Introduction
Fluctuations in the composition and distribution of Pennsylvanian (323–299 Ma) vegetation in the lowlands of tropical Euramerica were intricately linked to climatic and glacioeustatic oscillations that occurred on a variety of spatial and temporal scales (DiMichele et al., Reference DiMichele, Pfefferkorn and Gastaldo2001a, Reference DiMichele, Montañez, Poulsen and Tabor2009, Reference DiMichele, Cecil, Montañez and Falcon-Lang2010a; Falcon-Lang, Reference Falcon-Lang2003a, Reference Falcon-Lang2004; Poulsen et al., Reference Poulsen, Pollard, Montañez and Rowley2007; Cecil et al., Reference Cecil, DiMichele and Elrick2014; DiMichele, Reference DiMichele2014). When the climate was perhumid (everwet) to subhumid with limited seasonal dryness, the wetland biome flourished in peat-forming mires (a peat-substrate wetland, sensu Greb et al., Reference Greb, DiMichele and Gastaldo2006) and associated clastic substrates over enormous tracts of equatorial Euramerica (Greb et al., Reference Greb, Andrews, Eble, DiMichele, Cecil and Hower2003; Eble et al., Reference Eble, Grady and Pierce2006). Wetland communities, which were dominated by lycopsids, sphenopsids, ferns, pteridosperms, and wetland cordaitaleans (DiMichele and Phillips, Reference DiMichele and Phillips1994), have come to epitomize Pennsylvanian ecosystems because the plant-fossil record is strongly biased towards wet and waterlogged substrates, where burial and long-term preservation is enhanced (DiMichele and Gastaldo, Reference DiMichele and Gastaldo2008; Gastaldo and Demko, Reference Gastaldo and Demko2010). In contrast, intensified seasonality under a subhumid to semiarid climate witnessed expansion of the dryland biome into the lowlands, thereby forcing wetland vegetation into restricted refugia (Falcon-Lang and DiMichele, Reference Falcon-Lang and DiMichele2010; Looy et al., Reference Looy, Stevensen, Van Hoof and Mander2014a). The gymnosperm-dominated dryland floras, which included pteridosperms, dryland cordaitaleans, primitive conifers, and numerous plants of ambiguous systematic affinity (DiMichele and Aronson, Reference DiMichele and Aronson1992; Kerp, Reference Kerp1996; DiMichele et al., Reference DiMichele, Kerp, Tabor and Looy2008, Reference DiMichele, Cecil, Montañez and Falcon-Lang2010a), are poorly represented in Pennsylvanian strata because they occupied moisture-deficient habitats, where susceptibility to oxidative decay limited their preservation potential. However, despite the taphonomic megabiases that lead to overrepresentation of wetland floras in the plant-fossil record, tenure of the dryland biome in equatorial lowlands of Euramerica may have exceeded that of the more conspicuous wetland ecosystems in any given glacial-interglacial cycle (Falcon-Lang et al., Reference Falcon-Lang, Nelson, Looy, Ames, Elrick and DiMichele2009).
Although there is general consensus on the relationship between Pennsylvanian climate and the prevailing vegetation in tropical lowlands (see Bashforth et al., Reference Bashforth, Cleal, Gibling, Falcon-Lang and Miller2014, table 1), disagreements persist on the placement and dominance of the wetland versus dryland biome in glacioeustatic context (e.g., Falcon-Lang and DiMichele, Reference Falcon-Lang and DiMichele2010, fig. 1). One hypothesis contends that dryland vegetation typified sea-level lowstand and early transgression, whereas wetland floras dominated during late transgression and sea-level highstand (Falcon-Lang, Reference Falcon-Lang2003a, Reference Falcon-Lang2004; Dolby et al., Reference Dolby, Falcon-Lang and Gibling2011). This paradigm hinges on the interpretation that Pennsylvanian interglacials were akin to those of the Pleistocene, and recorded the wettest part of a cycle (but see Bush and de Oliviera, Reference Bush and de Oliveira2006; Boyce et al., Reference Boyce, Lee, Field, Brodribb and Zwieniecki2010), fostering widespread peat accumulation (the precursor to economic coal seams). Glacial phases, on the other hand, are inferred to have been seasonally dry, with evapotranspiration exceeding precipitation over part of the year, resulting in deposition and soil formation under oxidizing conditions and associated paleovalley incision (Gibling and Bird, Reference Gibling and Bird1994; Tandon and Gibling, Reference Tandon and Gibling1994; Allen et al., Reference Allen, Fielding, Gibling and Rygel2011). This model is not linked to an independent explanation of climate control. Rather, peat accumulation generally is attributed to the effects of rising sea level and coastal paludification (e.g., Soreghan, Reference Soreghan1994; Aitkin and Flint, Reference Aitken and Flint1995; Heckel, Reference Heckel1995; Bohacs and Suter, Reference Bohacs and Suter1997; Olszewski and Patzkowsky, Reference Olszewski and Patzkowsky2003; Gibling et al., Reference Gibling, Saunders, Tibert and White2004; Jerrett et al., Reference Jerrett, Flint, Davies and Hodgson2011), with a perhumid climate invoked post hoc for intervals where coal is present.
An alternative hypothesis asserts that extensive peatlands developed during late stages of sea-level lowstand at glacial maximum, and then began to fragment at the onset of marine transgression. In contrast, the dryland biome was predominant through the remainder of a glacial-interglacial cycle, particularly under the variably seasonal conditions that coincided with interglacial phases during late transgression through regression to early lowstand (Gastaldo et al., Reference Gastaldo, Demko and Liu1993; DiMichele et al., Reference DiMichele, Cecil, Montañez and Falcon-Lang2010a; DiMichele, Reference DiMichele2014). This interpretation is explicitly tied to independent climate models that incorporate teleconnections among atmospheric circulation, glacial dynamics, and sea level oscillations (e.g., Cecil, Reference Cecil1990, Reference Cecil2003; Cecil et al., Reference Cecil, Dulong, West, Stamm, Wardlaw and Edgar2003, Reference Cecil, DiMichele and Elrick2014; Poulsen et al., Reference Poulsen, Pollard, Montañez and Rowley2007; Eros et al., Reference Eros, Montañez, Osleger, Davydov, Nemyrovska, Poletaev and Zhykalyak2012; Horton et al., Reference Horton, Poulsen, Montañez and DiMichele2012). Hence, while both of these models recognize the cyclical alternation of dryland and wetland vegetation in the lowlands of equatorial Euramerica, the interpreted positions of the Pennsylvanian biomes in a glacioeustatic framework are ‘out of phase.’
This contribution focuses on an unusual Middle Pennsylvanian (middle Moscovian) macrofloral assemblage collected from a distinct lithological unit below the Minshall Coal of Indiana, situated in the uppermost Brazil Formation on the eastern margin of the Illinois Basin. The taphocoenosis is significant because it is dominated by elements of the underrepresented dryland biome, including Cordaites Unger, Reference Unger1850, Lesleya Lesquereux, 1879-80, and Taeniopteris Brongniart, Reference Brongniart1828a, which are broad-leaved gymnosperms with mesophytic to xerophytic characteristics that are consistent with sedimentological indicators of deposition under a regime of strongly seasonal precipitation. The presence of Taeniopteris is noteworthy because the fossil-genus is more typical of Late Pennsylvanian and Permian dryland floras (Kerp and Fichter, Reference Kerp and Fichter1985; DiMichele et al., Reference DiMichele, Cecil, Montañez and Falcon-Lang2010a). This occurrence may be the earliest known in the plant-fossil record. A detailed descriptive and illustrated account of the macrofloral assemblage is the centerpiece of the paper, although the microfloral assemblage in the fossiliferous unit also is tabulated. Homotaxial biostratigraphic comparisons with established American and European biozonation schemes indicate that the dryland unit accumulated close to the Atokan-Desmoinesian Stage boundary, which has been correlated with the Bolsovian-Asturian Substage boundary of Western Europe (Peppers, Reference Peppers1996; Blake et al., Reference Blake, Cross, Eble, Gillespie and Pfefferkorn2002). This age is compatible with biostratigraphic analyses of macrofloral and microfloral assemblages from the overlying Minshall Coal and its ‘roof-shales’ at the same locality (Bashforth et al., Reference Bashforth, DiMichele, Eble and Nelson2016). Furthermore, the stratigraphic proximity of the dryland unit to wetland deposits recorded by the Minshall Coal permits assessment of the changeover between the dryland and wetland biomes in a single glacial-interglacial cycle, and helps clarify the paleobiogeographic and paleoecologic context of dryland ecosystems in the tropical lowlands of Pennsylvanian Euramerica.
Geologic setting
Illinois Basin
The Illinois Basin (also termed the Eastern Interior Basin), an interior cratonic basin (Leighton et al., Reference Leighton, Kolata, Oltz and Eidel1991), is one of several large depocenters in the USA that contain coal-bearing strata of predominantly Pennsylvanian age (Fig. 1.1). In its present configuration, the basin underlies much of Illinois and parts of western Indiana and western Kentucky, with small outliers in Iowa and Missouri (Fig. 1.2). The Illinois Basin contains a largely siliciclastic succession of Morrowan to Virgilian (Bashkirian to Gzhelian, upper Namurian to upper Stephanian) strata (Nelson et al., Reference Nelson, Trask, Jacobson, Damberger, Williamson and Williams1991, Reference Nelson, Greb and Weibel2013), which accumulated on a low-gradient continental platform (Watney et al., Reference Watney, Wong and French1989; Greb et al., Reference Greb, Andrews, Eble, DiMichele, Cecil and Hower2003). Glacioeustatic fluctuations resulted in cyclic transgressions of shallow epeiric waters from the west (Wanless and Shepard, Reference Wanless and Shepard1935, Reference Wanless and Shepard1936; Heckel, Reference Heckel1986; Langenheim and Nelson, Reference Langenheim and Nelson1992; Algeo and Heckel, Reference Algeo and Heckel2008), and in accord with its paleogeographic position, depositional environments in the Illinois Basin are intermediate between marine-dominated rocks of the Western Interior (Midcontinent) Basin and mainly deltaic to terrestrial strata of the Appalachian Basin to the east (Fig. 1.1; Heckel, Reference Heckel1977, Reference Heckel1980; Nelson et al., Reference Nelson, Greb and Weibel2013). These basins developed in a paleoequatorial setting in the west-central part of Euramerica (Witzke, Reference Witzke1990). During the Atokan-Desmoinesian transition, they were separated from eastern areas of the paleocontinent (present-day Europe) by uplands of the Laurentian Shield and, in part, by the Appalachian-Variscan Mountains (Blakey, Reference Blakey2013).
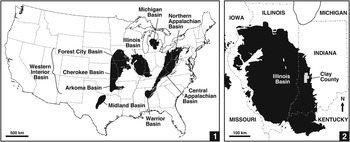
Figure 1 Basins in contiguous USA that contain coal-bearing strata of Pennsylvanian age: (1) Western Interior, Illinois, Appalachian, and Michigan basins, and their components; (2) Illinois Basin, and position of Clay County, Indiana on eastern margin of basin.
Study locality
The study area is situated in Clay County, west-central Indiana, on the eastern margin of the Illinois Basin (Fig. 1.2), where the thin and laterally discontinuous Lower Block, Upper Block, and Minshall coals of the upper Atokan Brazil Formation (Fig. 2) have been exploited due to their low-to-medium sulfur and ash content (Mastalerz et al., Reference Mastalerz, Padgett and Eble2000, Reference Mastalerz, Ames and Padgett2003; Kvale et al., Reference Kvale, Mastalerz, Furer, Engelhardt, Rexroad and Eble2004). The Brazil Formation, which extends from the base of the Lower Block Coal to the top of the Minshall Coal (Hutchison, Reference Hutchison1976), is equivalent to the middle part of the Tradewater Formation of Illinois and Kentucky (The Tri-State Committee on Correlation of the Pennsylvanian System in the Illinois Basin, 2001). In Indiana, strata above the Minshall Coal are assigned to the Staunton Formation, which includes the Perth Limestone, the top of which defines the Atokan-Desmoinesian Stage boundary based on palynology (Peppers, Reference Peppers1996). The Minshall Coal and overlying Perth Limestone correlate with the Rock Island (No. 1) Coal and Seville Limestone of northwestern Illinois and the Empire Coal and Curlew Limestone of southwestern Kentucky based on microfloral and marine-microfossil content (Fig. 2; Shaver, Reference Shaver1984; Douglass, Reference Douglass1987; Peppers, Reference Peppers1996; Rexroad et al., Reference Rexroad, Brown, Devera and Suman1998; Tri-State Committee, 2001; Nelson et al., Reference Nelson, Greb and Weibel2013).
