Introduction
Estuaries are very productive ecosystems, considered nursery areas for marine and estuarine fish and invertebrates (e.g. the blue crab Callinectes sapidus Rathbun, 1896). Within estuaries, shallow-water habitats such as seagrass beds, oyster reefs and salt marshes are recognized to enhance the survival, movement and feeding rates of recruits, so are vital habitats for nursery areas (Minello et al., Reference Minello, Able, Weinstein and Hays2003; Lipicius et al., Reference Lipcius, Seitz, Seebo and Colon-Carrion2005; Johnson & Eggleston, Reference Johnson and Eggleston2010).
Callinectes sapidus is the dominant and most abundant swimming crab in South Brazilian estuaries. Its original disjunct distribution in the western Atlantic ranges from the USA to Venezuela and from Brazil to Argentina (Williams, Reference Williams1974). There are growing reports of new occurrences of the blue crab in Europe, Asia and Africa, probably due to larval introduction through ballast waters (Galil, Reference Galil2000; Nehring, Reference Nehring, Galil, Clark and Carlton2011; Carrozzo et al., Reference Carrozzo, Potenza, Carlino, Costantini, Rossi and Mancinelli2014; Mancinelli et al., Reference Mancinelli, Chainho, Cilenti, Falcod, Kapiris, Katselis and Ribeiro2017; Garcia et al., Reference Garcia, Pinya, Colomar, París, Puig, Rebassa and Mayol2018).
The blue crab is an important ecological and commercial species. It plays a fundamental role in the structure and function of coastal benthic food webs, either as a keystone species or by inducing trophic cascades (Hines, Reference Hines, Kenney and Cronin2007). It is recognized as eurythermal and euryhaline with high fecundity, strong swimming capacity and a pronounced aggressiveness (Rodrigues & D'Incao, Reference Rodrigues and D'Incao2014; Garcia et al., Reference Garcia, Pinya, Colomar, París, Puig, Rebassa and Mayol2018). Callinectes sapidus is considered an estuarine-dependent species (Williams, Reference Williams1974), because the adults inhabit estuarine zones (Yeager et al., Reference Yeager, Krebs, Mcivor and Brame2007), while the larvae develop in oceanic waters (Epifanio, Reference Epifanio, Kennedy and Cronin2007). Its life cycle begins inside estuaries when mating occurs, and it is tightly controlled by the moult cycle, and consequently, by temperature (Hines et al., Reference Hines, Lipicius and Haddon1987). Then, males migrate to the upper estuary while inseminated females descend to the high salinity waters of the estuary mouth and adjacent shelf areas for egg release (Hines et al., Reference Hines, Lipicius and Haddon1987; Aguilar et al., Reference Aguilar, Hines, Wolcott, Wolcott, Kramer and Lipcius2005; Rodrigues & D'Incao, Reference Rodrigues and D'Incao2014). Larvae develop in the marine plankton and by selective use of tidal-streams, and sensory cues are transported back to the estuaries when they reach the megalopa stage and settle preferentially into vegetated areas (Tankersley & Forward, Reference Tankersley, Forward, Kennedy and Cronin2007).
The growth, development and reproduction of crustaceans depends on ecdysis (or moulting), indicating that this is a critical biological process that occurs several times throughout the life-cycle (Chang & Mykles, Reference Chang and Mykles2011; Huang et al., Reference Huang, Wang, Yue, Chen, Gaughan, Lu, Lu and Wang2015). In the moulting process, individuals become vulnerable to predation due to difficulties in feeding and movement (Kennedy & Cronin, Reference Kennedy and Cronin2007), and also because visual acuity decreases substantially in the days before ecdysis (Baldwin & Johnsen, Reference Baldwin and Johnsen2011). Therefore, they need to search for refuge in this period, and it has been observed that crabs segregate in estuaries by moulting stage, with higher numbers of pre-moult males in the estuary head and bigger post-moult males and mature females in the mouth of the estuary (Hines et al., Reference Hines, Lipicius and Haddon1987; Posey et al., Reference Posey, Alphin, Harwell and Allen2005; Ruas et al., Reference Ruas, Rodrigues, Dumont and D'Incao2014).
Considering the commercial importance of the blue crab, global catches of ~74,357 tons were reported in 2014, with the USA as the country with the most significant contribution (FAO, 2019). These crabs are known as a by-catch of several fisheries but are the target of artisanal fishers (Mendonça et al., Reference Mendonça, Verani and Nordi2010). In Brazil, the catch per unit effort (CPUE) of this species was estimated to be 4.0 kg h−1 in 2005, making C. sapidus the most exploited portunid species in the country (Mendonça et al., Reference Mendonça, Verani and Nordi2010). In addition, this species is profoundly affected by artisanal trawl fisheries along the Brazilian coast, and their economic use depends on local culture, the magnitude of other fisheries and the seasonality of the market (Graça-Lopes et al., Reference Graça-Lopes, Puzzi, Severino-Rodrigues, Bartolotto, Guerra and Figueiredo2002; Severino-Rodrigues et al., Reference Severino-Rodrigues, Guerra and Graça-Lopes2002; Branco & Fracasso, Reference Branco and Fracasso2004; Dumont & D'Incao, Reference Dumont and D'Incao2011; Tudesco et al., Reference Tudesco, Fernandes and Di Beneditto2012). The few records available for blue crab catch in the Patos Lagoon (Southern Brazil) show annual productivity of 400 tons per year, with maximum values recorded of ~1600 tons per year (Kalikoski & Vasconcellos, Reference Kalikoski and Vasconcellos2013). Values from 92.25 ± 49.59 to 125 ± 28.65 g h−1 have been reported as by-catch of shrimp fisheries with fyke nets, with highest catches in embayments from the mid to the upper estuary (Ruas et al., Reference Ruas, Becker and D'Incao2017). However, recent research using local ecological knowledge revealed that largest CPUEs of blue crab decreased from ~10 kg net metre−1 day−1 in the 1980s to 2 kg net metre−1 day−1 in 2018 (Thykjaer et al., Reference Thykjaer, Rodrigues, Haimovici and Cardoso2019).
