Introduction
Obsessive-compulsive disorder (OCD) is characterized by intrusive thoughts (obsessions) and repetitive behaviors (compulsions), has a lifetime prevalence of 2–3% in the general population, and is associated with substantial morbidity (Ruscio et al., Reference Ruscio, Stein, Chiu and Kessler2010). It is also a chronic disabling mental disorder, seriously affecting the patient's personal life, and resulting in heavy economic burdens on families and society.
Neurophysiological studies have reported a number of cognitive dysfunction in patients with OCD, including impairment in memory, attention, planning, response inhibition and decision-making (Benzina et al., Reference Benzina, Mallet, Burguiere, N'Diaye and Pelissolo2016). And a growing body of neuroimaging studies have indicated that altered activation in a broad range of brain networks may underlie cognitive impairment and clinical symptoms in OCD (Nakao et al., Reference Nakao, Okada and Kanba2014; Heinzel et al., Reference Heinzel, Kaufmann, Grützmann, Hummel, Klawohn, Riesel, Bey, Lennertz, Wagner and Kathmann2017). Resting-state functional magnetic resonance imaging (rs-fMRI) studies detecting intrinsic activity fluctuations have identified a number of well-defined networks, including the default-mode network (DMN), executive control network (ECN), salience network (SN; Goulden et al., Reference Goulden, Khusnulina, Davis, Bracewell, Bokde, McNulty and Mullins2014), and etc. The DMN includes several brain regions, such as medial prefrontal cortex (mPFC), precuneus, posterior cingulate cortex (PCC) and bilateral inferior parietal lobule (Raichle and Snyder, Reference Raichle and Snyder2007), involved in cognitive functions, including intrinsic stimulus-independent thoughts (Gilbert et al., Reference Gilbert, Dumontheil, Simons, Frith and Burgess2007), autobiographical memory, and monitoring of internal and external environment (Buckner et al., Reference Buckner, Andrews-Hanna and Schacter2008). The ECN, often characterized as a task-positive network, mainly encompasses dorso-lateral prefrontal cortex, posterior parietal cortex, angular gyrus and occipital cortex, and is associated with the performance of cognitively demanding tasks (Sridharan et al., Reference Sridharan, Levitin and Menon2008). A growing number of studies have reported that patients with OCD exhibit altered functional connectivity within and between the DMN and ECN (Peng et al., Reference Peng, Xu and He2014; Chen et al., Reference Chen, Meng, Hu, Cui, Ding, Kang, Juhas, Greenshaw, Zhao, Wang, Cui and Li2016; Fan et al., Reference Fan, Zhong, Gan, Liu, Niu, Liao, Zhang, Yi, Chan, Tan and Zhu2017), which may be associated with impairment of monitoring and goal-directed behavior (Fink et al., Reference Fink, Hendrikx, Stierle, Stengler, Jahn and Exner2017; Vaghi et al., Reference Vaghi, Hampshire, Fineberg, Kaser, Brühl, Sahakian, Chamberlain and Robbins2017). In addition, neuroimaging studies have revealed abnormal activation and connectivity in motor network and frontal-limbic circuits in patients with OCD (Mantovani et al., Reference Mantovani, Rossi, Bassi, Simpson, Fallon and Lisanby2013; de Vries et al., Reference de Vries, de Wit, van den Heuvel, Veltman, Cath, van Balkom and van der Werf2017), associated with inhibition deficits and dysregulation of emotion (Heinzel et al., Reference Heinzel, Kaufmann, Grützmann, Hummel, Klawohn, Riesel, Bey, Lennertz, Wagner and Kathmann2017; Thorsen et al., Reference Thorsen, Hagland, Radua, Mataix-Cols, Kvale, Hansen and van den Heuvel2018) in this condition.
