta, Paoletti and Pasqualetti2016). Since it was impossible to obtain additional ITS sequences from herbarium samples, we decided to use another molecular marker based on the sequence of the chloroplast psbB gene of C. subellipsoidea (SAG 216-13). The phylogenetic analysis of the chloroplast psbB sequences of the chlorobiont from L. meridionalis together with other closely related Coccomyxa species (Fig. 5) showed a basal lineage represented by C. dispar (labelled as strain SAG 49.84), which is the chlorobiont of Multiclavula vernalis, and at least four lineages of Coccomyxa (L1–L4). Each lineage included algae associated with either Basidiomycota (Lichenomphalia and Multiclavula) or Ascomycota (Peltigera and Solorina). Lineage L1 included C. viridis, either as chlorobiont of Peltigera aphthosa or free-living. Lineage L2 included C. subellipsoidea as chlorobiont of Lichenomphalia (L. meridionalis and L. umbellifera). Lineage L3 included C. simplex either as chlorobiont of Solorina saccata or free-living. Lineage L4 included C. dispar as chlorobiont of Multiclavula vernalis.

Fig. 4. Phylogram based on the nrITS sequences of the chlorobiont isolated from Lichenomphalia meridionalis (Lms12), together with those from other Coccomyxa microalgae. Taxon sampling and clade names are according to Malavasi et al. (Reference Malavasi, Skaloud, Rindi, Tempesta, Paoletti and Pasqualetti2016). GenBank Accession numbers of the sequences are indicated after the asterisks. Numbers below branches are ML bootstrapping values. The scale bar corresponds to 0.01 nucleotide substitutions. The phylogeny was rooted with Coccomyxa simplex.

Fig. 5. Phylogram and electropherogram obtained from chloroplast psbB sequences of Lichenomphalia meridionalis chlorobionts. A, phylogram and parsimony network of haplotypes based on the chloroplast psbB sequences of L. meridionalis chlorobionts are shown on the left and right, respectively. In the phylogram, numbers above or below branches are ML bootstrapping values. The phylogeny was rooted with Elliptochloris bilobata strain CAUP < CZH>:H7103. GenBank Accession numbers are given, and algal species and fungal hosts are indicated in parentheses. Vertical bars delimit each Coccomyxa lineage according to Darienko et al. (Reference Darienko, Gustavs, Eggert, Wolf and Pröschold2015) (L1/C. viridis, L2/C. subellipsoidea, L3/C. simplex and L4/C. dispar). Sub-lineages or strains are indicated with (a) and (b). Haplotypes found in L. meridionalis chlorobionts are indicated as 1 to 4. The scale bar corresponds to 0.1 nucleotide substitutions. In the parsimony network, each rectangle/ellipse corresponds to a particular algal type (1, 2, 3 and 4). Each bar in the network represents a single mutation step and each small empty circle represents an additional change. B, sequence electropherogram of the chloroplast psbB gene showing double peaks at specific positions (‘a’ and ‘b’, arrowed), which correspond to the differences between the sequences of the psbB haplotypes (1), (2) and (3). In colour online.
The chlorobionts of L. meridionalis were included within L2, which corresponded to C. subellipsoidea. The psbB sequences of L. meridionalis represented three haplotypes ((1) to (3) in Fig. 5A). It is likely that haplotypes (1) and (3) each belong to a different algal strain, L2a and L2b, respectively. Interestingly, sample Lms5 showed double peaks at positions corresponding to differences between haplotypes (1) and (3) (Fig. 5B), which suggests a mixture of both algal strains in this sample. Two previously sequenced samples of L. umbellifera had a single haplotype (4). Interestingly, the chlorobiont C. simplex, associated with Peltigerales such as Solorina saccata, was more closely related to C. subellipsoidea occurring in Lichenomphalia than to C. viridis associated with other Peltigerales such as Peltigera aphthosa.