Blue crab commercial use has stimulated a high number of studies about species biology, physiology and distribution, mainly in the Western North Atlantic (e.g. Pile et al., Reference Pile, Lipcius, van Montfrans and Orth1996; Heck et al., Reference Heck, Coen and Morgan2001; Posey et al., Reference Posey, Alphin, Harwell and Allen2005). Despite its economic importance in Brazil, few studies concerning the ecology and population dynamics of C. sapidus have been published, and most information is available in the form of grey literature. However, such studies are essential for establishing management strategies of the blue crab fishery stocks.
Studies on the Patos Lagoon estuary have investigated C. sapidus juvenile development (Barutot et al., Reference Barutot, Vieira and Rieger2001), diet (Oliveira et al., Reference Oliveira, Pinto, Santos and D'Incao2006; Ferreira et al., Reference Ferreira, Barros, Barutot and D´Incao2011), reproductive biology (Rodrigues & D'Incao, Reference Rodrigues and D'Incao2014), influence of salinity and seagrass meadows on spatial distribution (Ruas et al., Reference Ruas, Rodrigues, Dumont and D'Incao2014), population connectivity with other estuaries of South Brazil (Lacerda et al., Reference Lacerda, Kersanach, Cortinhas, Prata, Dumont, Proietti, Maggioni and D'Incao2016) and the distribution of recruits (Rodrigues et al., Reference Rodrigues, Ortega and D'Incao2019); but some further aspects still remain to be detailed. These studies concluded that there is a high connectivity among blue crab populations of the south-western Atlantic (Lacerda et al., Reference Lacerda, Kersanach, Cortinhas, Prata, Dumont, Proietti, Maggioni and D'Incao2016); their diet is influenced by food availability in the environment and is mainly based on macroinfaunal organisms (Oliveira et al., Reference Oliveira, Pinto, Santos and D'Incao2006; Ferreira et al., Reference Ferreira, Barros, Barutot and D´Incao2011); and habitat selection is influenced by salinity and presence of seagrass meadows (Ruas et al., Reference Ruas, Rodrigues, Dumont and D'Incao2014), but in the absence of seagrass smaller individuals search for refuge in areas protected from the high currents of the estuary (Rodrigues et al., Reference Rodrigues, Ortega and D'Incao2019).
Here we tested hypotheses concerning the environmental drivers for habitat selection in estuarine areas. We hypothesize that sediment characteristics and presence of vegetated areas will be essential factors for recruits' settlement and development, as for the search of refuge in the early life and moulting phases. In this context, this study aimed to analyse the preferred areas for the spatial distribution of juveniles and moulting individuals of Callinectes sapidus in the shallow areas of the Patos Lagoon estuary and the adjacent marine reproductive area, in relation to some environmental parameters.
Materials and methods
Study area
The Patos Lagoon and the adjacent coastal area is one of the essential fishery sites of southern Brazil (Garcia et al., Reference Garcia, Vieira and Winemiller2001), providing the primary source of income to about 3600 fishers from surrounding communities (D'Incao, Reference D'Incao1991; Reis & D'Incao, Reference Reis and D'Incao2000; Kalikoski & Vasconcellos, Reference Kalikoski and Vasconcellos2012). The Patos Lagoon estuary (PLE) is located on the southern Brazilian coastline between 30–32°S and 50–52°W (Figure 1). The estuary is connected to the South Atlantic Ocean via a 20-km-long and 1-km-wide human-made inlet channel, with a maximum depth of 18 m (Fernandes et al., Reference Fernandes, Dyer, Möller and Niencheski2002, Reference Fernandes, Dyer and Möller2005). Through this channel, estuarine dependent and marine vagrant species migrate to complete their lifecycle or enter the estuary searching for nursery areas (Garcia et al., Reference Garcia, Vieira, Winemiller and Grimm2004). It is a micro-tidal estuary with dynamics depending on wind and freshwater discharge (Möller et al., Reference Möller, Castaing, Salomon and Lazure2001; Fernandes et al., Reference Fernandes, Dyer, Möller and Niencheski2002). The seaward flow of fresh water is mainly driven by north-eastern winds, while south-western winds pump marine water into the estuarine region (Möller et al., Reference Möller, Castaing, Salomon and Lazure2001). The combination of high rainfall and north-east winds results in large freshwater outflows, with significant inter-annual variations ranging from 500–12,000 m3 s−1 associated with El Niño events (El Niño Southern Oscillation-El Niño; Vaz et al., Reference Vaz, Möller and Almeida2006). The sizeable seasonal variability of these factors regulates both salinity and water level along the estuary (Möller et al., Reference Möller, Castaing, Salomon and Lazure2001, Reference Möller, Castello and Vaz2009; Fernandes et al., Reference Fernandes, Dyer, Möller and Niencheski2002; Möller & Fernandes, Reference Möller, Fernandes, Seelinger and Odebrecht2010). The exposed margins of this estuary are dominated by fine sands and the shallow embayments by fine sediments, which also may be retained by saltmarshes. The hydrodynamics of the estuarine region vary with the effect of meteorological parameters and its morphometry (Souza & Hartmann, Reference Souza and Hartmann2008). Most of the estuarine area has depths less than 1 m, the deepest zones being associated with natural and man-modified navigation channels that can reach up to 18 m in depth (Souza & Hartmann, Reference Souza and Hartmann2008). Field observations indicated submersed vegetation (seagrasses, Ruppia maritima, macroalgae) for most of the study period at Porto Rei and Torotama (upper estuary), and no vegetation in the other sampled sites. The submerged vegetation in the estuary is dependent on the salinity gradient, sediment type and wave exposure (Costa & Seeliger, Reference Costa and Seeliger1989; Mazo, Reference Mazo1994); with its seasonality depending on the photoperiod, salinity and temperature, which influences the reproductive cycle and biomass formation (Cafruni et al., Reference Cafruni, Krieger and Seeliger1978; Colares & Seeliger, Reference Colares and Seeliger2006). The highest biomass is observed in summer, and this declines in winter. Costa et al. (Reference Costa, Seelinger, Oliveira and Mazo1997) mapped for the best atmospheric and hydrological conditions, possible areas with a predominance of submerged vegetation. Their results show that the embayments ‘Saco do Silveira’, ‘Saco de Mendanha’ and the upper part of the ‘Saco do Arraial’ in the upper estuary, and some patches in the ‘Saco de Mangueira’ (mid estuary) were the areas with the highest biomass of submerged vegetation (localization of those embayments is presented in Figure 1).