Despite these important findings, previous studies have not yet systematically explored the role of the cerebellum in OCD. Cerebellum is traditionally considered to participate in motor coordination and physical balance (Schmahmann, Reference Schmahmann2004), while evidence from recent animal and human studies has indicated that the cerebellum has reciprocal connections with prefrontal and parietal cortices (Schmahmann and Pandya, Reference Schmahmann and Pandya1989; Middleton and Strick, Reference Middleton2000; Kelly and Strick, Reference Kelly and Strick2003; Ramnani et al., Reference Ramnani, Behrens, Johansen-Berg, Richter, Pinsk, Andersson, Rudebeck, Ciccarelli, Richter, Thompsen, Gross, Robsen, Kastner and Matthews2006), and plays a pivotal role in cognitive and affective processes (Schmahmann, Reference Schmahmann2004). Growing evidence from resting-state functional connectivity (rsFC) studies have indicated that separate regions of the cerebellum are connected to distinct cerebral areas, forming a complex topography function (Habas et al., Reference Habas, Kamdar, Nguyen, Prater, Beckmann, Menon and Greicius2009; Krienen and Buckner, Reference Krienen and Buckner2009). Segregated cerebral-cerebellar loops may mediate executive control, effective, default-mode, and sensorimotor function (Habas et al., Reference Habas, Kamdar, Nguyen, Prater, Beckmann, Menon and Greicius2009; Krienen and Buckner, Reference Krienen and Buckner2009). Recent studies have indicated that altered cerebro-cerebellar functional connectivity is associated with several psychiatric disorders, including autism spectrum disorder (Khan et al., Reference Khan, Nair and Keown2015), schizophrenia (Guo et al., Reference Guo, Liu, Zhang and Zhao2015), and major depressive disorder (MDD; Guo et al., Reference Guo, Liu, Xue, Gao, Liu, Xiao, Chen and Zhao2013). Moreover, altered functional connectivity between the cerebellum and the cognitive and affective cortex are correlated with the severity of symptom in MDD (Alalade et al., Reference Alalade, Denny, Potter, Steffens and Wang2011) and cognitive impairment in seasonal affective disorder (Yuan et al., Reference Yuan, Meng, Zhang, Nie, Ren, Zhu, Li, Lui, Gong, Qiu and Zhang2017).
There has, however, been relatively little attention to cerebellar-cerebral functional connectivity in OCD. This is an important question given the growing evidence from brain imaging studies for a role of the cerebellum in OCD. A number of publications have indicated alterations in cerebellar structure (Pujol et al., Reference Pujol, Soriano-Mas and Alonso2004; Nakao et al., Reference Nakao, Nakagawa, Yoshiura, Nakatani, Nabeyama, Yoshizato, Kudoh, Tada, Yoshioka, Kawamoto, Togao and Kanba2005; Nabeyama et al., Reference Nabeyama, Nakagawa, Yoshiura, Nakao, Nakatani, Togao, Yoshizato, Yoshioka, Mayumi and Kanba2008; de wit et al., Reference de wit, Alonso and Schweren2014; Narayanaswamy et al., Reference Narayanaswamy, Jose, Kalmady, Agarwal, Venkatasubramanian and Janardhan Reddy2016) or function (Nabeyama et al., Reference Nabeyama, Nakagawa, Yoshiura, Nakao, Nakatani, Togao, Yoshizato, Yoshioka, Mayumi and Kanba2008; Ping, Reference Ping2013; Nakao et al., Reference Nakao, Okada and Kanba2014) in this condition, including decreased right cerebellar tonsil activation (Nabeyama et al., Reference Nabeyama, Nakagawa, Yoshiura, Nakao, Nakatani, Togao, Yoshizato, Yoshioka, Mayumi and Kanba2008), reduced cerebellar volume (Narayanaswamy et al., Reference Narayanaswamy, Jose, Kalmady, Agarwal, Venkatasubramanian and Janardhan Reddy2016), increased bilateral cerebellar region homogeneity in resting-state and the inverse correlation with the severity of compulsion in patients with OCD (Ping, Reference Ping2013). Given the cognitive impairment, dysregulation of emotion and behavioral dysfunction (Heinzel et al., Reference Heinzel, Kaufmann, Grützmann, Hummel, Klawohn, Riesel, Bey, Lennertz, Wagner and Kathmann2017; Thorsen et al., Reference Thorsen, Hagland, Radua, Mataix-Cols, Kvale, Hansen and van den Heuvel2018), combined with alterations in a broad range of brain networks in OCD (Fan et al., Reference Fan, Zhong, Gan, Liu, Niu, Liao, Zhang, Yi, Chan, Tan and Zhu2017; Posner et al., Reference Posner, Song, Lee, Rodriguez, Moore, Marsh and Blair Simpson2017), we therefore compared cerebellar-cerebral functional connectivity, with a focus on executive control, effective, default-mode, and sensorimotor networks in OCD patients and healthy controls (HCs), using seeds in the cerebellum that have been suggested to be involved in multiple functions (Habas et al., Reference Habas, Kamdar, Nguyen, Prater, Beckmann, Menon and Greicius2009; Krienen and Buckner, Reference Krienen and Buckner2009). We hypothesized (1) that OCD patients would exhibit disrupted functional connectivity in cerebellar-cerebral networks that mediate executive control, effective regulation, default-mode, and sensorimotor function and (2) that altered cerebellar-cerebral functional connectivity would be associated with clinical symptoms.
