INTRODUCTION
Estadio de Quillota (EDQ) is the largest known pre-Columbian cemetery site within the Aconcagua Valley and has been described as one of the most important sites for the archaeology of Central Chile (Ávalos and Saunier Reference Ávalos and Saunier2011). The use of the cemetery has been presumed to be relatively continuous from at least the beginning of the Early Ceramic (ca. 300 BC), through until the Late Period when the region came under Inka influence (ca. AD 1450–1536) (Gajardo-Tobar and Silva Reference Gajardo-Tobar and Silva1970; Didier and Ávalos Reference Didier and Ávalos2009a, Reference Didier and Ávalos2009b). This time span encompasses an array of technological, social and cultural developments, from hunting and gathering, to the initial use of ceramics, early exploitation of cultigens, the intensification of maize (Zea mays) farming, and the burgeoning influence of the Inka Empire. The use of a cemetery at a single location seeing all of these developments would, in principle, provide an excellent opportunity to explore their impacts on human diet and health, changes in demographics, lifeways and mortuary practices. However, detailed contextual information for individual burials is lacking and existing chronological data for EDQ are limited. Questions remain regarding the history of the site and of the Aconcagua Valley, particularly around the timing and nature of the adoption and expansion of maize agriculture, as well as the timing of Inka influence in the region.
The Aconcagua Valley lies on the periphery of intensive maize agriculture in the Americas and also on the southern frontier of the Inka Empire. Despite the rich cultural heritage of the region, there are few existing radiocarbon dates for the large cemetery site at EDQ, or indeed the Aconcagua Valley, and a robust chronological framework is lacking. Much of the extant research and knowledge of the Valley’s archaeology is confined to the excavation and salvage reports of specific sites, especially for the mid to lower reaches of the river system. Temporal associations have so far largely been assigned using ceramic typo-chronologies and thermoluminescence (TL) dating alongside limited radiocarbon dates (Sánchez et al. Reference Sánchez, Pavlovic, Gonzalez and Troncoso2004; Ávalos and Saunier Reference Ávalos and Saunier2011; Cornejo Reference Cornejo2014).
This paper aims to establish an initial radiocarbon chronology for EDQ and determine where it fits within the chronology of the Aconcagua river valley system. This is important in terms of providing a framework within which to address questions around the timing of socio-political transitions and variations in subsistence strategies in the Aconcagua Valley that warrant further investigation. To this end, we present a preliminary radiocarbon chronology for EDQ by dating human bone collagen from 17 selected burials. New dietary stable isotope analysis (δ13C and δ15N) of human bone collagen for the EDQ burials with direct dates (including six pre-existing dates from Fuenzalida [Reference Fuenzalida2014]) are also presented to address dietary change.
Given the putative long timescale of burial at EDQ, stable isotope data from directly dated individuals can inform on changes in diet over time, particularly regarding the introduction and intensification of maize agriculture. The presence of culturally heterogeneous groups has been postulated for both EDQ and the Aconcagua Valley as a whole (Sánchez et al. Reference Sánchez, Pavlovic, Gonzalez and Troncoso2004; Sánchez Romero Reference Sánchez Romero2004; Ávalos and Saunier Reference Ávalos and Saunier2011; Fuenzalida Reference Fuenzalida2014). Limited previous stable isotope (δ13C and δ15N) evidence supports this by demonstrating considerable dietary variability among pre-Columbian groups in the Valley, particularly concerning the consumption of maize and marine resources (Swift et al. Reference Swift, Oyanedel Perez and Lopez Labbe2017). The stable isotope data when combined with radiocarbon dating can also address the extent to which dietary variability is diachronic or synchronic, the latter suggesting that different communities with distinct dietary practices utilized the same cemetery to bury their dead (cf. Santana-Sagredo et al. Reference Santana-Sagredo, Lee-Thorp, Schulting and Uribe2015).
There is also a question around the timing of the arrival of Inka influence in Central Chile, on the southern frontier of the Empire. Traditionally, the southward expansion of the Inka Empire has been based on a date of AD 1471, though nowadays many sources place it some decades earlier, at ca. AD 1450 (Uribe and Sánchez Reference Uribe, Sánchez, Falabella, Uribe and Sanhueza2016). However, Bayesian modeling of radiometric dates from other frontier regions of the Inka Empire in Ecuador and neighbouring regions of Argentina is increasingly suggesting that the traditional chronology of Inka expansion derived from the historical accounts of Spanish chroniclers may be incorrect (Ogburn Reference Ogburn2012; Marsh et al. Reference Marsh, Kidd, Ogburn and Durán2017). Of the EDQ burials with direct radiocarbon dates, nine have been identified as Late Period, of which seven were buried with “Local Inka Phase” ceramic offerings, the most abundant ceramic style observed in Late Period contexts (Fuenzalida Reference Fuenzalida2014; Dávila et al. Reference Dávila, Cortés, Mártinez, Hermosilla, Fuenzalida and Pavlovic2018). Direct dating of these burials should aid in clarifying the timing of the transition between the Late Intermediate Period and the Late Period, which saw the expansion of Inka influence into Central Chile.
The new radiocarbon dates, along with associated stable isotope analysis and chronometric modeling presented here provide a greater understanding not only of this important site and where it fits within the occupation sequence of the Valley, but also of the region’s broader archaeology, and questions surrounding the use and control of food resources on the southern frontier of intensive maize agriculture in the Americas, as well as the expansion of the Inka into their southern frontier.
BACKGROUND
The Aconcagua Valley extends from the Andes in the east to the Pacific Ocean in the west and encompasses diverse ecological zones. Prehistoric sites have been recorded along the length of the river valley, the course of which can be divided into several geographic zones: the lower course, from the coast to the coastal cordillera; the middle course, which includes the broad, fertile inland valley area running from north to south between the coastal cordillera and the Andean cordillera; and the upper course, comprising the upper basin of the Aconcagua in the foothills of the Andes. The tumulos-style cemetery at Estadio de Quillota is situated on the inland side of the coastal cordillera on the broad fluvial terrace of the middle course of the Aconcagua River. It is located within the modern city of Quillota, approximately 83 km northwest of Santiago, and 38 km northeast of the port city of Valparaíso (Figure 1).

Figure 1 (a) Map of the study area showing Estadio de Quillota and other sites from which there are previously published thermoluminescence or radiocarbon dates. The sites represented with bold text have existing radiocarbon dates (n = 8). Site represented with italic text have both radiocarbon and thermoluminescence dates. Sites represented with a square symbol have identified Late Period/Inka contexts (n = 13). The remaining sites have only thermoluminescence dates (n = 24). (b) Cross-section (not to scale) depicting the diverse geographical zones of the Aconcagua River Valley system, and the location of Estadio de Quillota.
There have been multiple excavations at EDQ beginning in 1955–1956 (Gajardo-Tobar and Silva Reference Gajardo-Tobar and Silva1970) with subsequent commercial salvage excavations in 2009 and 2010 (Didier and Ávalos Reference Didier and Ávalos2009a, Reference Didier and Ávalos2009b; Baeza Reference Baeza2010). Over 200 burials have been excavated, estimated to represent only a proportion of the total number at the site. Human remains have also been recovered from salvage excavations at the adjoining site of Calle Arauco, which forms an extension of the EDQ cemetery, and is also included in this study.