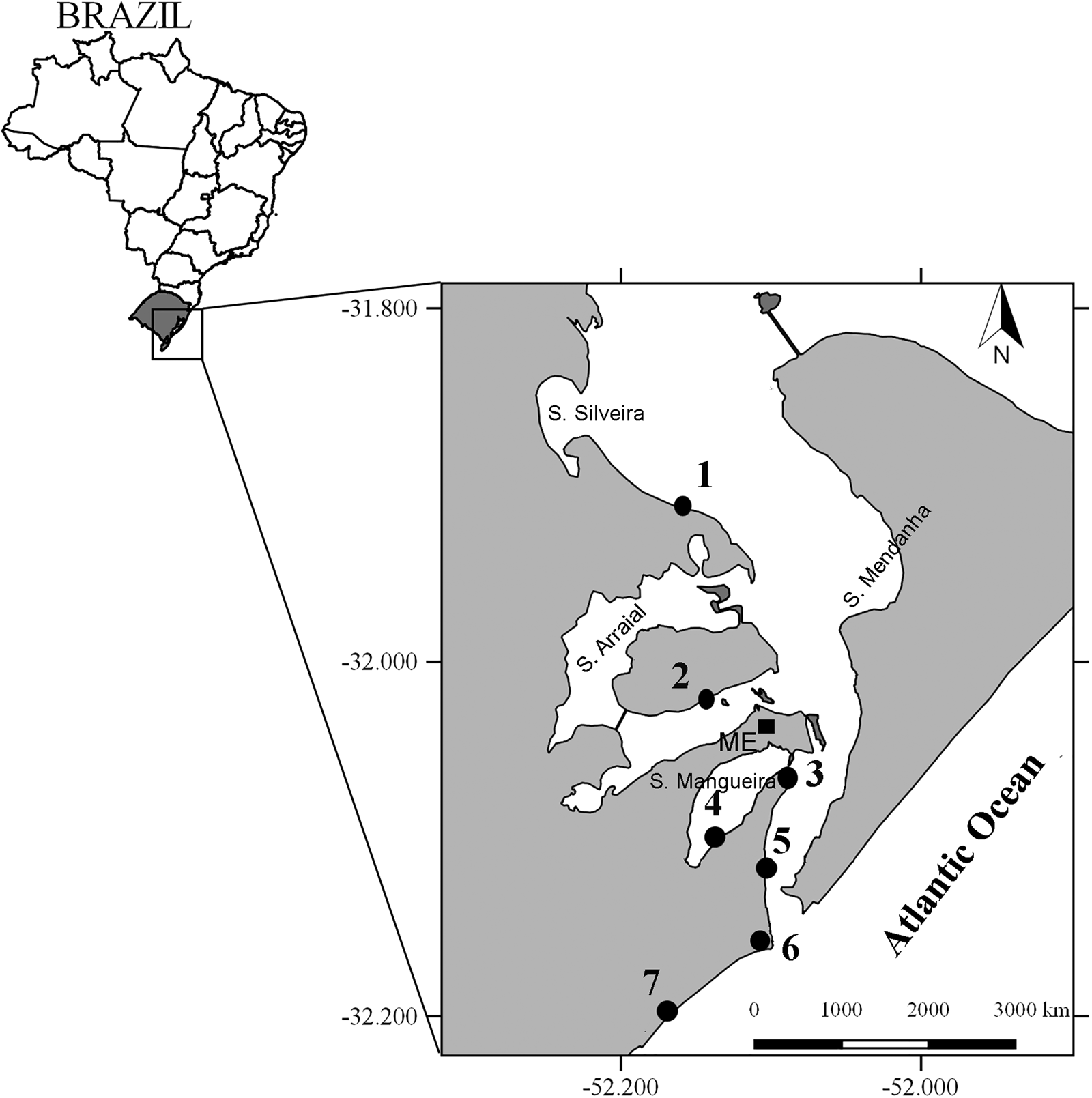
Fig. 1. Map of Brazil and the location of the Patos Lagoon in Rio Grande do Sul (RS) state with a detail showing the sampled sites on the Patos Lagoon estuary. 1 = Torotama, 2 = Porto Rei, 3 = Franceses, 4 = Mangueira, 5 = Prainha, 6 = Molhes, 7 = EMA. ME = Rio Grande Meteorological Station.