Materials and methods
Subjects
Twenty-seven right-handed adult patients with OCD were recruited from the Shanghai Mental Health Center, Shanghai, China, between June 2016 and April 2017. All patients were diagnosed by a psychiatrist according to DSM-IV criteria. Of the 27 OCD patients, 14 were drug-naïve and 13 were medication-free (patients who discontinued medication for at least 8 weeks were considered medication-free), the medication-free patients mainly had received treatment with selective serotonin reuptake inhibitors, and had to stop the medication by themselves for different reasons, with the obsessive-compulsive symptom still exists or relapse when the study is recruited. There are no any significant differences in age, gender, education level, Y-BOCS score, illness duration and mean frame-wise displacement between the drug-naïve and the medication-free patients (see Table 1 in Supplement). The exclusion criteria for the OCD group included any axis I psychiatric disorder comorbidity. Twenty-one HCs were recruited from the community, and matched for age, gender, handedness, and education, individuals who had any psychiatric history or family history were excluded. Additional exclusion criteria for all participants were a history of drug or alcohol abuse, craniocerebral trauma, serious physical illness, pregnancy, and contraindications to MRI. Individuals younger than 18 years or older than 65 years were also excluded.
Clinical measures
The severity of OCD symptoms was assessed using the Yale-Brown Obsessive-Compulsive Scale (Y-BOCS; Goodman et al., Reference Goodman, Price, Rasmussen, Mazure, Delgado, Heninger and Charney1989). To be eligible for the study, patients were required to have a Y-BOCS total score of ⩾16. Current symptoms of depression and anxiety were assessed using the Beck Depression Inventory-second edition (BDI-II; Beck et al., Reference Beck, Steer, Ball and Ranieri1996) and the Beck Anxiety Inventory (BAI; Steer et al., Reference Steer, Rissmiller, Ranieri and Beck1993), respectively. All participants were screened with the Mini-International Neuropsychiatric Interview (M.I.N.I.; Sheehan et al., Reference Sheehan, Lecrubier, Sheehan, Amorim, Janavs, Weiller, Hergueta, Baker and Dunbar1998) to ascertain that there was no history of a DSM-IV psychiatric disorder.
Image acquisition
MRI scans were obtained with a 3.0 T Verio scanner (Siemens, Erlangen, Germany) with a 32-channel phased-array head coil. During the MRI scans, all participants were instructed to keep their eyes closed, to relax but not fall asleep, and to lie still without moving. Three-dimensional T1-weighted images were acquired by employing a 3D-MPRAGE sequence with the following parameters: time repetition (TR) = 2300 ms, time echo (TE) = 3.5 ms, flip angle = 9°, matrix size = 64 × 64, field of view (FOV) = 256 mm, 192 sagittal slices, slice thickness = 1 mm, acquisition voxel size = 1 × 1 × 1 mm3. After structural MRI scannings, resting-state fMRI scans were acquired with the following parameters: TR = 2000 ms, TE = 30 ms, FOV = 220 mm, flip angle = 77°, matrix size = 64 × 64, voxel size = 3.0 × 3.0 × 3.0 mm3, 50 axial slices with a slice thickness of 3 mm and no slice gap, the fMRI scanning lasted for 480 seconds, and 240 volumes were obtained.
Data processing
MRI data analysis was carried out using the standard pipeline integrating SPM8 (http://www.fil.ion.ucl.ac.uk/spm/software/spm8) and REST (http://restfmri.net/forum/REST_V1.8) as implemented in Data Processing & Analysis for Brain Imaging (DPABI_v2.3) (Yan et al., Reference Yan, Wang, Zuo and Zang2016). For image preprocessing, the first 10 volumes were removed for steady-state magnetization. The remaining 230 volumes were corrected for slice timing and realigned for head motion correction. Then the participants’ structural images were manually co-registered and realigned, and subsequently co-registered to the mean functional image, and segmented as grey matter, white matter, and cerebrospinal fluid. The head motion of all participants during resting-state fMRI acquisition was examined, no participants had more than 1.5° of maximal rotation and 1.5 mm of maximal translation. Six head motion parameters, the average signals from cerebrospinal fluid and white matter were regressed in first-level analysis, we also calculated the mean frame-wise displacement (FD) of each participant (Power et al., Reference Power, Barnes, Snyder, Schlaggar and Petersen2012) and took it as a confounding variable in subsequent analysis (Yan et al., Reference Yan, Cheung and Kelly2013; eMethods in the Supplement). Next, all functional data were normalized to Montreal Neurological Institute (MNI) space and the processed images were spatially smoothed with a 4 mm full width at the half maximum Gaussian kernel. Further preprocessing included removing linear trends and temporal band-pass filtering (0.01–0.08 Hz) to reduce the effects of low-frequency drift and high-frequency physiologic noise.