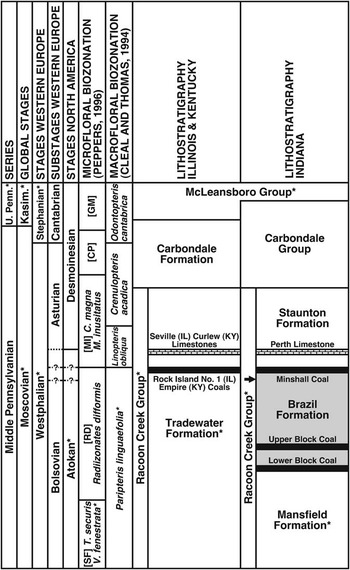
Figure 2 Stratigraphy of Middle Pennsylvanian of the Illinois Basin, showing correlation between chronostratigraphic, lithostratigraphic, and biostratigraphic units (* indicates incomplete unit). Compiled from Cleal and Thomas (Reference Cleal and Thomas1994, figs. 92–94), Peppers (Reference Peppers1996, pl. 1), Davydov et al. (Reference Davydov, Crowley, Schmitz and Poletaev2010, fig. 2), Falcon-Lang et al. (Reference Falcon-Lang, Heckel, DiMichele, Blake, Easterday, Eble, Elrick, Gastaldo, Greb, Martino, Nelson, Pfefferkorn, Phillips and Rosscoe2011a, fig. 1), and Nelson et al. (Reference Nelson, Greb and Weibel2013, fig. 2). Brazil Formation (shaded) incorporates strata from base of Lower Block Coal to top of Minshall Coal. Macrofloral assemblage collected from uppermost Brazil Formation (arrow). Atokan-Desmoinesian Stage boundary (solid line) and Bolsovian-Asturian Substage boundary (dotted line) considered coeval by Peppers (Reference Peppers1996) and equivalent to top of Perth, Seville, and Curlew limestones, but biostratigraphic data of Bashforth et al. (Reference Bashforth, DiMichele, Eble and Nelson2016) indicate one or both boundaries may be at top of Minshall Coal or at lower level (dashed lines with ?). Microfloral biozones: Torispora securis-Vestispora fenestrata (SF), Cadiospora magna-Mooreisporites inusitatus (MI), Schopfites colchesterensis-Thymospora pseudothiessenii (CP), and Lycospora granulata-Granasporites medius (GM).
The macrofloral assemblage was collected from a small open-pit mine north of Indiana Route 46, the now reclaimed and inaccessible Ashboro Pit (SW 1/4, SE 1/4, Section 17, Township 11N, Range 6W) operated by Log Cabin Coal Company. DiMichele et al. (Reference DiMichele, Cecil, Montañez and Falcon-Lang2010a, p. 338) provided a general description of the locality. The Lower Block and Upper Block coals were being mined during a reconnaissance in 1980, when plant fossils were collected, but the pit was idle and partly filled with water during a 1982 visit. Sketches of the west-facing and north-facing highwalls of the L-shaped pit are reproduced schematically in Figure 3. About 15 m of the Brazil and overlying Staunton formations were exposed, the lithostratigraphic boundary being the top of the Minshall Coal.
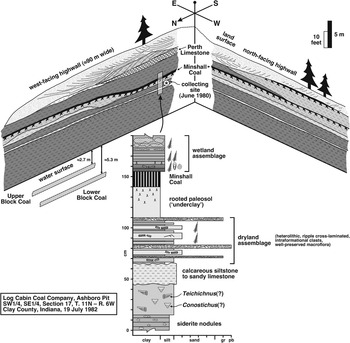
Figure 3 Schematic drawing of succession exposed in Ashboro Pit (now inaccessible open-pit mine of Log Cabin Coal Company; SW 1/4, SE 1/4, Section 17, Township 11N, Range 6W), north of Indiana Route 46, Clay County, Indiana, during visits in June 1980 and July 1982. Brazil Formation incorporates strata from base of Lower Block Coal (under water) to top of Minshall Coal, and overlying strata belong to Staunton Formation. Top of Perth Limestone corresponds to Atokan-Desmoinesian boundary (Peppers, Reference Peppers1996). ‘Dryland assemblage’ documented herein collected from heterolithic unit (USNM locality 38353) between calcareous unit (of presumed marine origin) and paleosol (‘underclay’) below Minshall Coal on west-facing highwall. Bashforth et al. (Reference Bashforth, DiMichele, Eble and Nelson2016) documented ‘wetland assemblage’ from roof-shales above Minshall Coal.
Plant fossils were collected from a heterolithic unit (USNM Locality 38353) situated directly beneath the paleosol (‘underclay’) of the Minshall Coal on the west-facing highwall (Fig. 3), and thus belong to the uppermost Brazil Formation. According to the microfloral biozonation scheme of Peppers (Reference Peppers1996), the stratum is upper Atokan, correlative with the upper Bolsovian of Western Europe, and equivalent to the uppermost Radiizonates difformis (RD) Biozone (Fig. 2). However, Bashforth et al. (Reference Bashforth, DiMichele, Eble and Nelson2016) evaluated microfloral and macrofloral assemblages in the Minshall Coal and overlying roof-shales (‘wetland assemblage’ in Figure 3) at the locality, and proposed that one or both of the Atokan-Desmoinesian and Bolsovian-Asturian boundaries (equivalent, according to Peppers, Reference Peppers1996) may be positioned at the top of the coal seam or somewhere beneath its paleosol (demonstrated by the uncertainties in Figure 2). In this paper, and in accord with the two scenarios favored by Bashforth et al. (Reference Bashforth, DiMichele, Eble and Nelson2016, fig. 7.4, 7.5), the Atokan-Desmoinesian boundary is retained at the top of the Perth Limestone, whereas the Bolsovian-Asturian boundary is either situated at the top of the Minshall Coal or at a level approximating the fossiliferous unit documented here (Fig. 2). Based on this stratigraphic framework, the dryland assemblage is late Atokan and either latest Bolsovian or earliest Asturian in age (see Biostratigraphic correlations and age section).
Fossiliferous unit
Two distinct beds underlie the plant-fossil-bearing unit (Fig. 3). Immediately below the fossiliferous package is a laterally extensive bed that varies from sandy dolomite or limestone to calcareous siltstone, and that has a nodular texture and knobby upper and lower surfaces. Beneath this is a thoroughly bioturbated siltstone that contains possible Conostichus Lesquereux, Reference Lesquereux1876 and large gutter-stacked burrows similar to Teichichnus Seilacher, Reference Seilacher1955. These ichnogenera indicate restricted-marine to brackish conditions for the siltstone (Devera, Reference Devera1989; Archer et al., Reference Archer, Feldman, Kvale and Lanier1994; Buatois et al., Reference Buatois, Gingras, Maceachern, Mángano, Zonneveld, Pemberton, Netto and Martin2005), and a marine-influenced setting is equally probable for the calcareous bed above it.
The heterolithic unit itself (~45 cm thick) consists of irregularly arranged interbeds of medium-gray siltstone and light-gray, very fine- to fine-grained sandstone, between which thin, dark-gray shale laminae are interspersed. Coarser beds may be ripple cross-laminated, and horizons of granule- to pebble-size, subangular to subrounded, intraformational clasts (claystone, shale, siderite? nodules) occur sporadically. The largest and best-preserved macrofloral remains are concentrated along shale laminae, whereas large, partially flattened, coalified axes and fragmentary foliage are more common in siltstone, sandstone, and intraclast-rich beds. Macroscopic (mm- to cm-scale) fusain fragments (charcoal) are abundant.
Numerous features in the fossiliferous unit point to accumulation under variable flow conditions, particularly the irregularly arranged interbeds of strikingly different lithology. For example, a thin shale lamina with well-preserved and whole fossil leaves can rest directly atop a sandstone interbed with ripple cross-laminations and large axis fragments. Such a relationship records a rapid shift from moderately high-energy flow, probably during a flood pulse, to low-energy conditions when entrained plant remains settled from suspension as floodwaters waned. The presence of intraclast-rich horizons implies exposure and erosion of local substrates, probably during flashflood events. In addition, the abundance of macroscopic charcoal fragments indicates that the source vegetation was subjected to intense oxidation (presumably by wildfires; Scott, Reference Scott2010) prior to transport and burial. All of these criteria support the interpretation that the heterolithic unit that contains the dryland assemblage accumulated under a regime of highly seasonal precipitation, probably typified by a protracted, fire-prone dry season and a short wet season with torrential rainfall and flashy discharge.
The genetic relationship between the fossiliferous unit and subjacent rocks is uncertain. However, the fact that a decidedly terrestrial package rests directly on marine-influenced strata points to an interval of subaerial exposure and erosion of the coastal plain after withdrawal, a consequence of regression on approach to a glacial phase. Likewise, although the heterolithic bed is sheet-like over the extent of the highwall face, the unit may have been deposited in a shallow and narrow drainage network, possibly lateral to a coeval paleosol (e.g., Feldman et al., Reference Feldman, Franseen, Joeckel and Heckel2005).
The plant-fossil-bearing bed is directly overlain by a light-gray (gleyed), rooted paleosol, which is in turn capped by the Minshall Coal, here only 15 cm thick. The coal seam is relatively uniform in thickness but undulatory due to irregularities on the subjacent paleosol surface. The paleosol is interpreted as the product of prolonged subaerial exposure and soil development under a seasonal precipitation regime in late stages of regression. However, thereafter the paleosol was overprinted by gleyed features, reflecting the mobilization and removal of iron from the free-draining soil as precipitation increased (Driese and Ober, Reference Driese and Ober2005; Rosenau et al., Reference Rosenau, Tabor, Elrick and Nelson2013a, Reference Rosenau, Tabor, Elrick and Nelson2013b). As base level rose through the transition to perhumid climatic conditions, the abundant and equable rainfall led to paludification and peat buildup during sea-level lowstand at maximum glaciation (Cecil et al., Reference Cecil, Stanton, Neuzil, Dulong, Ruppert and Pierce1985, Reference Cecil, Dulong, West, Stamm, Wardlaw and Edgar2003, Reference Cecil, DiMichele and Elrick2014; Cecil, Reference Cecil1990; Rosenau et al., Reference Rosenau, Tabor, Elrick and Nelson2013b, DiMichele, Reference DiMichele2014). Significantly, the vertical proximity of the heterolithic unit and superjacent paleosol, which formed during late regression, and the overlying Minshall Coal provides strong supporting evidence for the ‘lowstand-coal model.’
Floral assemblages
Macrofloral Assemblage
Plant fossils were collected in June 1980, and some components of the assemblage were included in a dissertation that documented a so-called ‘upland’ flora from the Ashboro Pit (Comer, Reference Comer1992). However, this thesis combined specimens from several stratigraphic levels and depositional facies, including roof-shales of the Minshall Coal (‘wetland assemblage’ in Fig. 3; Bashforth et al., Reference Bashforth, DiMichele, Eble and Nelson2016) and the Upper Block Coal, which explains the mixed ‘lowland-upland’ floristic signature identified by Comer (Reference Comer1992).
Table 1 presents a quantitative breakdown of the rock slabs and fossil-taxa (sensu Cleal and Thomas, Reference Cleal and Thomas2010) found in the macrofloral assemblage. Of the 41 slabs collected, 72 slab faces (excluding barren faces and counterparts) bear macrofloral remains. The quantification technique used is a variant of that described by Pfefferkorn et al. (Reference Pfefferkorn, Mustafa and Hass1975): each slab face was treated as a quadrat, and a fossil-taxon was recorded as ‘present,’ regardless of the number of specimens on the face. Through this process, 179 macrofloral occurrences were recorded on the 72 fossiliferous slab faces. Excluding seeds and some axes, which could not be tied to a fossil-plant taxon with confidence (‘unidentifiable’ macrofloral occurrences in Table 1), there were 109 ‘identifiable’ macrofloral occurrences.
Table 1 Fossil-taxa in macrofloral assemblage, from heterolithic unit below Minshall Coal, segregated into plant groupings, and showing typical habitat. Symbols: (a) total slabs in collection; (b) slab faces with macrofloral remains (i.e., excludes barren faces and counterparts); (c) identifiable macrofloral occurrences+‘unidentifiable’ macrofloral occurrences (e.g., axes and seeds); (d) occurrence indicates presence (in any quantity) on slab face; (e) ‘biological’ fossil-taxa+‘non-biological’ fossil-taxa (Calamites Brongniart, Reference Brongniart1828b [sp. indet.] and Calamostachys Schimper, Reference Schimper1869 [sp. indet.]); (f) reassembled conceptualization of once-living, whole fossil-plant. See text for details.
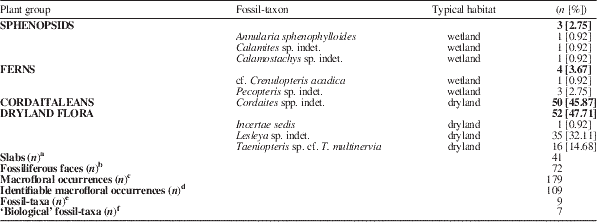
Of the nine fossil-taxa identified in the low-diversity assemblage (Table 1), seven are considered ‘biological’ taxa (i.e., a reassembled conceptualization of a whole fossil plant). The only ‘non-biological’ taxa are Calamites Brongniart, Reference Brongniart1828b (sp. indet.) and Calamostachys Schimper, Reference Schimper1869 (sp. indet.), a sphenopsid axis and ‘cone,’ respectively. The assemblage is co-dominated by cordaitaleans (45.9%), all being included in the fossil-leaf genus Cordaites spp. indet., and enigmatic dryland plants (47.7%) that belong to three biological taxa: (1) leaves assigned to Lesleya sp. indet. (32.1%), which compare most closely with Lesleya grandis Lesquereux, 1879-80 or Lesleya cheimarosa Leary and Pfefferkorn, Reference Leary and Pfefferkorn1977; (2) leaves of Taeniopteris sp. cf. T. multinervia Weiss, Reference Weiss1869 (14.7%); and (3) an unusual specimen referred to Incertae sedis (0.9%). Ferns (3.7%) are represented by two biological species: cf. Crenulopteris acadica (Bell, Reference Bell1962) Wittry et al., Reference Wittry, Glasspool, Béthoux, Koll and Cleal2014 and Pecopteris (Brongniart, Reference Brongniart1822) Sternberg, Reference Sternberg1825 sp. indet.). Sphenopsids are equally rare (2.8%), with only one biological species, Annularia sphenophylloides (Zenker, Reference Zenker1833) Gutbier, Reference Gutbier1837. Identifiable lycopsid and pteridosperm organs are conspicuously absent.