Sampling and laboratory procedure
Standardized samples were collected from seven sites in the estuary and adjacent marine area, with depth varying between 1–1.5 m (Figure 1). Sites were located in four regions: Porto Rei and Torotama (upper estuary), Mangueira and Franceses (mid estuary), Prainha (lower estuary), Molhes and EMA – in front of the Aquiculture Marine Station – (adjacent marine area). Due to access difficulties, it was not possible to sample in a second site in the lower estuary.
Samples were collected using a beach seine pulled manually for 5 min for fauna extraction. Five samples were obtained monthly in each site between February 2015 and June 2016. The net was 9 m long, with a mouth of 1.8 m width, and mesh size (different knots) of 5 mm in the central panel and 13 mm in the wings. In October 2015, samples in Porto Rei and Torotama were not performed for logistical reasons (flooding). All collected material was separated in the field (blue crabs from the other fish and invertebrate species) and frozen until processed. Water temperature (mercury thermometer), salinity (refractometer) and transparency (Secchi disc) data were collected simultaneously with biological sampling. In addition, three monthly sediment samples per site with a core of 10 cm diameter (area: 0.00784 m2) up to 5 cm depth were collected between December 2015 and June 2016 for grain size and organic matter analysis.
Moult stage classifications for C. sapidus were evaluated monthly between December 2015 and June 2016, the period when larval recruitment occurs and there is rapid growth during the first life stages in the estuary, according to the growth curve described for the species by Rodrigues & D'Incao (Reference Rodrigues and D'Incao2008). The identification of the stages followed by Kennedy & Cronin (Reference Kennedy and Cronin2007), through the colour analysis of the swimming appendage (5th pereopod): intermoult, early pre-moult, pre-moult and soft crab.
Carapace width was measured based on the distance between the anterolateral spines (calliper ± 0.05 mm). Individuals were grouped by size class in three categories based on juveniles' habitat use (Posey et al., Reference Posey, Alphin, Harwell and Allen2005): Class 1 (<13.0 mm CW), Class 2 (13.0–24.0 mm CW), Class 3 (24.1–54.3 mm CW). We added a fourth class (Class 4) to indicate adults, and grouped individuals with carapace width equal to or larger than 54.4 mm, the minimum size of maturation found in this study. The sizes used by Posey et al. (Reference Posey, Alphin, Harwell and Allen2005) were chosen because they represent sizes that other studies have indicated may differ in habitat use. They noted that juveniles <13 mm CW are strongly associated with submerged vegetation, when it is present, while juveniles larger than 24 mm CW move into the marsh or unvegetated shallow-water habitats, with the intermediate size class being transitional in habitat use. Maturity was verified through abdominal inspection (Van Engel, Reference Van Engel1958).
Grain size analyses of sediment samples were performed by dry mechanical sieving through a column of sieves from 4 to 0.063 mm, following the Wentworth classification system in intervals of one phi (Suguio, Reference Suguio1973). Grain size composition was expressed as a percentage of the total sample weight. To highlight the main grain sizes found in the estuary sizes higher than 500 µm (Very coarse sand, Very fine gravel and Fine gravel) were grouped as coarse sediments and those smaller than 125 µm (Very fine sands, Silt and Clay) as fine sediments. Organic matter percentage was calculated by differences in weight before and after calcination for 2 h at 375°C (Davies, Reference Davies1974). Hourly precipitation data were obtained from the National Institute of Meteorology (Instituto Nacional de Meteorologia–INMET) from the Rio Grande Station (Figure 1) for the period between January 2015–December 2016. We calculated monthly accumulation values for a clearer plot representation and interpretation.
Statistical analysis
The mean of the five tows performed at each site during each field trip was used to estimate the CPUE, defined as the number of individuals per 5 minutes pulled. The CPUE of all size classes and total densities showed considerable variability between samples and a predominance of zero values, making it unfeasible to perform traditional analyses, that assume error normality. Thus, we used non-parametric approaches (Anderson & Millar, Reference Anderson and Millar2004). Density values were transformed by log (x + 1) to down-weight the importance of high abundance counts. A Euclidian distance matrix was constructed with the transformed data and to reduce the effect of the absence of individuals in samples (Clarke et al., Reference Clarke, Somerfield and Chapman2006). A four-factor permutational analysis of variance (Permanova, P < 0.05, with permutation of residuals under a reduced model) tested the null hypotheses of no difference in CPUE of each size class/moult stage among the factors Regions (fixed), Sites (random, nested in Regions), Seasons (fixed) and Month (random, nested in Seasons). For each factor, 4999 permutations of raw data units were applied to obtain P-values (Anderson, Reference Anderson2005). The moult stage data matrix was constructed just with the samplings where we could determine at least one individual's moult stage, so analysis does not confound a zero of non-individuals with the non-determined stage. Spearman correlation was performed to evaluate the relationship between environmental variables and size class abundance/moult stage.
Results
Environmental variables
Precipitation during the studied period presented a reasonably large oscillation, varying between 0.6–278.8 mm per month. The highest precipitation rates were measured in September and October of 2015 (278.8 and 203.8 mm respectively) and the lowest values were recorded in the period between February and April of 2015 (<5 mm per month) (Figure 2). Data from May to July of 2016 are missing due to technical problems with the equipment.