To identify cerebellar-cerebral functional networks, seed-based correlation analyses were carried out by using regions of interest (ROIs) in the cerebellum. Previous studies (Habas et al., Reference Habas, Kamdar, Nguyen, Prater, Beckmann, Menon and Greicius2009; Krienen and Buckner, Reference Krienen and Buckner2009) have found that cerebellum seeds are able to identify cerebellar-cerebral executive control, default-mode, affective-limbic, and motor networks, and we used these seeds here (Table 1) (Krienen and Buckner, Reference Krienen and Buckner2009). A 6 mm radius sphere was drawn from each center point as an ROI. Pearson correlation coefficients were computed between the seeds and the voxels of the whole brain to create the correlation maps for each seed and each participant. Finally, the correlation maps were z-transformed with Fisher's r-to-z transformation to improve the Gaussianity of their distribution.
Table 1. Cerebellar seeds and coordinates grouped by network

R, right, L, left. Exec, executive; DMN, default-mode network; Aff, affective; Mot, motor.
Statistical analysis
We compared distributions of age, gender and years of education across the groups, using t tests and Chi-square tests, as appropriate. To verify the results of previous studies on the cerebellar topologies of different networks in HC group (Habas et al., Reference Habas, Kamdar, Nguyen, Prater, Beckmann, Menon and Greicius2009; Krienen and Buckner, Reference Krienen and Buckner2009), and to further explore the spatial topography of cerebellar-cerebral functional connectivity in OCD group, one-sample t tests were conducted to explore the spatial topography of cerebellar-cerebral functional connectivity in HC and OCD group, separately. Multiple comparisons for the one-sample t tests were corrected by Monto Carlo stimulations with a combined individual voxel threshold at p < 0.001 and the required cluster size corresponded to the Alphasim correction of p < 0.05 (cluster size >50 voxels) (http://afni.nih.gov/afni/docpdf/AlphaSim.pdf), calculated using DPABI (Yan et al., Reference Yan, Wang, Zuo and Zang2016). We then conducted a two-sample t test to compare differences in cerebellar-cerebral functional connectivity in OCD group relative to HCs, using an explicit mask made by DPABI (Yan et al., Reference Yan, Wang, Zuo and Zang2016; eMethods in Supplement for details). The significance level was set at the individual voxel p < 0.005 with Alphasim cluster correction of p < 0.05 (cluster size >90 voxels).
Mean z scores were extracted from connected cerebral regions which showed significant differences in the OCD group relative to the HC group, and correlation analyses were conducted to examine the relation between mean z values and severity of OCD symptoms, with significance level set at p < 0.05.
Post-hoc analysis was performed on brain regions showing significant differences in functional connectivity between patients and controls. We used G × Power to calculate Cohen's d, which was applied to determine the robustness of findings (Cohen, 1977). Effect sizes were considered as small with d value below 0.2, medium with d value between 0.2 and 0.5, and large with d value above 0.8.
Results
Subjects
Demographic and clinical characteristics of the participants are listed in Table 2. There was no significant difference between the OCD group and the control group in age, gender or years of education. The mean (s.d.) Y-BOCS total score for the OCD group was 28.6 (s.d. = 4.3), and depression and anxiety scores were significantly higher in the OCD group than in the control group (two sample t test, both p < 0.001).
Table 2. Participant demographic and clinical features

FD, frame-wise displacement; Y-BOCS, Yale-Brown Obsessive-Compulsive Scale; BDI-II, Beck Depression Inventory-second edition; BAI, Beck Anxiety Inventory.
Cerebellar-cerebral functional connectivity in HC group
In the HCs group, we found that cerebellar seeds had functional connectivity with distributed cerebral areas (Fig. 1). When we used a more conservative threshold of p < 0.0001 (with Alphasim correction of p < 0.05), good specificity for cerebral-cerebellar functional connectivity was seen (Fig. 2). We found significant connectivity in (1) an executive network (including middle frontal cortex, inferior parietal cortex, temporal cortex, and thalamus; Figure 2); (2) an effective regulation network (including inferior parietal cortex, superior frontal gyrus, middle frontal gyrus, caudate and precuneus) (Fig. 2); (3) the DMN (including mPFC, PCC, precuneus and bilateral parietal lobe; Fig. 2); and (4) a sensorimotor network (including supplementary motor area, parietal lobe, lingual gyrus and occipital lobe) (Fig. 2).