Microfloral Assemblage
A sample of the fossiliferous unit was prepared for palynological analysis using the method described by Bashforth et al. (Reference Bashforth, DiMichele, Eble and Nelson2016). Slides, maceration residues, and unused portions are stored at the Kentucky Geological Survey, University of Kentucky (Lexington, KY) under accession number KGS 3262.
The results of palynological analysis are presented in Table 2, which gives a quantitative breakdown of the plant groups and fossil-taxa. Thirty-one miospore species were identified, only one of unknown affinity. The unit is dominated by tree-fern spores (57.2%), particularly Punctatisporites minutus (Kosanke, Reference Kosanke1950) Peppers, Reference Peppers1964, and the key biostratigraphic indicator Thymospora pseudothiessenii (Kosanke, Reference Kosanke1950) Wilson and Venkatachala, Reference Wilson and Venkatachala1963 occurs in statistically significant abundance (7.2%). A diverse array of spores derived from non-arborescent ferns (15.2%) and three genera of arborescent lycopsids (10.4%; Lepidophloios Sternberg, Reference Sternberg1825, Paralycopodites Morey and Morey, Reference Morey and Morey1977, Lepidodendron Sternberg, Reference Sternberg1820) are present, as are two types of cordaitalean pollen (10.4%). Spores of sub-arborescent lycopsids (4.0%) and calamitaleans (2.0%) are rare. The sample contained no pteridosperm pollen, despite targeted searches in the maceration residues. The mismatch between the dominance-diversity profiles of the microfloral and macrofloral assemblages follows an emerging pattern as comparative studies are carried out elsewhere on Pennsylvanian and younger strata (e.g., Mander et al., Reference Mander, Kürschner and McElwain2010; Looy et al., Reference Looy, Stevensen, Van Hoof and Mander2014a; Looy and Hotton, Reference Looy and Hotton2014). Because this paper focuses on the macrofloral assemblage, palynological data are only used for complementary biostratigraphic control.
Table 2 Fossil-taxa in microfloral assemblage, from heterolithic unit below Minshall Coal, segregated into plant groupings/growth forms.
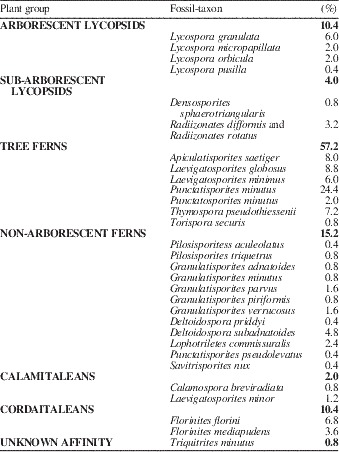
Biostratigraphic correlations and age
Macrofloral Assemblage
Cordaites, the most common biological taxon in the assemblage (Table 1), ranges through the late Paleozoic in both dryland and wetland assemblages in Euramerica and offers no biostratigraphic control at the genus level. Lesleya is an archetypal component of the dryland biome in Lower to Middle Pennsylvanian deposits (DiMichele et al., Reference DiMichele, Cecil, Montañez and Falcon-Lang2010a), although the fossil-genus has a spotty distribution from the Late Mississippian to late Permian (Šimůnek, Reference Šimůnek1996). One of two fossil-species probably is present: Lesleya grandis or Lesleya cheimarosa. According to Leary and Pfefferkorn (Reference Leary and Pfefferkorn1977, p. 25), the stratum typicum of L. grandis is the “level of the Rock Island (No. 1) Coal” (i.e., a correlate of the Minshall Coal; Fig. 2), and the taxon also may be present in the Bolsovian of the Czech Republic (Šimůnek, Reference Šimůnek1996). In contrast, L. cheimarosa is best known from basal Morrowan (Namurian B or C) rocks of western Illinois (Leary and Pfefferkorn, Reference Leary and Pfefferkorn1977; Leary, Reference Leary1981). Comer (Reference Comer1992) identified specimens purported to have come from directly above the Upper Block Coal (upper Atokan, middle? Bolsovian) at the locality (Figs. 2, 3), although as mentioned above, the source horizon is unclear. On the other hand, Taeniopteris is more typical of Late Pennsylvanian and Permian dryland floras (DiMichele et al., Reference DiMichele, Cecil, Montañez and Falcon-Lang2010a), and Taeniopteris multinervia has only been confirmed in uppermost Pennsylvanian to lower Permian strata of Europe and China (Seward, Reference Seward1910; Halle, Reference Halle1927a; Kerp and Fichter, Reference Kerp and Fichter1985; Opluštil et al., Reference Opluštil, Šimůnek, Zajíc and Mencl2013), with unsubstantiated records in the USA (Darrah, Reference Darrah1935). If the identification of T. multinervia in the assemblage could be confirmed, the first appearance of the species would be about 10 Ma earlier than known to date.
Only two biological taxa in the macrofloral assemblage, Annularia sphenophylloides and cf. Crenulopteris acadica, are characteristic of wetland floras. Crenulopteris acadica, used sensu Wittry et al. (Reference Wittry, Glasspool, Béthoux, Koll and Cleal2014), as a replacement for what Wagner (Reference Wagner1958, Reference Wagner1971) identified as Lobatopteris vestita (Lesquereux, 1879-80) Wagner, Reference Wagner1958, occurs in lower Desmoinesian to lower Virgilian rocks in the USA, but is most common in upper Desmoinesian to Missourian rocks (Darrah, Reference Darrah1969; Blake et al., Reference Blake, Cross, Eble, Gillespie and Pfefferkorn2002; Wittry et al., Reference Wittry, Glasspool, Béthoux, Koll and Cleal2014). A comparable epibole of late Asturian to Cantabrian age is known for Europe and Atlantic Canada (Wittry et al., Reference Wittry, Glasspool, Béthoux, Koll and Cleal2014), although the species may extend down to the uppermost Bolsovian in the Sydney Coalfield (Bell, Reference Bell1938). Annularia sphenophylloides is long ranging, occurring from the late Atokan to late Virgilian in the USA, with most examples from the Desmoinesian and above (Abbott, Reference Abbott1958; Gillespie and Pfefferkorn, Reference Gillespie and Pfefferkorn1979; Blake et al., Reference Blake, Cross, Eble, Gillespie and Pfefferkorn2002). Likewise, the species is common in lower Bolsovian to lower Cantabrian strata in the UK and Atlantic Canada (Bell, Reference Bell1938; Cleal and Thomas, Reference Cleal and Thomas1994), but can range to uppermost Pennsylvanian in Europe (Wagner, Reference Wagner1984).
This analysis indicates that the macrofloral assemblage provides little clarity on the age of the fossiliferous unit (i.e., if the Bolsovian-Asturian boundary lies above or below the bed; Fig. 2; Bashforth et al., Reference Bashforth, DiMichele, Eble and Nelson2016). Such ambiguous results are partly a consequence of the low diversity and number of tentative specific assignments, and the fact that the assemblage contains a mixture of elements drawn from the dryland and wetland biomes compounds the uncertainty. The precise stratigraphic distribution of most dryland plants is poorly known, primarily due to their rarity in the fossil record on account of the preservational megabias against organic matter in seasonally dry habitats (DiMichele and Gastaldo, Reference DiMichele and Gastaldo2008; Gastaldo and Demko, Reference Gastaldo and Demko2010; Looy et al., Reference Lyons and Darrah2014b), and because dryland communities mainly are comprised of derived taxa of uncertain systematic affinity (DiMichele and Aronson, Reference DiMichele and Aronson1992; DiMichele et al., Reference DiMichele, Kerp, Tabor and Looy2008, Reference DiMichele, Cecil, Montañez and Falcon-Lang2010a). Likewise, existing biozonation schemes for Euramerican macrofloras (e.g., Read and Mamay, Reference Read and Mamay1964; Wagner, Reference Wagner1984; Cleal, Reference Cleal1991; Cleal and Thomas, Reference Cleal and Thomas1994) were largely constructed based on homotaxial comparisons of wetland assemblages collected from isotaphonomic facies, and do not take into account the possibility of prior origination outside of wetland habitats (DiMichele and Aronson, Reference DiMichele and Aronson1992; Kerp, Reference Kerp1996; DiMichele et al., Reference DiMichele, Kerp, Tabor and Looy2008; Bashforth et al., Reference Bashforth, Cleal, Gibling, Falcon-Lang and Miller2014; Looy et al., Reference Looy, Kerp, Duijnstee and DiMichele2014b). Accordingly, even though typical wetland plants may be present in the macrofloral assemblage, the full temporal range of those species is uncertain, making biostratigraphic correlations tenuous (Falcon-Lang et al., Reference Falcon-Lang, Heckel, DiMichele, Blake, Easterday, Eble, Elrick, Gastaldo, Greb, Martino, Nelson, Pfefferkorn, Phillips and Rosscoe2011a). Nonetheless, if the presence of Annularia sphenophylloides and cf. Crenulopteris acadica is taken at face value, the study unit falls in an age range of late Bolsovian to early Asturian, approximating the first appearance of both species in wetland habitats. These ages are equivalent to the uppermost Paripteris linguaefolia or lowermost Linopteris obliqua biozones, respectively, of the macrofloral biozonation framework used in Western Europe (Fig. 2).
Microfloral Assemblage
According to Bashforth et al. (Reference Bashforth, DiMichele, Eble and Nelson2016), miospores in the Minshall Coal indicate assignment to the upper Atokan Radiizonates difformis (RD) Biozone, the upper boundary of which corresponds to the top of the coal seam (Peppers, Reference Peppers1996). Consequently, the underlying dryland unit must also belong to the biozone, which traditionally has been correlated with the upper Bolsovian (Fig. 2). Nonetheless, the presence of two miospore taxa in the microfloral assemblage challenges this convention and supports the idea that the Bolsovian-Asturian boundary lies below the Minshall Coal (Bashforth et al., Reference Bashforth, DiMichele, Eble and Nelson2016).
The tree-fern spore Thymospora pseudothiessenii is relatively abundant in the microfloral assemblage (Table 2). Although the taxon occurs sparingly in uppermost Bolsovian rocks, it is considered diagnostic of the basal Asturian and reaches its epibole in the middle Asturian Schopfites colchesterensis-Thymospora pseudothiessenii (CP) Biozone (Fig. 2; Peppers, Reference Peppers1996; Lesnikowska and Willard, Reference Lesnikowska and Willard1997). Equally significant is the fact that Savitrisporites nux (Butterworth and Williams, Reference Butterworth and Williams1958) Smith and Butterworth, Reference Smith and Butterworth1967 is present (albeit rare) in the dryland unit (Table 2), but is absent in the overlying Minshall Coal (Bashforth et al., Reference Bashforth, DiMichele, Eble and Nelson2016). Because the last occurrence of the spore is just below the Bolsovian-Asturian boundary (Peppers, Reference Peppers1996), placement of this boundary between the fossiliferous unit and the overlying coal is tenable. If so, the uppermost part of the R. difformis (RD) Biozone would be early Asturian rather than late Bolsovian in age (Fig. 2). It is crucial to note, however, that the biostratigraphic scheme of Peppers (Reference Peppers1996) is primarily based on palynomorphs from coal seams, meaning that many taxon ranges would be extended if clastic lithologies had been incorporated. Nonetheless, the results of this analysis are in line with those determined from the macrofloral assemblage, both indicating accumulation of the dryland unit in the late Bolsovian or early Asturian.
Implications for understanding the dryland biome
Glacioeustatic context
Models that link the cyclical recurrence of wetland and dryland biomes with glacioeustatic, atmospheric, and climatic oscillations propose that the tropical lowlands of Euramerica experienced markedly seasonal precipitation during sea-level regression, an increasingly wetter climate as the lowstand progressed, and the onset of perhumid conditions at glacial maximum during late lowstand (Cecil et al., Reference Cecil, Dulong, West, Stamm, Wardlaw and Edgar2003, Reference Cecil, DiMichele and Elrick2014; Poulsen et al., Reference Poulsen, Pollard, Montañez and Rowley2007; DiMichele et al., Reference DiMichele, Cecil, Montañez and Falcon-Lang2010a; Eros et al., Reference Eros, Montañez, Osleger, Davydov, Nemyrovska, Poletaev and Zhykalyak2012; Horton et al., Reference Horton, Poulsen, Montañez and DiMichele2012; Rosenau et al., Reference Rosenau, Tabor, Elrick and Nelson2013a, Reference Rosenau, Tabor, Elrick and Nelson2013b; DiMichele, Reference DiMichele2014). If true, this dynamic framework should produce a relatively predictable lithological succession, with regional differences contingent on paleogeographic position and background paleoclimatic conditions (e.g., Cecil et al., Reference Cecil, Dulong, West, Stamm, Wardlaw and Edgar2003). On the flat, low-gradient platform of west-central Euramerica, where the Illinois Basin was situated during the Middle Pennsylvanian, polar-ice buildup would have caused rapid withdrawal of epeiric waters and exposure of a shallow-marine to brackish shelf, above which terrestrial deposits should show evidence of erosion from intraformational sources, accumulation under a regime of seasonal precipitation, and the prevalence of dryland vegetation. With seasonal conditions persisting while sea level continued to recede, prolonged exposure of the intracontinental platform would have resulted in the development of a widespread and thick Vertisol or Calcisol. On approach to glacial maximum, an increasingly wet climate would have caused gleying of the preexisting soil, preserved as a paleosol with a polygenetic history. As base level continued to rise and perhumid conditions were reached at late lowstand, paludification and Histosol development should be expressed as an extensive coal seam that blankets the paleosol and contains palynomorphs derived from peat-inhabiting wetland vegetation. Finally, the initial stages of deglaciation would have triggered flooding of the low-gradient peat surface by meltwater pulses during early trangression, which should be manifest as fine-grained clastic rocks that exhibit evidence of deposition in shallow, low-energy, dysoxic, and brackish to hyposaline waters of eustuaries and tidal flats. These roof-shales, which directly overlie the coal seam, should contain the remains of vegetation that occupied clastic wetlands.