Fig. 2. Monthly accumulated precipitation from the Rio Grande meteorological station for years 2015–2016. *Missing data.
There was a higher spatial than temporal variability of sediment texture among studied sites. Fine sands were the primary grain size in all studied sites. The highest percentage of fine sediments were found at Molhes (adjacent marine area), while sites in the upper and mid estuary at Franceses, Mangueira and Porto Rei were the sites with lower percentages of this grain size. We highlight that the highest percentages of fine sediments were found in the adjacent marine area and Torotama (upper estuary). Mangueira (mid estuary) was the site with coarsest grain sizes. The highest temporal variability was observed in Prainha (lower estuary) and Franceses (mid estuary), with variation mainly in the medium sand percentages (Figure 3).
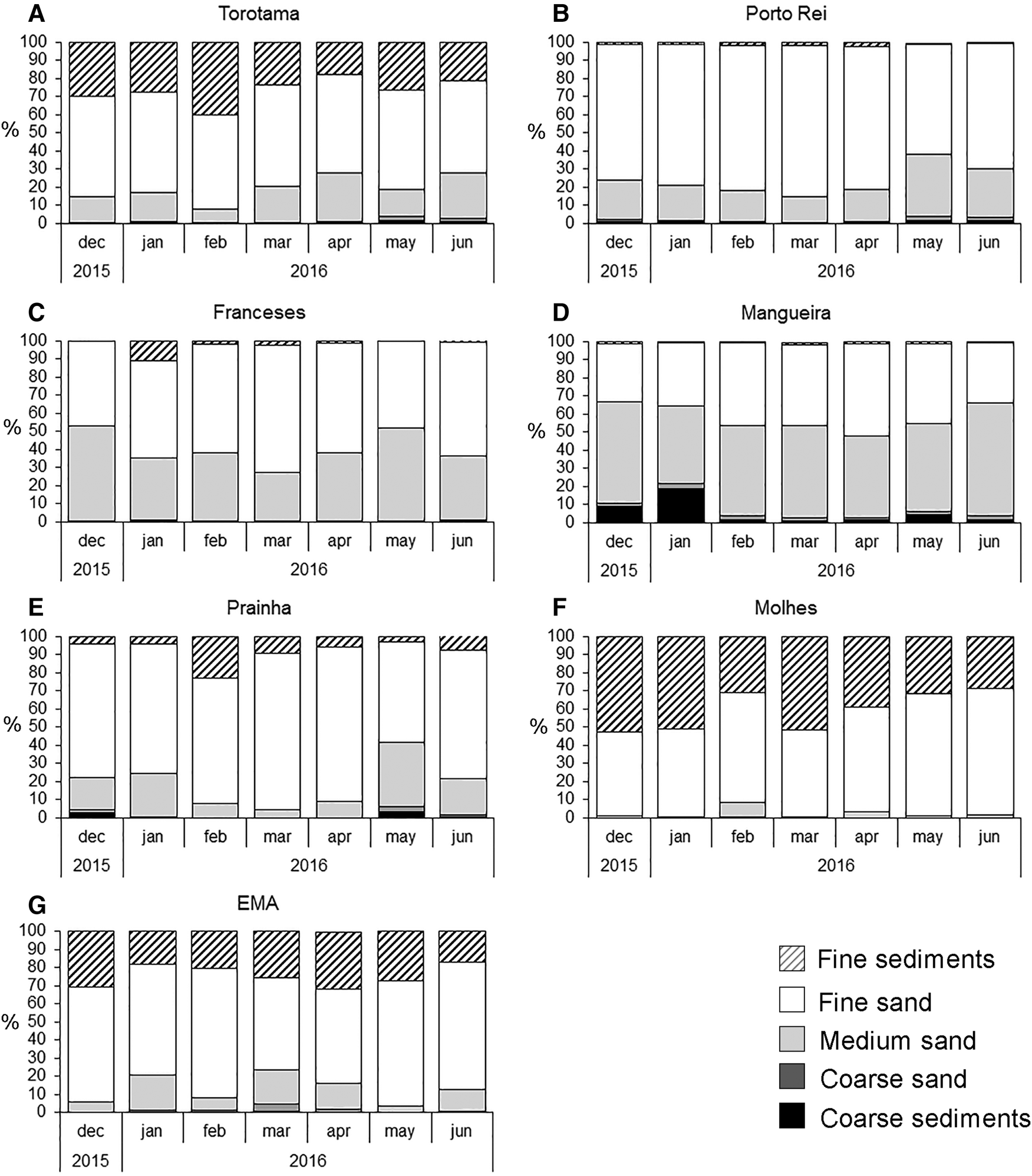
Fig. 3. Temporal variation of sediment grain sizes in the sampled sites of the Patos Lagoon estuary and adjacent marine area. Upper estuary – (A) Torotama, (B) Porto Rei; Mid estuary – (C) Franceses, (D) Mangueira; Lower estuary – (E) Prainha; Adjacent marine area- (F) Molhes, (G) EMA.
The adjacent marine area presented the highest values of organic matter compared with sites further within the estuary. Within the estuary, there was a decrease in organic matter percentages from the upper estuary to the lower estuary. EMA was the site with the highest organic matter percentages, and Franceses the lowest. The highest value of organic matter was found at EMA (adjacent marine area) in March 2016, followed by December 2015. The lowest values were found in Franceses (mid estuary, May 2016) and Torotama (upper estuary, April 2016). The highest organic matter percentages were found in spring for all sites (Table 1).
