INTRODUCTION
The Mytilida (=Mytiloidea) Férussac, 1822 is currently considered to be representative of the bivalve suborder Pteriomorphia within which it is located as a monophyletic clade with the Arcida, Pectinida, Ostreida and Pinnida (Bieler et al., Reference Bieler, Mikkelsen, Collins, Glover, Gonźalez, Graf, Harper, Healey, Kawauchi, Sharma, Staubach, Strong, Taylor, Tëmkin, Zardus, Clark, Guzmán, McIntyre, Sharp and Giribet2014). Although this higher clade taxonomy is generally agreed upon, the lower order taxonomy of the Mytiloidea is confused.
Soot-Ryen (Reference Soot-Ryen and Moore1969) considered that the Mytilida, with a single superfamily – the Mytilacea (=Mytiloidea) Rafinesque, 1815 – was divided into two families: the extinct, Triassic, Mysidellidae Cox, 1964 and the extant Mytilidae. The latter, in turn, was divided into four subfamilies – the Mytilinae, Crenellinae Adams & Adams, 1857, Lithophaginae Adams & Adams, 1857 and Modiolinae Keen, 1958 (= Modiolidae [Termier & Termier, 1950]). Scarlato & Starobogatov (Reference Scarlato and Starobogatov1979a, Reference Scarlato and Starobogatovb) subsequently erected four mytiloid families (although this number was reduced to three later), that is, the Mytilidae, Crenellidae, Lithophagidae and Septiferidae Scarlato & Starobogatov, 1979 (Starobogatov, Reference Starobogatov1992). Boss (Reference Boss and Parker1982), however, recognized only a single family – the Mytilidae – that was divided into four subfamilies: the Mytilinae, Crenellinae, Lithophaginae and Modiolinae. Bernard (Reference Bernard1983) adopted this classification scheme in his catalogue of the Bivalvia of the eastern Pacific Ocean. Later, however, Amler (Reference Amler1999) reduced the number of Scarlato & Starobogatov's families to three: the Mytilidae, Septiferidae and the fossil Mysidiellidae. Coan et al. (Reference Coan, Valentich-Scott and Bernard2000), however, followed Boss (Reference Boss and Parker1982) and Bernard (Reference Bernard1983) and recognized only a single family – the Mytilidae – but considered this to comprise seven subfamilies: the Mytilinae, Bathymodiolinae Kenk & Wilson, 1985, Crenellinae, Dacrydiinae Ockelmann, 1983, Lithophaginae, Modiolinae and Septiniferae. Coan & Valentich-Scott (2012) also considered that the Mytilidae was composed of the same seven subfamilies.
Latter authors (Bieler & Mikkelsen, Reference Bieler and Mikkelsen2006) stepped away from convention and described the Mytiloidea as comprising five families, that is, the Mytilidae, Crenellidae, Musculidae Iredale, 1939, Modiolidae and Septiferidae but identified eight notional subfamilies: Lithophaginae (= Lithodominae J.E. Gray 1857), Adulinae Scarlato & Starobogatov, 1979, Botulinae Scarlato & Starobogatov, 1979, Brachidontinae Scarlato & Starobogatov, 1979, Limnoperninae Scarlato & Starobogatov, Dacrydiinae, Crenomytilini(ae) Kafanov, 1984 and Bathymodiolinae. Subsequently, however, Bieler et al. (Reference Bieler, Carter, Coan, Bouchet and Rocroi2010 in Bouchet & Rocroi, Reference Bouchet and Rocroi2010) reverted to a single family platform and considered that although the Mytilidae comprised eight subfamilies, the Adulinae and Brachidontinae were considered synonyms of the Mytilinae, along with the Perninae Scarlato & Starobogatov, 1979, Crenomytilini(ae) and the Xenomytilinae Squires & Saul, 2006. Similarly, the Botulinae was considered synonymous with the Lithophaginae. Three more of their earlier recognized familial taxa, that is, the Modiolidae, Septiferidae and Crenellidae, were reduced to subfamily status, with a fourth, the Musculidae, synonymized with the Crenellinae along with the Arcuatulinae Scarlato & Starobogatov, 1979 and Trichomyinae Scarlato & Starobogatov, 1979.
The nomenclatorial chaos described above is only a small selection of the many taxonomic classifications of the Mytiloidea dating back to the 18th century, including Linnaeus (Reference Linnaeus1758). The problem arises from the fact that few mytilid taxa have been described anatomically so that ascertained diagnostic differences between them mainly comprise shell features such that virtually all of the classification schemes described above are based on these characters. Only a few authors have examined mytiloid morphology and these have been identified by Morton (Reference Morton2015a). Notwithstanding, the most recent classifications of the Mytiloidea have arisen from the results of molecular studies.
The molecular results of Distel (Reference Distel2000), Distel et al. (Reference Distel, Baco, Chuang, Morrill, Cavanaugh and Smith2000), Steiner & Hammer (Reference Steiner, Hammer, Harper, Taylor and Crame2000) and Giribet & Wheeler (Reference Giribet and Wheeler2002) all suggest that the Mytiloidea constitutes a monophyletic clade, which shell morphology evidence broadly supports. Distel (Reference Distel2000) and Steiner & Hammer (Reference Steiner, Hammer, Harper, Taylor and Crame2000) suggested, however, that subfamilial relationships of the Mytiloidea need re-examination and to this end Morton (Reference Morton2015a) re-examined the pericardial-posterior byssal retractor muscle relationships of a large number of mytiloid taxa and concluded that the superfamily comprised four families – the Modiolidae, Mytilidae, Musculidae and Crenellidae – a view which supported the classification suggested by Bieler & Mikkelsen (Reference Bieler and Mikkelsen2006) albeit with the latter author's addition of the Septiferidae. Finally, in a classification including fossil taxa, Carter et al. (Reference Carter, Altaba, Anderson, Araujo, Biakov, Bogan, Campbell, Campbell, Chen, Cope, Delvene, Dijkstra, Fang, Gardner, Gavrilova, Goncharova, Harries, Hartman, Hautmann, Hoeh, Hylleberg, Jiang, Johnston, Kirkendale, Kleemann, Koppka, Kříž, Machado, Malchus, Márquez-Aliaga, Masse, McRoberts, Middelfart, Mitchell, Nevesskaja, Özer, Pojeta, Polubotko, Pons, Popov, Sánchez, Sartori, Scott, Signorelli, Silantiev, Skelton, Steuber, Waterhouse, Wingard and Yancey2011), recognized the extinct Modiolopsoidea P. Fischer, 1886 as separate from the Mytiloidea and suggested that the latter comprised three families and 10 subfamilies – the Mytilidae (with the Mytilinae, Arcuatulinae, Bathymodiolinae, Lithophaginae, Modiolinae and the extinct Xenomytilinae Squires and Saul, 2006), Crenellinae (with the Crenellinae and Musculinae) and Septiferidae (with the Septiferinae and Limnoperninae).
Regardless of this broad consensus, the fact remains that many mytiloid taxa remain unstudied anatomically (largely accounting for the above taxonomic farrago) and in no family is this truer than for the Crenellidae. Jeffreys (Reference Jeffreys1863) provided notes on the mantle, foot and ctenidial morphology of British species of the Mytiloidea, including Crenella decussata (Montagu, 1808). Pelseneer (Reference Pelseneer1911, plate IV, figure 5) also illustrated a species of Dacrydium (see below) from the Indian Ocean but with a shell length of ~15 mm and a full complement of muscles this identification may not be correct. The first modern study of crenellid anatomy was, however, by Soot-Ryen (Reference Soot-Ryen1955, figures 66 and 67). This author demonstrated the presence of a posterior adductor muscle, and highly reduced anterior adductor and anterior and posterior byssal retractor muscles in Solamen columbianum (Dall, 1897). The same author, however, suggested that the only, significant, muscle present in Crenella decussata was the posterior adductor (Soot-Ryen, Reference Soot-Ryen1955, figure 69). This is not true, however, as this study will show. Morton (Reference Morton2015a) has shown for Crenella ecuadoriana Pilsbry & Olsson, 1941 (Soot-Ryen, Reference Soot-Ryen1955) that there is a posterior adductor muscle, minute paired posterior byssal retractor muscles, but no anterior byssal retractor nor posterior pedal retractor muscles and a small anterior adductor muscle aligned thinly along the fused anterior mantle margins. Crenella ecuadoriana is currently synonymized with C. decussata s.s. due to indistinguishable adult shell features, but with the proviso that such taxa may comprise a species complex, that is C. decussata s.l. (Coan & Valentich-Scott, Reference Coan and Valentich-Scott2012, p. 126), a suggestion the present authors accept. Morton (Reference Morton2015a) identified this type of pericardial-posterior byssal retractor muscle complex as Category 4, with no posterior byssal retractor muscles, and as unique to the Crenellidae. A similar muscle arrangement was identified for Crenella decussata by Soot-Ryen (Reference Soot-Ryen1955, figure 69), the illustration albeit much simplified. It has also been shown and illustrated for Crenella sinica sp. nov. by Wang & Qi (Reference Wang and Qi1984, figure 23a). Solamen columbianum (Dall, 1897) also has reduced paired anterior byssal retractor muscles (Soot-Ryen, Reference Soot-Ryen1955, figure 67), while Wang & Qi (Reference Wang and Qi1984, figure 24.2) also illustrated how Solamen spectabilis (A. Adams, 1862) has a posterior adductor muscle and a small anterior adductor located in the antero-ventral mantle margin.
As noted earlier, it is believed that in addition to the Crenellinae, the Crenellidae comprises a second subfamily – the Dacrydiinae – first described by Ockelmann (Reference Ockelmann1983), but without reference to the internal anatomy. This situation was, however, rectified by Mattson & Warén (Reference Mattson and Warén1977) who described Dacrydium ockelmanni n. sp. as possessing anterior and posterior adductor muscles and both pairs of byssal retractor muscles. None of the above authors, however, provided information on any other aspects of the biology of the Crenelliidae studied by them.
In this paper, therefore, we (i) correct misconceptions about the anatomy of the circumboreal Crenella decussata; (ii) examine, from anatomical and experimental perspectives, a remarkable reproductive strategy and (iii) an unimagined life history trait unique within mytiloid bivalves. Specifically, this involves occupation of a granular crypt seen hitherto in only a few other, also highly specialized, bivalves, such as representatives of the Gastrochaenoidea (Morton, Reference Morton1983a) and the Clavagelloidea (Morton, Reference Morton2006), notably species of Dianadema (Morton, Reference Morton2003, Reference Morton2009) and with which comparisons will be made.
MATERIALS AND METHODS
Living adult individuals of Crenella decussata were collected from 10–12 m depth in waters south-east of the Hirsholmene in the northern Kattegat, Denmark. The substratum was well-sorted, yellow, sand-gravel with a grain size diameter of 0.4–2.0 mm. Individuals were maintained at the Helsingør Marine Biological Laboratory, University of Copenhagen, Denmark, in a 10°C culture room with a 12:12 h light:dark regime. The bivalves were reared in small lightly aerated aquaria with aged seawater at a salinity of 30 and a substratum of their natural sand-gravel environment. The culture was fed a daily algal mixture of Phaeodactylum, Dunaliella, Isochrysis and Monochrysis (5–10 ml of 50–60 million cells mL−1).
Regular examinations showed that the adult Crenella decussata remained alive and clusters of juveniles were retrieved from the granular crypts of five females after eight months in the aquaria. A few of these juveniles were observed for potential response(s) to adult pheromones. The remaining majority of individuals continued to be reared to increase stock numbers for experimental studies. Following on from the rearing programme, all adults were recovered from the sediment 10 days later. A single batch of 97 newly laid embryos and three undeveloped eggs were retrieved from one female (3.2 mm shell length) and, of 11 females, nine were each found to have an egg-mass allowing the retrieval of a total of ~300 embryos (and a few newly hatched juveniles). Subsequently, when all adults were recovered after 4 weeks, seven of the eleven females contained egg-masses, and three others had two egg-masses each. A total of 445 eggs and embryos or hatched young were retrieved, all of which had been produced within the intervening month. The developmental state of the embryos indicated that spawning had taken place on at least two occasions. Some of the embryos and juveniles were used subsequently for morphological, behavioural and growth studies.
Supplementary material used in the morphological and anatomical studies, comprising a few individuals stored in 70% ethanol, originated from the following locations: (i) eastern rim of Herthas Rev (Flak), 20 m, coarse sand and stones (leg. A.M. Christensen); (ii) south-east of Hjem, southern Kattegat, 13.4 m, coarse sand and stones; (iii) east of Fyns Hoved, Southern Kattegat (55°37.5′N 10°39.2′E), 16.5 m, stones and sand (leg. M. Jensen); (iv) the deep trench off Helsingborg, Øresund, Sweden, 36 m, coarse sand (leg. K.W. Ockelmann); and (v) Knæhagen, Øresund, Sweden, 27–30 m, sand, mud, shell debris and Modiolus modiolus reef (leg. K.W. Ockelmann).
Morphological studies
Subsequent to examination of the living individuals in the experiments to be described below, a few individuals of adult Crenella decussata were fixed in borax buffered 2% formaldehyde and preserved subsequently in 70% ethanol in seawater. Two individuals were removed from their crypts and, following routine histological procedures, sectioned transversely at 4 µm and the resulting slides stained in Ehrlich's haematoxylin and eosin. The shells of four further individuals were prepared for examination using scanning electron microscopy. These individuals were boiled lightly and the internal tissues removed before the shells were rinsed in ethanol, dried, placed on SEM-stubs layered with carbon tape and, subsequently, sputter-coated with gold for 4.5–5.0 min and examined in a scanning electron microscope (MAXIM 2040, CamScan Electron Optics Ltd, Cambridge, UK) equipped with a tungsten filament system at the Institute of Physical Science, University of Aarhus, Denmark.
Experimental studies
JUVENILE RESPONSES TO ADULT PHEROMONES
After being obtained from maternal tubes, larger juveniles were active using their foot to creep around their experimental containers. Later, they either attached themselves by a single byssal thread or remained motionless on the bottom of the bowl. Containers with juveniles were kept on orbital shakers at 100 rpm. Some of these juveniles (220 µm shell length) were exposed to the exhalant water of adult individuals. They responded by becoming active, stretching their foot outwards, creeping and undertaking searching movements, but no response to current direction was observed.
Observations upon potential response of juveniles to adult pheromone(s) were made with some of the juveniles one month after they had hatched. Thirty-nine juveniles were placed in a 50 mL glass beaker with aged seawater (temperature 10°C, salinity 30). Water from a bowl containing adults was pipetted into the beaker with the juveniles and their behaviour observed for 20 min. Most individuals became active within a few minutes after adult exhalant water was added but soon stopped.
Based on these initial observations, a hypothesis was formed that: (i) young individuals hatch from an egg mass deposited on the wall of a maternal exhalant tube (as will be described) and, after hatching, juveniles, that is, early post-metamorphosed juveniles, attach byssally to a specialized region of the inner lining of the tube; (ii) early juveniles remain in this region of the tube for some time while further differentiation proceeds and until they can suspension-feed independently, and that this juvenile behaviour is based upon and secured by a positive response to a pheromonal factor derived from the adults (probably the females); and (iii) at a later stage, the juvenile loses the response and becomes independent, that is, it enters a free-living phase. This may coincide (iv) with the end of the nepioconch and beginning of the dissoconch stages in shell growth. At this stage, young individuals obtain an adult morphology and acquire the burrowing and tube-building habits of their parents.
To test the above hypothesis, an experiment was set up in small vials with round bottoms at 45° inclinations each with a batch of newly hatched juveniles that were kept un-fed to avoid extraneous contamination. Two vials with aged seawater (temperature 10°C, salinity 30) received 25 individuals each and were kept at the same culture regime throughout the experiment. One vial was designated as the control while the other was exposed to adult exhalant water. Exposures to either aged seawater (control) or adult exhalant water (experiment) were carried out at 12, 17, 26 and 54 days post-hatching with regular observations of individual juvenile behaviour being carried out for 15–25 min.
JUVENILE GROWTH AND FEEDING
A batch of offspring were separated from the rearing chambers and kept in a small container with fine sand (grain size ≤160 µm). Subsequently, the embryos were sorted into separate groups. One group of 43 individuals was reared further without food. Another group of 100 individuals was fed daily on an algal mixture of Phaeodactylum, Dunaliella, Isochrysis and Monochrysis (2–5 ml of 50–60 million cells ml−1). Sub-samples of the embryos and later juveniles were measured at ~2-week intervals for 3 months (starved) and 7.5 months (fed).
RESULTS
Biology and lifestyle
Adult individuals of Crenella decussata form an adventitious crypt in the yellow sand-gravel of the fine shell-sand substratum they occur in. The crypts are made of the habitat's sand grains bound together with mucus. Figure 1 shows the crypts of three individuals all of which have a slightly bulbous anterior, enclosing the individual that constructed it and which divides posteriorly into two smaller arms that represent the positions of the inhalant and exhalant currents, as will be described. The white dots also seen in Figure 1 are released ova and embryonic larvae, again as will be described. The two tubes protrude upwards to the surface of the sediment some 5–10 mm above the bulbous crypt. Both tubes are lined internally with a light yellow sheet of mucus.