It is thought that EDQ, the largest cemetery in the region, was in use from the Early Ceramic Period (ECP) (ca. 300 BC–AD 1000/1200), through the Late Intermediate Period (LIP) (ca. AD 1000/1200 –1450), and into the Late Period (LP) (ca. AD 1450–1536) (Gajardo-Tobar and Silva Reference Gajardo-Tobar and Silva1970; Didier and Ávalos Reference Didier and Ávalos2009a; Baeza Reference Baeza2010; Ávalos and Saunier Reference Ávalos and Saunier2011). The Late Holocene in Central Chile, from ECP to LP, has been characterised by heterogeneous enclaves of at least partly contemporaneous local cultures (Bato, Llolleo and Aconcagua) alongside other cultural groups that expanded into the area from the semi-arid north, such as the Diaguita, followed by the expanding influence of the Inka Empire (Sánchez et al. Reference Sánchez, Pavlovic, Gonzalez and Troncoso2004; Ávalos and Saunier Reference Ávalos and Saunier2011; Falabella et al. Reference Falabella, Pavlovic, Planella, Sanhueza, Falabella, Uribe and Sanhueza2016; Uribe and Sánchez Reference Uribe, Sánchez, Falabella, Uribe and Sanhueza2016) (Table 1).
Table 1 Summary of chronological and cultural phases in the Aconcagua Valley from the Early Ceramic Period to the Late Period (see also Sánchez et al. Reference Sánchez, Pavlovic, Gonzalez and Troncoso2004; Ávalos and Saunier Reference Ávalos and Saunier2011; Falabella et al. Reference Falabella, Pavlovic, Planella, Sanhueza, Falabella, Uribe and Sanhueza2016a, 2016b; Uribe and Sánchez Reference Uribe, Sánchez, Falabella, Uribe and Sanhueza2016).
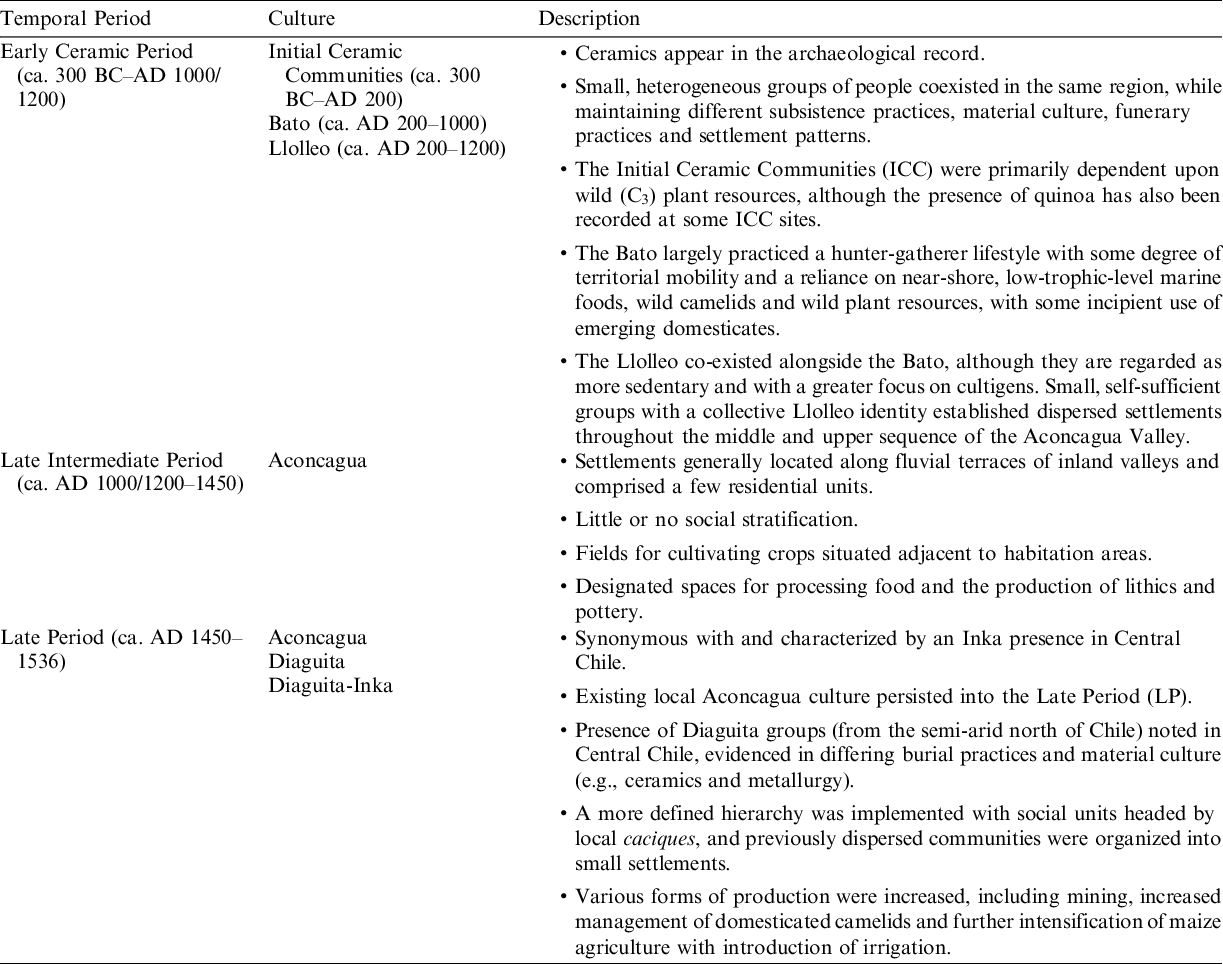
The contextual information available for individual burials at EDQ is extremely limited, largely due to the time constraints of salvage excavations. However, analysis of the ceramics from a subset of the burials (Fuenzalida Reference Fuenzalida2014), combined with limited descriptions and photographs of other burials has allowed for the attribution of some individuals to the Early Ceramic (ECP), Late Intermediate (LIP) and Late Periods (LP). LP ceramics, specifically of the “Local Inka Phase” style, have been identified from the grave goods of seven of the 23 individuals included in this study, and radiocarbon dates have previously been reported for six of those individuals (Fuenzalida Reference Fuenzalida2014), confirming use of the site into the early 15th century. Two thermoluminescence dates from pots not associated with burials have also been reported from one of the previous excavations: an anthropomorphic jug from the ECP (AD 405 ± 170) (typical of the Llolleo culture) and a cup with a stepped trichrome motif, identifiable as an LP style (AD 1420 ± 45) (reported without lab codes in Didier and Ávalos Reference Didier and Ávalos2009a).
Grave goods at EDQ mainly comprised pottery of varying forms, the majority being locally produced ceramics such as “Aconcagua Local” or “Local Inka Phase” (Fuenzalida Reference Fuenzalida2014). External influences, predominantly from the Diaguita culture of the semi-arid north, but also from the Inka are described in LP burials (Gajardo-Tobar and Silva Reference Gajardo-Tobar and Silva1970; Didier and Ávalos Reference Didier and Ávalos2009a; Baeza Reference Baeza2010). Other burial offerings included ceramic pipes, bone instruments, copper and stone jewellery, stone tools and projectile points. The presence of pipes, in particular, has led to an interpretation of special ritual and spiritual significance for this site (Ávalos and Saunier Reference Ávalos and Saunier2011).
Excavation reports note three distinct strata across the site at EDQ (Gajardo-Tobar and Silva Reference Gajardo-Tobar and Silva1970; Baeza Reference Baeza2010); however, this information is not available for each individual burial. It is recorded that burials from the upper two strata have been attributed to the LIP and LP, with many interred in tumuli with stones surrounding the body. These burials were often also accompanied by funerary ceramics with many of the LP burial offerings demonstrating influence of Diaguita and Inka styles (Gajardo-Tobar and Silva Reference Gajardo-Tobar and Silva1970; Didier and Ávalos Reference Didier and Ávalos2009b; Baeza Reference Baeza2010). The majority of LIP and LP burials were in a prone position, but some were supine, with body and limbs extended and typically oriented with the head pointing east or northeast. Burials attributed to the ECP, on the other hand, were generally found in the lowest strata and interred in a flexed position. Many burials, however, were considerably damaged due to previous construction work on the site, meaning that burial position often could not be recorded during excavation.