The stratigraphic succession below (documented here) and above (Bashforth et al., Reference Bashforth, DiMichele, Eble and Nelson2016) the Minshall Coal at the locality conforms with the aforementioned model (Fig. 3). A thin package of restricted-marine to brackish strata is overlain by a fossiliferous heterolithic unit dominated by dryland plants, reflecting rapid withdrawal of the sea during regression, subaerial exposure and erosion of the marine platform, and deposition under seasonal conditions. Above this, a gray, rooted paleosol with an irregular surface floors the Minshall Coal, the product of gleying, paludification, and ultimately peat accumulation above an undulatory soil horizon as the climate became progressively wetter on approach to late lowstand. Clastic rocks that preserve wetland vegetation overlie the coal seam, and record initial flooding of the mire during early stages of transgression.
Comparable successions are associated with coal seams throughout the Illinois Basin, supporting the concept that the dryland biome dominated tropical lowlands of Euramerica during early to middle lowstand, and that the wetland biome prevailed during late lowstand and early transgession (Cecil et al., Reference Cecil, DiMichele and Elrick2014; DiMichele, Reference DiMichele2014). It is noted, however, that the driest part of a glacial-interglacial cycle, when the dryland biome presumably was most widespread, probably was in late stages of transgression near sea-level highstand (Cecil et al., Reference Cecil, Dulong, West, Stamm, Wardlaw and Edgar2003, Reference Cecil, DiMichele and Elrick2014; Horton et al., Reference Horton, Poulsen, Montañez and DiMichele2012; DiMichele, Reference DiMichele2014). If so, given the grade of the paleosurface in west-central Euramerica, much of the intracontinental platform would have been covered by a shallow, inland sea during Pennsylvanian interglacials (Algeo and Heckel, Reference Algeo and Heckel2008; Archer et al., Reference Archer, Elrick, Nelson and DiMichele2016). Hence, all vegetation would have been pushed to basin-margin positions peripheral to the epeiric sea, and despite its dominance, the preservation potential of the dryland biome would be greatly reduced in rocks deposited during late transgression or highstand.
Dryland vegetation clearly was replaced by wetland vegetation at the locality, but the duration of the transition between the biomes is equivocal. Because the top of the fossiliferous unit that hosts the dryland assemblage is only 30 cm below the top of the overlying paleosol (Fig. 3), it is tempting to assume that the changeover was rapid. However, a direct relationship cannot be drawn between the thickness of a siliciclastic bed and the time it took to accumulate, especially in shallow-marine and terrestrial strata, which largely record hiatuses rather than deposition (Miall, Reference Miall2014). Given that the heterolithic unit accumulated sporadically during seasonal flashfood events, and contains many beds with intraformational clasts eroded from local basement rocks, appreciable time may be represented in the unit. Furthermore, some 103–104 years may have elapsed during the multistage evolution of the mineral paleosol (Hembree and Nadon, Reference Hembree and Nadon2011; Rosenau et al., Reference Rosenau, Tabor, Elrick and Nelson2013b), and a hiatus may exist between the paleosol and overlying Minshall Coal (Scott and Stephens, Reference Scott and Stephens2014). Accordingly, the time involved in the shift from a dryland- to wetland-dominated biome on the coastal plain remains an open question, although it may have been upwards of 104 years.
The nature of the transition from the dryland to wetland biome also is unclear. Because the microfloral assemblage in the dryland unit is dominated by palynomorphs derived from wetland communities, wetland vegetation may have been present somewhere ‘out there,’ perhaps in localized refugia alongside fluvial corridors or other topographic lows in an otherwise seasonally dry landscape (Falcon-Lang et al., Reference Falcon-Lang, Rygel, Gibling and Calder2004; DiMichele et al., Reference DiMichele, Tabor, Chaney and Nelson2006; Falcon-Lang and DiMichele, Reference Falcon-Lang and DiMichele2010; Opluštil et al., Reference Opluštil, Šimůnek, Zajíc and Mencl2013; Bashforth et al., Reference Bashforth, Cleal, Gibling, Falcon-Lang and Miller2014). Such heterogeneity of habitats and vegetation is typical of seasonally dry Pennsylvanian landscapes (DiMichele et al., Reference DiMichele, Cecil, Montañez and Falcon-Lang2010a; Bashforth et al., Reference Bashforth, Drábková, Opluštil, Gibling and Falcon-Lang2011, Reference Bashforth, Cleal, Gibling, Falcon-Lang and Miller2014). At other localities where Pennsylvanian microfloral and macrofloral assemblages have been tracked through short-term changes in background climate, there is evidence for gradual fragmentation of the dryland biome, a period of coexistence, and finally replacement by the wetland biome as the tropical lowlands became wetter throughout a eustatic lowstand (Tabor et al., Reference Tabor, Romanchock, Looy, Hotton, DiMichele and Chaney2013; Looy et al., Reference Looy, Stevensen, Van Hoof and Mander2014a; Looy and Hotton, Reference Looy and Hotton2014).
Paleobiogeographic context: basinal lowlands or uplands?
Although the vast majority of Pennsylvanian macrofloral assemblages are typified by wetland elements, taphocoenoses that are gymnosperm-dominated and/or contain derived taxa with mesic or xeric characters provide rare glimpses of tropical vegetation adapted to better-drained and/or seasonally dry conditions. Given the paucity of these exceptional occurrences and the physiological traits of the vegetation, such assemblages have sometimes been termed ‘upland’ or ‘extrabasinal’ floras based on the premise that the plants were transported to basinal lowlands from well-drained, elevated habitats (e.g., Chaloner, Reference Chaloner1958; Havlena, Reference Havlena1961, Reference Havlena1971; Cridland and Morris, Reference Cridland and Morris1963; Leary and Pfefferkorn, Reference Leary and Pfefferkorn1977; Pfefferkorn, Reference Pfefferkorn1980; Lyons and Darrah, Reference Lyons and Darrah1989; Broutin et al., Reference Broutin, Doubinger, Farjanel, Freytet, Kerp, Langiaux, Lebreton, Sebban and Satta1990; Dimitrova et al., Reference Dimitrova, Cleal and Thomas2011). However, the descriptors ‘seasonally dry’ or ‘dryland’ (used herein) are more appropriate because the floras also existed within depocenters rather than being confined to marginal settings, with prior adaptation to moisture limitation enabling the plants to disperse into basinal lowlands during episodes of climatic drying (e.g., Moore et al., Reference Moore, Elias and Newell1936; DiMichele and Aronson, Reference DiMichele and Aronson1992; Gastaldo, Reference Gastaldo1996; DiMichele et al., Reference DiMichele, Kerp, Tabor and Looy2008, Reference DiMichele, Cecil, Montañez and Falcon-Lang2010a; Falcon-Lang et al., Reference Falcon-Lang, Nelson, Looy, Ames, Elrick and DiMichele2009, Reference Falcon-Lang, Jud, Nelson, DiMichele, Chaney and Lucas2011b, Reference Falcon-Lang, Cleal, Pendleton and Wellman2012; Dolby et al., Reference Dolby, Falcon-Lang and Gibling2011; Van Hoof et al., Reference Van Hoof, Falcon-Lang, Hartkopf-Fröder and Kerp2013; Bashforth et al., Reference Bashforth, Cleal, Gibling, Falcon-Lang and Miller2014; DiMichele, Reference DiMichele2014; Ielpi et al., Reference Ielpi, Gibling, Bashforth, Lally, Rygel and Al-Silwadi2014; Looy and Hotton, Reference Looy and Hotton2014; Looy et al., Reference Looy, Stevensen, Van Hoof and Mander2014a, Reference Looy, Kerp, Duijnstee and DiMichele2014b).
The macrofloral assemblage documented herein is dominated by foliage of the gymnosperms Cordaites (produced by ‘dryland cordaitaleans’), Lesleya (a rare taxon of ambiguous affinity), and Taeniopteris (a derived taxon and possibly the oldest known examples in the fossil record). Hence, the taphocoenosis provides an exemplary record of the Euramerican dryland biome, albeit one that clearly was deposited in a basinal setting. Numerous factors indicate that the macrofloral assemblage was drawn from plant communities in the immediate vicinity of the lowland burial site, rather than having experienced long-distance transport from elevated areas outside the depositional basin:
-
(1) Most canopy litter in modern (para)tropical forests is drawn from trees growing within an ~0.1 Ha area (Burnham, Reference Burnham1993), and is prone to rapid decay when exposed on the forest floor (Ferguson, Reference Ferguson1985; Burnham, Reference Burnham1989; Gastaldo, Reference Gastaldo1994; Gastaldo and Staub, Reference Gastaldo and Staub1999). Accordingly, most fossilized macrofloral assemblages are parautochthonous and were rapidly buried, presenting an ‘ecological snapshot’ of the local vegetation (Wing and DiMichele, Reference Wing and DiMichele1995; DiMichele and Gastaldo, Reference DiMichele and Gastaldo2008).
-
(2) The macrofloral assemblage has a low diversity and a low evenness, being strongly dominated by elements typical of dryland (94%) rather than wetland (6%) environments (Table 1). Low diversity epitomizes (par)autochthonous assemblages in Pennsylvanian strata (Bashforth et al., Reference Bashforth, Falcon-Lang and Gibling2010, Reference Bashforth, Drábková, Opluštil, Gibling and Falcon-Lang2011, Reference Bashforth, Cleal, Gibling, Falcon-Lang and Miller2014), and if extensive transport had occurred, the taphocoenosis would have a higher diversity and evenness due to mixing of plant remains from various sources (Bashforth et al., Reference Bashforth, Falcon-Lang and Gibling2010).
-
(3) Most leaves in the taphocoenosis are entire (or nearly so), and show no evidence of fragmentation, abrasion, or subaqueous decay due to prolonged residency or bedload contact in a fluvial system (Ferguson, Reference Ferguson1985, Reference Ferguson2005; Spicer, Reference Spicer1989).
-
(4) Paleoenvironmental indicators of intensely seasonal conditions in the heterolithic unit are consistent with the dryland flora that it contains, including evidence for rapid shifts in flow dynamics, exposure and erosion of local substrates, and an ecosystem prone to wildfires.
-
(5) Paleogeographic reconstructions (and paleocoordinates) situate the study locality at least 250 km southwest of the Laurentian Shield during the Pennsylvanian (e.g., Algeo and Heckel, Reference Algeo and Heckel2008, fig. 2a). Although of low relief, this emergent cratonic surface would have been the closest upland source based on the orientiation of major paleovalleys and fluvial channels in the Illinois Basin succession, which indicate overall southwestward paleoflow (Archer and Greb, Reference Archer and Greb1995; Nelson et al., Reference Nelson, Greb and Weibel2013).
The amalgamation of these independent lines of argument confirms that the gymnosperm-dominated dryland assemblage documented herein occupied basinal lowland habitats of the Illinois Basin during a seasonally dry climatic interval, and were not transported from a distant upland or extrabasinal source. Nonetheless, resident populations of the dryland biome almost certainly did exist in elevated hinterlands (e.g., Laurentian Shield, Appalachian-Variscan Mountains) or at dry tropical paleolatitudes (e.g., western Pangea, at ~15–20° N), from which the vegetation dispersed when lowlands astride the equator experienced drier conditions (DiMichele et al., Reference DiMichele, Cecil, Montañez and Falcon-Lang2010a; DiMichele, Reference DiMichele2014).