Fig. 1. Crenella decussata. The adventitious crypt made of sand grains of three individuals. The white dots are released fertilized ova and larvae.
Fully developed embryos, that is, late larvae, obtained from the females’ crypts were small, 185–195 µm, and globose with no nepioconch growth visible as yet and, thus, appeared to have not yet hatched and metamorphosed. In the exhalant tube, as will be described, of one females’ (shell length 3.1 mm) crypt, 89 juveniles were clustered and byssally attached to its wall in the region where the lining was notably thicker. The shells of newly hatched individuals, that is with a fully developed prodissoconch (I and II), were somewhat convex and measured ~220 µm, and with initial nepioconch growth just visible along the shell margin. A few juveniles were larger, up to 260 µm, which indicated that the females had released fertilized eggs and/or early embryos on at least three separate occasions.
A second cluster of newly laid spherical ova attached inside a female's exhalant tube measured 135 µm in diameter. Fertilization was inferred to have occurred with initial development taking place in the mantle cavity before the embryos were released into the tube as early embryos. The 97 counted embryos in the tube were embedded in a transparent, colourless, mucus, the whole egg-mass adopting the form of the maternal tube with a central ridge where it had been most firmly attached.
The shell
THE LARVAL SHELL – PRODISSOCONCHS I AND II
The larval shell of Crenella decussata at various, sequential, stages of development is illustrated in Figure 2(A–D), these all being shown from the right side to facilitate comparison. Mature eggs of Crenella decussata measure between 135–150 µm with fertilization occurring within the supra-branchial chamber of the female and where, as will be described, development proceeds to be followed by a phase of incubation within the infra-branchial chamber of the mantle cavity. The prodissoconch (I + II) (Figure 2A and B) is clearly differentiated and measures up to 185–195 µm in diameter. The embryo and early larval shell stage are both globular – their shape reflecting that of the ovum. The relatively large egg size and no clear delineation of the prodissoconchs I and II shell also confirms lecithotrophic development (Ockelmann, Reference Ockelmann1965). The surface of the prodissoconch shell is slightly nodular initially and then becomes smoother (Figures 2A, B and 3A, B).