Phylogenetic reconstructions and parsimony networks of haplotypes based on sequences of the nuclear RPL10A gene allowed the discrimination of four different haplotypes ((1) to (4) in Fig. 6A). The chlorobiont of L. meridionalis Lms 4 had nucleotide substitutions found in both haplotypes (1) and (3), as evidenced by double peaks at the corresponding positions (Fig. 6B). Phylogenetic reconstructions and parsimony networks of haplotypes based on sequences of the chloroplast 23S rDNA revealed the presence of three different haplotypes ((1) to (3) in Fig. 6C). Haplotype (1) was found in chlorobionts of L. umbellifera and L. meridionalis whereas haplotype (2) was found only in chlorobionts of L. meridionalis.
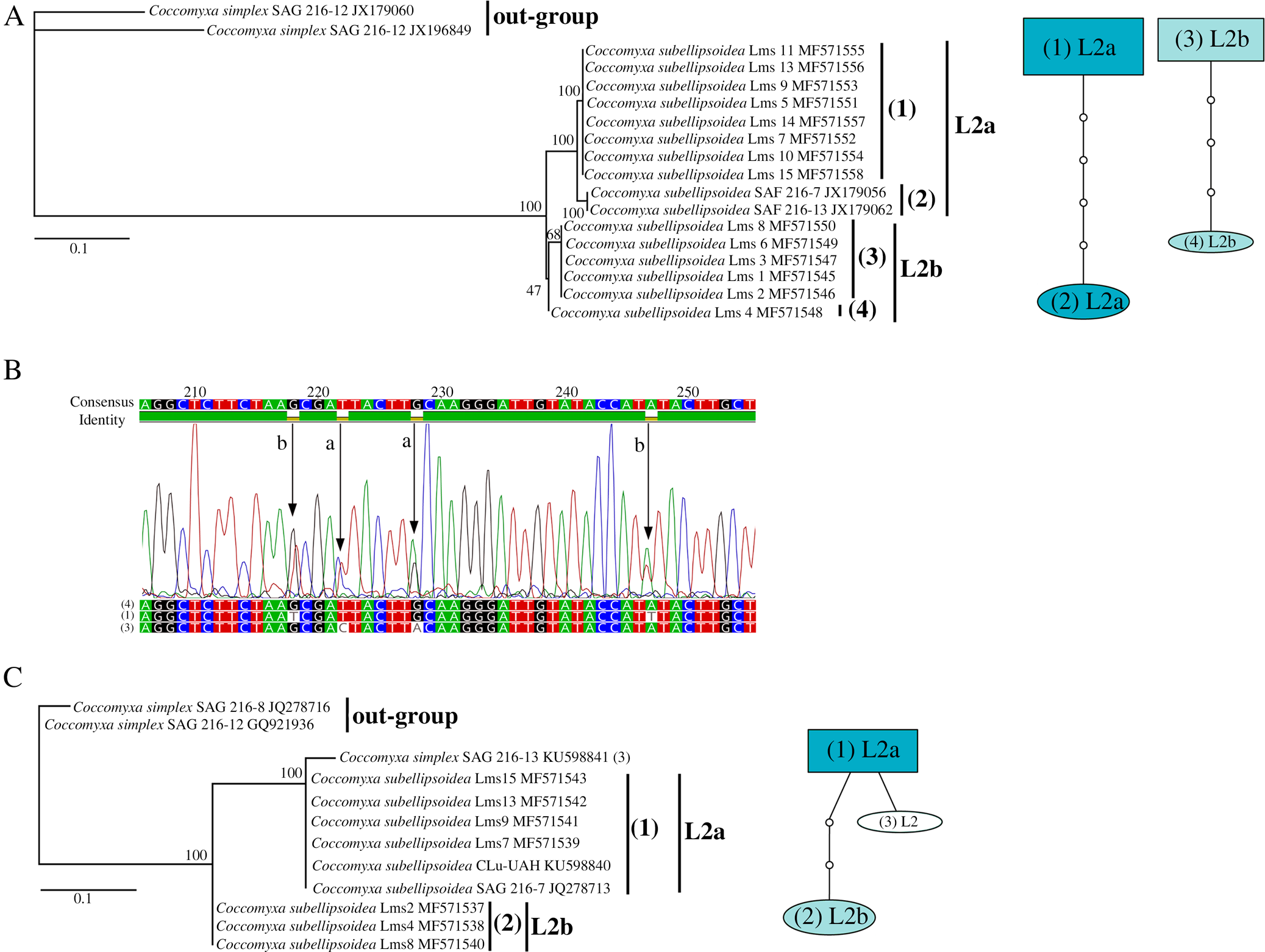
Fig. 6. Phylograms based on the nuclear RPL10A and the chloroplast 23S rDNA sequences of Lichenomphalia meridionalis chlorobionts and electropherogram of the nuclear RPL10A gene. A, phylogram and parsimony networks of haplotypes based on nuclear RPL10A sequences of L. meridionalis chlorobionts are shown on the left and right, respectively. In the phylogram, numbers above branches are ML bootstrapping values. The phylogeny was rooted with Coccomyxa simplex. GenBank Accessions are shown to the right of each name. Vertical bars separate each algal sub-lineage associated with L. meridionalis (L2a and L2b). Haplotypes found in chlorobionts are indicated as 1 to 4. The scale bar corresponds to 0.1 nucleotide substitutions. In the parsimony networks, each rectangle/ellipse corresponds to a particular algal type (1, 2, 3 and 4). Each bar in the network represents a single mutation step and each small empty circle represents an additional change. B, sequence electropherogram of the nuclear RPL10A gene showing double peaks at specific positions (‘a’–‘d’, arrowed), which correspond to the differences between the sequences of the RPL10A haplotypes (1), (3) and (4). C, a phylogram and a parsimony network of haplotypes based on chloroplast 23S rDNA sequences of L. meridionalis chlorobionts are shown on the left and right, respectively (for further explanation see A above). In colour online.