Paleoecology: habitat preferences and physiological adaptations
The abundance of Cordaites leaves in the macrofloral assemblage is consistent with the prevalence of dryland cordaitaleans in mesic habitats of equatorial Euramerica during the Pennsylvanian and into the Permian. These slow-growing gymnosperms had dense, conifer-like wood, large, coriaceous leaves, and wind-dispersed seeds and pollen (Rothwell, Reference Rothwell1988; DiMichele and Phillips, Reference DiMichele and Phillips1994, Reference DiMichele and Phillips1996). Compared to low-stature forms on peat and clastic wetland substrates (Rothwell and Warner, Reference Rothwell and Warner1984; Šimůnek et al., Reference Šimůnek, Opluštil and Drábková2009; Raymond et al., Reference Raymond, Lambert, Costanza, Slone and Cutlip2010), cordaitaleans that inhabited well-drained substrates attained gigantic heights (Falcon-Lang and Bashforth, Reference Falcon-Lang and Bashforth2004, Reference Falcon-Lang and Bashforth2005), implying prolonged growth on stable landforms where habitat disturbance was limited (Bashforth et al., Reference Bashforth, Drábková, Opluštil, Gibling and Falcon-Lang2011, Reference Bashforth, Cleal, Gibling, Falcon-Lang and Miller2014). Furthermore, the dryland cordaitaleans had deep and laterally extensive root systems that enabled occupancy of moisture-stressed clastic soils, including coarse-grained (porous) substrates in riparian settings (Falcon-Lang Reference Falcon-Lang2003b, Reference Falcon-Lang2003c, Reference Falcon-Lang2006; Falcon-Lang and Bashforth, Reference Falcon-Lang and Bashforth2004, Reference Falcon-Lang and Bashforth2005; Falcon-Lang et al., Reference Falcon-Lang, Rygel, Gibling and Calder2004, Reference Falcon-Lang, Benton, Braddy and Davies2006, Reference Falcon-Lang, Jud, Nelson, DiMichele, Chaney and Lucas2011b; Bashforth et al., Reference Bashforth, Cleal, Gibling, Falcon-Lang and Miller2014; Ielpi et al., Reference Ielpi, Gibling, Bashforth, Lally, Rygel and Al-Silwadi2014). Based on these inferences, forests of gigantic cordaitaleans probably blanketed large areas of seasonally dry basinal lowlands, especially in habitats distant from frequent disturbance. The densely foliated and expansive crowns of the trees would have created a strongly shading canopy (Bashforth et al., Reference Bashforth, Cleal, Gibling, Falcon-Lang and Miller2014), and may have been the main fuel for wildfires that swept the landscape.
Little is known about the growth habit or physiology of plants that bore the large, simple, coriaceous leaves of Lesleya and Taeniopteris, although they almost invariably are found in strata that indicate moisture stress (DiMichele et al., Reference DiMichele, Kerp, Tabor and Looy2008, Reference DiMichele, Cecil, Montañez and Falcon-Lang2010a). Leary (Reference Leary1974a) speculated that Lesleya was the foliage of a large tree based on the diameter of associated branches, and physiological attributes of the leaf epidermis point to xerophylly (Remy and Remy, Reference Remy and Remy1975a). Furthermore, Lesleya typically is found in strata that filled channels incised into limestone bedrock, particularly near basin margins (White, Reference White1908; Leary, Reference Leary1974a, Reference Leary1974b, Reference Leary1981, Reference Leary1996; Leary and Pfefferkorn, Reference Leary and Pfefferkorn1977), suggesting that the plant may have been an edaphic specialist on thin, nutrient-poor, and alkaline soils (White, Reference White1931; DiMichele et al., Reference DiMichele, Cecil, Montañez and Falcon-Lang2010a). The habit and ecology of the plant that bore Taeniopteris leaves is even more cryptic. The leaf epidermis displays xerophytic characters (Barthel, Reference Barthel1962) and the plant was speculatively reconstructed as shrub-like with clustered leaves on thin axes (Cridland and Morris, Reference Cridland and Morris1963; Axsmith et al., Reference Axsmith, Serbet, Krings, Taylor, Taylor and Mamay2003), suggesting that it may have been an understory component of dryland forests. Taeniopteris leaves of the form recovered from the macrofloral assemblage are generally considered to be gymnosperms, perhaps representing an early cycad or close relative (Mamay, Reference Mamay1976; Gillespie and Pfefferkorn, Reference Gillespie and Pfefferkorn1986). The precocious occurrence of the fossil-genus at the study locality may be its earliest known record (a ‘Methuselah’ taxon, sensu Looy et al., Reference Looy, Kerp, Duijnstee and DiMichele2014b), and serves as yet another example of the connection between derived plant taxa and dryland settings, the presumed site of the majority of evolutionary innovations (DiMichele and Aronson, Reference DiMichele and Aronson1992; Kerp, Reference Kerp1996; DiMichele et al., Reference DiMichele, Mamay, Chaney, Hook and Nelson2001b, Reference DiMichele, Kerp, Tabor and Looy2008, Reference DiMichele, Cecil, Montañez and Falcon-Lang2010a).
Perhaps the most intriguing fossil in the macrofloral assemblage is the specimen assigned to Incertae sedis, a perplexing plant that has a planate form and consists of a thick rachis, from which stiff, strongly confluent, and parallel-veined pinnule-like structures arise. The affinities of this enigmatic plant are unknown, although it superficially resembles some late Paleozoic noeggarathialeans known from Euramerica, including Noeggerathia Sternberg, Reference Sternberg1821, Plagiozamites Zeiller, Reference Zeiller1894, Charliea Mamay, Reference Mamay1990, and Yuania Sze, Reference Sze1953. The specimen also shares features with Late Pennsylvanian and Permian cycad-like fossils traditionally assigned to Pterophyllum Brongniart, Reference Brongniart1824 (see Pott et al., Reference Pott, McLoughlin and Lindström2010), and with the early Mesozoic broad-leaved conifer Podozamites Braun, Reference Braun1843, putative examples of which have been documented from Upper Pennsylvanian and lower Permian rocks of the USA (Mamay, Reference Mamay1990; Mamay and Mapes, Reference Mamay and Mapes1992; DiMichele et al., Reference DiMichele, Mamay, Chaney, Hook and Nelson2001b). Although this peculiar fossil cannot be positively identified, it almost certainly represents another Methuselah taxon.
The fact that the macrofloral assemblage is dominated by gymnosperms with broad, coriaceous foliage (Cordaites, Lesleya, Taeniopteris), and that the plant assigned to Incertae sedis also has a robust construction and xeromorphic aspect, is consistent with a flora that withstood at least seasonal moisture stress. The large surface area of the leaves points to a subhumid rather than semiarid climate, with periodic but not extended intervals of drought. A dry subhumid climate, characterized by 3–5 ‘wet’ months (consecutive months when precipitation exceeds evaporation) and maximum seasonality, is compatible with the sedimentological and macrofloral evidence (Bashforth et al., Reference Bashforth, Cleal, Gibling, Falcon-Lang and Miller2014, table 1). These genera appear to comprise physiologically ‘slow’ plants, when viewed from the perspective of the modern, angiosperm-based ‘leaf-economics spectrum’ (Wright et al., Reference Wright, Reich, Westoby, Ackerly, Baruch, Bongers, Cavender-Bares, Chapin, Cornelissen, Diemer, Flexas, Garnier, Groom, Gulias, Hikosaka, Lamont, Lee, Lee, Lusk, Midgley, Navas, Niinemets, Oleksyn, Osada, Poorter, Poot, Prior, Prankov, Roumet, Thomas, Tjoelker, Veneklaas and Villar2004). In fact, the generally robust construction of most late Paleozoic equatorial vegetation may fit the slow-plant pattern, implying long life spans (Stull et al., Reference Stull, DiMichele, Falcon-Lang, Nelson and Elrick2012). However, compared to the physiological spectrum of wetland plants (Raymond et al., Reference Raymond, Slone and Wehner2013, Reference Raymond, Wehner and Costanza2014), traits diagnostic of xerophylly and slow growth appear to be exaggerated in vegetation of the dryland biome. This research domain is expanding as understanding of the physiological attributes of late Paleozoic vegetation intensifies (Boyce, Reference Boyce2008; Wilson et al., Reference Wilson, Knoll, Holbrook and Marshall2008; Boyce et al., Reference Boyce, Lee, Field, Brodribb and Zwieniecki2010; Green, Reference Green2010; Wilson and Knoll, Reference Wilson and Knoll2010), and patterns of insect damage (Beck and Labandeira, Reference Beck and Labandeira1998; Labandeira and Allen, Reference Labandeira and Allen2007; Schachat et al., Reference Schachat, Labandeira, Gordon, Chaney, Levi, Halthore and Alvarez2014) may serve as a proxy for the ‘fast vs. slow’ leaf-economics axis. Such studies hold considerable promise for enhancing our understanding of evolutionary and ecological frameworks in Paleozoic terrestrial ecosystems.
Materials and methods
Publications that provide detailed descriptive and illustrated records of Middle Pennsylvanian adpressions from North America were the main source used for comparison with the macrofloral assemblage (see Bashforth et al., Reference Bashforth, DiMichele, Eble and Nelson2016, table 3). The selected literature focuses on the Illinois Basin, and Indiana in particular, but includes records from the Western Interior and Appalachian basins of the USA, and the Maritimes Basin of Atlantic Canada.
The plant-fossil collection is stored at the National Museum of Natural History, Smithsonian Institution (Washington, DC), and illustrated specimens are reposited in the Plant Type and Illustrated Collection under USNM numbers 544585, 606684, 606686, 606688, 606691, 606693, 606697, 606700, 606702, 606704, 606705, 606707, 606715, and 606716.
Systematic paleontology
Division Tracheophyta
Class Sphenopsida
Order Equisetales Du Mortier, Reference Du Mortier1829
Family Calamitaceae Unger, Reference Unger1840
Genus Annularia Sternberg, Reference Sternberg1821
Annularia sphenophylloides (Zenker, Reference Zenker1833) Gutbier, Reference Gutbier1837
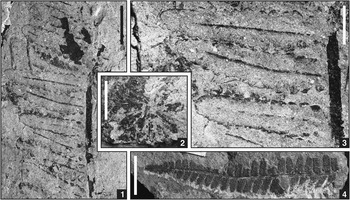
Figure 4 Photographs of sphenopsid and fern specimens in dryland assemblage from below Minshall Coal, Clay County, Indiana; scale bars=10 mm (1, 4) or 5 mm (2, 3). (1, 3) cf. Crenulopteris acadica (Bell, Reference Bell1962) Wittry et al., Reference Wittry, Glasspool, Béthoux, Koll and Cleal2014, marattialean fern foliage, ultimate pinna with wide rachis and linguaeform, pinnatifid pinnules with parallel lateral margins, obtusely rounded tops, slightly constricted bases, and non-decurrent midveins, USNM 606705. (2) Annularia sphenophylloides (Zenker, Reference Zenker1833) Gutbier, Reference Gutbier1837, calamitalean foliage, isolated, poorly preserved whorl, USNM 606716. (4) Pecopteris (Brongniart, Reference Brongniart1822) Sternberg, Reference Sternberg1825 (sp. indet.), marattialean fern foliage, ultimate pinna with small linguaeform pinnules and topped by lobate terminus, USNM 544585.
1833 Galium sphenophylloides Reference ZenkerZenker, p. 398, pl. 5, figs. 6–9.
1837 Annularia sphenophylloides (Zenker) Reference GutbierGutbier, p. 436.
1969 Annularia sphenophylloides; Reference CrookallCrookall, p. 733, text-fig. 215a [holotype].
See Bashforth et al. (Reference Bashforth, DiMichele, Eble and Nelson2016) for full synonymy list.
Holotype
Private collection of Dr. Schüler of Jena, Thüringen, Germany, from Carboniferous of Zittau, Sachsen, Germany (Crookall, Reference Crookall1969, p. 736).
Occurrence
USA: upper Atokan to upper Virgilian, most common above Atokan (Abbott, Reference Abbott1958; Darrah, Reference Darrah1969; Gillespie and Pfefferkorn, Reference Gillespie and Pfefferkorn1979; Blake et al., Reference Blake, Cross, Eble, Gillespie and Pfefferkorn2002). UK and Atlantic Canada: lower Bolsovian to lower Cantabrian (Bell, Reference Bell1938; Cleal and Thomas, Reference Cleal and Thomas1994; Cleal, Reference Cleal1997). Europe: extends to top of Stephanian (Wagner, Reference Wagner1984).
Material
One specimen.
Description
The poorly preserved whorl is symmetrical and comprises about 15 crowded or partly overlapping leaves of similar length. Leaves are 4–5 mm long and ~1 mm wide (L:W ratio ~4), spatulate, broadest very close to the distal margin, and superficially appear to have obtusely rounded tops because mucronate tips are embedded in the matrix. Midveins are about 0.3 mm wide, longitudinally striated, and flare at the distal margin (terminal expansion) before protruding as an acuminate mucro.
Remarks
Annularia sphenophylloides is a common and easily recognized fossil-species from Middle to Upper Pennsylvanian strata of Euramerica. Although Kerp (Reference Kerp1984) noted possible confusion with small examples of Annularia carinata Gutbier, Reference Gutbier1849, the latter is more typical of the Upper Pennsylvanian and lower Permian. Despite the poor preservation, the fragmentary whorl conforms to A. sphenophylloides in all respects (Abbott, Reference Abbott1958). The small spatulate leaves are especially characteristic, and embedding of the mucronate tip in the matrix is common for the taxon.
Class Filicopsida
Order Marattiales Link, Reference Link1833
Family Psaroniaceae Unger in Endlicher, Reference Unger1842
Genus Crenulopteris Wittry et al., Reference Wittry, Glasspool, Béthoux, Koll and Cleal2014
cf. Crenulopteris acadica (Bell, Reference Bell1962) Wittry et al., Reference Wittry, Glasspool, Béthoux, Koll and Cleal2014
1925 Pecopteris vestita Lesquereux; Reference NoéNoé, pl. 29, figs. 2, 3, pl. 30, fig. 2.
1925 Pecopteris miltoni (Artis) Brongniart; Reference NoéNoé, pl. 30, fig. 1, pl. 34, fig. 4.
1938 Asterotheca miltoni (Artis) Kidston; Reference BellBell, p. 71, pl. 67, figs. 2, 3(?), 4, pl. 68, pl. 69.
1962 Pecopteris (Asterotheca) acadica Reference BellBell; p. 32, pl. 25, pl. 56, fig. 7.
1969 Pecopteris lamuriana Heer; Reference DarrahDarrah, p. 138, pl. 20, fig. 1, pl. 24(?), pl. 47, fig. 1, pl. 62, figs. 1, 2.