Fig. 2. Crenella decussata. Light microscopy photographs of the larval shell. (A), prodissoconch I; (B), prodissoconch (I + II, not delineated); the juvenile shell, (C), nepioconch; and the adult shell, (D), dissoconch. All shown from the right side.

Fig. 3. Crenella decussata. SEM photomicrographs of (A), an internal view of the dissoconch left valve, and (B), a more detailed hinge. (For a list of abbreviations see Box.)
THE POST-METAMORPHIC NEPIOCONCH
The early post-metamorphic shell of Crenella decussata, the nepioconch, has a smooth margin (Figure 2C). Nepioconch (Figure 2C) size varied between 745–770 µm and is probably dependent on the extent of endogenous nourishment, that is, the yolk, available to each individual, as well as the external nourishment available to it in its maternal parents’ granular crypt at this early stage of development (Figure 2B, C), as will be discussed. Jørgensen (Reference Jørgensen and Thorson1946) reported individuals, with shell lengths of 750 µm, of this species from the Sound between Denmark and Sweden to have outlines similar to that of the adult, and further noted that veligers of this size had not hitherto been recorded from these waters. This observation confirms that Jorgensen was, thus, describing nepioconchs rather than prodissoconchs. The surface of the nepioconch is characterized by fine radiating striations and equally fine commarginal growth lines although the valve margins remain relatively smooth.
Abbreviations used in the figures: AA, Anterior adductor muscle; ABR, Anterior byssal retractor muscle, ADT, Anterior dissoconch teeth; AFID, Anteriormost filament of the inner demibranch; AFOD, Anteriormost filament of the outer demibranch; AN, Anus; AU, Auricle of heart; BC, Blood cells; BG, Byssal groove; BGL, Byssal gland; BOD, Base of inner demibranch; BSC, Basiphilic secretory cells; C, Crenulated shell valve margin; CA, Ctenidial axis; CR, Chitinous rod; CS, Crystalline style; CSMG, Conjoined crystalline style sac and mid gut; CSS, Crystalline style sac; D, Dissoconch; DD, Digestive diverticulae; DIPC, Dorsal infra-pallial chamber; DK, Distal limb of the kidney; DO, Developing oocyte; DOG, Distal oral groove; ELFC, Eulatero-frontal cilia; ES, Exhalant siphon; ESC, Eosinophilic secretory cell; F, Foot; FC, Frontal cilia; FGID, Food groove of the inner demibranch; FGOD, Food groove of the outer demibranch; FO, Fertilized ovum; FP, Fused periostracum; GA, Gonadal aperture; H, Heart; HF, Heel of the foot; HG, Hind gut; IA, Inhalant aperture; ID, Inner demibranch; ILL, Inner ligament layer; IMF, Inner mantle fold; K, Kidney; L, Ligament; LAT, Left anterior testis follicle; LC, Lateral cilia; LPT, Left posterior testis follicle; M, Mouth; MA, Mantle aperture; MG, Mid gut; MM, Mantle margin; MMF, Middle mantle fold; MO, Mature oocyte; N, Nepioconch; O, Oesophagus; OD, Outer demibranch; OG, Osphradial gland; OMF, Outer mantle fold; OT, Ovarian tubule; OV, Ovary; P, Periostracum; PA, Posterior adductor muscle; PALOD, Point of attachment of the ascending lamella of the outer demibranch to the mantle; PBR, Posterior byssal retractor muscle; PC, Pallial crest; PDT, Posterior dissoconch teeth; PE, Pericardium; PEDG, Pedal ganglia; PEG, Periostracal groove; PG, Pedal gape; PGL, Pericardial gland; POG, Proximal oral groove; POLL, Posterior outer ligament layer; PR, Prodissoconch; PRM, Pallial retractor muscle; R, Rectum; RA, Renal aperture; RAT, Right anterior testis follicle; RPT, Right posterior testis follicle; SC, Secretory cells; SH, Shell; SHN, Shell nymph; SP, Spermatozoa; SPO, Spermatogonia; SPT, Spermatid; ST, Stomach; T, Testes; TC, Transverse connective; V, Ventricle of the heart; VLP, Vestigial labial palp; VM, Visceral mass; VMFG, Ventral marginal food groove.
THE DISSOCONCH – THE SHELL OF THE ADULT
The dissoconch (Figure 2D) is the final shell stage and becomes the shell of the adult Crenella decussata individual. Its maximum length ranged between 3.0–5.5 mm. Dissoconch shell surface sculpture comprised nodular radiating ribs overlain by clear commarginal growth lines thereby creating a reticulated impression. The radiating ribs terminate at each valve's margin resulting in a crenulated, lightly interlocking, rim, as described in more detail by Tebble (Reference Tebble1966).
Mytiloid hinge teeth formation has been described for some species inferring the ancestral state to be one tooth series on either side of the primary ligament in the near-linear hinge of prodissoconch I, with a second series of larger, iterated, teeth in prodissoconch II, and with a third and fourth taxodont teeth series anteriorly and posteriorly of the secondary ligament of the nepioconch and dissoconch stage (Ockelmann, Reference Ockelmann1995). Hinge teeth formation in Crenella decussata deviates from this pattern in that prodissoconch II is greatly reduced and not clearly delineated from prodissoconch I, which is formed by the embryonic shell gland. Effectively, therefore, there are only three shell stages, the larval prodissoconchs (I and II) and the post-metamorphic nepioconch and dissoconch. Each of these three shell stages has a series of anterior and posterior taxodont teeth, which iteratively increase in size with each shell stage (Figure 3A). Figure 3B is another, higher magnification SEM photograph of the umbonal region of the adult, dissoconch, shell showing the initially slightly nodular but subsequently smooth prodissoconch I and II and the progressively reticulated patterning of the late nepioconch and the dissoconch. Similarly, the SEM photomicrographs of larval and early post-metamorphic shell stages of Crenella decussata from Vostok Bay (Sea of Japan) provided by Semenikhina et al. (Reference Semenikhina, Kolotukhina and Evseev2008) and Kolotukhina et al. (Reference Kolotukhina, Kulikova and Evseev2011) showed only three clearly delineated shell stages, that is, a prodissoconch, and nepioconch and a dissoconch, and with a surface patterning similar to our findings. These authors also believed that in Vostok Bay, C. decussata had a short pelagic stage prior to metamorphosis, although this could not be verified by their employed sampling strategy. Several arcoid and mytilid species with planktotrophic development obtain a pair of ctenidial ocelli during their larval lives and which are used during the settlement phase (Dinesen & Ockelmann, unpublished information). Our observation that such ocelli are absent in C. decussata further supports the conclusion that a planktonic stage is lacking in this species.
THE ADULT SHELL
The adult shell of Crenella decussata is relatively small in comparison with most other mytiloids, generally being no longer antero-posteriorly than 3–5 mm (Figure 4A). The shell is delicately brittle, equivalve, rotund and distinctively inequilateral. That is, the posterior margin is enlarged and rounded whereas the anterior shell margin is less rounded and projects only slightly anteriorly beyond the umbones. The antero- and postero-dorsal shell margins are steep (the former slightly curved) and meet at a mid-dorsal, prosogyrous, apex. The shell is characterized by an external patterning of up to some 50 radiating ribs crossed by ≥40 commarginal growth lines creating a decussate surface. The delicate covering of periostracum is a light yellow-brown. From the posterior aspect (Figure 4B), the shell is laterally swollen, almost plump, mid dorso-ventrally (x------y, greatest shell width) and the valve margins interlock at a crenulated margin with no byssal notch or other marginal gape.