Maize Agriculture in Central Chile
The appearance and subsequent intensification of maize agriculture in the archaeological record of the Americas is an important indicator of agricultural expansion and is often concomitant with social and political developments (Hastorf and Johannessen Reference Hastorf and Johannessen1993; Emerson et al. Reference Emerson, Hedman and Simon2005; Finucane et al. Reference Finucane, Agurto and Isbell2006; Gil et al. Reference Gil, Tykot, Neme, Shelnut, Staller, Tykot and Benz2006). The South Central Andes (northwestern Argentina and Central to Central-South Chile) was home to some of the southernmost agriculturalists of South America, and the final region to adopt cultivation of pre-Hispanic crops (Gil Reference Gil2003). The broad fertile river valleys of Central Chile were ideally suited for large-scale agriculture, with chroniclers from the post-contact period describing the Aconcagua Valley in particular as an epicentre for the production of maize (Frézier Reference Frézier1717). Yet the majority of Central Chilean archaeological research, including stable isotopic studies, has concentrated on the Maipo-Mapocho basin around Santiago and the Cachapoal river to the south (Falabella et al. Reference Falabella, Pavlovic, Planella, Sanhueza, Falabella, Uribe and Sanhueza2016). The only previous isotopic study from the Aconcagua Valley incorporated a handful of sites from coastal and inland valley settings and found considerable variability in dietary practices from the ECP to the LP. This study found an increase in mean δ13C values during the LIP, suggesting that intensification of maize took place during this period (Swift et al. Reference Swift, Oyanedel Perez and Lopez Labbe2017). Isotopic reconstructions of diet in the Maipo-Mapocho basin to the south indicate that some of the inland communities of the ECP were among the first in Central Chile to adopt maize (Falabella et al. Reference Falabella, Planella and Tykot2008). However, intensive maize agriculture did not appear until ca. AD 1000-1200 (Falabella et al. Reference Falabella, Planella and Tykot2008, Reference Falabella, Pavlovic, Planella, Sanhueza, Falabella, Uribe and Sanhueza2016).
Stable carbon and nitrogen isotopic analyses are particularly suited to answering questions regarding the consumption of maize. Most wild plants and cultigens follow a C3 photosynthetic pathway, while maize uses the C4 pathway. The δ13C signature of C4 plants is distinct from C3 plants, such that incorporation of maize in the diet will result in significantly elevated stable carbon isotope values in body tissues. Maize is the most important Americas cereal and often recognized as the only C4 plant contributing significantly to diet in Central Chile (Falabella et al. Reference Falabella, Sanhueza, Abarca and José Herrera2020). However, in southern Peru, Cadwallader et al. (Reference Cadwallader, Beresford-Jones, Whaley and O’Connell2012) have identified various other C4 plants from the Graminae, Chenopodiaceae, Asteraceae and Amaranthaceae families, which include several important edible plants for both humans and animals. Hence, the possibility of C4 plants other than maize contributing to elevated δ13C values cannot be entirely excluded, though there is no palaeobotanical evidence for intensive use of C4 plants apart from Zea mays.
Stable nitrogen (δ15N) isotope analysis complements the interpretation of the δ13C data by providing information about dietary trophic level, which can be useful in distinguishing mixed C3-C4 terrestrial diets from mixed marine diets. However, it is important to bear in mind that some agricultural practices, such as fertilizing crops, can substantially raise δ15N values in horticultural produce (Szpak et al. Reference Szpak, Millaire, White and Longstaffe2012; Santana-Sagredo et al. Reference Santana-Sagredo, Schulting, Méndez-Quiros, Vidal-Elgueta, Uribe, Loyola, Maturana-Fernández, Díaz, Latorre and McRostie2021). Climate changes can also cause variation in δ15N, with increased aridity, and δ13C, with proportional variations in C4 plants linked to temperature. Central Chile is subject to a Mediterranean climate with dry summers and wet winters. The current climatic conditions have remained much the same for the last 3000 years with seasonal and inter-annual variations in rainfall influenced by El Niño Southern Oscillation fluctuations (Villagrán and Varela Reference Villagrán and Varela1990; Jenny et al. Reference Jenny, Valero-Garcés, Urrutia, Kelts, Veit, Appleby and Geyh2002; Villa-Martínez et al. Reference Villa-Martínez, Villagrán and Jenny2003).
The Inka Empire in the Aconcagua Valley
The Inka Empire, Tawantinsuyu, the “Land of the Four Quarters,” comprised four regions of expansion: Antisuyu, Cuntisuyu, Chinchasuyu and Collasuyu, with Cuzco located at the nexus of the quarters. Collasuyu was the southernmost and the largest quarter of the Empire, incorporating parts of modern southern Peru, western Bolivia, northwestern Argentina, northern Chile and extending as far south as Central Chile (Moseley Reference Moseley2001; D’Altroy Reference D’Altroy2002). The Aconcagua Valley of Central Chile held strategic significance in the Inka State during the Late Period as an administrative centre for the southern reaches of the Empire (Mariño de Lobera Reference Mariño de Lobera1970 [1580]; Vivar Reference Vivar1988 [1558]; Valdivia Reference Valdivia1991 [1555]; de Góngora Marmolejo 2010 [1524-1575]).
Inka control of Central Chile is generally held to have been via a system of indirect government (Silva Reference Silva1978; Falabella Reference Falabella1994; Stehberg Reference Stehberg1995; Uribe Reference Uribe2000; Sánchez Romero Reference Sánchez Romero2002; Hermosilla et al. Reference Hermosilla, González and Baudet2005). Recent discussions surrounding the nature of the expansion of Tawantinsuyu into the Maipo-Mapocho basin surrounding Santiago have presented arguments for an initial phase of Inka influence in the region, followed by a secondary phase of Imperial occupation (eg. Alconini Reference Alconini2016; Dávila et al. Reference Dávila, Cortés, Mártinez, Hermosilla, Fuenzalida and Pavlovic2018; Pavlovic et al. Reference Pavlovic, Sánchez, Pascual, Martínez, Cortés, D’ávila and La Mura2019; Mundt and Marsh Reference Mundt and Marsh2021). In the Aconcagua Valley it is proposed that the initial phase of Inka influence involved varied interaction between the Inka and the local populations and included the circulation of ideas, material culture, political negotiation and redistribution, while the subsequent phase saw the consolidation of Central Chile into the Empire’s southern frontier and the appearance of Inka structures, tambos (state-owned lodgings located on Inka roads), qollqas (storehouses), pukaras (fortified sites), huacas (shrines), high altitude ceremonial sites and cemeteries. Such Inka emplacements are present at various intervals along the Aconcagua Valley from coast to mountains (Coros and Coros Reference Coros and Coros1999; Sánchez Romero Reference Sánchez Romero2002, Reference Sánchez Romero2004; Troncoso et al. Reference Troncoso, Pavlovic, Acuto, Sánchez and González2012; Pavlovic et al. Reference Pavlovic, Pascual, Cortés, Martínez, Albán, Dávila, Rosende, Villela, Falabella, Sanhueza and Cornejo2014; Dávila et al. Reference Dávila, Cortés, Mártinez, Hermosilla, Fuenzalida and Pavlovic2018).
The existing local culture, the Aconcagua, persisted into the early stages of the Late Period, but exhibited signs of “foreign” Diaguita and Inka influences in their material culture. The heterogeneity observed in Late Period ceramic morphology and technology has been interpreted as a representation of different degrees of local integration with the Inka State, where principles of exclusion and inclusion operated between Tawantinsuyu and different local communities (Martínez Reference Martínez2011; Fuenzalida Reference Fuenzalida2014; Dávila et al. Reference Dávila, Cortés, Mártinez, Hermosilla, Fuenzalida and Pavlovic2018; Pavlovic et al. Reference Pavlovic, Sánchez, Pascual, Martínez, Cortés, D’ávila and La Mura2019). A burgeoning Inka occupation subsequently saw various Imperial structures used for ceremonial, defensive and administrative purposes established at strategic points along the river valleys and longitudinal roads (Stehberg Reference Stehberg1995; Coros and Coros Reference Coros and Coros1999; Troncoso et al. Reference Troncoso, Pavlovic, Acuto, Sánchez and González2012).
