Introduction
Bergmann's rule (Bergmann, Reference Bergmann1847) postulates that, for any given genus of homeothermic animals, large-sized species rather than small-sized species will occur in cooler climates. Although Bergmann (Reference Bergmann1847) considered thermoregulation as the main factor accounting for size clines (James, Reference James1970; Blackburn et al., Reference Blackburn, Gaston and Loder1999; Ashton et al., Reference Ashton, Tracy and de Queiroz2000), this hypothesis was later reviewed by Rensch (Reference Rensch1938, Reference Rensch1959) and Mayr (Reference Mayr1956, Reference Mayr1963) with respect to empirical patterns, independently of ecophysiological interpretations and pertinent to intraspecific variation. James (Reference James1970) supported the intraspecific considerations although stressing the importance of climatic factors in determining size clines. Recently, Blackburn et al. (Reference Blackburn, Gaston and Loder1999), reviewing the current principles of Bergmann's rule, readdressed the conceptual basis of interspecific variation. However, most of the presently available literature deals with intraspecific body size variation, and many different hypotheses from the original thermoregulation postulate have been put forward for explaining Bergmannian (and converse Bergmannian) body-size clines (Atkinson & Sibly, Reference Atkinson and Sibly1997; Ashton et al., Reference Ashton, Tracy and de Queiroz2000; Ashton, Reference Ashton2001a, Reference Ashtonb; Reference Ashton2002a, Reference Ashtonb; Ashton & Feldman, Reference Ashton and Feldman2003; Meiri & Dayan, Reference Meiri and Dayan2003; Blanckenhorn & Demont, Reference Blanckenhorn and Demont2004).
Although Bergmann's rule was originally proposed for homeotherms, it was later shown that Bergmannian (and converse Bergmannian) clines could also be found among ectothermic organisms (Ray, Reference Ray1960; Masaki, Reference Masaki1967, Reference Masaki and Dingle1978; Honek, Reference Honek1993; Atkinson, Reference Atkinson1994; Mousseau, Reference Mousseau1997; Angilletta & Dunham, Reference Angilletta and Dunham2003), either invertebrate (Arnett & Gotelli, Reference Arnett and Gotelli1999; Brisola Marcondes et al., Reference Brisola Marcondes, Leuch Lozovei, Falqueto, Brazil, Galati, Aguiar and Souza1999; Byers, Reference Byers2000; Huey et al., Reference Huey, Gilchrist, Carlson, Berrigan and Serra2000; Smith et al., Reference Smith, Hines, Richmond, Merrick, Drew and Fargo2000; Trussell, Reference Trussell2000; Roy & Martien, Reference Roy and Martien2001; Hausdorf, Reference Hausdorf2003; Heinze et al., Reference Heinze, Foitzik, Fischer, Wanke and Kipyatkov2003; Johansson, Reference Johansson2003; Blanckenhorn & Demont, Reference Blanckenhorn and Demont2004; Peat et al., Reference Peat, Darvill, Ellis and Goulson2005; Bidau & Martí, Reference Bidau and Martí2006) or vertebrate (Ashton, Reference Ashton2001a, Reference Ashton2002a; Belk & Houston, Reference Belk and Houston2002; Ashton & Feldman, Reference Ashton and Feldman2003; Laugen et al., Reference Laugen, Laurila, Räsänen and Merilä2003, Reference Laugen, Laurila, Jönsson, Söderman and Merilä2005; Litzgus et al., Reference Litzgus, Durant and Mousseau2004; Schäuble, Reference Schäuble2004; Cruz et al., Reference Cruz, Fitzgerald, Espinoza and Schulte2005).
The presence of Bergmannian patterns in ectotherms requires a different explanation from the one accounting for their presence in endotherms. Furthermore, converse Bergmannian clines are much more frequent in ectotherms, mainly in insects (Brennan & Fairbairn, Reference Brennan and Fairbairn1995; Mousseau, Reference Mousseau1997; Fischer & Fiedler, Reference Fischer and Fiedler2002; Blanckenhorn & Demont, Reference Blanckenhorn and Demont2004; Bidau & Martí, Reference Bidau and Martí2006), than in endotherms (Ochocinska & Taylor, Reference Ochocinska and Taylor2003; Medina et al., Reference Medina, Martí and Bidau2006). Body size clines in insects probably result from climatic selection on duration of egg and nymphal development combined with different life cycle types (univoltine, bivoltine, multivoltine), which may result in a variety of body-size distribution patterns, including some actually converse to Bergmann's rule (Masaki, Reference Masaki1967; Roff, Reference Roff1980; Blanckenhorn & Demont, Reference Blanckenhorn and Demont2004).