Fig. 4. Crenella decussata. The adult shell as seen from (A), the right side and (B), the posterior aspect. (x------y = greatest shell width).
The hinge areas of the right (Figure 5A) and left (Figure 5B) shell valves of Crenella decussata are characterized by an internal opisthodetic ligament (L) situated on shallow nymphs and located beneath the postero-dorsal margin of the shell. The ligament commences beneath the prodissoconch (PR) and posterior to a series of internal anterior dissoconch teeth (ADT) whose numbers increase with age and shell size. Above and continuing posteriorly to the ligament are a series of smaller and more numerous posterior taxodont teeth (PDT) that also increase in number and size with age. In transverse section (Figure 5C), the ligament is seen to be situated between and arch beneath and attach to the left and right shallow dorsal shell nymphs. The posterior outer ligament layer (POLL), there being no anterior equivalent, is situated between the nymphs whereas the long opisthodetic inner ligament layer (ILL) is located beneath them. The periostracum (P) that covers the shell is formed into a darkened pad of ‘fused’ periostracum (FP) above the posterior outer ligament layer. ‘Fused’ because although Owen et al. (Reference Owen, Trueman and Yonge1953) coined the term ‘fusion layer’ for the thickened pad covering the primary ligament, Yonge (Reference Yonge1978) clarified its periostracal nature. Clearly, however, since periostracum must cover the entire shell, there is no fusion, although the term is herein retained simply to emphasize that it is present in all other bivalves.

Fig. 5. Crenella decussata. The hinge area of (A), the right and (B), the left valves. (C), The ligament, as seen in transverse section. (For a list of abbreviations see Box.)
THE MANTLE
Figure 6 is an illustration of a living Crenella decussata seen from the posterior aspect and redrawn after Jeffreys (Reference Jeffreys1863), with the foot (F) fully extended. The structure of the foot, including the byssal gland and groove (BG) will be described later. Jeffreys’ figure shows, however, in addition to the relatively enormous size of the foot, that there is a small exhalant siphon (ES) separate from an inhalant aperture (IA) that is congruent with the pedal gape (PG) from which the foot can be, obviously dramatically, extended.

Fig. 6. Crenella decussata. An individual seen from the posterior aspect with the foot fully extended. Redrawn after Jeffreys (Reference Jeffreys1863). (For a list of abbreviations see Box.)
The posterior end of the left shell valve and mantle lobe and the inner and outer demibranchs (ID, OD) of the right ctenidium, of Crenella decussata are illustrated in Figure 7. The illustration shows the postulated directions of the exhalant (open arrow) and inhalant (black arrows) streams (the latter congruent with the pedal gape [PG]) and how, as in the typical mytiloid, pseudo-faeces are ejected via the former rather than the latter at the point of fusion separating exhalant from inhalant flows (small arrows) as originally described for Limnoperna fortunei (Dunker, 1857) by Morton (Reference Morton1973a). This current on both mantle lobes is created by cilia on the inner mantle folds (IMF).

Fig. 7. Crenella decussata. The posterior end of the left shell valve and mantle lobe showing the inhalant (black arrows) and exhalant (open arrow) streams and how in the typical mytiloid, pseudo-faeces are ejected from the dorsal edge of the former beneath that of the latter (For a list of abbreviations see Box.)
The left ventral mantle margin of Crenella decussata is shown in transverse section in Figure 8. As described above, the mantle margins are united only between the inhalant aperture and exhalant siphon. In mid-ventral section, therefore, the left mantle margin comprises the usual three folds, inner (IMF), middle (MMF) and outer (OMF) (Yonge, Reference Yonge1957, Reference Yonge1982). The latter two are, however, small and uncomplicated and between them is the long periostracal groove (PEG) from the base of which arises the periostracum (P). The inner mantle fold is much larger, swollen, and contains eosinophilic sub-epithelial secretory cells. The pallial retractor muscle (PRM) is also small. Where the inner fold joins the general internal mantle epithelium is a poorly defined ciliated rejection tract that is responsible for transporting unwanted filtered particles posteriorly where they are rejected from the mantle cavity via the inhalant aperture as pseudo-faeces (see above). Secretions from the inner fold glands may assist in binding these unwanted particles together into the pseudo-faecal string. The gland may also have some significance with regard to larval brooding, as will be described.

Fig. 8. Crenella decussata. A transverse section through the left ventral mantle margin. (For a list of abbreviations see Box.)
THE ORGANS OF THE MANTLE CAVITY
The organs of the mantle cavity of Crenella decussata, as seen from the right side after removal of the right shell valve and most of the right mantle lobe, are shown in Figure 9. As with Figure 7, inhalant and exhalant streams are illustrated by black and open arrows, respectively. The exhalant siphon of C. decussata (Figure 9) is transparent white and comprises a low, unadorned, cone. It is situated posterior to the posterior adductor muscle (PA). The long conjoined inhalant aperture and pedal gape is similarly lacking in pigmentation and is marginally apapillate everywhere. The fusions that create the inhalant/pedal aperture and exhalant siphon comprise the left and right inner mantle folds only, that is, Type A (Yonge, Reference Yonge1957, Reference Yonge1982).

Fig. 9. Crenella decussata. The organs of the mantle cavity as seen from the right side after removal of the right shell valve and most of the right mantle lobe. Inhalant and exhalant streams are illustrated by black and open arrows, respectively. (For a list of abbreviations see Box.)
The ctenidia, comprising a complete inner demibranch (ID) and an anteriorly steeply foreshortened outer demibranch (OD) divide the mantle cavity into two chambers, supra-branchial and infra-branchial. The significance of this with regard to larval brooding is described below.
The foot (F) of Crenella decussata is also illustrated in Figure 9 and shows a swollen region occupied by the byssal gland (BGL) and how the posterior end of the foot is formed into a small pointed ‘heel’ (HF) although as seen in Figure 6, the foot's anterior ‘toe’ can be extended enormously. Figure 9 also illustrates the general disposition of the large posterior byssal retractor muscle (PBR) and small anterior adductor muscle (AA) located antero-ventrally and equally small paired anterior byssal retractor muscles (ABR) arising just internal to the anterior hinge teeth illustrated in Figure 5. Similarly, the pericardium (PE) and contained heart (H) is situated above the ctenidia and between the paired posterior byssal retractor muscles and the digestive diverticulae (DD) of the visceral mass.
Returning to the ctenidia of Crenella decussata, however, both demibranchs are made up of simple filaments and one of these is illustrated in transverse section in Figure 10. The ciliation is of the typical mytilid form described in detail by Atkins (Reference Atkins1937a, Reference Atkinsb) with short (20 µm) frontal cilia (FC), the inhalant current-creating lateral cilia (LC, 25 µm) and filtering eulatero-frontal cilia (ELFC, ~35 µm). The anterior and posterior sides of the filaments are supported by chitinous rods (CR) in turn cross-united by transverse connectives (TC). Unusually, and so far unique for any mytiloid, the bases of each filament are composed of large (~20 µm) eosinophilic secretory cells. Such basal secretory cells have been described for a number of ctenidial brooding bivalves (Morton, Reference Morton1977) and their significance will be discussed.

Fig. 10. Crenella decussata. A transverse section through a single ctenidial filament. (For a list of abbreviations see Box.)
The anterior terminus of the right ctenidium of Crenella decussata (Figure 11) is in many ways typical of the other representatives of the Mytiloidea. That is, the outer demibranch (OD) is anteriorly foreshortened and the terminal filament (AFOD) is somewhat larger than its posterior neighbours, especially ventrally. In the case of C. decussata, however, this anterior foreshortening is more exaggerated than in other mytiloids (e.g. Modiolus modiolus (Linnaeus, Reference Linnaeus1758) (Dinesen & Morton, Reference Dinesen and Morton2014, figure 10). The foreshortening of the demibranch may also be responsible for the loss of cephalic eyes in C. decussata – a feature of the dorsal edge of the anterior-most filaments of both outer demibranchs of many mytiloids, and as seen, for example, in M. modiolus (Dinesen & Morton, Reference Dinesen and Morton2014, figure 10). The anterior-most filaments of the inner demibranchs (AFID) are, however, of normal proportions.