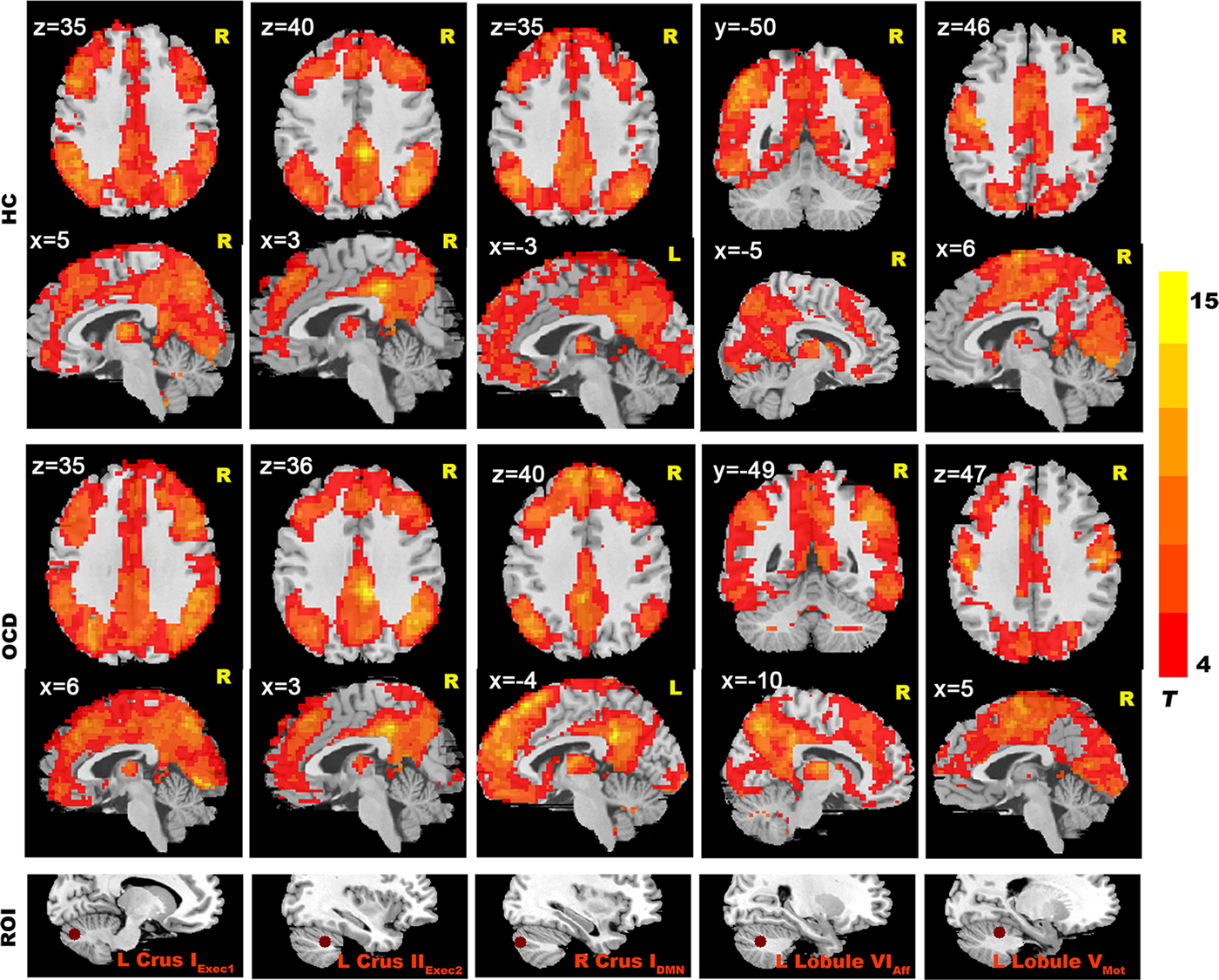
Fig. 1. Resting-state cerebellar-cerebral functional connectivity in the OCD group vs. HC group (p < 0.001).

Fig. 2. Resting-state cerebellar-cerebral functional connectivity in the OCD group vs. the HC group (p < 0.0001). The seed regions shown here include: left Crus IExec1, left Crus IIExec2, right Crus IDMN, left Lobule VIAff, left Lobule VMot.
In the OCD group, we also found significant functional connectivity in multiple cerebellar-cerebral network, and the OCD group showed similar cerebellar-cerebral connectivity patterns with the HC group (Fig. 2 and eResults in the Supplement).
Cerebellar-cerebral functional connectivity in OCD group relative to HC group
Relative to HCs, patients with OCD showed significantly decreased functional connectivity in cerebellar-cerebral networks mediating executive, affective-limbic and motor functions (Table 3).
Table 3. Brain regions showing decreased cerebellar-cerebral FC in the OCD group compared with the HC group

Exec, executive control network; DMN, default-mode network; Aff, affective; Mot, motor network.