Species with widespread, latitudinal and/or altitudinal geographic ranges are useful models for analysing body size distribution at intraspecific levels, as is the case of some South American grasshopper species of the melanopline genus Dichroplus (Cigliano & Otte, Reference Cigliano and Otte2003; Bidau & Martí, Reference Bidau and Martí2006).
In this paper, we analyse the geographic (latitudinal and altitudinal) distribution of body size in populations of Dichroplus vittatus Bruner (Orthoptera: Acrididae), a species belonging to the Maculipennis group of Dichroplus (Cigliano & Otte, Reference Cigliano and Otte2003) with respect to climatic variables, to contribute to the understanding of acridoid body size clines in nature, which are largely unknown, especially in widely distributed Neotropical species and also, considering the extensive size and allometric variations occurring within this species and among Dichroplus species in general, to help resolve the systematics, ecological constraints and evolutionary dynamics of this genus.
Materials and methods
Adult male and female D. vittatus were collected at 19 Argentine localities spanning across 20° latitude, and at altitudes ranging from 36 to 2758 m above sea level during the summer months of 2001, except for a sample from Villa del Rosario which was collected during the summer of 1991 (fig. 1, table 1). Six morphometric external characters were measured in preserved specimens: (i) total body length (BL) (from the tip of the head to the distal end of the left third femur when aligned parallel to the longitudinal axis of the body following Martí (Reference Martí2002), for avoiding errors resulting from shrinking of the abdomen in dried specimens); (ii) length of left hind femur (F3L); (iii) length of left hind tibia (T3L); (iv) length of tegmina (TeL); (v) mid-dorsal length of pronotum (PL); and (vi) height of pronotum (PH). The maximum total body length (MBL) for each sex (body length of the largest male and female in each sample) was also used as a dependent variable. The coefficient of variation of all characters was calculated as CV=s*100/. Measurements were taken with high precision calipers (0.01 mm).

Fig. 1. Geographic distribution of the Argentine populations of Dichroplus vittatus analysed in this study.
Table 1. Means and standard errors of six morphometric characters in 19 populations of Dichroplus vittatus.

Populations are those indicated in fig. 1. Lat (S): latitude; Lon (W): longitude; A: altitude (metres above seal level); N: number of individuals; BL: total body length; F3L: left femur 3 length; T3L: left tibia 3 length; TeL: tegmina length; PL: pronotum length; PH: pronotum height; SE: standard error of the mean.
Climatic data for all samples were obtained from Leemans & Cramer (Reference Leemans and Cramer1991) (table 2), and included: TMEA, mean annual temperature; TMAX, mean maximum monthly temperature; TMIN, mean minimum monthly temperature; PANN, total annual precipitation; PMAX, mean maximum monthly precipitation, and PMIN, mean minimum monthly precipitation. To estimate seasonality, the annual variability of climatic factors was calculated. Annual variability of temperature was estimated through the coefficient of variation of TMEA (CVT) and the difference between TMAX and TMIN (TM-m). Variability of precipitation was assessed by the CV of mean annual precipitation (CVP), and the difference between maximum and minimum monthly precipitation (PM-m). Actual evapotranspiration (AET), potential evapotranspiration (PET) and water balance (WB) were obtained for each locality. We used vectors, databases and maps for AET, PET and WB from Ahn & Tateishi (Reference Ahn and Tateishi1994a, Reference Ahn and Tateishib) (table 2). Data analysis was performed with the Geomatica FreeView V. 10.0 software (PCI Geomatics, Ontario, Canada. www.pcigeomatics.com). All data are expressed in mm per year.
Table 2. Temperature, precipitation, evapotranspiration and water balance parameters of 19 Argentine localities where samples of Dichroplus vittatus were obtained.

All temperature parameters except CVT in °C; all precipitation parameters except CVP, in mm. AET, PET and WB in mm per year. See text for nomenclature of variables.
The normality of data was estimated through the Kolmogorov-Smirnov (with Liliefors correction) test. The Durbin-Watson statistic was employed for estimating serial autocorrelation. All measurements were log-transformed except for proportions between morphological measurements, for which the arcsine (angular) transformation was applied. Latitude (LAT) and longitude (LON) were converted to decimal units.