Fig. 11. Crenella decussata. The anterior terminus of the right ctenidium. (For a list of abbreviations see Box.)
Orally directed ciliary currents are essentially the same as in other mytiloids (Atkins, Reference Atkins1937a, Reference Atkinsb), so that there are ventral marginal food grooves (VMFG) on both demibranchs, in the ctenidial axis (CA) and in the junctions between the descending lamellae of the inner and outer demibranchs (FGID, FGOD) with the visceral mass and mantle, respectively. The ctenidia thus have a ciliation of type B(I) (Atkins, Reference Atkins1937a). As a consequence of the foreshortening of the outer demibranchs, however, only the anterior-most tips of the filaments of the inner demibranch (ID) and dorsal food grooves described above feed directly into the mouth (M). The labial palps of all mytiloids hitherto described effect the transfer of potential food particles collected on the filaments of the ctenidial demibranchs to the mouth after sorting upon their ridged inner surfaces. This is ctenidial-labial palp junction type 1 (Stasek, Reference Stasek1963). Crenella decussata conforms to this type with one distinctive and unique (for the Mytiloidea) exception. That is, the outer palps are absent and the inner pair is reduced, on both sides left and right, to miniscule vestiges (VLP). Effectively, therefore, there is no potential at the mouth for any sorting of the possible food particles collected by the ctenidia, nor for the rejection of any unwanted particles as is the case for virtually all bivalves save for predatory taxa (Morton, Reference Morton, Wilbur and Saleuddin1983b).
Figure 12 is a transverse section through the interior of the mouth, that is, the beginning of the oesophagus (O) of Crenella decussata showing the single pair of vestigial labial palps (VLP) located latero-ventrally to it. Internal to each palp vestige is the ciliated proximal oral groove (POG), while dorsal to them is the similarly ciliated distal oral groove (DOG). The palps therefore represent the inner pair so that it is the outer pair, which has been lost. The reasons for the loss of this will be discussed.

Fig. 12. Crenella decussata. A transverse section through the mouth showing the single pair of vestigial, lateral labial palps. (For a list of abbreviations see Box.)
THE VISCERAL MASS
The musculature of the foot and the visceral mass of Crenella decussata is illustrated in Figure 13 from A, the right side, after removal of the right shell valve, mantle lobe and ctenidium, and B, the anterior aspect. Firstly, this illustration represents the contracted state of the foot because, as illustrated by Jeffreys (Reference Jeffreys1863) and shown in Figure 6, the foot can in life be extended an enormous distance.

Fig. 13. Crenella decussata. The musculature and foot of the visceral mass as seen from (A), the right side (after removal of the right shell valve, mantle lobe and ctenidium) and (B), the anterior aspect. (For a list of abbreviations see Box.)
The visceral mass is formed posteriorly into a point, which represents the heel of the foot (HF). There is a large posterior adductor muscle (PA), internal to which is a single pair of posterior byssal retractor muscles (PBR) but no posterior pedal retractor muscle, as seen in other mytiloids. There is also a pair of thin anterior byssal retractor muscles (ABR) with the anterior adductor muscle (AA), as noted earlier, represented by a thin expansion of the antero-ventral mantle margin. Seen from the anterior aspect, the base of the foot (F) contains paired, left and right, byssal glands (BGL) and there is a minute medial byssal groove (BG). The thin anterior byssal retractor muscles (ABR) extend into visceral mass (VM) and situated below them are paired pedal ganglia (PEDG).
The digestive organs of the visceral mass of Crenella decussata, as seen from the right side after removal of the right shell valve, mantle lobe and ctenidium, are illustrated in Figure 14. The stomach is located anteriorly, unseen and surrounded by the dark digestive diverticulae (DD). From the posterior margin of the stomach arises the conjoined style sac and mid gut (CSMG). This passes under the pericardium (PE) containing the heart (H). In other mytiloids, the conjoined style sac and mid gut and the terminally separated mid gut pass forwards between large and multiple-blocks of paired byssal retractor muscles (Purchon, Reference Purchon1957). This situation is represented by lightly stippled areas in Figure 14 and is described in detail for other mytiloids by Morton (Reference Morton2015a). This author also suggested that such a musculature has, presumably, been lost in C. ecuadoriana and in C. decussata s.s.

Fig. 14. Crenella decussata. The digestive organs of the visceral mass as seen from the right side after removal of the right shell valve, mantle lobe and ctenidium. Lightly stippled areas represent the musculature seen in other mytiloids but, presumably, lost in C. decussata. (For a list of abbreviations see Box.)
The separated mid gut (MG) of Crenella decussata loops over the digestive diverticulae on the right side of the body and then passes upwards and posteriorly again to become the hind gut (HG) and then the rectum (R) which penetrates the ventricle of the heart, as in all mytiloids, passes between the reduced and paired posterior byssal retractor muscles, over the posterior adductor muscle (PA) where it terminates on the posterior face of this muscle in an anus (AN).
The relationship of the visceral mass to the rest of the body of Crenella decussata is shown in Figure 15. The shell (SH) is shown in transverse section with the two valves linked by the internal ligament (L) dorsally and the narrow anterior adductor muscle (AA) ventrally and within the fused ventral mantle margin (MM). The periostracum (PE) covers the shell. In this the anterior region of the visceral mass, the oesophagus (O) is surrounded by the digestive diverticulae (DD) and there are tiny anterior byssal retractor muscles (ABR) lying directly above the secretory cells (SC) of the byssal gland.

Fig. 15. Crenella decussata. A transverse section through the shell, fused ventral mantle margin and anterior region of the visceral mass. (For a list of abbreviations see Box.)
The byssal gland complex of Crenella decussata is shown in Figure 16. The byssal gland (BGL) comprises left and right bulbous bases to the foot (F). The paired glands each comprise two components: a large cluster of eosinophilic cells (ESC) and, surrounding the byssal groove (BG), smaller groups of basophilic cells (BSC). Within the visceral mass (VM) above the glands are the paired pedal ganglia (PEDG) and the posterior byssal retractor muscles (PBR), which are just as small as their anterior counterparts (Figure 15, ABR).

Fig. 16. Crenella decussata. A transverse section through the foot in the region of its swollen glandular area and the pedal ganglia. (For a list of abbreviations see Box.)
The size of the anterior adductor muscle of Crenella decussata is compared with the posterior equivalent (Figure 17, PA). A transverse section through this muscle is illustrated with the rectum (R) located above it within the dorsal infra-pallial chamber (DIPC) created by the fusion of left and right mantle margins here resulting in a pallial crest (PC) although the left and right periostracal grooves remain separated each secreting its periostracal film (P). The epithelium beneath the posterior adductor muscle, that is, the dorsal lining to the supra-branchial chamber comprises tall (250 µm) secretory cells and these constitute the osphradial gland (OG).

Fig. 17. Crenella decussata. A transverse section through the posterior adductor muscle with the rectum situated above it. (For a list of abbreviations see Box.)
THE PERICARDIUM
The organs of the pericardium of Crenella decussata, as seen from the right side, are shown in Figure 18. The pericardium is located anterior to the posterior byssal retractor muscle (PBR) and contains the heart (H) the ventricle of which is penetrated by the rectum. Between pericardium and the byssal retractor muscle is a pair of kidneys (K). As will be shown in Figure 19, the left and right auricles of the heart contain the light brown pericardial gland. The left and right renal apertures (RA), which are separate from but adjacent to the gonadal apertures (GA), open from the visceral mass into that component of the supra-branchial chamber contained within the cavity created by the ctenidial axis (CA) and the point of attachment of the ascending lamella of the outer demibranch (PALOD) to the dorsal mantle. Because the outer demibranch (OD) has been extensively foreshortened anteriorly, however, the gonadal apertures open directly into the infra-branchial chamber. As a consequence, mature and fertilized ova (FO) are brooded in the mantle cavity in the left and right spaces vacated by the anteriorly foreshortened outer demibranchs and adjacent to the filaments of the descending lamellae of the left and right inner demibranchs (ID) – both possessing ventral marginal food grooves (VMFG).

Fig. 18. Crenella decussata. The organs of the pericardium as seen from the right side also showing mature ova being brooded in the mantle cavity. Some of the anteriormost filaments of the outer demibranch have been removed for clarity. (For a list of abbreviations see Box.)