Even though such a substantial Inka presence has been recorded in the Aconcagua Valley from the coast to the Andes, systematic archaeological work in the inland valley along the midcourse of the Aconcagua River is scarce, despite the importance of this section of the valley. Rather, previous work has been concentrated in the upper basin of the Aconcagua. It has been postulated recently that the upper basin of the Aconcagua may have been the initial focus of imperial activity and the point of diffusion for Inka expansion in Central Chile (Mundt and Marsh Reference Mundt and Marsh2021). However, this assertion is based on a compilation of TL dates, the reliability of which have been called into question in this region, with discrepancies in TL dates noted by several sources (Schiappacasse et al. Reference Schiappacasse, Román, Muñoz, Deza, Focacci and Niemeyer1991; Pavlovic et al. Reference Pavlovic, Troncoso, Sánchez and Pascual2012; Marsh et al. Reference Marsh, Korpisaari, Mundt, Gasco and Durán2021).
Radiocarbon dating of Inka contexts has also been limited to the upper reaches of the Aconcagua at the sites of El Tigre, a site with monumental Inka architecture (Pavlovic et al. Reference Pavlovic, Troncoso, Sánchez and Pascual2012), and Los Nogales, a domestic site where Inka metallurgical practices had been adopted (Plaza and Martinón-Torres Reference Plaza and Martinón-Torres2015). The body of a frozen and naturally mummified child sacrificed in an Inka capacocha ritual has also been recovered from a high altitude ceremonial site on Mt Aconcagua, which lies over the modern border in Argentina but sits directly at the head of the Aconcagua Valley, and dated to cal AD 1407–1491 (GX-19991-AMS: 370 ± 70 14C yr BP; and Beta-88785: 480 ± 40 14C yr BP, 453 ± 35 14C yr BP, χ2, T=1.8 (5%3.8)) (Fernández et al. Reference Fernández, Panarello and Schobinger1999; Marsh et al. Reference Marsh, Kidd, Ogburn and Durán2017). Each of these established sites is from the later phase of Imperial occupation in the region. However, there have been no efforts thus far towards radiocarbon dating earlier contexts likely to represent the initial dispersion of Inka influence into central Chile.
The traditional chronology of Inka expansion was derived from the historical accounts of Spanish chroniclers. In particular, the writings of Caballo Balboa (Reference Caballo Balboa1951 [1586]) were accepted as reliable accounts and from these, a traditional chronology and succession of Inka kings was proposed by Rowe (Reference Rowe1944, Reference Rowe1945). Despite some opposing views, Rowe’s chronology dominated the argument and thus became the most widely accepted and utilized (Pärssinen Reference Pärssinen1992). According to this chronology, there was a pre-Imperial phase in Cuzco from ca. AD 1200 to 1438, after which the Inka began conquering nearby neighbouring regions of the four quarters under the reign of Pachacuti Inka Yupanqui. The majority of expansion south into Collasuyu supposedly took place after incursions north into Peru and Ecuador, and after Topa Inka Yupanqui succeeded his father, from AD 1471 to 1493 (Figure 2). However, the chronicle of Betanzos (Reference Betanzos1987 [1551]), which was published after Rowe had already determined his chronology, indicates that certain parts in the north of Collasuyu were incorporated into the Empire during the reign of Pachacuti Inka Yupanqui (ca. 1438–1463), and therefore several decades earlier than postulated by Rowe.

Figure 2 Map of the Inka Empire based on Rowe’s (1944, 1945) chronology, depicting the phases of Imperial expansion under different Inka rulers according to the traditional chronology, with the southern expansion into Collasuyu taking place after much of the northern territories had already been integrated into the Empire.
There is now growing evidence to support the hypothesis that the Inka may have expanded into the four quarters of Tawantinsuyu earlier than recorded in Rowe’s chronology; various radiocarbon dates from Inka contexts indicate that the expansion of the Empire towards its southern limits may have taken place several decades before AD 1471 (Rodríguez et al. Reference Rodríguez, Morales, González and Jackson1993; Williams and D’Altroy Reference Williams and D’Altroy1998; Raffino and Stehberg Reference Raffino, Stehberg, Politis and Alberti1999; Sánchez Romero Reference Sánchez Romero2004; Hermosilla et al. Reference Hermosilla, González and Baudet2005; Ogburn Reference Ogburn2012; Cornejo Reference Cornejo2014; Alconini Reference Alconini2016; Mundt and Marsh Reference Mundt and Marsh2021). Recent Bayesian modeling of radiometric dates from other sites along the Empire’s southern reaches in Argentina has also suggested an Inka presence up to 100 years earlier than the historically recorded date, with an estimated arrival of cal AD 1350–1440 (95% probability) (Marsh et al. Reference Marsh, Kidd, Ogburn and Durán2017). These findings have been followed by calls for more radiometric dating of Inka contexts from frontier zones of the Empire, and have not only raised questions about the timing of the Inka expansion, but also the order in which each of the territories were subjugated by Inka forces (Ogburn Reference Ogburn2012; Marsh et al. Reference Marsh, Kidd, Ogburn and Durán2017).
MATERIALS AND METHODS
Samples of human bone were obtained from 23 individuals held in the Museo Historico-Arqueologico de Quillota’s collections from the sites of Estadio de Quillota and Calle Arauco, two adjacent sites that formed part of the same pre-Columbian cemetery complex (Table 2; Figure 3). AMS radiocarbon dating was carried out on bone collagen for 17 of the individuals, the remaining six of the 23 having been previously dated (Fuenzalida Reference Fuenzalida2014), but lacking δ13C and δ15N measurements. Stable carbon and nitrogen isotope measurements were made separately for all 23 samples of human bone collagen.
Table 2 Samples of human bone from Estadio de Quillota (samples with EDQ prefix) and Calle Arauco (Ar prefix) used for AMS radiocarbon dating, and δ13C and δ15N measurements.

* Samples used only for δ13C and δ15N measurements, as radiocarbon dates for the same individuals are already provided in Fuenzalida (Reference Fuenzalida2014).
Sex estimates include F = female, F? = probable female, ? = indeterminate sex, M? = probable male, M = male. Age categories include Adult = 18 years of age or older, Sub-adult = under 18 years of age. Recorded burial sectors include CA = Calle Arauco (Arauco Street), TA = Tribuna Andes (eastern grandstand), TAS = Tribuna Andes Sur (south-eastern grandstand), C = Cancha (playing field). Burial positions include Ext. = extended, Flexed = flexed, Pr.= prone, Sup. = supine, Lat. (L or R) = lateral (left or right side down). Burial direction records the cardinal direction towards which the individual’s head was oriented. Burial offerings include C = ceramic (with unclassified temporal/cultural period), CLP = Late Period ceramic, F = faunal remains, S = shell, L = lithic.

Figure 3 Site plan of Estadio de Quillota from 2009 excavations (adapted from Baeza Reference Baeza2010).
The age-at-death of each individual sampled was estimated based on dental eruption for subadults (Ubelaker Reference Ubelaker1989) and dental attrition for adults (Lovejoy Reference Lovejoy1985). Sex was estimated, where possible, by morphological assessment of pelvic and skull fragments (Buikstra and Ubelaker Reference Buikstra and Ubelaker1994). Where available, information regarding burial location, position, orientation and grave goods was taken from the excavation reports (Baeza Reference Baeza2010). The human bone samples analyzed here derive from burials in four adjoining sectors of the cemetery. The majority of individuals at EDQ were buried in an extended prone position, which is reflected in the sample for radiocarbon dating. There is information available for 18 of the 23 individuals included in this study regarding burial position, with 13 in an extended prone position, one in an extended lateral to prone position, three in an extended supine position, and one in a flexed position.