Principal components analysis (PCA) was employed for analysing the relationship between body size and geographic and climatic variables. Factors extracted in each analysis were rotated with the VARIMAX procedure with Kaiser normalization and used as dependent variables in correlation/regression tests.
Results
Geographic variation of body size in D. vittatus
As shown in table 1, body size varied widely between localities. The smallest and largest females showed body lengths of 17.30 mm (Playa Unión) and 29.59 mm (La Viña) respectively, while the smallest sampled male showed a body length of 14.40 mm (Playa Unión), contrary to the largest, with 22.02 mm (La Viña). This species shows a marked sex dimorphism in body size, with females being considerably larger than males across their geographic distribution (C.J. Bidau & D.A. Martí, unpublished). We studied the geographic variation of body size separately, in each sex, using BL and MBL as dependent variables against latitude or altitude in regression analyses. Initially, LAT was used as an independent variable because this parameter can be easily obtained and because of its likely correlation with several factors influencing body size clines (Blackburn et al., Reference Blackburn, Gaston and Loder1999). In all four analyses, body size significantly decreased with increasing latitude (fig. 2a,b; table 4). However, no statistically significant, altitudinal body size clines were observed despite that the population from Huacalera (the population of D. vittatus occurring at the highest known altitude, at 2758 m above sea level), showed some of the lowest means in body size. In fact, when this exceptional population was removed from analyses, latitudinal clines became more steep and statistically significant (MBL, r=−0.885, P=0.000; MMBL, r=−0.835, P=0.000; FBL, r=−0.841, P=0.000; MFBL=−0.660, P=0.003).
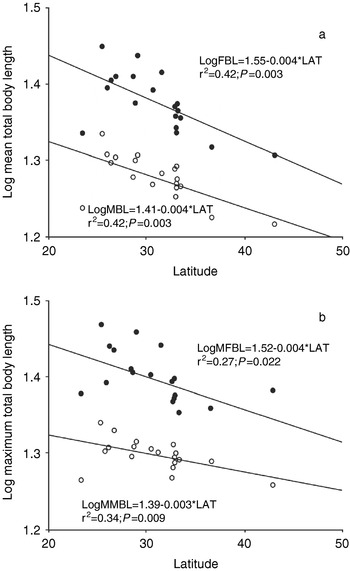
Fig. 2. Linear relationships between estimators of body size of female and male Dichroplus vittatus, and latitude. a. Log mean total body length vs. latitude. b. Log maximum body length vs. latitude. ●, females; ○, males.
To test if general body size followed the same trend, factors extracted by principal components analyses (table 3) were regressed against LAT and ALT. As shown in table 4 and fig. 3a,b, statistically significant, negative correlations between PCA factors and LAT (but not with ALT) were observed.
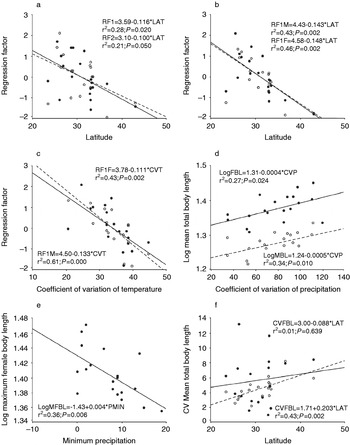
Fig. 3. Linear relationships between (a) the first (full line) and second (dashed line) principal components from the joint female-male PCA and latitude; (b) the first principal component of each individual PCA, and latitude (female: full line; male: dashed line); (c) the first principal component of each individual PCA (female: full line; male: dashed line), and the coefficient of variation of annual temperature; (d) female (●) and male (○) mean total body length, and the coefficient of variation of precipitation; (e) maximum female body length and minimum precipitation; (f) the coefficients of variation of female (●) and male (○) mean total body length and latitude.
Table 3. Principal components analysis of morphological data of populations of Dichroplus vittatus from Argentina.
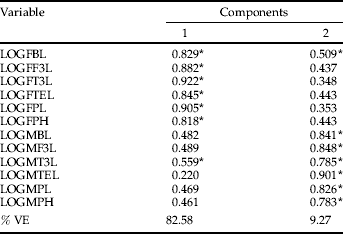
Two factors were extracted and rotated with the VARIMAX procedure with Kaiser normalization for 12 morphometric variables of males and females (see Materials and methods and table 2 for nomenclature of variables). Values correspond to correlation coefficients between variables and factors. Relatively high loadings (|r|>0.5) are marked with an asterisk. %VE, % total variance explained.