Table 1. Seasonal variation (mean ± standard deviation) in the environmental variables measured by site

Salinity follows the estuarine gradient with highest values in the adjacent marine area and lower values in the upper estuary. The lowest salinities were measured in winter and spring (Table 1, Figure 4). The temperature oscillation pattern was similar for all sites (Table 1, Figure 4). The minimum value was 12°C in winter and a maximum of 34°C in summer. Transparency was very low during the whole studied period, with values under 0.5 m common (Table 1).

Fig. 4. Mean density (ind 5 min−1) of blue crab Callinectes sapidus by studied regions and months. (A) Upper estuary, (B) Mid estuary, (C) Lower estuary, (D) Adjacent marine area. Vertical bars indicate standard error.
Spatial and temporal variations of blue crabs by size classes
We quantified 1174 blue crabs during the studied period, with the highest number in the upper estuary (359 individuals in Torotama and 475 in Porto Rei). Individuals in the lower estuary and adjacent marine area were scarce, with the lowest account in Molhes (2 individuals in the whole period).
There were significant differences in the total abundance among the Regions (Pseudo-F: 113.79, P < 0.001), the Month within the Seasons (Pseudo-F: 7.30, P < 0.001) and the interaction between the factors Regions and Month (Pseudo-F: 3.12, P < 0.001) and between Sites and Month (Pseudo-F: 1.88, P = 0.009). There was an apparent decrease in total abundance from the upper estuary to the adjacent marine area (Figure 4). In the upper estuary, the highest densities were found in March and April 2016 (end of summer and beginning of autumn). In mid estuary, the highest densities were found in March and April 2015 (end of summer and beginning of autumn) and October 2016 (spring). Densities in the lower estuary and the adjacent marine area were very low during the whole studied period.
Analysing by size classes, all classes varied significantly by Regions (P < 0.01) and Month (P < 0.01). In particular, juveniles' abundance demonstrated a significant variation in the Site by Month interaction (P < 0.01). The variations in adults' abundance were significant in the interaction between Regions and Month (P = 0.038) (Table 2).
Table 2. PERMANOVA results for Callinectes sapidus total densities and each size class densities

Bold numbers indicate significant results.
The smallest classes were mainly found in the upper estuary while the biggest ones were in the adjacent marine area (Figure 5). Juveniles of Class 1 were mainly found in Torotama and Porto Rei (upper estuary), followed by Mangueira (mid estuary). Highest abundances were found mainly at the end of summer with a second peak of abundance in August (winter). Notable are the recruits found in EMA in February 2015, which were unexpected in that area. Class 2 was also mainly found in Torotama, Porto Rei and Mangueira. The highest densities in Torotama were in January 2016; while in Porto Rei and Mangueira the peak was in March and April. Class 3 was randomly found in all sites throughout the year, and Class 4 was mainly found in EMA. The two individuals found in Molhes were a juvenile Class 2 found in March 2015 and an adult Class 4 found in November 2015.

Fig. 5. Total density (ind 5 min−1) of Callinectes sapidus size classes in each sampled site by month. Upper estuary – (A) Torotama, (B) Porto Rei; Mid estuary – (C) Franceses, (D) Mangueira; Lower estuary – (E) Prainha; Adjacent marine area – (F) EMA.
Spatial-temporal distribution of moult stages
We determined the moult stage of 296 individuals; the majority of them were in the intermoult stage (160 individuals; 54%). The abundance of intermoult individuals varied significantly between Seasons (Pseudo-F: 3.3349, P = 0.0472), and Month (Pseudo-F: 4.6452, P = 0.0072). The abundance of individuals beginning the moulting process varied significantly among Regions (Pseudo-F: 3.04796, P = 0.0356), with higher abundances in the mid and upper estuary.
We could not determine any spatial or temporal significant differences on pre-moult and soft crab stages individuals. The individuals in pre-moult and soft crab stages were found just in the mid and upper estuary, while the intermoult stages were found throughout the estuary with the exception of the lower reaches (Figure 6). Individuals at the beginning of the moulting process were found just inside the estuary. Concerning the temporal factors, the highest percentages of individuals in pre-moult and soft crab stages were in the warmer months (December to March) (Figure 6).

Fig. 6. Percentage of Callinectes sapidus individuals in different moulting stages by (A) estuarine regions, (B) measured months.
Relationship between environmental parameters and size class and moult stage
There was not a clear pattern in the relationship of crab size class and environmental parameters. However, more environmental parameters correlated with the abundance of juveniles than adults. Densities of juvenile Class 1 presented significant correlations with water transparency (P < 0.01) and temperature (P = 0.03); Class 2 with temperature (P < 0.01), water transparency (P = 0.01), fine sands (P < 0.01), salinity (P < 0.01) and organic matter percentage (P = 0.04); and Class 3 with temperature (P < 0.01), salinity (P < 0.01) and organic matter percentages (P = 0.01). Adults were correlated negatively with salinity (P < 0.01) (Table 3).
Table 3. Spearman correlations between Callinectes sapidus size classes densities and environmental parameters on the Patos Lagoon estuary and adjacent marine area

Bold numbers indicate significant correlations.
In different moulting stages, crab abundance was correlated differently with environmental characteristics. Individuals at the beginning of the moulting process and in pre-moult stage showed significant correlation with sediment characteristics (P < 0.05). In the next moult stage, the abundance of individuals correlated with water column properties (P = 0.02). The abundance of individuals in the intermoult stage did not correlate with any measured environmental variable (Table 4).