First, for the executive network, patients with OCD had reduced functional connectivity between (1) the bilateral Crus IExec1 and the fusiform, lingual gyrus, temporal lobe, occipital lobe, inferior parietal lobule and postcentral gyrus, (2) the bilateral Crus IIExec2 and the superior occipital gyrus, lingual gyrus and parahippocampal gyrus, and (3) the right Lobule VI Exec3 and bilateral inferior temporal gyrus and right inferior parietal gyrus (Fig. 3).

Fig. 3. Decreased cerebral-cerebellar functional connectivity in patients with OCD relative to HCs. The widths of the line are proportional to the t values. MTG = Middle Temporal Gyrus; ITG = Inferior Temporal Gyrus; LG = Lingual Gyrus; Parahippo = Parahippocampal Gyrus; Fus = Fusiform; SFG = Superior Frontal Gyrus; Pcu = Precuneus; IPL = Inferior Parietal Lobule; SOG = Superior Occipital Gyrus.
Second, for the effective network, patients with OCD showed decreased functional connectivity between the right lobule VIAff and parahippocampal gyrus and middle temporal gyrus, between the left lobule VIAff and lingual gyrus, and between the VermisLimbic and the fusiform gyrus (Fig. 3).
Third, for the sensorimotor network, patients with OCD had reduced functional connectivity between the right lobule VMot and the superior frontal gyrus (Fig. 3).
Potential confounders
Decreased cerebellar-cerebral functional connectivity in executive, affective-limbic and sensorimotor networks in OCD remained significant after adjusting for age and head motion. In addition, we also found decreased cerebellar functional connectivity with cingulate and thalamus, and for the DMN, patients with OCD had reduced cerebellar functional connectivity with temporal gyrus (eResults and eTable 2 in the Supplement).
Correlation between cerebellar-cerebral FC and clinical features of OCD
Within the OCD group, connectivity of the right Crus IExec1-inferior parietal lobule was positively correlated with symptom severity of OCD measured by Y-BOCS score (r = 0.422, p = 0.028 uncorrected;) (Fig. 4a), further analysis showed that the main contribution was from the compulsive subscale score (r = 0.427, p = 0.026 uncorrected); furthermore, within OCD group, connectivity of the left lobule VIAff-lingual gyrus coupling was negatively correlated with illness duration (r = −0.631, p < 0.001 uncorrected; p = 0.04 Bonferroni corrected) (Fig. 4b).

Fig. 4. Correlation between the altered cerebellar-cerebral functional connectivity with clinical features of OCD: (a) significant positive correlation of decreased right Crus IExec1-inferior parietal lobule connectivity with the severity of obsessive-compulsive symptoms; (b) significant negative correlation of decreased left lobule VIAff–lingual gyrus connectivity with illness duration. Note: rsFC = resting-state functional connectivity; Y-BOCS = Yale-Brown Obsessive-Compulsive Scale; L = left; R = right.
Discussion
To the best of our knowledge, this study is the first to explore resting-state cerebellar-cerebral functional connectivity in OCD. We found decreased cerebellar-cerebral functional connectivity in OCD including in executive, default-mode, affective-limbic, and sensorimotor networks. Furthermore, within the OCD group, functional connectivity in an executive network spanning the right Crus IExec1 and the inferior parietal lobule was positively correlated with OCD symptom severity, and functional connectivity in an affective-limbic network spanning the left lobule VIAff and lingual gyrus was negatively correlated with illness duration, consistent with involvement of these networks in the pathophysiology of OCD (Chen et al., Reference Chen, Meng, Hu, Cui, Ding, Kang, Juhas, Greenshaw, Zhao, Wang, Cui and Li2016; de Vries et al., Reference de Vries, de Wit, van den Heuvel, Veltman, Cath, van Balkom and van der Werf2017).
The present study demonstrating that segregated cerebellar-cerebral connectivity exists both in HCs and OCD patients provides a partial replication of previous studies (Habas et al., Reference Habas, Kamdar, Nguyen, Prater, Beckmann, Menon and Greicius2009; Krienen and Buckner, Reference Krienen and Buckner2009). This work is in turn consistent with the increased recognition of the involvement of the cerebellum in emotional and cognitive processes mediated by a broad range of cerebellar-cerebral networks (Schmahmann, Reference Schmahmann2004; Stoodley and Schmahmann, Reference Stoodley and Schmahmann2010). Findings of altered cerebellar-cerebral functional connectivity in OCD patients may well underlie cognitive and affective dysfunctions in this condition.
