Introduction
Silages constitute an essential component of ruminant diets, especially because limited vegetation periods reduce the availability of fresh herbage. The principle of ensiling is the fermentation of sugars to lactic acid under anaerobic conditions by hetero- and homofermentative lactic acid bacteria (LAB), which reduces pH and consequently suppresses spoilage microorganisms (McDonald et al., Reference McDonald, Henderson, Heron and Marlow1991). To promote sufficient lactic acid fermentation in the silo and therefore superior silage quality, high abundances of LAB, in particular homofermentative LAB, and low abundances of spoilage microbes are desired (McDonald et al., Reference McDonald, Henderson, Heron and Marlow1991; Muck, Reference Muck2010).
The majority of silage microbiology-related studies applied cultivation methods, which cover only a small proportion of the present microorganisms (McAllister et al., Reference McAllister, Dunière, Drouin, Xu, Wang, Munns and Zaheer2018). Besides, several bacteria and fungi can change to a viable but not-cultivable state when they are exposed to stress (del Mar Lleo' et al., Reference del Mar Lleo’, Tafi and Canepari1998; McAllister et al., Reference McAllister, Dunière, Drouin, Xu, Wang, Munns and Zaheer2018), which should inevitably be triggered by oxygen contact when opening silos for sample collection. To avoid such limitations, the application of culture-independent approaches has notably increased in recent decades (Schmidt et al., Reference Schmidt, Emara and Kung2008; Eikmeyer et al., Reference Eikmeyer, Köfinger, Poschenel, Jünemann, Zakrzewski, Heinl, Mayrhuber, Grabherr, Pühler, Schwab and Schlüter2013; McAllister et al., Reference McAllister, Dunière, Drouin, Xu, Wang, Munns and Zaheer2018). By now, microbial community compositions can comprehensively be assessed by 16S rRNA gene sequencing. However, this method typically allows a reliable classification only up to genus level and therefore cannot provide differentiated information on the presence of hetero- and homofermentative LAB. As lactic acid fermentation by homofermentative LAB is targeted during ensiling (McDonald et al., Reference McDonald, Henderson, Heron and Marlow1991), quantification of this specific group within the genus Lactobacillus is indeed of great interest. Therefore, quantitative real-time polymerase chain reaction (qPCR) represents a culture-independent method allowing species-specific identification (Schmidt et al., Reference Schmidt, Emara and Kung2008) along with an absolute quantification, which is impossible when using sequencing-based approaches (Rebollar et al., Reference Rebollar, Antwis, Becker, Belden, Bletz, Brucker, Harrison, Hughey, Kueneman, Loudon, McKenzie, Medina, Minbiole, Rollins-Smith, Walke, Weiss, Woodhams and Harris2016). In fact, the quantitative aspect may be of substantial informative value as treatment effects could rather arise from overall varying microbial loads than from differences in the relative microbial composition.
Regarding spoilage of silages, high abundances of enterobacteria and clostridia are associated with excessive proteolysis, formation of biogenic amines and production of acetic and butyric acid (McDonald et al., Reference McDonald, Henderson, Heron and Marlow1991; Kung and Shaver, Reference Kung and Shaver2001; Hoedtke et al., Reference Hoedtke, Gabel and Zeyner2010), altogether leading to high energy losses and poor silage quality. In consequence, homo- and heterofermentative LAB species, such as Lact. plantarum and Lact. buchneri, as well as spoilage-related enterobacteria and clostridia, may primarily determine silage quality and therefore constitute bacterial key players during ensiling as thoroughly elucidated by Muck (Reference Muck2010). It was hypothesized that the abundance of these bacterial key players is related to silage quality and that different lucerne (Medicago sativa L.) silage treatments will cause varying silage qualities and also quantities of these bacterial key players. Thus, lucerne silages produced with different additives and ensiled at two dry matter (DM) concentrations (280 and 380 g/kg) were analysed for fermentation characteristics as well as absolute abundances of total bacteria, Lactobacillus spp., Lact. plantarum, Lact. buchneri, Clostridium spp. and Enterobacteriaceae.