Table 4. Correlation coefficients and their statistical significance between estimators of body size of Dichroplus vittatus and geographic and climatic variables.

In the case of the temperature parameters (except CVT), Spearman non-parametric correlation was performed (see Materials and methods). See text for nomenclature of variables.
A further interesting finding indicated that variability of male body length, denoted by its coefficient of variation, showed a highly significant positive correlation with latitude, showing that male body size appeared to be more variable towards higher latitudes rather than to higher altitudes (table 4; fig. 3d). The CV of TeL and PH showed a comparable behaviour respective to latitude (r2=0.520; a=−35.300; b=1.497; P=0.000, and r2=0.311; a=−2.691; b=0.263; P=0.013), while similar positive but non-significant trends occurred for the other estimates. No significant correlations between the CVs of morphometric characters and latitude or altitude were observed for females.
Geographic variation of allometric relationships
We analysed the variation of the relative size of F3, T3, Te and pronotum with respect to BL, as well as other relevant morphometric relationships (T3L/F3L, PH/PL, PL/TeL, and PH/TeL) in males and females, by means of linear regression. All regressions indicated a strong negative allometry except for female T3L/F3L, which showed a slope not significantly different from 1.
We tested whether these allometrically significant proportions also showed a geographic variation. Five proportions showed statistically significant or marginally significant latitudinal clines: MPH/BL (r=0.432, P=0.065), FTeL/BL (r=−0.637, P=0.003), FPL/TeL (r=0.633, P=0.004), MPH/TeL (r=0.405, P=0.086), and FPH/TeL (r=0.553, P=0.014).
Correlations of body size with climatic factors
Body size clines were tested against climatic parameters and measurements of climate variability (tables 2, 4). We found significant positive correlations between body size estimators and temperature means (TMEA and TMIN), but a negative, significant correlation with CVT. It is worth mentioning that neither TMEA nor TMIN were significantly correlated with latitude (Spearman's rho=−0.348, P=0.144, and Spearman's rho=−0.415, P=0.077, respectively), a reason why temperature clines could not be attributed to colinearity between latitude and temperature. CVT was also non-significantly correlated with latitude (r=0.413, P=0.079). Regarding precipitation, we found a negative correlation between PMIN and several body size estimators, and a consistent, positive correlation between body size and CVP (table 4). In both cases, strong correlations between PMIN, CVP and latitude occurred (r=0.699, P=0.001, and r=−0.725, P=0.000, respectively). No significant correlations were found between temperature and the above mentioned precipitation parameters. With PMIN, the respective r estimates were: PMIN/TMEA=−0.017, PMIN/TMIN=−0.027 and PMIN/CVT=0.232. With CVP, r estimates were: CVP/TMEA=−0.081, CVP/TMIN=−0.017 and CVP/CVT=−0.371.
The CV of male TBL was highly significantly correlated with both CVT (r2=0.306; a=0.067; b=0.132; P=0.014) and CVP (r2=0.247; a=6.766; b=−0.028; P=0.031). Female CVs for the same characters appeared to be independent of these seasonality factors.
Regarding estimators of water balance, no consistent results were obtained despite the finding of some significant correlations with body size estimators (table 4). Both PET and WB were highly significantly correlated with latitude (r=−0.740, P=0.000, and r=0.642, P=0.003, respectively), while AET was not (r=−0.199, P=0.414).
Discussion
In ectothermic species of widespread latitudinal/altitudinal distribution, several geographic body size patterns have been found: Bergmannian, converse Bergmannian and combined (Chown & Gaston, Reference Chown and Gaston1999; Chown & Klok, Reference Chown and Klok2003; Johansson, Reference Johansson2003; Bidau & Martí, Reference Bidau and Martí2006). Blanckenhorn & Demont (Reference Blanckenhorn and Demont2004) listed 46 insect species belonging to 11 orders with 17 (37%) species following Bergmann's rule, and 29 (63%) with a converse pattern, suggesting that these two clinal patterns might be part of a continuum of adaptive responses to ambient temperature (following Bergmann's rule) or growth duration (converse Bergmann's rule). These different effects, as well as counter gradient variation might be jointly operating to produce a variety of clines, from typically Bergmannian to converse Bergmannian (Blanckenhorn & Demont, Reference Blanckenhorn and Demont2004).