Decreased connectivity of the cerebellum-cerebral executive network in OCD
We found lower functional connectivity of the cerebellum with a cerebral executive network in OCD. Previous work has reported that the executive network is strongly involved in higher cognitive function, including attention control, planning and decision-making (Chamberlain et al., Reference Chamberlain, Fineberg, Blackwell, Robbins and Sahakian2006; Sylvester et al., Reference Sylvester, Corbetta, Raichle, Rodebaugh, Schlaggar, Sheline, Zorumski and Lenze2012; Dong et al., Reference Dong, Lin and Potenza2015). Functional abnormality of this network has been reported in patients with OCD (Chen et al., Reference Chen, Meng, Hu, Cui, Ding, Kang, Juhas, Greenshaw, Zhao, Wang, Cui and Li2016), which may lead to a dysfunctional strategies for coping with threat and uncertainty (Gottlich et al., Reference Gottlich, Kramer, Kordon, Hohagen and Zurowski2014), related to the clinical symptoms of OCD, such as excessive monitoring of intrusive thoughts and behavior. Peterburs and Desmond (Reference Peterburs and Desmond2016) indicated that the cerebellum contributes to performance monitoring, a set of cognitive and affective functions underlying adaptive behavior, and altered functional connectivity of the cerebellum-cerebral executive network has associated with anxiety vulnerability (Caulfield et al., Reference Caulfield, Zhu, McAuley and Servatius2016). Thus lower the cerebellar-cerebral ECN connectivity may reflect abnormal external monitoring, with excessive attention to unrelated external stimulation, which subsequently evokes excessive anxiety in OCD, it may explain why patients must exert great effort to resist the obsessive–compulsive urges. Furthermore, the right Crus IExec1-inferior parietal lobule coupling was positively correlated with symptom severity of OCD (mainly compulsive symptom). Parietal cortex is an important part of the executive network, and is involved in attention and response inhibition (Dong et al., Reference Dong, Lin and Potenza2015), we hypothesize that decreased functional connectivity between the cerebellum and inferior parietal lobule may underlie inhibitory impairment, resulting in excessive repetitive behavior in OCD.
Decreased connectivity of the cerebellum-cerebral sensorimotor network in OCD
In addition, compared with HCs, patients with OCD showed significantly decreased functional connectivity between the cerebellum and sensory cortex (including superior occipital gyrus, inferior occipital gyrus, and inferior temporal gyrus). This may reflect aberrant information transition between these brain regions, associated with excessive sensitivity to external stimulation (Andreasen and Pierson, Reference Andreasen and Pierson2008). Neurophysiological studies in OCD have revealed that abnormal activation of temporal gyrus is associated with dysfunctions in early inhibitory control processes and difficulties inhibiting task-irrelevant information (Wolff et al., Reference Wolff, Buse, Tost, Roessner and Beste2017). Thus altered connectivity between the cerebellum and temporal gyrus might result in failure to suppress irrelevant information, associated with the rigid and repetitive thoughts in OCD.
In addition, there was decreased functional connectivity between the sensorimotor area of the cerebellum and the superior frontal gyrus in OCD patients relative to HCs in this study. Cerebellar activation has been shown to contribute to decreased activation of the sensorimotor cortex during self-produced tactile stimulation (Blakemore et al., Reference Blakemore, Frith and Wolpert1999). In line with cerebellar involvement in sensory prediction (Jutta et al., 2016), altered cerebellar-cerebral connectivity in the sensorimotor network in OCD might be consistent with alterations in visuospatial processing and perception in this disorder (Wolff et al., Reference Wolff, Buse, Tost, Roessner and Beste2017; Geller et al., Reference Geller, Abramovitch, Mittelman, Stark, Ramsey, Cooperman, Baer and Stewart2018).
We also found the decreased functional connectivity of left lobule VIAff-lingual gyrus coupling in patients with OCD, which was negatively correlated with illness duration. Neuroimaging studies have pointed to the role of lingual gyrus in processing emotional related visual stimuli (Lane et al., Reference Lane, Chua and Dolan1999). In light of the functional role of the lingual gyrus, we hypothesis that our observation relates to the phenomenology of OCD, whereby specific visual stimuli, such as messy scenes or images that may induce contamination or check related behaviors. Furthermore, the correlation of cerebellar-lingual gyrus coupling with the illness duration is perhaps consistent with previous work indicating that thickness and surface area of lingual gyrus are negatively correlated with the illness severity in OCD (Venkatasubramanian et al., Reference Venkatasubramanian, Zutshi, Jindal, Srikanth, Kovoor, Kumar and Janardhan Reddy2012).