The results obtained from sequences for psbB (Fig. 5), RPL10A (Fig. 6A) and 23S rDNA (Fig. 6B) are consistent with the notion of the existence of two different strains of alga: L2a (in samples Lms 7, Lms 9, Lms 10, Lms 11, Lms 13, Lms 14 and Lms 15) and L2b (in samples Lms 1, Lms 2, Lms 3, Lms 6 and Lms 8), which may coexist in some samples (Lms 4 and Lms 5). It should be noted that the sequences obtained from the isolated chlorobiont (Fig. 1E) were identical to those obtained from the thallus and corresponded to the L2a algal strain. It is noteworthy that samples with the L2b alga were generally collected in localities at higher altitudes than samples with the L2a alga, whereas the samples found to contain both algal strains were generally collected at intermediate altitudes (c. 1393 m above sea level (a.s.l.)). Statistical analyses supported the notion that samples containing the L2a alga thrived in localities with an average altitude significantly lower (P ≤ 0.001) than those with the L2b alga: 1646 versus 1046 m a.s.l., respectively (Fig. 7). Similarly, we found a positive correlation between the association with the L2a strain of chlorobiont and the presence of a cyanobacterium, since all samples associated with L2a chlorobionts carried cyanobacteria. These results suggest the possible existence of an altitude pattern in the appearance of each algal strain associated with L. meridionalis and/or the presence of cyanobacteria (Fig. 7). However, it should be noted that the specimens were not collected along one continuous altitudinal gradient, but from various places and at different times.