Materials and methods
Preparation of lucerne silages
Samples for the fermentation product and microbial analyses were obtained from an experiment conducted by Scherer et al. (Reference Scherer, Gerlach, Taubert, Adolph, Weiß and Südekum2019), who comprehensively described the preparation of the lucerne silages. Briefly, a pure stand of lucerne (cultivar Plato; first cut) was harvested at the late vegetative stage. The plant material was separated into two equal proportions and wilted either to a low DM concentration of ~280 g/kg or to a high DM concentration of ~380 g/kg. The chemical composition of wilted substrates before ensiling and the basic microbiological characterization of harvested lucerne are presented in Table 1.
Table 1. Chemical composition of wilted plant material before ensiling and basic microbiological characterization of harvested lucerne
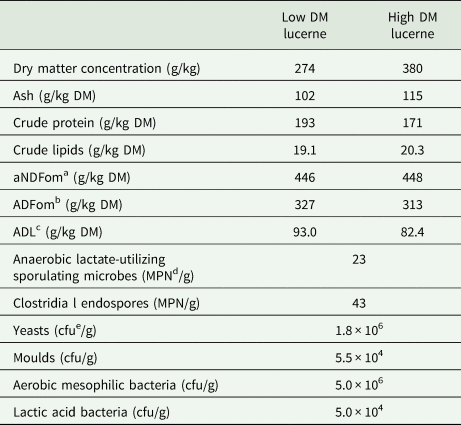
a Neutral detergent fibre assayed with heat-stable amylase and expressed exclusive residual ash.
b Acid detergent fibre expressed exclusive residual ash.
c Acid detergent lignin.
d Most probable number.
e Colony-forming units.
Lucerne was sampled directly after harvesting.
Before ensiling in 1.5 l glass jars, the wilted lucerne was treated with either one of three silage additives or soil (Table 2). All applied silage additives were selected due to their scope of application and mode of action as recommended by the Bundesarbeitskreis Futterkonservierung (2011). For instance, formic acid-based silage additives are proposed for difficultly fermentable substrates with low DM, i.e. less than 300 g/kg, whereas LAB-based additives are suited for DM concentration higher than 300 g/kg. Similarly, a targeted soil addition to induce clostridial fermentation is more promoted under moist conditions (Kung et al., Reference Kung, Shaver, Grant and Schmidt2018) and therefore was added to the low DM lucerne material. Control silages for low and high DM concentration, respectively, were included, resulting in six different treatments that were each prepared in triplicate. Consequently, a broad variety of silage fermentation qualities was produced on purpose.
Table 2. Overview of lucerne treatments and the associated dry matter (DM) concentrations
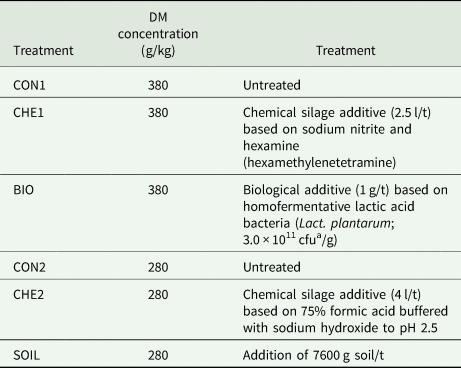
a Colony-forming units.
Additionally, sucrose at 125 g/kg DM was added to all treatments to exclude a lack of rapidly-fermentable carbohydrates in the silo as the buffering capacity is high, but the availability of sugars is naturally low in lucerne and therefore impedes sufficient lactic acid fermentation (Seale et al., Reference Seale, Henderson, Pettersson and Lowe1986; Hartinger et al., Reference Hartinger, Gresner and Südekum2019). In the following, the high DM lucerne silages are referred to as CON1, CHE1 and BIO and the low DM lucerne silages as CON2, CHE2 and SOIL (Table 2). After 30 days of storage, the glass jars were opened and samples were taken for analysis of fermentation products and DNA extraction.