Ectotherms grow slower at lower temperatures but reach larger adult sizes than their counterparts from more temperate climates (Ray, Reference Ray1960; Atkinson, Reference Atkinson1994; Atkinson & Sibly, Reference Atkinson and Sibly1997; Angilletta & Dunham, Reference Angilletta and Dunham2003), thus supporting Bergmann's rule. However, this might not necessarily apply to univoltine insects of widespread distribution like D. vittatus, for which duration of growing season might represent a powerful limiting factor (Masaki, Reference Masaki1967; Roff, Reference Roff1980).
Dichroplus vittatus goes through an obligatory egg diapause, with only one generation per year, resulting in a 7-month arrest of embryogenesis at the end of anatrepsis, with eggs initiating diapause for several months. The number of months in diapause varies in different geographic regions (Campodónico, Reference Campodónico1968; Turk & Barrera, Reference Turk and Barrera1979). Obligatory diapause and one generation per year represents the normal condition of D. vittatus natural populations (Cigliano & Otte, Reference Cigliano and Otte2003). However, Turk & Barrera (Reference Turk and Barrera1979) observed that, under certain laboratory conditions, some eggs, in a population captured at low latitude, hatched without undergoing diapause and produced a second generation, although hatching rate was low (10–15%). Dichroplus vittatus eggs also showed high tolerance to external conditions. Turk & Barrera (Reference Turk and Barrera1979) obtained some hatchlings from eggs that had been kept under uncontrolled room conditions for 2 years after collection. These features may be related to the arid habitat and seasonal conditions to which this species is exposed.
The number of nymphal stadia is five in males and six in females, and the duration of the nymphal cycle varies negatively with temperature, from 51 or 53 days (means for males and females, respectively) at 23.8°C in the field at c. 26°S/65°W and 577 m altitude, to 33 or 36 days at 27° in laboratory conditions (Turk & Barrera, Reference Turk and Barrera1979).
Dichroplus vittatus exhibits the converse patterns to Bergmann's rule with respect to latitude because the body size of this species decreases with increasing latitude. This is similar to a sister species, D. pratensis Bruner, whose body size decreases both with increasing latitude and altitude (Bidau & Martí, Reference Bidau and Martí2006). However, the underlying mechanisms may not be the same for these different taxa.
In D. vittatus, significant positive correlations with temperature (TMEA and TMIN) were observed, and these two parameters were not correlated with latitude. Temperature per se may explain Bergmann's rule in ectotherms (Atkinson & Sibly, Reference Atkinson and Sibly1997; Blanckenhorn & Demont, Reference Blanckenhorn and Demont2004) but not its converse pattern except when temperature operates as an indirect selective factor on body size by limiting nymphal growth and development (Masaki, Reference Masaki1967). Converse patterns are often genetic and probably adaptive (Masaki, Reference Masaki1967, Reference Masaki and Dingle1978; Roff, Reference Roff1980; Blanckenhorn & Fairbairn, Reference Blanckenhorn and Fairbairn1995; Blanckenhorn & Demont, Reference Blanckenhorn and Demont2004), and essentially depend on constraints imposed by season length on growth and development (Masaki, Reference Masaki1967; Roff, Reference Roff1980; Blanckenhorn & Demont, Reference Blanckenhorn and Demont2004). In D. vittatus (and D. pratensis;Bidau & Martí, Reference Bidau and Martí2006), the converse Bergmannian pattern is probably a consequence of these constraints. However, it is not clear why an altitudinal consistent pattern (as in D. pratensis) was not found in D. vittatus. Other climatic factors like precipitation, may play a role in the latitudinal converse cline of D. vittatus (table 4). However, the most consistent correlations were observed between body size and seasonality estimates (table 4) although it must be borne in mind that, while CVP is colinear with latitude, CVT is not. Furthermore, when partial correlations controlling for latitude were performed between body size and CVT, their significance increased (e.g. MBL/CVT, r=−0.630, P=0.005).