Table 4. Spearman correlations between densities of Callinectes sapidus by moulting stages and environmental parameters on the Patos Lagoon estuary and adjacent marine area
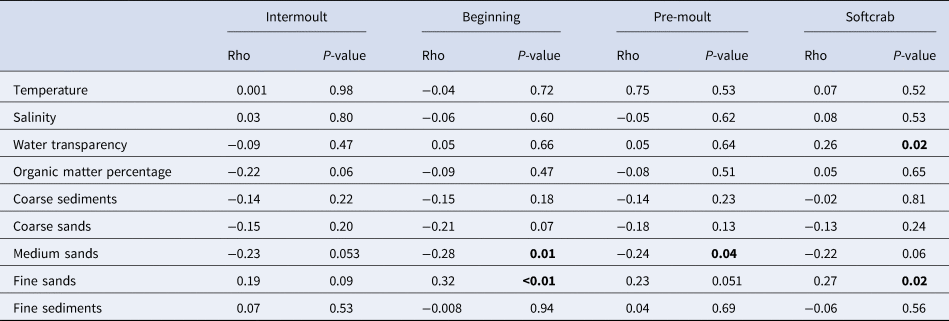
Bold numbers indicate significant correlations.
Discussion
Habitat selection
Sediment properties and the presence of submersed vegetation are important factors for habitat selection in the recruitment, settlement, development and moulting of early stage blue crabs. Habitat preference and spatial distribution of Callinectes sapidus are possibly determined by a synergistic action of the environmental cues analysed in this study, but the intensity of this preference depends on moult stage and ontogenetic development phase. In this sense, even though recruitment and moulting could happen in any part of the estuary, crabs seem to migrate to zones with higher probabilities of survival. The probability of migration depends on a combination of biotic and environmental factors such as habitat, wind, currents and crab density (Blackmon & Eggleston, Reference Blackmon and Eggleston2001; Reyns & Eggleston, Reference Reyns and Eggleston2004; Reyns et al., Reference Reyns, Eggleston and Leuttich2006). The highest abundance determined in this study, in the upper estuary zones of moulting individuals and smaller juveniles (Classes 1 and 2), initially, seems to contradict the high metabolic cost of living in an environment with highly variable salinity (Guerin & Stickle, Reference Guerin and Stickle1992). However, the high availability of prey in these zones (Kinsey & Lee, Reference Kinsey and Lee2003) may act as an energetic compensation, and the presence of minerals and nutrients allows recalcification of the exoskeleton (Vigh & Dendinger, Reference Vigh and Dendinger1982). Furthermore, low salinity zones in coastal estuaries are suited for recruitment, due to cannibalism and predation of juveniles in higher salinity areas (Hines et al., Reference Hines, Lipicius and Haddon1987; Posey et al., Reference Posey, Alphin, Harwell and Allen2005). This may also be true for moulting individuals (present study).
In the studied period, submerged vegetation was observed only in the upper estuary. So, in addition to the previously discussed characteristics, individuals from Class 1 and 2 and in moulting stage possibly presented higher abundances in this area due to the refuges that vegetated zones present, the finer sediments, high abundance of prey and the availability of hiding from predators, as also suggested by Posey et al. (Reference Posey, Alphin, Harwell and Allen2005). The presence of submerged vegetation near the lower salinity and finer grain sizes appear to be ecosystem indicators selected by both juveniles (Ruas et al., Reference Ruas, Rodrigues, Dumont and D'Incao2014 and present study) and individuals in moult (present study) in the PLE in order to reduce predation. In the absence of vegetation, all juveniles tend to prefer the estuary embayment which offers protection against high energy events (high currents) of the main lagoon channel (Rodrigues et al., Reference Rodrigues, Ortega and D'Incao2019).
The maintenance of this zonation of C. sapidus juveniles in the upper estuary due to the reduction of predation, in addition to the refuge provided by the submerged vegetation, becomes plausible since it has been demonstrated that indeed there are fewer predators. The guild of predatory fish that feed on zoobenthos, especially Brachyura, in the PLE is composed of mainly estuarine-dependent species or marine visitors, for example the catfish Genidens genidens (Cuvier, 1829), the black drum Pogonias cromis (Linnaeus, 1766) and the bluewing searobin Prionotus punctatus (Bloch, 1793). These fish are more abundant in the mid and lower estuary and the region towards the upper estuary (from lower to upper estuary direction) that these fish may reach depends on the penetration of seawater (Possamai et al., Reference Possamai, Vieira, Grimm and Garcia2018).
Although smaller classes clearly prefer upper estuary areas, recruitment of individuals in the adjacent marine region (EMA) during February 2015 is noteworthy. Heavy rains during this period over the Patos Lagoon basin (www.inmet.gov.br) caused a high flow out of the lagoon, probably making it difficult for planktonic larvae to enter the estuary. Due to this large flow and consequent reduction of salinity, along with the contribution of fine sediments in suspension that settled in the marine adjacent region (EMA) (also reported by Calliari et al., Reference Calliari, Muehc, Hoefel and Toldo2003), the conditions of the region resembled a region suitable for larval settlement (similar to fishes, e.g. Mont'Alverne et al., Reference Mont'Alverne, Moraes, Rodrigues and Vieira2012). As settlement of blue crab recruits depends on the spawning site and the hydrodynamics of the ocean and estuary, in combination with winds, that drive the entrance of the post-larvae to the nursery areas (Etherington & Eggleston, Reference Etherington and Eggleston2003), these unusual conditions in the adjacent marine areas might be suitable for larval recruitment. Due to the unusual and short duration of these environmental conditions in the marine area that allowed the presence of recruits, it remains to be understood if these recruits can settle, survive and reach adulthood, or if they are eliminated by the beach characteristics when it changes back to normal conditions.