Analysis of fermentation products
The analysis of the fermentation products was performed as described in detail by Scherer et al. (Reference Scherer, Gerlach, Taubert, Adolph, Weiß and Südekum2019). Briefly, a cold-water extract was prepared from all samples, respectively, and the pH of the extracts was determined potentiometrically. The lactic acid concentration was determined by high-performance liquid chromatography with refractive index detection and the ammonia-nitrogen (N) concentration was analysed by colorimetry based on the Berthelot reaction (Hinds and Lowe, Reference Hinds and Lowe1980). Acetic acid, butyric acid, 1,2-propanediol and ethanol concentrations were analysed by gas chromatography and the concentration of water-soluble carbohydrates (WSC) was determined using the anthrone method (von Lengerken and Zimmermann, Reference von Lengerken and Zimmermann1991). Additionally, the DM and total N concentrations were analysed according to Association of German Agricultural Analytic and Research Institutes (VDLUFA, 2012). The DM concentration was determined by drying the fresh lucerne silages overnight at 60°C and subsequently at 105°C for at least 3 h (method 3.1). Afterwards, DM was corrected for losses of volatile compounds by applying the equation of Weissbach and Kuhla (Reference Weissbach and Kuhla1995). Using the Kjeldahl technique (method 4.1.1), the total N concentration was determined in a Vapodest 50s carousel (Gerhardt, Königswinter, Germany).
DNA extraction
Prior to DNA extraction, each fresh lucerne silage sample was treated with liquid N and ground using a mortar and pestle. Subsequently, total DNA was extracted from ~90 mg of ground material using the First-DNA all tissue kit (GEN-IAL GmbH, Troisdorf, Germany) according to the manufacturer's protocol with specific modifications. Briefly, the lysis buffer volumes were increased, a bead-beating step (Precellys® 24 tissue homogenizer, Bertin Instruments, Montigny-le-Bretonneux, France) was included to increase DNA recovery as well as an RNase A treatment (VWR International GmbH, Darmstadt, Germany) to remove RNA contamination. Both DNA yield and purity were determined by spectrophotometry using a Nanodrop 8000 (NanoDrop® Technologies, Thermo Scientific, Waltham, MA, USA). The integrity of the DNA was checked by 1% (w/v) agarose gel electrophoresis and DNA extracts were stored at −20°C until further qPCR analysis.
Quantitative real-time polymerase chain reaction
The qPCR analysis was conducted in accordance with the MIQE guidelines (Bustin et al., Reference Bustin, Benes, Garson, Hellemans, Huggett, Kubista, Mueller, Nolan, Pfaffl, Shipley, Vandesompele and Wittwer2009). Before analysing the samples, DNA amount per reaction and primer conditions, i.e. annealing temperature and primer concentration, were optimized using a pool sample as recommended (Rochelle et al., Reference Rochelle, Leon, Stewart and Wolfe1997; Bustin et al., Reference Bustin, Benes, Garson, Hellemans, Huggett, Kubista, Mueller, Nolan, Pfaffl, Shipley, Vandesompele and Wittwer2009; Mullins et al., Reference Mullins, Mamedova, Carpenter, Ying, Allen, Yoon and Bradford2013). In addition, the primer efficiency was tested (Bustin et al., Reference Bustin, Benes, Garson, Hellemans, Huggett, Kubista, Mueller, Nolan, Pfaffl, Shipley, Vandesompele and Wittwer2009).