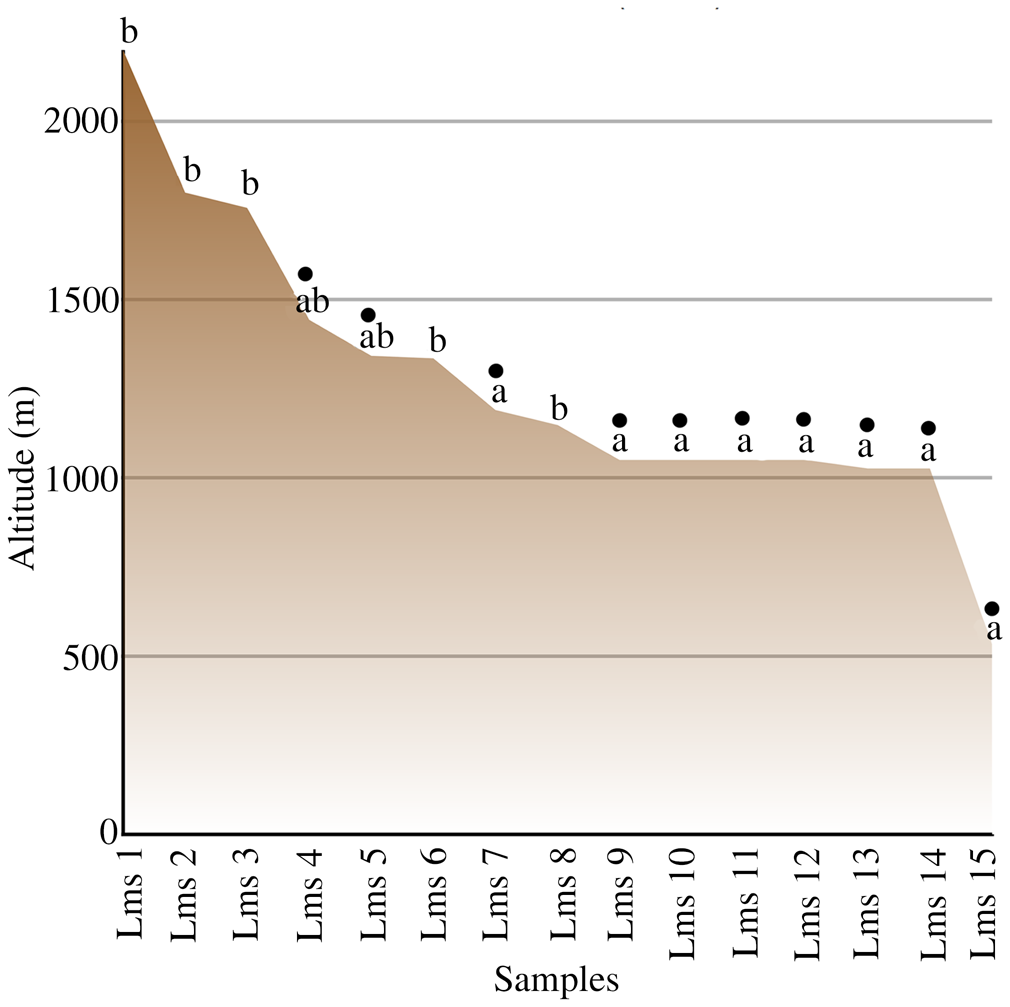
Fig. 7. The relationship between altitude (m above sea level) and occurrence of Coccomyxa photobiont strains L2a and L2b in Lichenomphalia meridionalis, together with the presence of detectable cyanobacteria associated with the thalli (filled circles). In colour online.