Fig. 19. Crenella decussata. A transverse section through the heart with, below it, the separated mid gut. (For a list of abbreviations see Box.)
The pericardium (PE) of Crenella decussata is shown in transverse in Figure 19. Also shown in transverse sections are: the heart with the rectum (R) penetrating the ventricle (V), the lateral auricles each filled with blood cells (BC) and the pericardial glands (PGL) integral with the epithelium of the auricles. Below the pericardium is the mid gut (MG), the lateral distal limbs of the kidneys (DK) and elements of the posterior byssal retractor muscles (PBR). The roof of the supra-branchial chamber is lined with the osphradial gland (OG). In this sectioned individual the ovaries (OV) are located beneath the left and right mantle lobes.
A more anteriorly transverse section through the pericardium of Crenella decussata (Figure 20) shows the rectum (R) within the heart's ventricle (V) and left and right auricles (AU). Below it, is the visceral mass showing the conjoined crystalline style sac and mid gut (CSMG) with its crystalline style (CS), the separated mid gut (MG) and elements of the digestive diverticulae (DD). The section also shows two lobes of the ovarian tubules (OV) occupying the most dorsal regions of this component of the visceral mass.

Fig. 20. Crenella decussata. An anterior transverse section through the heart showing the separated crystalline style sac and mid gut and the ovarian tubules occupying the dorsal regions of this component of the visceral mass. (For a list of abbreviations see Box.)
THE REPRODUCTIVE SYSTEM
Unlike in Mytilus edulis Linnaeus, Reference Linnaeus1758 (White, Reference White1937), the gonadal tissues of Crenella decussata never invade the mantle, the ctenidia nor the foot. Rather, as shown above, the ovarian follicles of C. decussata are located in the dorsal region only of the visceral mass. Crenella decussata is dioecious and, as with the ovaries, the testes are also located in mesosomal lobes in the dorsal visceral mass (Figure 21, T). Posterior to the pericardium, a transverse section through the visceral mass of C. decussata shows the rectum (R) situated above the posterior adductor muscle (PA) and with the posterior byssal retractor muscles (PBR) penetrating it and passing between elements of the distal kidneys (DK). In this position too are elements of the testes (T) situated atop the digestive diverticulae (DD).

Fig. 21. Crenella decussata. A transverse section through the visceral mass posterior to the heart showing the dorsal position of the testicular follicles. (For a list of abbreviations see Box.)
This situation is more clearly illustrated in Figure 22 where a horizontal section through the stomach (ST), crystalline style sac (CSS), with its style (CS) and separated mid gut (MG) of Crenella decussata shows how the testicular follicles are organized into four lobes when seen from the dorsal aspect: left anterior (LAT), left posterior (LPT), right anterior (RAT) and right posterior (RPT) – all inter-mingling with dorsal elements of the digestive diverticulae (DD). The same arrangement also holds true for the ovarian tubules.

Fig. 22. Crenella decussata. A horizontal section through the stomach showing how the testicular follicles are organized into four lobes when seen from the dorsal aspect. (For a list of abbreviations see Box.)
Detailed transverse sections through a single testicular follicle (Figure 23A) and a single ovarian tubule (Figure 23B) of different male and female individuals of Crenella decussata are illustrated. In the former, are seen spermatozoa at various stages of maturity. Among the germ cells there are Sertoli cells. These rest on the basement membrane of the germinal epithelium that lines each of the testicular follicles and extends toward their lumina, coming into contact with spermatogonia (SPO), spermatids (SPT) and with spermatocytes (SP), also filling each follicle lumen. In the latter, developing oocytes (DO) at various stages of maturation, line the walls of each ovarian tubule (OT) with, in this individual, a single mature oocyte (MO) ready for release. Each mature oocyte contains a nucleus ~50 µm in diameter, with a distinctive nucleolus, and is surrounded by large accumulations of yolk.

Fig. 23. Crenella decussata. Transverse sections through (A), a single testicular follicle with spermatozoa at various stages of maturity and (B), a single ovarian tubule also with oocytes at various stages of maturation. (For a list of abbreviations see Box.)
ONTOGENY
Mature oocytes of Crenella decussata measure between 135–150 µm and fertilization is believed to occur within the mantle cavity of the female, where further development of fertilized ova also takes place. This is illustrated in Figure 18 (FO) where developing larvae are being brooded in the expanded infra-branchial chamber created by the great anterior foreshortening of the outer demibranchs and loss of the outer labial palps and reduction to vestigial status of the inner ones. In the developing embryo, the prodissoconch (I) eventually becomes distinguishable, and measures up to 185–195 µm in diameter (Figure 2A–D).
This study, however, shows that larval brooding continues after the prodissoconch stage larvae have been released from the mantle cavity. Figure 24A illustrates a female individual of Crenella decussata situated within the basal swollen region of her sand grain crypt. The opened exhalant component of the crypt revealed the fertilized and secondarily brooded embryos (Figure 24B). The smallest of these (shell diameter ~190 µm) were prodissoconch larvae but most were post-metamorphosed nepioconch stages and were byssally attached, typically by a single fine thread, to the mucoid inner surface of this arm of the crypt.

Fig. 24. Crenella decussata. (A) An individual occupying its sand grain crypt and (B) the opened exhalant component of the crypt showing the brooded fertilized ova and embryos. (For a list of abbreviations see Box.)
The foot and, thus, the byssal glands of these individuals of Crenella decussata were functional at this time. In the largest larval (220 µm) individuals, primordial anterior and posterior adductor muscles were present, the foot was long and slender when extended and tipped with a cluster of conspicuous stiff cirri. These cirri probably serve a sensory function. Each demibranch of the nepioconch has two ctenidial filaments, which are not fused ventrally so that there are no ostia and, thus, no continuous ventral marginal food groove. The cilia of the ctenidial rudiments do, however, beat vigorously. There are no ctenidial eyespots, otherwise typical of many mytiloid larva and adults, for example Mytilus edulis (White, Reference White1937), Musculus (formerly Modiolarca) subpictus (Cantraine, 1835) (Morton & Dinesen, Reference Morton and Dinesen2011) (Modiolarca Gray, 1847 was recently synonymized with Gaimardia Gould, 1852, see Gofas (2015) in MolluscaBase accessed via http://www.marinespecies.org) and Modiolus modiolus (Dinesen & Morton, Reference Dinesen and Morton2014, figure 10), possibly because of the ontogenetic anterior foreshortening of the outer demibranch on which they would typically be situated. The remains of yolk-reserves are still present at this time. Eventually, such juvenile individuals are released from their maternal crypts.
Experimental studies
JUVENILE RESPONSES TO ADULT PHEROMONES
Initially after being washed out from the maternal crypt tubes, the smallest juveniles of Crenella decussata remained relatively immobile. Larger juveniles, however, became active, creeping around in their container. Later, they attached by a fine byssal thread or remained lying on the bottom of the bowl. In the embryos and early juveniles (shell length 185–195 µm) retrieved from egg-masses in late summer, a vestigial foot could be seen and some possessed a 1st ciliated ctenidial filament. After about one week, gill formation had proceeded and some individuals seemed able to produce a byssus and move the foot (but not creep). After another week, most juveniles had attached themselves by either one or a few byssal threads to one or two sand grains (grain size ~400 µm) similar to those of the adult crypts. At between 200–220 µm shell lengths, anterior and posterior adductor muscles were visibly present. When extended, the foot was long and slender but formed a bulbous tip when contracted, which had numerous and conspicuous stiff cirri. These probably serve a sensory function, related to sensing and/or handling of sediment grains. To identify the timing of active crawling and byssus production, pheromonal experiments were carried out 12 days post-hatching, at a shell length of 200–220 µm, and were repeated until 54 days thereafter.
From the culture of Crenella decussata juveniles hatched in late summer and separated into non-fed cultures, 34 out of 39 juveniles with shell lengths of between 190–240 µm (Table 1) became active a few minutes after addition of water from the bowl in which adults were kept. Figure 25 shows the results of the repeated pheromonal experiment with 50 juveniles from the batch hatched in late summer (see Table 1 for further details of the pheromonal experiments.). When tested 12 days after hatching, significantly more juveniles attached in the presence of adult water than in the control. Upon subsequent exposures, a similar response was observed after 17 and 26 days, but not after 54 days. On the basis of this observation, we suggest pheromonal responses cease when the offspring is sufficiently developed to take up an adult life in its own crypt.