Pretreatment and Collagen Extraction
Collagen was extracted from the bone samples using a modified Longin (Reference Longin1971) protocol. Bone fragments were cleaned by shot blasting with aluminium oxide pellets. Subsamples were crushed and demineralised in 0.5M HCl at 5ºC for several days. The HCl solution was changed periodically until the samples ceased to produce observable CO2. The demineralized samples were placed into sealed tubes of pH3 H2O and gelatinized at 70ºC for 48 hours. The supernatant was filtered to remove insoluble residue using 45–90 μm Ezee-filtersTM and then freeze-dried for approximately 48 hours.
Stable Isotope Analysis
Approximately 1 mg of collagen for each of the samples was weighed into tin capsules. All samples were analyzed using a Sercon 20/22 Isotope Ratio Mass Spectrometer in continuous flow mode at the University of Oxford’s Research Laboratory for Archaeology and the History of Art. All material was analyzed in duplicate to ensure reproducibility, with a precision greater than ± 0.2‰ based on repeat run of standards. Alanine standards of known value (δ13C = –27.2 ± 0.1‰, δ15N = 1.6 ± 0.2‰) were included at intervals throughout runs to allow for drift correction. Matrix-matched, in-house standards of cow (δ13C = –24.3 ± 0.1‰, δ15N = 7.9 ± 0.2‰) and seal collagen (δ13C = –12.5 ± 0.1‰, δ15N = 16.1 ± 0.2‰) were also included to allow for two-point calibration of the results (Coplen et al. Reference Coplen, Brand, Gehre, Gröning, Meijer, Toman and Verkouteren2006). The mean calibrated δ13C and δ15N measurements for all samples are reported in units per mil (‰) relative to international standards for δ13C (VPDB) and δ15N (AIR).
AMS Radiocarbon Dating
Approximately 5 mg of collagen from the 17 samples to be dated were combusted, graphitised and pressed into targets following routine protocol for the Oxford Radiocarbon Accelerators Unit (ORAU) (Brock et al. Reference Brock, Higham, Dichfield and Ramsey2010). Collagen was prepared following the protocol outlined above and was not subjected to ultrafiltration. Radiocarbon dating was undertaken at ORAU’s High Voltage Engineering Europa (HVEE) AMS. The resulting stable isotope corrected 14C dates were calculated following Bronk Ramsey et al. (Reference Bronk Ramsey, Higham and Leach2004).
Calibration and Modeling
All calibrations and Bayesian models were determined using OxCal version 4.4 (Bronk Ramsey Reference Bronk Ramsey2020). The radiocarbon dates were calibrated to a 95.4% probability range, using the SHCal20 Southern Hemisphere atmospheric curve (Hogg et al. Reference Hogg, Heaton, Hua, Palmer, Turney, Southon, Bayliss, Blackwell, Boswijk and Bronk Ramsey2020). Bayesian modeling utilizes a statistical approach to interpreting radiocarbon dates, incorporating assumptions known as priors, to constrain the resulting calibrated probability distributions for groups of dates (Bayliss and Bronk Ramsey Reference Bayliss, Bronk Ramsey, Buck and Millard2004; Bronk Ramsey Reference Bronk Ramsey, Barcelo and Bogdanovic2015). Bayesian modeling of prior assumptions alongside radiocarbon dates can be used to obtain improved chronological resolution for sites and events, e.g., the timing of Inka expansion. Kernel Density Estimates are employed to create a continuous probability density distribution with modeled boundaries to summarise groups of dates (Bronk Ramsey Reference Bronk Ramsey2017). Modeled results are presented in italics. Both individual dates and modeled ranges are presented at 95.4% confidence.
Statistics
Apart from the Bayesian modeling and Kernel Density Estimates of radiocarbon dates in OxCal 4.4, all other statistical analyses were carried out using R version 3.5.0 (RCoreTeam 2018) with RStudio 1.3.1056 (RStudioTeam 2020). Shapiro-Wilk tests indicated that subgroups of radiocarbon dates and stable isotopic data did not depart significantly from a normal distribution (p > 0.05). Therefore, parametric two-sided Student’s t-tests were employed, with α = 0.05, for all statistical comparisons of means.
Assessment of Collagen Quality
The quality of collagen preservation for both radiocarbon dating and stable isotope analysis was assessed by collagen yield (% wt greater than 1%), % wt of carbon and nitrogen relative to the combusted collagen (30–50% C; 11–16% N), and the atomic ratio of carbon to nitrogen (C:N between 2.9 and 3.6) (DeNiro Reference DeNiro1985; Ambrose Reference Ambrose1990; Van Klinken Reference Van Klinken1999; Brock et al. Reference Brock, Higham, Dichfield and Ramsey2010). All collagen analyzed within this study, except for EDQ17037 (UGAMS 13208), fell within ranges indicating acceptable preservation. The stable isotope results for EDQ17037 and the corresponding previous radiocarbon date (UGAMS 13208), for which collagen quality data were not originally reported, were excluded from further analysis, interpretation and chronometric modeling.
RESULTS
Radiocarbon Dates
The new radiocarbon dates for Estadio de Quillota range from 399 to 196 cal BC (OxA-V-2735–19) to cal AD 1413–1458 (OxA-V-2735-18) (Table 3). The four earliest dates 339–196 cal BC to cal AD 128–339 (OxA-V-2737-44) are followed by a gap of roughly 1000 years before a cluster of 13 later dates between cal AD 1280–1387 (OxA-2730-55) and 1413–1458.
Table 3 Radiocarbon dates and stable carbon (δ13C) and nitrogen (δ15N) results from the human bone collagen samples from Estadio de Quillota.

1 Age categories include A (Adult) = 18 years of age or older, SA (Sub-adult) = under 18 years of age.
2 Recorded sex estimates include F = female, F? = probable female, ? = indeterminate sex, M? = probable male, M = male. ‘OxA V’ designations refer to the fact that the collagen was not subjected to ultrafiltration.
* Dates with a UGAMS code originally reported in Fuenzalida (Reference Fuenzalida2014).
∼ As a random internal control, OxA V-2730-53 and OxA V-2730-54 (EDQ 16077) are duplicate dates from the same collagen sample and have been calibrated together using the R_combine function in OxCal 4.4 (χ2 test: df=1 t=1.0(5% 3.8)).
LP Samples obtained from known Late Period burial contexts.
^ The radiocarbon date and the isotopic data for EDQ17037 (UGAMS13208) were excluded from any further analysis due to poor collagen yield and quality. While falling within the expected date range, the radiocarbon date is also excluded from further consideration as collagen quality data were not reported when originally dated (Fuenzalida Reference Fuenzalida2014).
Stable Isotopes
The δ13C results have a wide range from –21.5‰ to -8.4‰ (X̄ = –12.8‰ ± 4.2, n = 22) (Table 3). The δ15N results range from 4.4‰ to 8.6‰ (X̄ = 7.0‰ ± 1.3, n = 22). There is a strong positive linear correlation between δ13C and δ15N (r 2 = 0.84, p < 0.001). It is clear that the δ13C and the δ15N results are distributed bimodally with one smaller group of lower values (X̄ δ13C = –21.1‰ ± 0.5; δ15N = 4.7‰ ± 0.6, n = 4) and one of higher values (X̄ δ13C = –11.0‰ ± 1.4; δ15N = 7.5‰ ± 0.8, n = 18). The group of four lower δ13C and δ15N values corresponds to the four earlier radiocarbon dates reported above, while the group of higher values corresponds to the cluster of later dates. EDQ is an inland site and—despite the correlation between the two isotopes (see below)—there is no indication of any significant consumption of marine or freshwater resources that would skew the radiocarbon dates, meaning no reservoir corrections are necessary.