1974 Asterotheca miltoni; Reference BonehamBoneham, p. 102, pl. 3, fig. 3.
1977 Pecopteris lamuriana; Reference GastaldoGastaldo, text-fig. 38.
1979 Asterotheca miltoni; Reference JanssenJanssen, p. 127, text-figs. 110(?), 111.
1980 Pecopteris (Asterotheca) acadica; Reference Zodrow and McCandlishZodrow and McCandlish, p. 59, pl. 72, fig. 2, pl. 77, fig. 2.
1982 Pecopteris lamuriana; Reference OleksyshynOleksyshyn, p. 80, text-fig. 16e–g.
2006 Lobatopteris lamuriana (Heer) Wagner; Reference WittryWittry, p. 11–14, figs. 2, 3(?), 7–10, 11, 13(?).
2014 Lobatopteris vestita (Lesquereux) Wagner; Reference Moore, Wittry and DiMicheleMoore et al., p. 34, pl. 4, figs. 1–3, 4(?), 5(?), 6(?), 7, 8, 9(?).
2014 Crenulopteris acadica (Bell) Reference Wittry, Glasspool, Béthoux, Koll and ClealWittry et al.; p. 12, text-figs. 2–6, 9, 13 [holotype: text-fig. 13a, c, d, f].
Holotype
GSC 847, National Type Collection of Invertebrate and Plant Fossils, Geological Survey of Canada, Ottawa, from Beersville Seam, Richibucto Formation (middle? Asturian), Central Basin, New Brunswick, Canada.
Occurrence
USA: lower Desmoinesian to lower Virgilian, common in upper Desmoinesian and Missourian (Darrah, Reference Darrah1969; Blake et al., Reference Blake, Cross, Eble, Gillespie and Pfefferkorn2002; Wittry et al., Reference Wittry, Glasspool, Béthoux, Koll and Cleal2014); if specimen at hand conspecific, lower range extends to uppermost Atokan. Europe: upper Asturian to Cantabrian (Wittry et al., Reference Wittry, Glasspool, Béthoux, Koll and Cleal2014). Atlantic Canada: middle(?) Asturian to lower Cantabrian (Bell, Reference Bell1938, Reference Bell1962; Zodrow and McCandlish, Reference Zodrow and McCandlish1980; Zodrow, Reference Zodrow1986; Wittry et al., Reference Wittry, Glasspool, Béthoux, Koll and Cleal2014); lower range may extend to uppermost Bolsovian (Bell, Reference Bell1938, pl. 69, fig. 3).
Material
One specimen.
Description
The ultimate pinna (Fig. 4.1) is at least 67 mm long (longer in life), ~45 mm wide (probably widest in medial part of pinna), and inserted on a thick (up to 5 mm wide), punctate, and faintly longitudinally striated rachis; the apex is not preserved. Pinnules (Fig. 4.2) are inserted slightly obliquely to the rachis (70–85°), linguaeform with parallel lateral margins and an obtusely rounded top, somewhat constricted at the base, pinnatifid with about eight lobes on either side, are closely spaced and may touch their neighbors. Measurable pinnules range from 18–22 mm long and 4.5–6.5 mm wide (L:W ratio ~3.5). Midveins appear non-decurrent and robust, and occupy a furrow that extends straight to near the pinnule apex. Details of the lateral veins are lacking.
Remarks
Pertinent morphological details of this frond fragment are obscured due to compaction. However, the outline of the pinnatifid pinnules indicates that the plant probably represents marattialean tree-fern foliage referable to the fossil-genus Crenulopteris, which Wittry et al. (Reference Wittry, Glasspool, Béthoux, Koll and Cleal2014) introduced as a segregate of Lobatopteris Wagner, Reference Wagner1958. These genera involve a group of species formerly included in Pecopteris sensu lato, and are characterized by a frond with predominantly pinnatifid pinnules and lateral veins with a distinct branching pattern (Wagner, Reference Wagner1958). Crenulopteris differs from Lobatopteris in having pinnatifid pinnules over most of the frond, fewer decurrent pinnules and midveins, more bulbous sporangia (Wittry et al., Reference Wittry, Glasspool, Béthoux, Koll and Cleal2014), and by producing monolete rather than trilete spores (Laveine, Reference Laveine1970).
The main attributes of the specimen include a thick rachis and pinnules that are relatively large, markedly elongate, pinnatifid with about eight lobes, and parallel-sided with a non-decurrent, constricted base and an obtusely rounded apex. According to Wittry et al. (Reference Wittry, Glasspool, Béthoux, Koll and Cleal2014), two fossil-taxa with comparable morphology are expected in upper Bolsovian or lower Asturian strata: Lobatopteris miltonii (Artis, Reference Artis1825) Wagner, Reference Wagner1958 and Crenulopteris micromiltonii (Bertrand ex Corsin, Reference Corsin1951) Wittry et al., Reference Wittry, Glasspool, Béthoux, Koll and Cleal2014. However, comparing equivalent frond segments, the specimen has pinnatifid pinnules, whereas L. miltonii has somewhat wider and more deeply divided pinnules that are essentially already ultimate pinnae (Dalinval, Reference Dalinval1960; Shute and Cleal, Reference Shute and Cleal1989; Pšenička et al., Reference Pšenička, Bek, Cleal, Wittry and Zodrow2009; Wittry et al., Reference Wittry, Glasspool, Béthoux, Koll and Cleal2014). In contrast, the dimensions of the ultimate pinna and pinnatifid pinnules of the specimen are too large to fit the circumscription of C. micromiltonii (Corsin, Reference Corsin1951; Laveine, Reference Laveine1989; Wittry et al., Reference Wittry, Glasspool, Béthoux, Koll and Cleal2014). Instead, the best comparison is with Crenulopteris acadica, a fossil-species that supplanted the taxonomic concept of what Wagner (Reference Wagner1958, Reference Wagner1971) considered to identify with Lobatopteris vestita (see Moore et al., Reference Moore, Wittry and DiMichele2014; Wittry et al., Reference Wittry, Glasspool, Béthoux, Koll and Cleal2014). In North America, C. acadica has previously been confused with several allied taxa, particularly Crenulopteris lamuriana (Heer, Reference Heer1865) Wittry et al., Reference Wittry, Glasspool, Béthoux, Koll and Cleal2014, L. miltonii, and L. vestita, the latter being a junior synonym of L. miltonii (Pšenička et al., Reference Pšenička, Bek, Cleal, Wittry and Zodrow2009). Wittry et al. (Reference Wittry, Glasspool, Béthoux, Koll and Cleal2014) claimed that C. acadica is restricted to upper Asturian to Cantabrian strata, younger than the age assumed for the study unit. However, auxiliary support for assignment to C. acadica is the fact that Punctatisporites minutus, one of its spores (Wittry et al., Reference Wittry, Glasspool, Béthoux, Koll and Cleal2014), is the dominant component of the microfloral assemblage (Table 2).
Genus Pecopteris (Brongniart, Reference Brongniart1822) Sternberg, Reference Sternberg1825
Pecopteris sp. indet.
Occurrence
Euramerica: fossil-genus common in Middle and Upper Pennsylvanian.
Material
Three specimens.
Description
Ultimate pinnae are linear, over 50 mm long and 11 mm wide, centered by a thin (up to 0.6 mm wide), depressed rachis, and are mostly parallel-sided, but taper to an acutely rounded and lobate terminus that is 4.5 mm long and 2 mm wide. Pinnules are 1.5–5 mm long and 1.5–2 mm wide (L:W ratio ~2), linguaeform, inserted normal to the rachis on one side and more obliquely on the other, and crowded but not overlapping. Midveins arise perpendicular to the rachis (becoming slightly decurrent near the apex), appear robust, and give rise to sparse lateral veins that branch once at the midvein and arch to reach the lateral margin at 45–55°.
Remarks
Specimens are assigned to Pecopteris, which primarily encompasses foliage of marattialean tree ferns. The myriad species are notoriously difficult to differentiate, and because the fragments are sterile and the lateral veins are poorly preserved, species-level determination has not been attempted. Comer (Reference Comer1992, pl. 7, fig. 1) identified the illustrated example as Pecopteris (Senftenbergia) plumosa (Artis, Reference Artis1825) Radforth, Reference Radforth1938, a probable misnomer.
Class Spermatopsida
Order Cordaitanthales Meyen, Reference Meyen1984
Family Cordaitanthaceae Meyen, Reference Meyen1984
Genus Cordaites Unger, Reference Unger1850
Cordaites spp. indet.
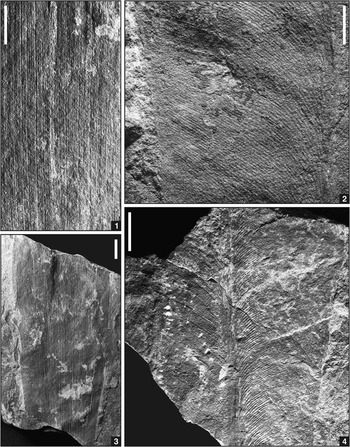
Figure 5 Photographs of gymnosperm specimens in dryland assemblage from below Minshall Coal, Clay County, Indiana; scale bars=10 mm (3, 4) or 5 mm (1, 2). (1, 3) Cordaites Unger, Reference Unger1850 (sp. indet.), cordaitalean foliage, showing details of leaf surface in 1, USNM 606715. (2) Lesleya Lesquereux, 1879-80 (sp. indet.), medial part of leaf exhibiting subparallel lateral margin and oblique, slightly S-shaped lateral veins typical of taxon (magnification of leaf in Fig. 6.1), USNM 606700. (4) Lesleya sp. indet., proximal part of leaf with subparallel lateral margins, pronounced midvein, and well-preserved venation, USNM 606693.
Occurrence
Euramerica: fossil-genus common throughout Carboniferous and Permian.
Material
Fifty specimens.
Description
Leaves are thick, coriaceous, linear-lanceolate, reaching at least 290 mm long (some probably an order of magnitude longer in life), and 20–110 mm wide (full width not always preserved). Lateral margins are entire, subparallel, and taper (Fig. 5.3) towards the base and apex (neither preserved), resulting in a strap-shaped leaf. Leaf surfaces comprise parallel longitudinal ridges and furrows (Fig. 5.1), representing the position of primary veins and intercostal parts of the lamina. Primary veins bifurcate rarely at a very low angle, and venation densities range from 7–42 veins per cm in central positions, becoming denser near lateral margins. The trace of intermediate sclerotic strands, exhibited as faint longitudinal lines between primary veins, may be present.
Remarks
Classifying adpressed Cordaites based on the size, shape, and venation pattern of leaves is recognized as wholly artificial (Šimůnek, Reference Šimůnek2006). Different leaf types can be found on a single branch (Césari and Hünicken, Reference Césari and Hünicken2013), and venation schemes are not diagnostic because the spacing of primary veins and the presence or absence (and number) of sclerotic strands between veins can differ depending on placement on a branch, maturity of the plant, the leaf surface exposed, and position on a single leaf (Harms and Leisman, Reference Harms and Leisman1961; Crookall, Reference Crookall1970; Barthel, Reference Barthel1976; Bashforth, Reference Bashforth2005). Although adpressed Cordaites shows conservative leaf morphology, cuticular studies highlight considerable diversity in the group (Zodrow et al., Reference Zodrow, Šimůnek and Bashforth2000; Šimůnek, Reference Šimůnek2006). Because no cuticles were procured, all leaves are assigned to Cordaites spp. indet. Cordaites leaves are difficult to differentiate from axes with densely spaced longitudinal striations, particularly when leaf apices or bases are not preserved. Hence, only specimens that could confidently be assigned to Cordaites were described and measured.
Order Phasmatocycadales Doweld, Reference Doweld2001
Family Phasmatocycadaceae Doweld, Reference Doweld2001
Genus Lesleya Lesquereux, 1879-80
Lesleya sp. indet.
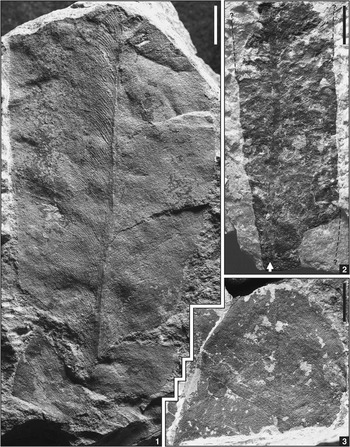
Figure 6 Photographs of Lesleya Lesquereux, 1879-80 (sp. indet.) specimens in dryland assemblage from below Minshall Coal, Clay County, Indiana; scale bars=10 mm. (1) Large proximal to medial leaf fragment showing pronounced midvein, subparallel lateral margins, and oblique, slightly Z-shaped lateral veins typical of taxon (venation magnified in Fig. 5.2). Note that lateral veins run less obliquely on right side than on left side, USNM 606700; (2) basal part of leaf, showing pronounced asymmetry of lamina on either side of midvein (arrow). Photograph digitally manipulated by overlaying matrix with translucent layer to enhance leaf outline, with dashed lines indicating presumed position of lateral margins, USNM 606686; (3) obtusely rounded leaf apex, with midvein extending close to top (see Fig. 7), USNM 606684.