All qPCR reactions were performed on a StepOnePlus™ Real-Time PCR System (Thermo Scientific GmbH, Schwerte, Germany) associated with the StepOne™ Software v2.1 (Thermo Scientific GmbH, Schwerte, Germany). Each reaction contained 10 μl primaQUANT CYBR-Green mix (Steinbrenner Laborsysteme GmbH, Wiesenbach, Germany), the respective primer proportion (Table 3) and 1 μl template DNA (10 ng/μl) and was then filled up with polymerase chain reaction (PCR) grade water (VWR International GmbH, Darmstadt, Germany) to a final volume of 20 μl. The reaction mixtures were loaded on MicroAmp® Fast Optical 96-Well reaction plates (Thermo Scientific GmbH, Schwerte, Germany) and sealed with StarSeal Advanced Polyolefin Film (StarLab, Hamburg, Germany). The cycling conditions consisted of an initial denaturation step for 10 min at 95°C, followed by 40 cycles of: 5 s at 95°C, 20 s at the respective annealing temperature and an elongation for 1 s at 72°C. The fluorescence signals were read at the end of each cycle. Finally, a melt curve was generated by increasing the temperature stepwise (0.3°C) from 60°C to 95°C.
Table 3. Oligonucleotide primers used for qPCR

a Forward.
b Reverse.
For absolute quantification of the 16S rRNA gene copy numbers, internal standards were created using a pool sample (Kaewtapee et al., Reference Kaewtapee, Burbach, Tomforde, Hartinger, Camarinha-Silva, Heinritz, Seifert, Wiltafsky, Mosenthin and Rosenfelder-Kuon2017). Standards were purified using the GeneJET PCR Purification Kit (Thermo Scientific, Schwerte, Germany) and fluorometrically quantified using the Synergy HTX Multi-Mode Reader (BioTek Instruments, Inc., Winooski, VT, USA) and the Quant-iT™ dsDNA Broad-Range Assay Kit (Thermo Scientific, Schwerte, Germany). The samples were analysed in duplicate and the 10-fold dilution series of the respective standards were run along in triplicate. Furthermore, no-template controls were added on each plate in triplicate. All PCR products were checked by melt curve analysis and 2% (w/v) agarose gel electrophoresis.
Calculation of 16s rRNA gene copy numbers and statistical analysis
The starting 16S rRNA gene copy numbers were determined by relating the C t values to the respective standard curves. Subsequently, the 16s rRNA gene copy numbers per gram lucerne silage were calculated according to the equation (Li et al., Reference Li, Penner, Hernandez-Sanabria, Oba and Guan2009):

where QM refers to the quantity mean of gene copy numbers, C is the DNA concentration of each sample, DV refers to the dilution volume of extracted DNA, S refers to the DNA amount (ng) for each reaction and V refers to the weight of the sample (g) subjected to DNA extraction. Finally, the calculated gene copy numbers were Log10 transformed to obtain Log10 16S rRNA gene copies per gram silage.
The statistical analysis was performed using the UNIVARIATE procedure of SAS version 9.4 (SAS Institute Inc., Cary, NC, USA) to test normal distribution of the data by analysis of the residuals. Further, the effect of lucerne silage treatment was tested by one-way analysis of variance using the GLM procedure of SAS version 9.4 (SAS Institute Inc., Cary, NC, USA) with the following model:

where Y i is the observed response, μ is the mean, Ti is the treatment effect and ei is the residual error. Differences between the least squares means were post-hoc tested using a Tukey's test. Significance was defined at P < 0.05 and a trend at 0.05 ≤ P < 0.1.
Results
Fermentation products in differently treated lucerne silages
Highest pH was observed for CHE1, followed by CON1 and then the four remaining treatments, which had the lowest pH and did not differ from each other (Table 4). Except for CHE1, lactic acid concentration was more than twice the concentration of acetic acid. Moreover, acetic acid concentration was highest for SOIL. Likewise, also ethanol concentration was highest for SOIL and lowest for CHE1 and CHE2 with CON1, CON2 and BIO in between. The ratio of lactic to acetic acid was lowest for CHE1 and highest for BIO, whereas the ammonia-N concentration was highest for SOIL and differed from CON1, BIO and CHE2, with the latter treatment showing the lowest ammonia-N concentration. The WSC concentration was highest in CHE1 and lowest in CON2 and SOIL. Butyric acid and 1,2-propanediol were not detected in the lucerne silages.