DISCUSSION
Chronology of Estadio de Quillota
The radiocarbon dates from EDQ fall into two distinct phases of use, which correspond to the new isotopic data for dependence on maize-based resources, discussed further below. These two phases, with an interval of approximately 1000 years between them, contrast with previous expectations of a continuous and extended use of the cemetery. The samples were selected to provide coverage of the different sectors of the cemetery. However, a considerable number of burials are known to remain in situ at EDQ, so it is important to acknowledge that this 1000-year gap (which would correspond to the majority of the ECP and the first half of the LIP using the existing chronological framework for Central Chile) could be the result of a sampling bias. Future efforts towards conservation and analysis of the ceramics recovered from EDQ, coupled with further radiometric dating, would help to confirm the finding here of two distinct phases of use.
The earlier phase with four dates from 399–196 cal BC (OxA-V-2735-19) to cal AD 128–339 (OxA-V-2737-44) aligns with the beginning of the ECP in the existing Central Chilean chronological framework (ca. 300 BC–AD 1000/1200), particularly the period when the Initial Ceramic Communities (ICC) (ca. 300 BC–AD 200) appear in the archaeological record of the region. One of two available TL dates (AD 405 ± 170) for EDQ, from a Llolleo pot (Didier and Ávalos Reference Didier and Ávalos2009a, reported without lab code), falls after this cluster of radiocarbon dates, but within the range of the Llolleo culture (ca. AD 200–1200).
Based on the current chronological framework for the region, the appearance of the ICC at the beginning of the ECP, for which the defining material correlate is the presence of ceramics, is assumed to have occurred around ca. 300 BC (Sanhueza and Falabella Reference Sanhueza and Falabella1999). However, recent Bayesian modeling of the earliest dated ceramics from Central Chile indicates this technology most likely appeared around ∼180 cal BC (Marsh Reference Marsh2017), coincident with other adjacent regions, suggesting that the current chronological framework requires some reconsideration regarding the commencement of the ECP.
The two more recent of the four dated individuals from this earlier phase of use at EDQ ((EDQ16099: 146 cal BC-cal AD 22 (OxA-V-2730-57) and EDQ17061: cal AD 128-339 (OxA- V-2737-44)) were recorded with ceramic offerings as well as lithics and faunal remains, and EDQ16099 was also found with shells. There are no further details regarding the pottery styles or descriptions of the other artefacts. However, these two new radiocarbon dates fall within Marsh’s (Reference Marsh2017) proposed starting boundary for the ECP in Central Chile around ∼180 cal BC. Of the other two individuals from this earlier phase of use, EDQ17034 was not interred with any funerary offerings, and for Ar16016 there are no records to reveal whether any grave goods accompanied this burial.
A possible shift in the style of burial position may also be indicative of cultural differences. EDQ17034 was buried in a flexed position, which is characteristic of ECP and earlier burials. However, the site plan for EDQ suggests that EDQ16099 and EDQ17061 were buried in an extended position, a burial style more usually considered to be associated with LIP burials (Saunier and Ávalos Reference Saunier and Ávalos2010).
The early phase dates from EDQ are followed by a hiatus of ca. 1000 years before a later phase of 18 dates with a start boundary of cal AD 1290–1380 to an end boundary of cal AD 1420–1470 (Table 4). The cluster of later dates from EDQ is noticeably constrained with this modeled phase lasting for a span of only 47 to 145 years (95.4%). The later phase of use falls within what is currently accepted as the terminal stages of the LIP. However, eight of these burials are from LP contexts, seven of whom were buried with pottery defined as “Local Inka Phase” Aconcagua ceramics (Figure 4). “Local Inka Phase” ceramics are vessels made following the local (Aconcagua) pottery traditions, but with new decorative and morphological features (e.g., inscribed angles and handles attached to the lip), and the incorporation of other foreign references (e.g., Inka or Diaguita) (Fuenzalida Reference Fuenzalida2014; Dávila et al. Reference Dávila, Cortés, Mártinez, Hermosilla, Fuenzalida and Pavlovic2018). This suggests the transition to the LP and arrival of Inka influence may have occurred slightly earlier than the commonly used date of ca. cal AD 1450 (or the cal AD 1471 date from Rowe’s chronology of Inka expansion) for the beginning of the LP.
Table 4 Bayesian model of the later phase of use observed at Estadio de Quillota. Amodel=95.7.


Figure 4 Examples of ceramic pieces, two restricted vessels with necks, from Estadio de Quillota demonstrating stylistic differences between Late Intermediate Period ceramics in the Aconcagua Valley and Local Inka Phase ceramics of the Late Period (Photographs by N. Fuenzalida). (a) A Late Intermediate Period Aconcagua vessel with compound profile, spherical body, hyperboloid neck and a lip bonded strap handle from EDQ (ID 252-3) with both morphology and decoration typical of the Aconcagua Culture (Massone Reference Massone1978). The black on Aconcagua salmon decorative motifs correspond to the triangles with tabs of the Aconcagua “trinacrio” design. (b) A Local Inka Phase vessel with compound profile, ellipsoid body, hyperboloid neck and two lip-bonded strap handles from EDQ (ID B1112). The burial which this vessel accompanied (Individual 27, burial 18, EDQ16081) has been directly dated to 1318–1408 cal AD (UGAMS 13205, Fuenzalida, Reference Fuenzalida2014). The vessel has a local (lower course of the Aconcagua) morphology, but decorative features of the Diaguita (colours and bidirectional configuration) and Inka (the oblique reticulated motif arranged on the neck), with a stepped triangle and Greek key motif which is proportionally adapted to the form of the body (González Reference González1995; Fuenzalida-Bahamondes Reference Fuenzalida-Bahamondes2021).
A TL date of AD 1420 ± 45 from an LP vessel with a stepped trichrome pattern (Didier and Ávalos Reference Didier and Ávalos2009a, reported without lab code) falls within the late cluster of new radiocarbon dates from EDQ and is another example of Inka influence appearing in the archaeological record and the transition to the LP occurring slightly before the currently accepted start date of ca. cal AD 1450. There are no further details available regarding the style of pottery found with the other individuals from the later phase of use at EDQ who were also recorded with ceramic grave offerings.
All of the individuals from this phase were buried in an extended position, mostly prone, but two were supine (EDQ16079 and EDQ17011), and one was lying on their left-hand side (EDQ17045). These trends are comparable to descriptions of burial patterns from other Central Chilean sites, in which flexed positions dominate in the ECP, and extended prone positions dominate in the LIP and LP (Saunier and Ávalos Reference Saunier and Ávalos2010).
Diet and Maize at Estadio de Quillota
The stable isotope results demonstrate a strong diachronic shift in dietary practices, particularly regarding the consumption of C4 resources, i.e., maize. The inland location of the site (approximately 25km from the coast and on the inland side of the coastal cordillera) and the low δ15N values indicate terrestrially based diets, with little or no input from marine resources. The wide range of δ13C values (–21.5‰ to –8.4‰) particularly points towards increased inclusion of maize in the diet over time. There is a significant dietary split between the earlier and later phases of the burials dated at EDQ, with the four lower δ13C and δ15N values corresponding to the four earlier dates, and the higher δ13C and δ15N values corresponding to the later cluster of dates (Figure 5).

Figure 5 Stable carbon (δ13C) and nitrogen (δ15N) isotope results of human bone collagen samples from Estadio de Quillota.