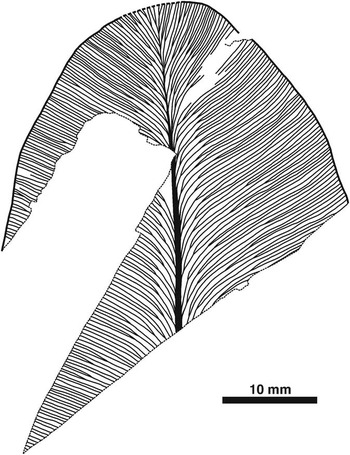
Figure 7 Line drawing of obtusely rounded apex of Lesleya Lesquereux, 1879-80 (sp. indet.) leaf (USNM 606704), showing venation typical of taxon in dryland assemblage from below Minshall Coal, Clay County, Indiana. Thin, densely spaced lateral veins arise at acute angle, and arch slightly before forking once or twice near midvein, resulting in two- or four-pronged vein sets that run Z-shaped course to reach lateral margin at oblique angle; additional bifurcations occur at irregular intervals. Note that veins on right side curve upwards near margin, and that midvein terminates just before apex.
Occurrence
Euramerica: rare and sparsely distributed Upper Mississippian to upper Permian fossil-genus (Šimůnek, Reference Šimůnek1996).
Material
Thirty-five specimens.
Description
Leaves are moderately thick, appear coriaceous, may be slightly vaulted, reach at least 140 mm long (although most probably much longer in life), and range from 20–82 mm wide (mean: 48 mm). No complete leaves are present, but lateral margins are entire and subparallel for most of their length (Figs. 5.2, 5.4, 6.1), taper slowly towards the base (which may be asymmetrical, the lamina on one side merging with the midvein before the other; Fig. 6.2), and converge rapidly to an obtusely rounded apex (Figs. 6.3, 7). Hence, the typical leaf outline varies among linear-spathulate, oblanceolate, and (more rarely) strap-shaped. Midveins are rigid, longitudinally striated, 0.5–4 mm wide (tapering distally), and extend to (or almost to) the leaf apex (Figs. 6.3, 7). Midveins were (semi)circular in cross-section and subtended the leaf, occupying a pronounced furrow on the adaxial surface and are flattened on the abaxial surface. Lateral veins (Figs. 5.2, 5.4, 6. 1, 6.3, 7) arise at an acute angle (~25–30°), arch slightly, and branch once or twice in close succession near the midvein, resulting in a set of two or four veins that extend in tandem to the lateral margin; additional bifurcations occur at irregular intervals. The thin and densely spaced veins typically follow a Z-shaped course, running at 55–70° for most of their length, and generally curve upwards before the lateral margin. In some instances, however, the veins run at ~90° after arching from the midvein (i.e., like Taeniopteris), and the course can differ drastically on either side of the midvein (e.g., nearly straight and ~90° on one side, and more oblique and Z-shaped on the other; Fig. 6.1). The venation density typically is 28–34 veins per cm (range: 25–46, mean: 31) on the lateral margin and may differ on either side of the leaf.
Remarks
Lesleya is a poorly delineated fossil-genus of ambiguous systematic affinity. Based on a combination of epidermal and morphological features, Florin (Reference Florin1934) regarded the taxon as a primitive gymnosperm, whereas others considered it a pteridosperm (Němejc, Reference Němejc1968), a relative of Glossopteris Brongniart, Reference Brongniart1828a (Leary, Reference Leary1993, Reference Leary1998), or ancestral to the cycads (Remy and Remy, Reference Remy and Remy1978; Leary, Reference Leary1990). The systematics of Doweld (Reference Doweld2001) and Anderson et al. (Reference Anderson, Anderson and Cleal2007) are followed, although endorsement is tentative.
The main characters of Lesleya include large, simple leaves with entire, subparallel lateral margins, a thick midvein, and lateral veins that tend to be dichotomous and follow a straight or curved yet oblique course to the lateral margin (Lesquereux, 1879-80, p. 142; Leary, Reference Leary1980). Megalopteris Dawson, Reference Dawson1871 ex Andrews, Reference Andrews1875 shares many morphological attributes with Lesleya, but the former comprises a compound leaf with three or more lobes or pinnules (Dawson, Reference Dawson1871; Andrews, Reference Andrews1875). These genera are thus difficult to differentiate when only distal laminar fragments are preserved (Leary, Reference Leary1980), although the taxa can be separated based on epidermal details (Florin, Reference Florin1934; Šimůnek, Reference Šimůnek1996). In addition, Lesleya and Megalopteris can easily be confused with Taeniopteris (Bell, Reference Bell1940; Remy and Remy, Reference Remy and Remy1978; Leary, Reference Leary1990), which also is present in the assemblage. Problems associated with the definition and separation of Lesleya and Taeniopteris are discussed below.
Many of the large, simple leaf fragments in the assemblage can be accommodated within the broad diagnosis of Lesleya, although specific assignment is premature because homotaxial comparisons have proven inconclusive. In defining the genus, Lesquereux (1879-80, p. 143, pl. 25, figs. 1–3) noted that leaves of the type species (Lesleya grandis) reach 220 mm long and 80 mm wide (based on a nearly complete specimen); these dimensions approximate those assumed for the largest examples described here. However, the lateral margins of L. grandis leaves are markedly curved, whereas those of the current specimens are subparallel for much of their length. Furthermore, Lesquereux (1879-80) described the apex of L. grandis as obtusely pointed, and although one his specimens (pl. 25, fig. 3) is obtusely rounded, it is not as rounded as the apices described here (Figs. 6.3, 7). The venation density of L. grandis (25–30 veins per cm) is comparable with the present material (generally 28–34 veins per cm), but the lateral veins appear not to arch as strongly and run more obliquely than in the latter. Šimůnek (Reference Šimůnek1996) also described and illustrated a leaf fragment of Lesleya sp. (type Tř 1) from the Czech Republic that he compared with L. grandis.
A similar fossil-species is Lesleya cheimarosa, which has leaves up to 200 mm long and also are parallel-sided for much of their length (Leary and Pfefferkorn, Reference Leary and Pfefferkorn1977). However, leaves of L. cheimarosa apparently are only up to 40 mm wide (narrower than the material at hand), and have an acute rather than obtusely rounded apex (compare Leary and Pfefferkorn,Reference Leary and Pfefferkorn1977, pl. 8.1 and Figs. 6.3, 7). Despite these differences, the taxa share many morphological attributes, including: (1) venation scheme (branching of lateral veins once or twice near the midvein, resulting in two- or four-pronged vein sets that run a Z-shaped course to the lateral margin; compare Leary and Pfefferkorn, Reference Leary and Pfefferkorn1977, fig. 8c and Fig. 7); (2) venation density (24–36 veins per cm for L. cheimarosa versus mainly 28–34 veins per cm for the present leaves); and (3) an asymmetrical leaf base, as found in the assemblage (compare Leary and Pfefferkorn,Reference Leary and Pfefferkorn1977, pl. 8.1 and Fig. 6.2), although L. cheimarosa also may have a symmetrical base with a long petiole (Leary, Reference Leary1990, Reference Leary1996, pl. 5, fig. 2). Comer (Reference Comer1992, pl. 3, fig. 1) assigned at least one specimen from the current assemblage to L. cheimarosa.
This analysis indicates that specimens in the assemblage cannot be confidently assigned to either Lesleya grandis or Lesleya cheimarosa (the only comparable species known), although the taxa have many features in common. Furthermore, the leaves may belong to a new species, and thus have been kept in open nomenclature. A preliminary assessment indicates that cuticles may be preserved, and future comparison with the epidermal details of other Lesleya leaves (e.g., Florin, Reference Florin1934; Remy and Remy, Reference Remy and Remy1975a; Šimůnek, Reference Šimůnek1996) may clarify species assignment.
Genus Taeniopteris Brongniart, Reference Brongniart1828a
Taeniopteris sp. cf. T. multinervia Weiss, Reference Weiss1869
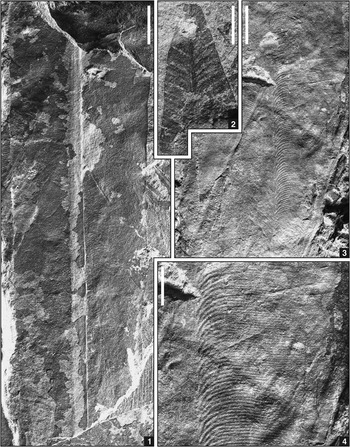
Figure 8 Photographs of Taeniopteris sp. cf. T. multinervia Weiss, Reference Weiss1869 specimens in dryland assemblage from below Minshall Coal, Clay County, Indiana; scale bars=10 mm (1, 3) or 5 mm (2, 4). (1) Abaxial leaf surface that exhibits parallel lateral margins, lateral veins that run perpendicular to margins for most of their length, and pronounced, rigid midvein that is flattened and obscures most proximal part of lateral veins, USNM 606691; (2) acutely rounded (?) leaf apex presumed to belong to taxon, with midvein that extends close to tip and lateral veins that gently arch away from midvein to reach lateral margin at oblique angle, USNM 606702; (3, 4) medial part of leaf that demonstrates venation of taxon (magnified in 4). Thin and densely spaced lateral veins arise at acute angle, and arch strongly before forking once or twice near midvein, resulting in two- or four-pronged vein sets that run in tandem to lateral margin; additional bifurcations occur at irregular intervals, USNM 606688.
1869 Taeniopteris multinervia Reference WeissWeiss, p. 98, pl. 6, fig. 13.
1927a Taeniopteris multinervis; Reference HalleHalle, pl. 37, fig. 1 [holotype].
1977 Taeniopteris multinervia; Reference Remy and RemyRemy and Remy, p. 175, text-figs. 66a, 66b [holotype].
Holotype
S042025, Paleobotany (Goldenberg) Collection, Naturhistoriksa riksmuseet, Stockholm, from ‘Thoneisenstein’ (Humberg lacustrine horizon, Odernheim Subformation, upper Meisenheim Formation, Glan Subgroup; Schindler and Heidtke, Reference Schindler and Heidtke2007) (upper Lower Rotliegend; Roscher and Schneider, Reference Roscher and Schneider2006), Saar-Nahe Basin, Berschweiler, Saarland State, Germany.
Occurrence
USA: Taeniopteris locally abundant in Upper Pennsylvanian and lower Permian (Lesquereux, 1879-80; Fontaine and White, Reference Fontaine and White1880; Sellards, Reference Sellards1901; White, Reference White1929; Moore et al., Reference Moore, Elias and Newell1936; Cridland and Morris, Reference Cridland and Morris1963; Winston, Reference Winston1983; McComas, Reference McComas1988; Wagner and Lyons, Reference Wagner and Lyons1997; Beck and Labandeira, Reference Beck and Labandeira1998; DiMichele et al., Reference DiMichele, Chaney, Dixon, Nelson and Hook2000, Reference DiMichele, Chaney, Kerp and Lucas2010b, Reference DiMichele, Wagner, Bashforth and Álvarez-Vázquez2013a, Reference DiMichele, Chaney, Lucas, Kerp and Voigt2013b; Chaney et al., Reference Chaney, Sues and DiMichele2005; Chaney and DiMichele, Reference Chaney and DiMichele2007; Schachat et al., Reference Schachat, Labandeira, Gordon, Chaney, Levi, Halthore and Alvarez2014); unsubstantiated records of Taeniopteris multinervia in southwestern USA (Darrah, Reference Darrah1935). Europe and China: Taeniopteris multinervia rare and sparsely distributed in uppermost Pennsylvanian and lower Permian (Seward, Reference Seward1910; Halle, Reference Halle1927a, Reference Halle1927b; Kerp and Fichter, Reference Kerp and Fichter1985; Opluštil et al., Reference Opluštil, Šimůnek, Zajíc and Mencl2013).
Material
Sixteen specimens.
Description
Leaves appear coriaceous and stiff with non-vaulted laminae, reach up to 120 mm long (although most probably longer in life), and are 16–54 mm wide (mean: 34 mm). Leaves are incomplete but probably were linear in outline. Lateral margins are entire and parallel for most of their length (Fig. 8.1), taper very slowly towards the base, and converge to an acutely rounded tip (Fig. 8.2). Midveins are rigid, longitudinally striated, 1.0–6.5 mm wide (probably commencing as a basal petiole and tapering to the leaf apex; Fig. 8.2), and occupy a pronounced trough (flat-bottomed) on the adaxial surface but are flattened and can obscure the proximal part of lateral veins on the abaxial surface (Figs. 8.1, 10.2). Lateral veins (Figs. 8.1, 8.3, 8.4, 10.2) are thin, arise at an acute angle (~20–30°), arch strongly, and branch once or twice in close succession near the midvein, resulting in a set of two or four veins that run in tandem to the lateral margin at 80–90°; additional bifurcations occur irregularly. Near the apex, lateral veins gently arch away from the midvein and reach the lateral margin at ~70° (Fig. 8.2). The venation density typically is 34–40 veins per cm (range: 28–45, mean: 37) on the lateral margin and is highest near the leaf tip.
Remarks
Taeniopteris is a broadly defined and long-ranging (Carboniferous to Cretaceous) fossil-genus that involves plants of numerous systematic affinities, having been attributed to late Paleozoic ferns and pteridosperms, late Paleozoic and Mesozoic cycads, Mesozoic bennettitaleans, and other gymnosperms (Voigt and Rößler, Reference Voigt and Rößler2004; Taylor et al., Reference Taylor, Taylor and Krings2009; Pott and Launis, Reference Pott and Launis2015). Taeniopteris has played an influential role in the discussion of cycad antiquity, with the arrangement of seeds and character of megasporophylls associated with Late Pennsylvanian and Permian foliage pointing to a late Paleozoic origin (Mamay, Reference Mamay1976; Gillespie and Pfefferkorn, Reference Gillespie and Pfefferkorn1986), a hypothesis queried by Axsmith et al. (Reference Axsmith, Serbet, Krings, Taylor, Taylor and Mamay2003).