Table 4. Means of fermentation products of differently treated lucerne silages
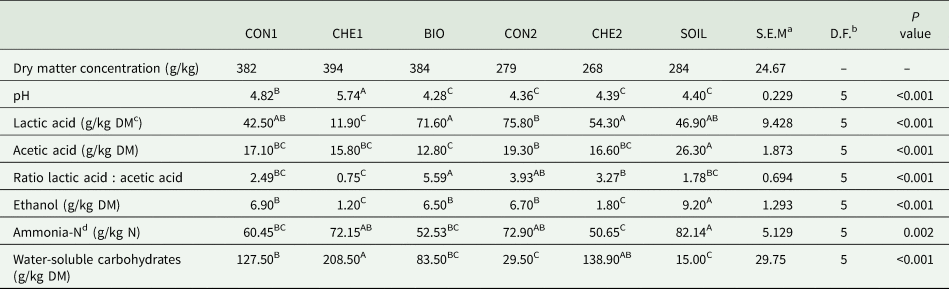
a Standard error of the mean.
b Degrees of freedom.
c Dry matter.
d Nitrogen.
Different superscript capitals within a row indicate significant differences (P < 0.05) for treatment effect.
Absolute abundances of bacterial key players in differently treated lucerne silages
Irrespective of silage additive or soil treatment, concentrations of LAB were substantially higher than spoilage microorganisms, i.e. Enterobacteriaceae and Clostridium spp. (Table 5). Silage treatments had an effect on the abundance of Lactobacillus spp. with higher gene copy numbers for SOIL and CON2 than for CHE1. On LAB species level, homofermentative Lact. plantarum was more abundant in all lucerne silages than heterofermentative Lact. buchneri. Among all treatments, CHE1 had the lowest concentration of Lact. plantarum. The inoculation with viable Lact. plantarum cultures (BIO) resulted in the highest concentration of this LAB species, but lowest concentrations of Lact. buchneri. No treatment effects or trends were observed for gene copy numbers of total bacteria, Enterobacteriaceae and Clostridium spp.
Table 5. Absolute abundances of bacterial key species (mean Log10 16S rRNA gene copy numbers/g silage) in differently treated lucerne silages

a Standard error of the mean.
b Degrees of freedom.
Different superscript capitals within a row indicate significant differences (P < 0.05) for treatment effect.
Discussion
The abundance of total bacteria was within a small range for all investigated lucerne silages and not affected by the treatments. Therefore, differences in fermentation quality seemed not to derive from overall distinct bacterial concentrations, but differences in the relative composition. Concerning the abundance of Lactobacillus spp., present concentrations were higher than those reported by Schmidt et al. (Reference Schmidt, Hu, Mills and Kung2009) or Zheng et al. (Reference Zheng, Niu, Jiang, Zuo and Xu2017). However, sucrose was added to lucerne before ensiling, thus enhancing competitiveness of LAB by providing metabolizable substrate (Driehuis and Oude Elferink, Reference Driehuis and Oude Elferink2000), which likely allowed their higher proliferation compared to the untreated lucerne silages from Schmidt et al. (Reference Schmidt, Hu, Mills and Kung2009) and Zheng et al. (Reference Zheng, Niu, Jiang, Zuo and Xu2017).
Lactobacillus plantarum was predominant for all treatments, which is in line with other studies observing a strong and fast proliferation of Lact. plantarum in both untreated silages or silages inoculated with Lact. plantarum and Lact. buchneri, respectively (Stevenson et al., Reference Stevenson, Muck, Shinners and Weimer2006; Pang et al., Reference Pang, Qin, Tan, Li, Wang and Cai2011; Guo et al., Reference Guo, Ke, Ding, Ding, Xu, Wang, Zhang and Yang2018). Guo et al. (Reference Guo, Ke, Ding, Ding, Xu, Wang, Zhang and Yang2018) reported highest abundance of Lact. plantarum in silages inoculated with this species, which is supported by the present observations for BIO. As indicated by the low pH of 4.28, lowest concentration of acetic acid and second highest lactic acid concentration among all lucerne silages, the silage quality of BIO can be assessed as excellent (Kung et al., Reference Kung, Shaver, Grant and Schmidt2018; Scherer et al., Reference Scherer, Gerlach, Taubert, Adolph, Weiß and Südekum2019). Consequently, treatment with viable Lact. plantarum cultures promoted an intensive lactic acid fermentation resulting in a well-preserved silage and therefore suggests a positive linkage between the abundance of Lact. plantarum and an improved lucerne silage quality. Consequently, this finding supports the present hypothesis and confirms that homofermentative LAB inoculants ensure rapid lactic acid fermentation early in storage as priorly stated by Muck (Reference Muck2010).