The relatively low mean δ13C (X̄ = –21.1‰ ± 0.5) of the earlier group indicates dependence on C3-based resources, with little to no inclusion of maize in the diet. At this time, only curagua, an early endemic variety of maize, is known to have been present in the region (Planella et al. Reference Planella and Belmar2014). The isotopic results show no significant consumption of this or other wild C4 grasses (cf. Cadwallader et al. Reference Cadwallader, Beresford-Jones, Whaley and O’Connell2012). The low mean δ15N value (X̄ = 4.7‰ ± 0.6) suggests a diet that was extremely low in animal proteins, possibly supplemented by the consumption of nitrogen-fixing legumes, which were present in Central Chile in several varieties of beans (Phaseolus spp.) and peanuts (Arachis hypogaea) (Planella and Tagle Reference Planella2004; Pardo and Pizarro Reference Pardo and Pizarro2013). Since these would be expected to have δ15N values near 0‰ (Tieszen and Chapman Reference Tieszen and Chapman1992), their consumption might be masking the contribution of animal protein; even so, it is unlikely to have been high. The isotopic results are similar to those previously observed at three other ECP sites in the inland valley of the Aconcagua Basin: Pocochay, Santa Cruz, and Las Chilcas (δ13C = –19.0‰ ± 1.6, δ15N = 5.4‰ ± 1.0, n = 10) (Swift et al. Reference Swift, Oyanedel Perez and Lopez Labbe2017), and to those more specifically identified as ICC (the initial cultural phase of the ECP) in the Maipo-Mapocho and Rancagua Basins immediately to the south of the study area (δ13C = –20.1‰ ± 0.3, δ15N = 4.5‰ ± 0.7, n = 6) (Falabella et al. Reference Falabella, Planella, Aspillaga, Sanhueza and Tykot2007).
Even though incipient use of C4 resources has been observed at some ECP sites in Santiago and Rancagua—usually those attributed to the Llolleo culture, a period not represented by the radiocarbon dates from Estadio de Quillota—it was not until the LIP and the arrival of the Aconcagua culture that a substantial uptake of maize is observed. This was followed by a marked intensification in the late 14th and early 15th centuries (Falabella et al. Reference Falabella, Sanhueza, Abarca and José Herrera2020), which is contemporary with the later phase of use at EDQ. The elevated δ13C values (X̄ = –11.0‰ ± 1.4) values among the group of 18 individuals from the later phase of EDQ likewise indicate a subsistence strategy that was heavily dependent on C4 resources. The δ15N values for this group (X̄ = 7.5‰ ± 0.8) are also higher than those observed during the earlier phase of use at EDQ. A positive linear relationship between δ13C and δ15N data from EDQ suggests either an increasing inclusion of animal protein in the diet or the manuring of maize (cf. Spzak et al. 2012). The possibility of a marine component is discounted based on the low δ15N values, the site’s distance from the coast, the lack of evidence of fishing technology at the site, and the lack of zooarchaeological evidence of marine fish or shellfish.
There was no significant difference between the δ13C and δ15N results for the individuals accompanied by “Local Inka Phase” Aconcagua pottery at EDQ (n = 8) compared to the other contemporary individuals lacking this affiliation (n = 10) (δ13C t = 0.30, p = 0.76; δ15N t = –0.44, p = 0.67). However, despite the small sample size, there is a significant difference observed between the sexes, with adult males (n = 6) being higher in both isotopes than adult females (n = 6) (δ13C t = –3.35, p = 0.01; δ15N t = –2.63, p = 0.03). This result suggests sex-based differential access to resources, with apparently greater availability of maize products and either higher trophic level protein sources, such as camelids foddered on maize stover, or increased access to fertilized maize crops for adult males when compared to adult females. A similar trend has recently been interpreted as greater consumption of maize-foddered camelids by adult males at sites ascribed to the Aconcagua culture further to the south (Falabella et al. Reference Falabella, Sanhueza, Abarca and José Herrera2020). Taken together, this indicates the possibility of widespread gendered patterns of maize consumption in Central Chile.
Chronology of the Aconcagua Valley
Existing TL and 14C dates for the Aconcagua Valley have been collated in combination with the new dates from EDQ (see supplementary material Appendix A). This collation of dates establishes a sequence of use for the Aconcagua Valley and a span of approximately 7000 years with the earliest date from a coastal shell midden at the site of S-Bato 1 (5561–5474 cal BC; Beta128761 (Seelenfreund and Westfall Reference Seelenfreund and Westfall2000)) to the latest date from the occupation of El Tigre, an Inka site in the Upper Basin (cal AD 1501–1649; UGAMS5980 (Pavlovic et al. Reference Pavlovic, Troncoso, Sánchez and Pascual2012)). The earliest dates predominately come from coastal sites, reflecting a similar pattern of use to that reported in the Maipo-Mapocho and Cachapoal Valleys of Central Chile, particularly in regards to earlier hunter-gatherers utilizing coastal sites and resources (Falabella et al. Reference Falabella, Planella, Aspillaga, Sanhueza and Tykot2007).
The radiocarbon dates from EDQ fall within the constraints of the overall occupation sequence for the Aconcagua Valley. With only six sites in the inland valley of the Aconcagua having either 14C or TL dates, there is a relative dearth of radiometric dates for the inland valley outside those presented here. In fact, there are no radiocarbon dates from any other inland valley sites apart from EDQ.
The Question of the Arrival of the Inka
Bayesian modeling of the radiocarbon dates for the eight identified LP burials at EDQ indicates the timing of the arrival of Inka influence to the Aconcagua Valley (Table 5). The start boundary for this model is cal AD 1290–1400. The end boundary is modeled as cal AD 1410–1470. Querying the chronometric model of these “Local Inka Phase” contexts using OxCal’s “Difference” command demonstrates that Inka influence was likely present at EDQ from 73 to 189 years before cal AD 1471, or from 50 to 160 years before cal AD 1450. The model of Late Period contexts with “Local Inka Phase” ceramics at EDQ accords with previous estimates based on an accumulated probabilities model for arrival of the Inka in northern Chile by the late 14th century AD (Cornejo Reference Cornejo2014).
Table 5 Bayesian model of the radiocarbon dates from identified Late Period burial contexts at EDQ. Amodel = 94.6.

Kernel Density Estimates were generated in OxCal to compare the 14C dates from EDQ’s later phase of use (n = 18), as well as the identified “Local Inka Phase” burials from EDQ (n = 8), with 14C dates from other known Late Period contexts from the Aconcagua Valley (n = 6), and also the 14C dates from other known Late Intermediate Period contexts from the Aconcagua Valley (n = 4) (Appendix B). It is important to note that the sample size for some of the KDEs is very small, particularly for the LIP contexts with only four other 14C dates available for comparison. This will have a large impact on the estimated start and end ranges. By contrast, there are approximately 35 TL dates from LIP contexts. Likewise, for the LP there are six 14C dates and 25 TL dates. However, a different pattern is apparent for the TL dates compared to the 14C dates in both the LIP and LP contexts and indeed there is a statistically significant difference in the median calibrated 14C and TL dates (LIP t = 3.59, p ≤ 0.000; LP t = 3.16, p = 0.004). A recent critique of the reliability of TL vs 14C in the Southern Andes concluded that 14C dates should be used in favor of TL dates to support cultural chronologies (Marsh et al. Reference Marsh, Korpisaari, Mundt, Gasco and Durán2021) and discrepancies have also previously been noted regarding TL dates in Chile (Schiappacasse et al. Reference Schiappacasse, Román, Muñoz, Deza, Focacci and Niemeyer1991; Pavlovic et al. Reference Pavlovic, Troncoso, Sánchez and Pascual2012). For these reasons, only the Aconcagua Valley 14C dates were used in the KDEs produced for direct comparison with KDEs of the 14C dates from EDQ.
These KDEs (Figure 6) indicate that the later phase of use observed at EDQ (start boundary ∼cal AD 1344 (1320–1380, 68.3%) to end ∼cal AD 1441 (1430–1450, 68.3%)), and indeed the burials identified with “Local Inka Phase” material culture (start boundary ∼cal AD 1379 (1370–1400, 68.3%) to end ∼cal AD 1430 (1420–1440, 68.3%)), fall just before the beginning of the known LP dates (start boundary ∼cal AD 1457 (1420–1490, 68.3%)) from the rest of the Aconcagua Valley and towards the end, or later than, the known LIP dates (end boundary ∼cal AD 1399 (1330–1430, 68.3%)) from the rest of the Valley.