Discussion
Basidiolichens have been overlooked for a long time because of the very small number of species, which are restricted to special niches. Lichenomphalia species are frequently found in arctic and alpine habitats (Oberwinkler Reference Oberwinkler and Hock2012), giving rise to the traditional concept of an arctic-alpine distribution. Therefore, the particular ecology of these species has discouraged research on Lichenomphalia in Mediterranean areas such as the Iberian Peninsula. Lichenomphalia meridionalis has been frequently mistaken for L. umbellifera and the analyzed samples of L. meridionalis in the current study showed distinct morphological characteristics of the basidiomata, contrasting with previous reports on those of L. umbellifera (Barrasa & Esteve-Raventós Reference Barrasa and Esteve-Raventós2000; Barrasa & Rico Reference Barrasa and Rico2001; Barrasa et al. Reference Barrasa, Esteve-Raventós and Rico2009). Distinguishing between Lichenomphalia species based on morphology is time-consuming and requires considerable expertise on the part of the observer. In our study, we found a microindel within the fungal nrITS2 (Fig. 3A) which can assist in the discrimination of L. meridionalis from other similar species. The presence of this indel is consistent with the phylogenetic relationships of Lichenomphalia species shown previously (Lücking et al. Reference Lücking, Thorn, Saar, Piercey-Normore, Moncada, Doering, Mann, Lebeuf, Voitk and Voitk2017b) and in the present study (Fig. 3B). LSU sequences (Fig. 2) show that phylogenetic relationships between L. meridionalis and other related species such as L. grisella are comparable to those between other closely related species, such as L. altoandina and L. umbellifera (which are distinguished according to morphological and molecular data (Sandoval-Leiva et al. Reference Sandoval-Leiva, Niveiro, Urbina-Casanova and Scherson2017)). These observations indicate that L. meridionalis can also be distinguished from the other Lichenomphalia species using molecular tools.
The genus Lichenomphalia has Coccomyxa species as chlorobionts, as do other basidiolichen genera (e.g. Multiclavula) (Nelsen et al. Reference Nelsen, Lücking, Umaña, Trest, Will-Wolf, Chaves and Gargas2007). At least five Lichenomphalia species (L. grisella, L. hudsoniana, L. luteovitellina, L. velutina and L. umbellifera) share a single phylogenetic species, C. subellipsoidea (Zoller & Lutzoni Reference Zoller and Lutzoni2003). The phylogeny of Coccomyxa (Fig. 5) is based on the species delimitation described by Darienko et al. (Reference Darienko, Gustavs, Eggert, Wolf and Pröschold2015) and Malavasi et al. (Reference Malavasi, Skaloud, Rindi, Tempesta, Paoletti and Pasqualetti2016). In our study, lineages L1, L2 and L4 corresponded to C. viridis, C. subellipsoidea and C. dispar, respectively. Lineage L3 corresponded to C. simplex according to Darienko et al. (Reference Darienko, Gustavs, Eggert, Wolf and Pröschold2015) or to C. simplex (C. chodatii and C. rayssiae) and C. solorinae (C. solorinae-saccatae) according to Malavasi et al. (Reference Malavasi, Skaloud, Rindi, Tempesta, Paoletti and Pasqualetti2016). The chlorobionts of L. meridionalis occurred within the L2 lineage, which corresponds to C. subellipsoidea, and is closely related to the chlorobionts of other Lichenomphalia species (see Zoller & Lutzoni Reference Zoller and Lutzoni2003). The presence of two different algal strains in the L2 lineage, which appeared to coexist in some samples, indicates that L. meridionalis thalli apparently represent a specific and selective form of symbiotic association. Strict preservation of this pattern of algal coexistence probably facilitates the proliferation of this lichen in a very restrictive habitat. In this study, lineage L2a represents C. subellipsoidea based on the inferred position of the ITS sequence from Lms12 in the phylogram of Fig. 4 and in relation to SAG 216 strains in Figs 4 and 5. However, the identity of lineage L2b is not clear because of the lack of ITS sequences from any herbarium sample. The phylogram in Fig. 4 is very similar to that of Malavasi et al. (Reference Malavasi, Skaloud, Rindi, Tempesta, Paoletti and Pasqualetti2016), which emphasized the importance of ecological differences among Coccomyxa species and recognized C. subellipsoidea and closely related clades as distinct species units. If L2b represents any such clade (e.g. clade F), then the ecological distinction between L2a and L2b would support the species delimitation criteria proposed by Malavasi et al. (Reference Malavasi, Skaloud, Rindi, Tempesta, Paoletti and Pasqualetti2016).