Larger individuals such as Class 3 juveniles and young adults are big and robust enough to defend themselves against predators and thus migrate from the vegetated zones. Once out of the nursery areas, they are distributed throughout the whole estuary without a particular pattern, as was also observed by Rodrigues et al. (Reference Rodrigues, Ortega and D'Incao2019). The low catch of adults (Class 4) seems to reflect their preference for areas with a depth greater than 1.5 m (depth limit sampled in this study), which would lead to a reduction in the competition for resources between adults and juveniles.
Temporal variation
The environmental conditions of a particular period may alter the classic temporal patterns of reproduction and recruitment of crustaceans (Möller et al., Reference Möller, Castello and Vaz2009). It has been established that C. sapidus postlarvae enter and recruit into the estuary in spring and summer (Lipcius et al., Reference Lipcius, Eggleston, Heck, Seitz, van Montfrans, Kennedy and Cronin2007). However, environmental conditions and a delay in spawning may reflect in recruitment in different seasons (Rodrigues et al., Reference Rodrigues, Ortega and D'Incao2019). Similar to the findings of Rodrigues et al. (Reference Rodrigues, Ortega and D'Incao2019), we observed two recruitment peaks, the first one in late spring and summer and the second one in winter. These peaks can be related to macroalgal blooms, that are controlled by a delicate balance of estuary conditions (Lanari & Copertino, Reference Lanari and Copertino2016).
Over the studied period, the first year was predominantly a stormy period due to a moderate El Niño event in 2015 and 2016 (NOAA, 2017) that resulted in a long rainy period in southern Brazil, causing a decrease in the abundance of recruits. Thus, periods of longstanding rains and the consequent decrease in salinity and enhancement of seaward flow on the estuary may compromise the entrance and survival of postlarvae to the PLE. For the portunid Portunus pelagicus (Linnaeus, 1758) it has been reported that mass migrations of juveniles to alternative habitats precede reductions of seasonal salinity in Australian estuaries (Potter & Lestang, Reference Potter and Lestang2000), which is a possibility for C. sapidus. This may also explain the change of patterns of higher abundance of blue crabs in Franceses (an embayment with higher salinities) during the first year and a decrease in the second one, contrasting with the pattern of Porto Rei (embayment in the upper estuary) where the higher abundances were encountered in the second year. Even with the contrast in patterns, there were always higher abundances in the second embayment, a zone more protected from the variations in the environmental conditions and with vegetated bottoms (Site 2 in Figure 1).
Management implications
Shallow zones of the upper estuary are areas that favour the protection, recruitment and growth of the blue crab (Rodrigues et al., Reference Rodrigues, Ortega and D'Incao2019 and present study), and are mainly used as nursery areas and refuges in the moulting period. These zones were also indicated to favour the recruitment of the pink shrimp Penaeus paulensis (Noleto-Filho et al., Reference Noleto-Filho, Pucciarelli and Dumont2017) and all shallow areas with depths less than 2 m are good recruitment habitat for 99 fish species (Vieira et al., Reference Vieira, Castello, Pereira, Seeliger, Odebrecht and Castello1998; Costa et al., Reference Costa, Possingham and Muelbert2016). In this sense, shallow areas favour the recruitment of all targets of artisanal fishing in the region (blue crab, pink shrimp and fish). Following the suggestions of Costa et al. (Reference Costa, Possingham and Muelbert2016) for the protection of shallow areas of the PLE, we suggest adding special protection for the recruitment areas of the spatial overlay of several groups such as the embayment around Marinheiros Island, where Torotama and Porto Rei are located. However, this region is also an important area for local fishers (Ruas, Reference Ruas2015; Thykjaer et al., Reference Thykjaer, Rodrigues, Haimovici and Cardoso2019), and is profoundly impacted by illegal shrimp trawl fisheries (Rezende et al., Reference Rezende, Neunfeld, Estima and Dumont2015). In this sense, we suggest (1) better promotion of efforts to study the communities of all shallow areas of this embayment to give complete tools for management stakeholders, (2) enforcement of environmental regulations and (3) taking new management decisions in conjunction with scientific researchers, government and fishermen, to better protect this area from anthropogenic impacts, establishing accurate measures for sustainable fisheries.
Conclusions
There is a delicate balance between the biological cycle of the blue crab and environmental conditions. It is of paramount importance to protect this ecological and economic resource during the critical phases described in the present study. The recruitment of blue crabs is highly dependent on the environmental and hydrodynamic conditions of the estuary. Vegetated areas with fine sediments and high organic matter content are the preferred places for recruitment, mainly in the upper estuary. Some environmental conditions that simulated the conditions of the upper estuary in the adjacent marine area were preferred by the larvae and may confound them and allow recruitment. There were two peaks of recruitment in the PLE, the first one in late spring and summer, and the second one in winter. It is essential to improve the management of the upper estuary embayment to increase the likelihood of successful recruitment of blue crabs and other species within the estuary.
Acknowledgements
The authors would like to thank all the personnel involved in the field trips, and the Laboratories of Crustaceans, Ichthyology and Geological Oceanography (IO/FURG) for the support in the sampling and analysis. This paper is part of the Long-Term Studies Program (PELD-FURG).
Financial support
This work was supported by the Coordination for the Improvement of Higher Education Personnel (CAPES) with grants to C.I., I.O. and the National Council for Scientific and Technological Development (CNPq) with the grant to L.R.