Fig. 25. Crenella decussata. The responses of post-metamorphosed juveniles to repeated exposure to the exhalant water from adults (pheromonal factor) and aged seawater (control). The cultures comprised 25 individuals each and were reared in aged seawater and starved to avoid contamination.
Table 1. Crenella decussata. Juvenile responses to repeated addition of adult exhalant water (factor) and aged seawater (control). Bold numbers indicate when significantly more juveniles were active in response to the exhalant water of adults.

a ~17 h after commencing the experiment, 13 individuals had crept up the vial with adult exhalant water while only one had done so in the control vial.
JUVENILE GROWTH AND FEEDING
At ~200 µm shell length, some 50% of the fed individuals had started to feed as indicated by a greenish colouration to the digestive diverticulae. This was not seen in newly hatched juveniles of 185–195 µm shell length, suggesting that they were not yet sufficiently differentiated to be able to feed. At 200–220 µm shell length, ctenidial filaments had increased to 2–3, that is, with 1–2 inter-filamental spaces or ostia. The cilia of these ctenidial rudiments were observed to beat vigorously, but remains of yolk-reserves were also still present at this stage.
Figure 26 shows the size frequency distribution of A, fed and B, starved embryos and juveniles. The ~100 offspring retrieved from parental crypts in late summer and which were fed continuously, reached an average shell length of between 210–220 µm after 35 days, and an average shell length of 375 µm after 7.5 months. After 1.5 years, the offspring had reached an average shell length of 500 µm, ranging widely between 330–870 µm. Figure 27 shows that growth of 43 starved individuals also retrieved in late summer, continued until an average shell length of ~245 µm was obtained after 2.3 months, subsequent to which growth ceased and several individuals had died. Starved spat continued to grow at a similar rate as the fed spat for 30–40 days after which, however, growth declined (Figure 27). The brooded offspring thus appear to rely on embryonic energy resources until metamorphosis, and for one additional month thereafter. Subsequently, suspension-feeding becomes essential for further growth and development before individuals are capable of leaving the maternal crypt.
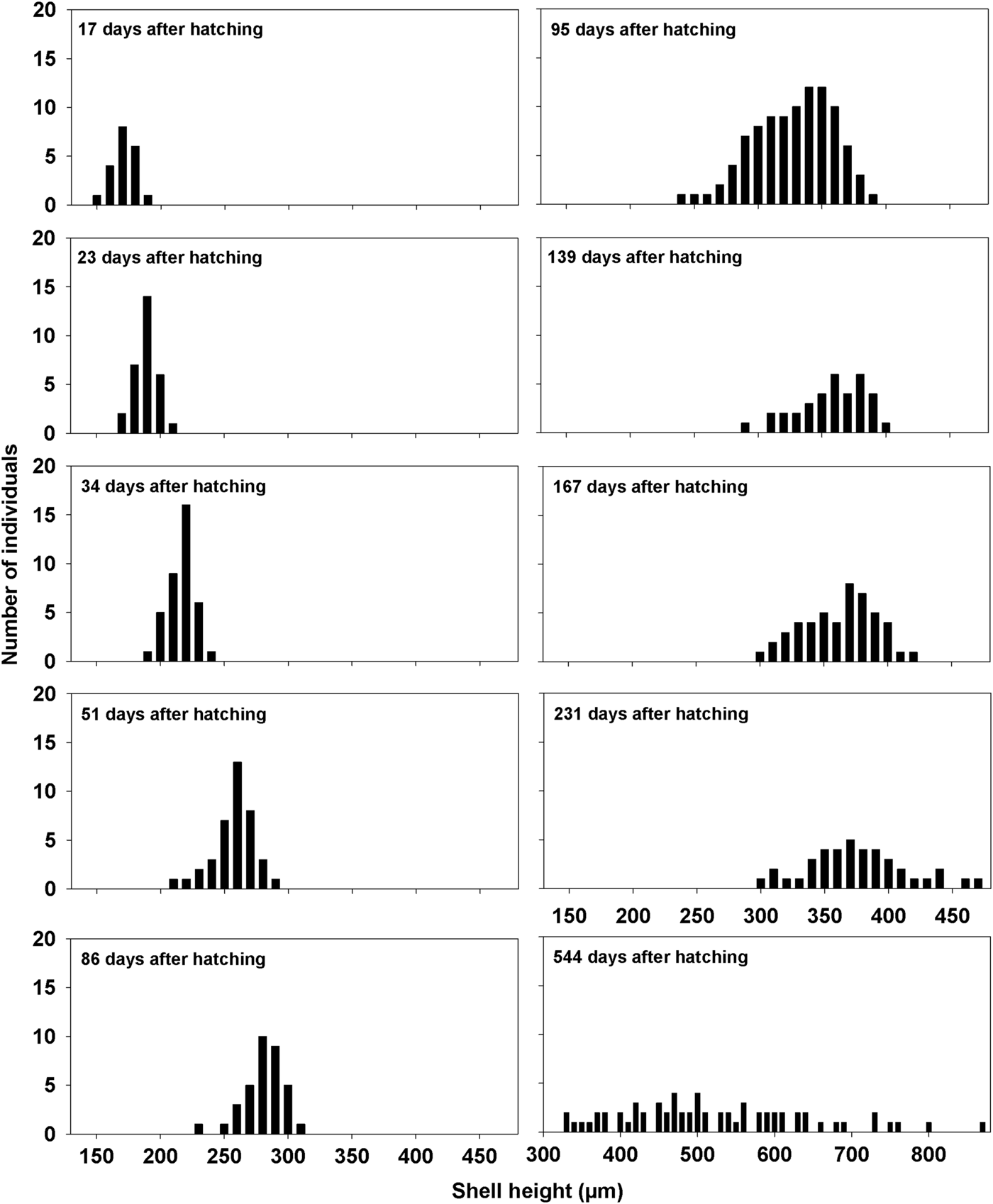
Fig. 26. Crenella decussata. Size frequency distributions of fed offspring from hatching until 1.5 years thereafter.

Fig. 27. Crenella decussata. Mean growth of fed (100 individuals) and starved (43 individuals) offspring for 7.5 months and 2.3 months after hatching, respectively. The error bars indicate 95% confidence intervals.
DISCUSSION
Crenella decussata has a circumboreal distribution and a depth distribution of from 32–149 m in the North Atlantic, and records down to 1100 m depth may represent shells only (Sneli et al., Reference Sneli, Schiøtte, Jensen, Wikander, Stokland and Sørensen2005). The anatomy of Crenella decussata is described herein for the first time and has revealed some remarkable features that allow for an interpretation of its reproductive strategy and life history traits. As an adult, C. decussata is not just small (<5 mm shell length) but has a thin, brittle, shell covered by a film-like periostracum. For a burrowing species, it clearly has few defences. But this is now explained by the fact that it lives within a granular crypt made up of sand grains from its well-sorted sub-tidal sand-gravel habitat and which are bound together by mucus possibly secreted from large secretory cells in each mantle margin. Unusually, however, the crypt has two posterior arms, which separate and accommodate the inhalant and exhalant streams. It is thought probable, again, that these are formed by the foot, which is capable of enormous extension (Figure 6, redrawn after Jeffreys [Reference Jeffreys1863]). It also possesses large byssal glands but, since adult individuals are not attached byssally inside their crypts, it seems possible that the glands are only active during the larval and juvenile stages in the life cycle, as in many bivalve larvae (Yonge, Reference Yonge1962). In this case, the secreted byssal threads are used to attach the young individuals to the internal lining of the exhalant tube of the maternal crypt. A similar situation is seen in Musculus subpictus, where juveniles use byssal threads to attach themselves to and mould pouches within the test of the ascidian Ascidiella aspersa (Müller, 1776) (List, Reference List1902; Morton & Dinesen, Reference Morton and Dinesen2011) although, in this case, the byssal threads are functional in the adult as well.
Table 2 compares the life history traits expressed by the type species of the mytiloid subfamilies Crenellinae, Musculinae and Modiolinae. The first two, Crenella decussata and Musculus discors (Linnaeus, 1767), are both small species that build a crypt and byssal nest, respectively, and reproduce by non-feeding larvae (lecithotrophic and direct development, respectively). The third, Modiolus modiolus, grows to a considerable size and lives byssally attached in dense aggregations on hard surfaces and in mixed sediments and reproduces by planktotrophic larvae. It also covers the shell in adventitious byssal setae soon after metamorphosis, possibly as a predator defence mechanism (Dinesen & Morton, Reference Dinesen and Morton2014). In comparison, byssal setae do not occur on the shells of the two nest-building mytiloids. Byssal setae are also absent in the musculine Modiolarca subpicta nestling in ascidian tests (Morton & Dinesen, Reference Morton and Dinesen2011), but are frequent in Idas argenteus Jeffreys, 1876 (Bathymodiolinae) dwelling in pholadid burrows and feeding on their offspring (Ockelmann & Dinesen, Reference Ockelmann and Dinesen2011).
Table 2. Comparative list of biological traits exhibited by the type species of the mytiloid subfamilies Crenellinae, Musculinae and Modiolinae.