Figure 6 Kernel density estimates (KDEs) of the Estadio de Quillota radiocarbon dates from known Late Period contexts (upper middle) and the later use at EDQ (lower middle), compared to KDEs of radiocarbon dates from other Late Period (top) and Late Intermediate Period (bottom) sites or contexts in the Aconcagua Valley. The KDEs are presented in greyscale. The start and end boundaries for each KDE are represented in green and red, respectively (color version available online). Samples sizes of available radiocarbon dates for the Late Period (n=6) and Late Intermediate Period (n=4) in the Aconcagua are both very small, which has an effect of exaggerating the boundaries, and demonstrates a need for further radiometric dating in the region.
The KDE for the “Local Inka Phase” material culture at EDQ indicates that Inka influence had arrived at this southern reach of the empire by the late 14th or early 15th century. The distribution of this KDE falls at the end boundary of the LIP dates for the Aconcagua Valley, and before the start boundary of other LP dates from the Aconcagua Valley. The “Local Inka Phase” material at EDQ likely represents the earlier stages of Inka influence in the Aconcagua Valley. The radiocarbon dates from the other known LP contexts, which are located in the upper basin of the Aconcagua, are likely from a later stage of the imperial expansion in this region being from sites (El Tigre, Los Nogales, and Mt. Aconcagua) with a much more established physical Inka presence. Our findings agree with the recent assertions of Mundt and Marsh (Reference Mundt and Marsh2021) that the Aconcagua may have been an initial nucleus for the spread of Inka influence in Central Chile. However, our data indicates this initial phase was not confined just to the upper basin, but also present in the inland valley at EDQ on the midcourse of the Aconcagua River.
The earlier dates from “Local Inka Phase” contexts at EDQ suggest imperial expansion in this region followed a process of increasing influence and integration over time: an initial phase of interaction, visible through the circulation of ideas, influence, and material culture such as at EDQ, followed by a greater physical presence and occupation as seen at sites such as Mt Aconcagua and El Tigre. There are at least 10 other sites with Inka contexts in the Aconcagua Valley (see Figure 1), but as yet without any radiocarbon dating. Further efforts towards radiocarbon dating of Inka contexts in the Aconcagua Valley would make a beneficial contribution towards refining both the local Central Chilean chronology as well as the timing of Inka expansion towards their southern frontier.
The new radiocarbon dates and chronometric models from EDQ presented here add to the growing evidence that Inka expansion commenced earlier than has been historically documented and provides a strong indication that Imperial influence had already arrived in Central Chile by at least ca. cal AD 1400. The early arrival of Inka influence in Central Chile has important implications, not just for the Empire’s expansion into this region, but also supports an argument that the Imperial phase in Cuzco began earlier than AD 1438, and that the order of the expansion into the different quarters should be reconsidered. The EDQ Late Period model presented here predates the Bayesian model of Inka expansion for the neighbouring Mendoza province on the eastern side of the Andes (boundary start date cal AD 1350–1440) (Marsh et al. Reference Marsh, Kidd, Ogburn and Durán2017). Both the model presented here for EDQ and the previous Marsh et al. (Reference Marsh, Kidd, Ogburn and Durán2017) model from Mendoza, collectively making up a considerable portion of the southern frontier of the Inka Empire, predate the Bayesian model from Chamical in southern Ecuador (boundary start date cal AD 1412–1457), which was part of Chinchasusyu and the northern quarter of the Empire (Ogburn Reference Ogburn2012). In contrast to the sequence of Imperial expansion proposed by the traditional chronologies, a reinterpretation of Inka Imperial chronology based on these Bayesian models of radiometric dates suggests that the southern expansion of the Empire into Collasuyu probably took place before conquering parts of northern Peru and the more resistant and hostile regions of Ecuador in Chinchasuyu.
This new evidence has implications for understanding the nature of Inka control in these regions. The earlier radiometric dates seen from the southern frontier territory, such as the new dates from EDQ, possibly reflect the initial arrival of material culture and ideological influence of the Inka. This initial phase was then followed by an increased investment at a later stage in the Imperial rule, which saw the creation of a more substantial and visible physical presence, in the form of Inka structures, fortifications and an Inka capacocha child sacrifice on Mt Aconcagua. The results presented here support the hypothesis of Ogburn (Reference Ogburn2012) and Marsh et al. (Reference Marsh, Kidd, Ogburn and Durán2017) that the traditional chronology of the Inka Empire, including the sequence of expansion into the four regions of Tawantinsuyu, needs to be reconsidered, and can be usefully investigated with further efforts towards radiometric dating and Bayesian modeling of Inka contexts on the frontiers of the Empire.
CONCLUSION
The new radiocarbon dates presented here place the large pre-Columbian cemetery site of Estadio de Quillota within the chronology of the Aconcagua Valley, which was located on both the southern frontier of intensive maize agriculture and the Inka Empire. The AMS 14C dates on human bone collagen from selected burials indicate that there were two distinct phases of use at EDQ separated by ca. 1000 years: An early phase from 339–196 cal BC to cal AD 128–339, roughly coincident with the Initial Ceramic Communities at the start of the Early Ceramic Period in Central Chile; and a late phase from cal AD 1280–1387 to cal AD 1413–1458 during which time Aconcagua, Diaguita, and Inka cultural influences are observed in the Valley.
The accompanying δ13C and δ15N stable isotopic analysis of the human bone collagen from 22 dated EDQ burials demonstrates a change in diet over time. The wide range of δ13C values point towards a reliance on C3 terrestrial resources during the earlier period of use, around the time of the Initial Ceramic Communities, followed by a heavy dependence on maize-based produce during the later phase of use observed at the EDQ cemetery. Due to the ca. 1000-year gap, such that most of the Early Ceramic Period is unrepresented in the dates from EDQ, it is not possible to give a more precise estimation of when or how quickly this transition in diet and subsistence strategy occurred. However, the isotopic data presented here appear to follow a similar pattern to that which has been observed at sites further to the south where the use of maize was established at some point during the Early Ceramic Period, but more substantial uptake occurred in the Late Intermediate Period and marked intensification followed into the late 14th and early 15th centuries (Falabella et al. Reference Falabella, Sanhueza, Abarca and José Herrera2020), coincident with the findings from the later phase of use at EDQ.
Finally, the dates presented here support growing evidence that the traditional chronology of the Inka Empire, which has been based on historical documentary sources, requires reconsideration. Eight of the radiocarbon dates from the later phase of use at EDQ are from the bone collagen of individuals buried with ceramic items from the Late Period, many of a “Local Inka Phase” style indicative of a process of social transformations having already reached the region. These burials, however, predate the traditionally accepted date of Inka expansion to the south (AD 1471) as well as the date that has recently become more commonly used for the beginning of the Central Chilean Late Period (AD 1450). It is proposed that the expansion may have taken place in two phases: an initial phase of interaction including the circulation of ideas and the emerging influence of a new socio-political context, followed by a second phase of occupation with the construction of architectural complexes and consolidation of the region into the Empire. Bayesian modeling of the dates presented here supports a conclusion that Inka influence had arrived at its southern reaches by the late 14th or early 15th century, providing increasing evidence that the initial phase of Inka influence occurred several decades earlier than the date used traditionally. The findings presented here reinforce calls (cf. Ogburn Reference Ogburn2012; Marsh et al. Reference Marsh, Kidd, Ogburn and Durán2017) to abandon the traditional chronology based upon ethnohistoric sources and consider a major revision of Inka chronology with greater importance placed on evidence from radiometric dating.
ACKNOWLEDGMENTS
We are grateful to the Museo Historico-Arqueologico de Quillota for their support and access to the bioanthropology collection from Estadio de Quillota. Approval for sampling and analysis was provided by Consejo de Monumentos Nacionales de Chile (CMN-ARQUE Nº516/2016). The radiocarbon dates were funded by a grant from the Natural Environment Research Council, UK (NF2017/1/5). We also thank the anonymous reviewers for their constructive comments, which have greatly benefited the paper.
Supplementary Material
To view supplementary material for this article, please visit https://doi.org/10.1017/RDC.2022.59