Our finding of globules containing a cyanobacterium in some L. meridionalis thalli corresponds to the notion that some other lichens contain both a green microalga (chlorobiont) and a cyanobacterium, which is generally localized in special structures, the cephalodia (Honegger Reference Honegger1993, Reference Honegger and Hock2012). Peltigera aphthosa (Peltigeraceae) is one of the most studied lichens with cephalodia, containing a green microalga from the genus Coccomyxa and a nitrogen-fixing cyanobacterium from the genus Nostoc (Büdel & Scheidegger Reference Büdel, Scheidegger and Nash2008). In this lichen, an increase in the abundance of cephalodia is indicative of higher rates of nitrogen fixation, as a consequence of a lower availability of nitrogen in the environment and serves as a potential marker of nitrogen and phosphorous content in the soil (Asplund & Wardle Reference Asplund and Wardle2015). Further analyses employing more specific molecular and microscopy techniques will be necessary to demonstrate whether cyanobacteria are stable partners of the Lichenomphalia holobiont, or have a commensalist relationship, or whether their coexistence is just due to common ecological preferences. If they indeed represent cephalodia, they can best be compared to internal cephalodia, for instance found in Lobaria pulmonaria.
The present study suggests that altitude influences the distribution of the coexisting chlorobionts (strains a and b) associated with the vegetative thallus of L. meridionalis. The occurrence of different photobionts along an altitudinal (climatic) gradient has been reported for epilithic lichens (Kroken & Taylor Reference Kroken and Taylor2000; Blaha et al. Reference Blaha, Baloch and Grube2006). Similarly, Řeháková et al. (Reference Řeháková, Chlumská and Doležal2011) demonstrated that soil phototrophs form complex communities with specific environmental associations and depend on site, altitude and vegetation type. Our results suggest that temperature might limit or facilitate the growth of the two microalgal strains. However, additional environmental factors such as humidity and soil characteristics (texture, pH, availability of mineral nutrients and organic matter content), which do not necessarily depend on altitude, cannot be excluded. Previous studies of the community composition of Trebouxia chlorobionts along an altitudinal gradient in the Mediterranean region revealed that both altitude and host genetic identity were strong predictors of photobiont community assembly (Dal Grande et al. Reference Dal Grande, Rolshausen, Divakar, Crespo, Otte, Schleuning and Schmitt2018). The lichen Ramalina farinacea establishes associations with two different Trebouxia species (TR1 and TR9) that always coexist within the thallus, but their relative proportions vary depending on ecological conditions (Casano et al. Reference Casano, del Campo, García-Breijo, Reig-Arminana, Gasulla, Del Hoyo, Guéra and Barreno2011). In vitro experiments have demonstrated that the abundance of each species is related to their physiological performance: whereas TR9 grows better under relatively high temperatures and irradiances, TR1 thrives under more temperate and shady conditions. Further studies on the physiological performance of each Coccomyxa strain in L. meridionalis under different environmental conditions could shed light on the influence of specific environmental conditions on the photobiont composition of this basidiolichen.
Acknowledgements
We would like to thank Dr Robert Lücking (Botanic Garden and Botanical Museum Berlin-Dahlem, Free University of Berlin) for his invaluable guidance and advice in the writing and presentation of this paper. This work was supported by the Spanish Secretary of Research and Innovation (grant no. CGL2012-40058-C02-02) and the Department of Education, Research and Innovation of the Government of the Autonomous Community of Castile-La Mancha (Spain), co-financed by the European Regional Development Fund (ERDF) (grant no. POII-2014-016A).
Supplementary Material
To view supplementary material for this article, please visit https://doi.org/10.1017/S002428291900046X