a Cephalic eyes present in some musculines, e.g. Musculus subpictus.
b External (broadcast spawning) in some, e.g. Musculus subpictus.
The pheromonal attraction of offspring by adult mytilids is poorly understood. Interestingly, pheromonal attraction has been documented for Modiolus modiolus. When exposed to exhalant water of adult individuals, competent larvae of different parental origins have been observed attaching themselves by byssal mucus strings and metamorphosing. In this species, this mechanism thus guides recruiting individuals to existing populations (Dinesen & Ockelmann, unpublished information). It is not known if adult stimuli continue to affect the behaviour of recruits post-metamorphosis, for example by keeping individuals within a certain range of each other or close to individuals of the opposite sex. The findings of pheromonal attraction between parents and offspring in Crenella decussata is thus the more exciting, as this study demonstrates for this species that the effects seem to wear off with time. This study also provides support for the hypothesis that in C. decussata pheromonal attraction to prolong the time of brooding well past metamorphosis allows the juvenile to grow to a near-adult developmental stage whereat it is capable of leaving the maternal crypt and establishing itself in an independent crypt nearby. Our field observations suggest that C. decussata is most often found in small groups of 5–6 adult individuals. This and the results of this study would seem to confirm the observation of Jeffreys (Reference Jeffreys1863) that C. decussata is gregarious. This would facilitate the transfer of sperm from males to females thereby securing the successful reproduction of a species living in their individual crypts.
The adoption by Crenella decussata of a life encased within an adventitious crypt has resulted in modifications to adult anatomy. For example, the pericardial complex is situated, typically, anterior to a single, paired, posterior byssal retractor muscle unit. In addition, the paired posterior pedal retractor muscles are lost and the also paired anterior byssal retractor muscles are tiny (Morton, Reference Morton2015a) – features shared with Dacrydium ockelmanni (Mattson & Warén, Reference Mattson and Warén1977). The argument that the Crenellidae comprises two putative subfamilies: the Crenellinae and Dacrydiinae (Ockelmann, Reference Ockelmann1959, Reference Ockelmann1983) is, thus, supported herein on internal morphological grounds as well as shell characters. In representatives of both taxa too the outer demibranchs are greatly foreshortened anteriorly. Such an arrangement is typical of the Mytiloidea in general, albeit less exaggerated in non-crenellid taxa, suggesting for species of Dacrydium that they possibly share a reproductive strategy with C. decussata. This trend towards reduction of the anterior elements of the outer demibranch is matched by a similar, overall reduction in the anterior region of the shell and body. So much so, that not only are the anterior adductor and byssal retractor muscles reduced, so are the posterior byssal retractors and, in addition, the posterior pedal retractor muscles are lost (Figure 14).
Another interesting aspect of this reduction of the anterior adductor muscle and its close relationship with the anterior pallial retractor muscles is that Yonge (Reference Yonge1947) considered such muscles to have evolved from modified and cross-fused pallial retractor muscles. Yonge & Thompson (Reference Yonge and Thompson1976, figure 78) illustrated how this might have occurred in evolutionary terms and say (p. 143): ‘As lateral compression increased, the pallial (retractor) muscles in the depths of the embayments (concavities anterior and posterior to the ligament) would eventually cross-connect to form the characteristic anterior and posterior adductor muscles’ unique to the Bivalvia. Such a perceived ‘ancestral’ condition in relation to the anterior adductor muscle of Crenella decussata could suggest neoteny, or paedomorphosis, although the posterior adductor muscle is of the typical bivalve form and location. Stasek (Reference Stasek1962) also suggested that the outer demibranch is the last to develop in veneroideans and that where it does not might be a reflection of neoteny or paedomorphosis. This is possibly true for the Dacrydiinae and Crenellinae too but, in part at least, it might also be attributed to their miniaturization as postulated for the similarly tiny protobranch Nucula pusilla Angas, 1877, the minute arcoid Limopsis cristata Jeffreys, 1876 and the also miniature species of the veneroidean Neolepton (Morton, Reference Morton2012, Reference Morton2013, Reference Morton2015b) all of which occupy clean sub-tidal, well-sorted, sands and gravels and are thus, in terms of size, indistinguishable from them. There is, however, another aspect to this scenario. With the exception of L. cristata (as far as is known), all the other taxa identified above brood their fertilized ova (Bergmans, Reference Bergmans1978; Morton, Reference Morton2015b) and in the case of Neolepton species not only are the fertilized ova brooded within the maternal ctenidia but also secondarily maintained within the mantle cavity. The situation in C. decussata thus mirrors closely these other tiny marine bivalves but also the primary ctenidial and secondarily mantle brooding species of the freshwater Congeria kusceri Bole, 1962 (Morton & Puljas, Reference Morton and Puljas2013).
Putting this into context, therefore, it seems likely that Crenella decussata is not an example per se of neoteny but of miniaturization. This allows the taxa to enjoy the defensive opportunities afforded by occupation of a specialized, granular, habitat. Just as importantly, however, it also allows the allocation of resources not into massive shell growth and longevity, up to 500 years in the case Arctica islandica (Linnaeus, 1767) (Butler et al., Reference Butler, Wanamaker, Scourse, Richardson and Reynolds2013), but into reproduction and, more specifically, brooding of a few fertilized lecithotrophic ova into crawl-away juveniles – that can re-occupy the immediate environment of the parents. This represents a K-selection strategy in contrast to the r-selection of most, as we know them, common burrowing and byssally-attaching bivalves (Pianka, Reference Pianka1970).
The real significance of the exaggerated foreshortening of the anterior-most filaments of the outer demibranchs and the reduction of the outer labial palps of Crenella decussata is that it allows for the earliest phase of embryo brooding (Phase 1) by the females (Figure 28). This is achieved because of three important anatomical changes resulting from the ctenidial and palp reduction. Firstly, the outer demibranch reduction is so great that the gonadal apertures now open into the infra- instead of the supra-branchial chambers. Ova are therefore released, uniquely for the Mytiloidea, into this chamber and, secondly, fertilized here directly by inhaled spermatozoa instead of them having to penetrate the ctenidial ostia to access the supra-branchial chamber as in the typical bivalve. Thirdly, however, the fertilized ova in the infra-branchial chambers are bathed directly by inhaled water and not by exhaled and oxygen-depleted supra-branchial water.

Fig. 28. Crenella decussata. A drawing showing how mantle cavity brooded embryos are transferred subsequently to the exhalant component of the sand grain crypt where they are further brooded secondarily.
Embryos, possibly at the prodissoconch stage, are removed from the infra-branchial chamber when sufficiently mature after a period of brooding (Phase 1), but become engaged by the rejectory currents of the mantle and, thereby, discharged to the base of the exhalant siphon and thence make their way into the exhalant tube of the maternal crypt. Figure 28 illustrates how this transfer of larvae occurs. Phase 2 is where the released larvae are incubated yet further within the exhalant tube of the crypt. Here the nepioconch larvae become attached to the walls of the maternal crypt by fine byssal threads. As far as is known, such a method of maternal care is unique to Crenella decussata in the Mytiloidea (but not other miniature bivalves, as discussed above).
Similarly, no other mytiloid is known to build a crypt, although a number of taxa including species of Amygdalum, Musculista, Arcuatula, Musculus and Gregariella, build byssal nests – the former three in sand, the latter two within crevices or on macrophytes (Merrill & Turner, Reference Merrill and Turner1963; Morton, Reference Morton1973b, Reference Morton1980, Reference Morton, Morton and Tseng1982) and, presumably accidentally, sand grains do become incorporated into them. As described, however, the crypt of Crenella decussata is not constructed of byssal threads but of mucus-held sand grains.
Finally, the crypt of Crenella decussata has a structure similar to that of species of Dianadema (Anomalodesmata: Clavagelloidea), individuals of which live in a basal bowl with fused tubes arising from it posteriorly (Morton, Reference Morton2003, Reference Morton2009). In this case, however, the crypt is secreted by the mantle as it is in other clavagellids and penicillids that live endo- and epi-benthically, including species of Stirpulina and Brechites, respectively (Harper & Morton, Reference Harper and Morton2004; Morton, Reference Morton2006). The unrelated Eufistulana mumia (Spengler, 1783) (Gastrochaenidae) (Morton, Reference Morton1983a) also builds an adventitious endobenthic crypt. As far as is known, however, none of these adventitious crypt or tube dwelling bivalves brood their fertilized eggs.
In such a scenario, therefore, the crypt of C. decussata is unique in that it is produced not only for adult protection but also brood protection at the most vulnerable time of the juveniles’ lives. This intimacy appears to be sustained by juvenile positive responses to pheromones released into the maternal exhalant water possibly from either the osphradia lining the supra-branchial chamber or secretions from the enlarged glands occupying the bases of the ctenidial filaments and, thus again, discharging into the exhalant stream.
ACKNOWLEDGEMENTS
The authors wish to thank Tom Fenchel for providing facilities for the experimental studies at the Helsingør Marine Biological Laboratory, University of Copenhagen. The authors are grateful to Sanja Puljas (University of Split, Croatia), for much appreciated histological assistance, and to Katrine Worsaae (University of Copenhagen) and Tomas Cedhagen (University of Aarhus) for guidance in SEM techniques.