Concentrations of Lactobacillus spp. and Lact. plantarum for CHE1 were among the lowest observed and likely caused by the silage additive ingredients sodium nitrite and hexamine. Both chemical compounds possess antimicrobial properties (Woolford, Reference Woolford1975; Lingvall and Lättemäe, Reference Lingvall and Lättemäe1999), which were earlier shown to reduce clostridia (Lingvall and Lättemäe, Reference Lingvall and Lättemäe1999) as well as LAB (Woolford, Reference Woolford1975). However, although CHE1 reduced the concentrations of LAB, no effect on gene copies of Enterobacteriaceae and Clostridium spp. was observed.
The reducing effect of CHE1, compared with all other treatments, on Lactobacillus gene copies, in particular homofermentative Lact. plantarum, was reflected in the silage fermentation pattern by a higher pH and lower lactic acid concentration. Thus, the decrease in LAB should have led to less lactic acid fermentation, which in turn inhibited a sufficient pH drop (Pahlow et al., Reference Pahlow, Muck, Driehuis, Oude Elferink, Spoelstra, Buxton, Muck and Harrison2003). Likewise, the high WSC concentration in CHE1 supports this assumption of reduced LAB activity and is in accordance with findings of McEniry et al. (Reference McEniry, O'Kiely, Clipson, Forristal and Doyle2007) and Conaghan et al. (Reference Conaghan, O'Kiely and O'Mara2010). Together with the low ethanol concentration, the high WSC concentration in CHE1 further indicated an inhibition of yeasts in this treatment as the main metabolite of sugar fermentation by yeasts is ethanol (McDonald et al., Reference McDonald, Henderson, Heron and Marlow1991). Accordingly, also Conaghan et al. (Reference Conaghan, O'Kiely and O'Mara2010) associated high WSC concentration in chemically treated perennial ryegrass silages with an inhibiting effect on yeasts of these chemicals, i.e. sodium nitrite and hexamine, which is also described by Bundesarbeitskreis Futterkonservierung (2011). However, because yeast concentrations were not determined in the present study, future investigations are needed for clarification. Further, the short ensiling duration of 30 days must be taken into account when interpreting the WSC concentrations, which should – depending on the extent of pH reduction – further decrease with prolonged silo storage. Hence, further efforts are needed to describe fermentation patterns and microbial abundances over longer storage periods.
It is worthy of remark that CHE1 seemed to have selectively suppressed homofermentative LAB as Lact. plantarum concentration was lowest in CHE1 and significantly lower compared to BIO, SOIL and CHE2, whereas Lact. buchneri concentration in CHE1 did not differ from these treatments. This is in accordance with Conaghan et al. (Reference Conaghan, O'Kiely and O'Mara2010), who also observed decreased lactic acid fermentation coupled with increased acetic acid concentration and concluded that acetic acid production by heterofermentative LAB was causative. This may be particularly true as enterobacteria-based fermentation would generate equimolar amounts of acetic acid and ethanol (McDonald et al., Reference McDonald, Henderson, Heron and Marlow1991), which was not the case for CHE1 and can therefore be excluded. As the ratio of lactic to acetic acid was lowest in CHE1 and significantly lower compared with BIO, CON2 and CHE2, a shift towards extended heterofermentative lactic acid fermentation was also indicated by this fermentation characteristic. However, the absence of 1,2-propanediol, which is a typical metabolite of Lact. buchneri (Oude Elferink et al., Reference Oude Elferink, Krooneman, Gottschal, Spoelstra, Faber and Driehuis2001), might partly question a substantial metabolic activity of this heterofermentative LAB species during lucerne ensiling.