How can seasonality be related to body size geographic variation in D. vittatus? An important and seldomly assessed problem is how body size variability might be affected by latitude, altitude and related parameters. Male D. vittatus body length variability increases with latitude while mean and maximum TBL decrease. In the closely related D. pratensis, a similar pattern occurs (Bidau & Martí, Reference Bidau and Martí2006). In the latter case, our proposed hypothesis postulates that smaller individuals occur in marginal habitats where genetic recombination indices are higher due to low chromosome polymorphisms and high chiasma frequencies (Bidau & Martí, Reference Bidau and Martí2002). Thus a higher genetic variability would be available for explaining the higher variation of body size dimensions despite an absolute decrease of body size (Bidau & Marti, Reference Bidau and Martí2005).
This hypothesis is not applicable to D. vittatus in which the frequency of recombination is uniformly low across the range (Bidau & Marti, Reference Bidau and Martí2000). However, the increasing body size, latitudinal variability may be related to the converse Bergmannian pattern if two factors were taken into account: seasonality and sexual size dimorphism.
Seasonality is a powerful determinant of body size variation (Chown & Gaston, Reference Chown and Gaston1999; Chown & Klok, Reference Chown and Klok2003). At higher latitudes and altitudes, ambient temperatures are likely to be lower, reducing the duration of insect growing season (Masaki, Reference Masaki1967). As seasonality tends to increase at higher latitudes and altitudes (Chown & Gaston, Reference Chown and Gaston1999; Körner, Reference Körner2000; Chown & Klok, Reference Chown and Klok2003), the availability of resources is affected, constraining growth and development to a degree depending on length of generation time relative to length of season (Chown & Klok, Reference Chown and Klok2003). With constant generation time across the geographic range of D. vittatus, resource limitations to development and growth will increase towards latitudes, with more seasonal climates producing smaller body sizes. This interpretation is supported by the plasticity in development observed under different laboratory conditions described above. The lack of altitudinal clines in this species is probably a consequence of the lack of correlation between altitude and seasonality within the study area.
Furthermore, D. vittatus is female-biased, size-dimorphic, although sexual size dimorphism varies across the geographic range following Rensch`s rule (C.J. Bidau & D.A. Martí, unpublished). Part of this sexual size dimorphism occurs due to protandry (Morbey & Ydenberg, Reference Morbey and Ydenberg2001) because males emerge and mature before females, a strategy for early access to females during the breeding season (Andersson, Reference Andersson1994; Morbey & Ydenberg, Reference Morbey and Ydenberg2001; C.J. Bidau & D.A. Martí, Reference Bidau and Martí2006, unpublished). This leaves males with less time to grow than females in all parts of the range, although this constraint is more stringent at higher than at lower latitudes, contributing to the smaller size of males towards the South. Laboratory experiments have shown sex differences in the time of development in different controlled conditions (Turk & Barrera, Reference Turk and Barrera1979). Natural variability for development and maturing time also occurs among males, while females tend to be more homogeneous (Turk & Barrera, Reference Turk and Barrera1979; Cigliano & Otte, Reference Cigliano and Otte2003; Bidau & Martí, Reference Bidau and Martí2006). Furthermore, with increasing latitude, populations occupy increasingly marginal, more seasonal, heterogeneous, changing and unpredictable habitats that simultaneously account for the latitudinal decrease in male body size and increase in body size variability. In low latitudes and less seasonal habitats, although protandry also occurs, the longer growing season and a greater availability of resources might have an homogenizing effect on body size, reducing variability among males although producing larger individuals of both sexes.
Several body dimensions also showed significant latitudinal clines, mainly involving the modification of pronotum morphology and a relative decrease of tegmina length. Since these insects are brachypterous and flightless, the significance of these variations are obscure.
Thus, converse Bergmannian clines may in principle result from climatic selection on the duration of the nymphal stage which indirectly brings about larger adult sizes at lower latitudes (Masaki, Reference Masaki1967, Reference Masaki and Dingle1978; Roff, Reference Roff1980; Blanckenhorn & Demont, Reference Blanckenhorn and Demont2004). We thus conclude that the converse Bergmannian clines of D. vittatus and the increase of body size variability in males result from a combination of a shorter growing season, increasing seasonality limiting available resources, and sexual size dimorphism resulting, partially, from protandry.
Acknowledgements
CJB is very grateful to the Conselho Nacional de Desenvolvimento Científico e Tecnológico (CNPq) of Brazil for a Visiting Researcher's grant that allowed the completion of this paper and to his family and all Brazilian friends that supported his modest research during hard times. The authors wish to specially thank Prof. Héctor Seuánez and Dr Rocío Hassan for kindly reviewing the manuscript. The expert comments of the associate editor and two anonymous reviewers substantially improved the manuscript.