Decreased connectivity of the cerebellum-cerebral affective-limbic network in OCD
We also found the decreased functional connectivity between the cerebellum and emotion processing (limbic) regions (including parahippocampal gyrus and cingulate gyrus) in OCD patients compared with HCs. The limbic system is involved in social cognition and emotional regulation, the parahippocampal gyrus is supposed to involved in episodic memory, and the cingulate gyrus participates in the regulation of emotion and social cognition (Campos et al., Reference Campos, Santos, Gagen, Machado, Rocha, Kurtz and Rocha2016). A meta-analysis concludes that the involvement of the cerebellum in social cognition depends on its functional connectivity with the cerebrum (Van Overwalle et al., Reference Van Overwalle, D'aes and Mariën2015). Moreover, cerebellar stimulation can alter limbic function and elicit behaviors like grooming (Reis et al., Reference Reis, Doba and Nathan1973), thus we hypotheses that decreased connectivity between the cerebellum and limbic system might underlie the phenomenology and dysregulation of emotion and cognition in OCD (Paul et al., Reference Paul, Simon, Endrass and Kathmann2016).
Decreased connectivity of the cerebellum-cerebral DMN in OCD
Additionally, we found decreased functional connectivity between the cerebellum and the DMN (including precuneus, cingulate gyrus, and superior temporal gyrus; eTable2 in Supplement). The DMN has been supposed to play an important role in self-referential, internal-directed attention (Gilbert et al., Reference Gilbert, Dumontheil, Simons, Frith and Burgess2007), while the executive network participates in external-stimuli related attention (Sridharan et al., Reference Sridharan, Levitin and Menon2008; Sylvester et al., Reference Sylvester, Corbetta, Raichle, Rodebaugh, Schlaggar, Sheline, Zorumski and Lenze2012). Cognitive behavior therapy may improve the reduced negative relationship between the DMN and the executive network revealed by previous research (Stern et al., Reference Stern, Fitzgerald, Welsh, Abelson and Taylor2012), suggesting that there are competitive relationships between these two networks. Our findings of decreased connectivity between cerebellum and both DMN and executive networks might underlie the difficult to ‘switch off’ when attention was needed to direct to external stimuli in a cognitive task, which in turn may lead to difficulties in separating from internally generated intrusive thoughts, and the thought-action fusion, that is, associated with obsessive symptom.
The present study reported altered cerebellar-cerebral connectivity in a broad of different networks in patients with OCD relative to HCs. However, we failed to identify any differences in cerebellar-striatum or cerebellar-amygdala connectivity in OCD. There are several possible reasons: on the one hand, the reproducibility of neuroimaging findings may decrease due to intrinsically low statistical power of the relatively small sample size; on the other hand, this study used the whole-brain voxel-wise analysis to compare group difference of the cerebellar-cerebral connectivity, increased number of multiple comparisons may mask the results of brain regions that contain few voxels. The question of cerebellum connectivity with striatum and amygdala in OCD should be further explored in future studies, with a more specific assumption and larger sample sizes.
A number of limitations of our study deserve emphasis. First, our sample size was relatively small, which may have limited our ability to find additional differences across groups, and increase the instability of the results. Second, due to the lack of objective measures of cognitive function in the present study, definitive conclusions about the relationship between functional connectivity and cognitive dysfunction cannot be drawn. Further work using neuropsychological testing and task-based fMRI is needed to confirm the hypotheses formulated here. Third, the above results and discussion are based on data processed without global signal regression, because the global signal may remove true blood oxygen dependence level (BOLD) signal and potentially alter group differences in functional connectivity (Saad et al., Reference Saad, Gotts, Murphy, Chen, Jo, Martin and Cox2012). Finally, we did not apply a statistical correction for the number of separate tests (13 ROIs), given that this was an exploratory study.
Conclusion
In conclusion, our study reports altered functional connectivity between the cerebellum and distributed cerebral regions in patients with OCD. These data prompt the hypothesis that a range of cortical-cerebellar networks may be involved in the pathophysiology of OCD, and provides a new direction for the study of OCD. However, the exact role of cerebellar-cerebral networks in the pathogenesis and development of OCD are not yet fully delineated. Future research, including neuropsychological testing, and focused on changes of functional connectivity in cerebellum-cerebral networks during treatment may be useful in further expanding this model of OCD, and in determining whether it has clinical utility.
Supplementary material
The supplementary material for this article can be found at https://doi.org/10.1017/S0033291718001915
Acknowledgements
This work was supported by National Natural Science Foundation of China (81671340); Shanghai Municipal Education Commission-Gao Feng Clinical Medicine Grant (20161321); Municipal Human Resources Development Program for Outstanding Leaders in Medical Disciplines in Shanghai (2017BR058); and the Shanghai Key Laboratory of Psychotic Disorders (No.13dz2260500). The authors report no financial relationships with commercial interests.
Declaration of interest
None.
Ethical standards
The authors note that all procedures contributing to this work comply with the ethical standards of the relevant national and institutional committees on human experimentation and with the Helsinki Declaration of 1975, as revised in 2008.