The lack of treatment effects on gene copy numbers of enterobacteria and clostridia are contrary to the hypothesis, as clostridia access silages mainly via soil contamination (McDonald et al., Reference McDonald, Henderson, Heron and Marlow1991). Therefore, concentration of Clostridium spp. was expected to be higher in SOIL silages, though it might be that the extent of soil contamination was too low to affect the abundance of this genus. Concerning silage quality characteristics, SOIL silages showed moderate lactic acid and high acetic acid concentrations as well as highest concentrations of ammonia-N and ethanol. Thus, the presence of the bacterial key players did not completely match silage quality. In fact, the high concentration of Lactobacillus spp. in SOIL was surprising and not reflected by a corresponding lactic acid concentration. However, it should again be noted that acetic acid can also be produced by heterofermentative LAB (McDonald et al., Reference McDonald, Henderson, Heron and Marlow1991) and thus, in turn, could partly explain the high acetic acid concentration but still low pH of SOIL silages. Besides, the highest ammonia-N and, although not statistically significant, highest concentrations of Enterobacteriaceae in SOIL still suggested that soil contamination was detrimental for silage quality. In addition, it can be speculated whether yeast concentration was promoted by soil treatment as indicated by the highest ethanol concentration of all silages as it may not solely derive from heterofermentative LAB and enterobacteria activity (Kung et al., Reference Kung, Shaver, Grant and Schmidt2018).
Other studies also reported inhibiting effects on clostridial proliferation by formic acid and Lact. plantarum inoculation (e.g. Wen et al., Reference Wen, Yuan, Wang, Desta and Shao2017; Zheng et al., Reference Zheng, Niu, Jiang, Zuo and Xu2017; Guo et al., Reference Guo, Ke, Ding, Ding, Xu, Wang, Zhang and Yang2018), but no effects of CHE2 or BIO on Clostridium spp. were observed in the present study. However, CHE2 showed numerically lowest Clostridium spp. concentration accompanied by lowest ammonia-N concentration, which still points to higher protein quality in silages being positively related to lower abundance of clostridia. Thus, in terms of spoilage bacteria, the concentrations of enterobacteria and clostridia only partly reflected silage quality and thus did not fully support the hypothesized relation between microbial abundance and silage quality. It has to be considered that the abundance of a specific group might not be equally related to its metabolic activity and could further be prone to overestimation by enumerating DNA from nonviable and dead cells during qPCR (McAllister et al., Reference McAllister, Dunière, Drouin, Xu, Wang, Munns and Zaheer2018). Concerning the abundance of LAB species, however, the hypothesis of a relation between microbial abundances and silage quality was confirmed in the present study.
Conclusions
The applied silage additive and soil treatments affected silage quality characteristics and also concentrations of the bacterial key players Lactobacillus spp., Lact. plantarum and Lact. buchneri, whereas total bacteria, Enterobacteriaceae and Clostridium spp. were not affected. Additionally, some treatments seemed to affect yeast proliferation, which should be assessed in future studies. Concerning LAB, the relation between their abundance and improved silage quality could be confirmed, which was particularly true for homofermentative Lact. plantarum. Regarding the spoilage-related bacterial key players, however, the treatment effects on silage quality characteristics were not fully reflected in abundances of enterobacteria and clostridia. Consequently, future studies investigating multiple fermentation time points are needed to substantiate the present observations.
Acknowledgements
The authors thank Rebecca Scherer for providing well-defined silage samples for this study and Helga Sauerwein for generously providing laboratory access.
Financial support
The work was funded by the ‘Deutsche Forschungsgemeinschaft’ (DFG, German Research Foundation, SU 124/31-1) and partly conducted in the Center of Integrated Dairy Research (CIDRe), University of Bonn (Bonn, Germany).
Conflict of interest
The authors declare there is no conflict of interest.
Ethical standards
Not applicable.