As diagnosed by Brongniart (Reference Brongniart1828a, p. 61), the primary characters of Taeniopteris include simple, linear leaves with parallel lateral margins, a thick midvein, and lateral veins that are unbranched or bifurcate near the midvein and run perpendicular to the lateral margin. Applying this plastic definition, as exemplified by Remy and Remy (Reference Remy and Remy1975b, table 2), some leaves in the assemblage can be assigned to the genus, making this one of the earliest occurrences in the fossil record.
Based on the work of Remy and Remy (Reference Remy and Remy1975b, table 2), the best comparison for the material at hand is with Taeniopteris multinervia, which Weiss (Reference Weiss1869) diagnosed as having lateral veins that arise at an acute angle, promptly arch to reach a right angle, and dichotomize twice close to the midvein before extending perpendicular to the lateral margin. Due to this branching pattern, lateral veins appear more loosely spaced near the midvein, a key characteristic shared with the study material. Photographs of the holotype of T. multinervia (Halle, Reference Halle1927a; Remy and Remy, Reference Remy and Remy1977) indicate that the venation density is 28–40 veins per cm, which is in line with specimens in the assemblage (full range of 28–45 veins per cm). Zeiller (Reference Zeiller1890, pl. 12, figs. 2–5, pl. 13, figs. 1, 1a, 1b) illustrated the venation scheme, although some lateral veins are more oblique than is typical. Halle (Reference Halle1927a, pl. 37) also illustrated comparable specimens, including leaves with acutely rounded tips like the single example in the assemblage. Nonetheless, Halle (Reference Halle1927a) and Remy and Remy (Reference Remy and Remy1977) considered the presence of circular ‘glands’ between the lateral veins as a diagnostic feature of T. multinervia. Such glands have not been observed in the present examples, although Halle (Reference Halle1927a) noted that they are not always preserved.
Fontaine and White (Reference Fontaine and White1880, p. 91, pl. 34, figs. 9, 9a) erected Taeniopteris lescuriana based on one(?) leaf fragment that also has a venation scheme similar to the study examples. Fontaine and White (Reference Fontaine and White1880) suggested that T. lescuriana was similar to Taeniopteris multinervia (an opinion shared by Zeiller, Reference Zeiller1890; Seward, Reference Seward1910; and Bell, Reference Bell1940), but differed in having larger leaves (up to 100 mm wide), a more slender midvein, and fewer lateral veins. Such features are difficult to judge from their illustrations, but McComas (Reference McComas1988, fig. 4.7) recorded a specimen that fits the protologue of T. lescuriana (listed as such in table 1, but illustrated as Taeniopteris sp.), having a thin midvein and less dense venation (measured as 19–27 veins per cm). Likewise, Beck and Labandeira (Reference Beck and Labandeira1998, pl. 4, figs. 1–9) illustrated relatively wide leaves of Taeniopteris sp. with lateral veins that are relatively loosely spaced (~25 veins per cm), arise from the midvein at an acute angle, and abruptly arch to run perpendicular to the lateral margin, comparing well with the circumscription of T. lescuriana. Thus, the characters that distinguish T. lescuriana from T. multinervia are the same as those that separate T. lescuriana from leaves in the collection, lending indirect support for assignment to T. multinervia.
Another similar fossil-species is Taeniopteris abnormis Gutbier, Reference Gutbier1835, which Zeiller (Reference Zeiller1894), Barthel (Reference Barthel1976, Reference Barthel2006), and Wagner and Álvarez-Vázquez (Reference Wagner and Álvarez-Vázquez2010) considered an earlier synonym of Taeniopteris multinervia. The diagnosis of T. abnormis (Gutbier, Reference Gutbier1835, p. 73, 74) lacks sufficient information (and the relevant plate was not published), but the description and illustrations of Sterzel (Reference Sterzel1876, p. 376, pl. 5, figs. 2–5, pl. 6) indicate that T. abnormis differs from examples in the assemblage in having: (1) larger leaves (up to 95 mm wide) that are obtusely rounded at the top; (2) thicker midveins (up to 7.5 mm wide); (3) some simple or once-forked lateral veins that may arise directly from the midvein at a right angle; and (4) a venation density around 20–25 veins per cm. The claim that some lateral veins are simple and arise normal to the midvein may be a consequence of preservation, as the venation adjacent to the midvein may be overlain and obscured on the abaxial surface due to flattening of the thick midvein. However, specimens of T. abnormis illustrated by Halle (Reference Halle1927b, pl.3, fig. 3) and Doubinger et al. (Reference Doubinger, Vetter, Langiaux, Galtier and Broutin1995, text-fig. 336), who considered the taxon distinct from T. multinervia, and another by Barthel (Reference Barthel2006, text-fig. 153), who did not, clearly show simple or once-forked veins arising perpendicular to the midvein. Similar characters are evident on leaves documented by DiMichele et al. (Reference DiMichele, Chaney, Kerp and Lucas2010b, text-fig. 23) and DiMichele et al. (Reference DiMichele, Wagner, Bashforth and Álvarez-Vázquez2013a, text-fig. 28), who compared their specimens with T. abnormis. As noted by Barthel (Reference Barthel1976) and Remy and Remy (Reference Remy and Remy1978), the lower part of T. abnormis leaves (corresponding to Taeniopteris schenkii Sterzel, Reference Sterzel1876) has lateral veins that arise at an acute angle, branch twice, and run on an oblique course (i.e., Lesleya-like) (see Sterzel, Reference Sterzel1876, pl. 5, fig. 6; Barthel, Reference Barthel1976, pl. 38, fig. 4). In contrast, the basal sections of leaves from the study assemblage exhibit perpendicular rather than oblique venation.
Taeniopteris smithii, erected based on one leaf fragment (Lesquereux, 1879-80, p. 153, 154, pl. 25, figs. 7, 7a), shares many features with Taeniopteris abnormis, including mostly simple lateral veins that arise approximately perpendicular to the midvein. However, the midvein was described as ‘canaliculate’ (having a central depressed channel) and the venation density as about 30–40 veins per cm. Halle (Reference Halle1927a) considered T. smithii to be a later synonym of Taeniopteris fallax Göppert, 1864-Reference Göppert65 (see DiMichele et al., Reference DiMichele, Wagner, Bashforth and Álvarez-Vázquez2013a), whereas Darrah (Reference Darrah1935) suggested that T. smithii belongs to the fossil-genus Megalopteris, ostensibly because it was found in Lower Pennsylvanian strata (Helena coal zone, Cahaba Coalfield, Alabama; Pashin and Gastaldo, Reference Pashin and Gastaldo2009, fig. 2.4), probably Langsettian in age (Eble et al., Reference Eble, Gillespie and Henry1991). If retention of T. smithii in Taeniopteris could be confirmed, this would be the oldest known record of the fossil-genus, predating a Bolsovian occurrence in northern Spain (Wagner in van Loon, Reference Van Loon1971; Wagner and Álvarez-Vázquez, Reference Wagner and Álvarez-Vázquez2010).
Specimens from the Upper Pennsylvanian of Kansas (Winston, Reference Winston1983, pl. 2, fig. 3, under Taeniopteris coriacea Göppert, 1864-Reference Göppert65; Cridland and Morris, Reference Cridland and Morris1960, text-fig. 1, under Taeniopteris sp.) and from the lower Permian of Texas (DiMichele et al., Reference DiMichele, Chaney, Dixon, Nelson and Hook2000, fig. 4b–f, under Taeniopteris sp.) resemble the narrowest leaves in the assemblage. Lateral veins arise from the midvein at an acute angle before abruptly turning to run perpendicular to the margin. In particular, the example of Winston (Reference Winston1983) has a comparable venation density (measured at 33–36 veins per cm), but the number and nature of forks near the midvein is unclear. Likewise, the leaves documented by DiMichele et al. (Reference DiMichele, Chaney, Dixon, Nelson and Hook2000) have venation densities of 35–40 veins per cm, but a maximum width of only 30 mm. In contrast, Cridland and Morris (Reference Cridland and Morris1960) stated that their specimen has lateral veins that are simple or dichotomize once immediately after arising from the midvein, and the venation density (measured at ~26 veins per cm) is lower than encountered herein. All of these specimens are narrower than is typical of Taeniopteris multinervia or the material at hand, and best fit the diagnosis of T. coriacea (Göppert, 1864-Reference Göppert65, p. 130). Other American examples attributed to T. coriacea (e.g., Sellards, Reference Sellards1901, Reference Sellards1908; White, Reference White1929; Cridland and Morris, Reference Cridland and Morris1960; Gillespie and Pfefferkorn, Reference Gillespie and Pfefferkorn1986), all of which are conspicuously narrow, also are distinct from leaves in the assemblage.
Based on this review of comparable taeniopterids, leaves in the dryland assemblage most resemble Taeniopteris multinervia. However, because T. multinervia has to date only been confirmed in uppermost Pennsylvanian to lower Permian strata of Europe and China (Darrah, Reference Darrah1935, did not provide citations to substantiate putative records from southwestern USA), assignment is with reservation. If identity with T. multinervia could be proven, the examples documented here would be the earliest known and the only confirmed records from North America. Preliminary assessment indicates that cuticles may be preserved, and future study of epidermal features could clarify identity.
Separation of Lesleya sp. indet. from Taeniopteris sp. cf. T. multinervia
The challenge of identifying and separating the fossil-genus Lesleya from non-pinnate forms of the fossil-genus Taeniopteris has previously been discussed (Remy and Remy, Reference Remy and Remy1978; Leary, Reference Leary1990). Comparing the diagnoses of Taeniopteris (Brongniart, Reference Brongniart1828a, p. 61) and Lesleya (Lesquereux, 1880, p. 142) indicates that both involve large simple leaves with a thick midvein that extends to (near) the apex. However, two fundamental differences stand out in the protologues: (1) the lateral margins of Taeniopteris are parallel, whereas those of Lesleya are curved to subparallel; and (2) the lateral veins of Taeniopteris are either simple or bifurcating and extend essentially perpendicularly between the midvein and lateral margin, whereas those of Lesleya are repeatedly dichotomous and run in a curved and oblique course to the lateral margin.
A literature review demonstrates that the morphology of many fossil-species combines features ascribed to both fossil-genera, and that generic assignment seemed to hinge on author bias. For example, Zeiller (Reference Zeiller1890, pl. 13, figs. 1, 1a, 1b) illustrated a specimen of Taeniopteris multinervia that has curved to subparallel lateral margins (like Lesleya) and lateral veins that arch away from the midvein before extending straight to reach the lateral margin at ~70° (a mix of Taeniopteris and Lesleya venation). In contrast, the T. multinervia leaf of Zeiller (Reference Zeiller1890, pl. 12, fig. 2) has straight lateral margins (Taeniopteris) and both perpendicular (Taeniopteris) and oblique, Z-shaped (Lesleya) lateral veins, a confounding blend of features discussed by Zeiller (Reference Zeiller1894). Examples assigned by Renault (1896, text-figs. 1, 2) to T. multinervia also display the oblique, Z-shaped venation scheme typical of Lesleya, as does one side of the Taeniopteris abnormis leaf in Sterzel (Reference Sterzel1876, pl. 6, fig. 1). Furthermore, the lower parts of T. abnormis leaves (Taeniopteris schenkii form) apparently have curved, oblique lateral veins that conform to Lesleya (Sterzel, Reference Sterzel1876, pl. 5, fig. 6; Barthel, Reference Barthel1976, pl. 38, fig. 4), whereas the upper parts of leaves have the perpendicular venation typical of Taeniopteris (Barthel, Reference Barthel1976; Remy and Remy, Reference Remy and Remy1978). The leaves of Lesleya eckardtii (Germar in Kurtze, Reference Germar1839) Remy and Remy, Reference Remy and Remy1977, reconstructed by Remy and Remy (Reference Remy and Remy1977, text-fig. 69), have mostly parallel lateral margins and simple, straight lateral veins, features typical of Taeniopteris, to which the species was originally ascribed. Finally, Schachat et al. (Reference Schachat, Labandeira, Gordon, Chaney, Levi, Halthore and Alvarez2014) documented leaves that have straight lateral margins (thus attributed to Taeniopteris spp.), but slightly sinusoidal, upward-concave lateral veins that ascend at ~45°.
The ambiguity surrounding differentiation of Taeniopteris and Lesleya is relevant because leaves that conform to both genera occur in the dryland assemblage, often on the same slab. Not unexpectedly, specimens initially were assumed to belong to one plant, with the subtle morphological differences recording intraspecific variation in a taxon characterized by large, simple leaves with a thick midvein and densely spaced lateral veins. Particularly perplexing is the similar venation scheme, as both leaf types have lateral veins that arise at an acute angle and branch once or twice close to the midvein, resulting in two- or four-pronged vein sets that extend in tandem to the lateral margin (e.g., compare Figs. 7 and 8.4). Equally confusing, some specimens (assigned to Lesleya sp. indet.) have lateral veins that, after arching from the midvein, extend perpendicular to the lateral margin on one side of the leaf (like those assigned to Taeniopteris sp. cf. T. multinervia), but run the usual oblique and Z-shaped course on the other (top of specimen in Fig. 6.1).
Despite the morphological similarities, critical observations indicate that leaves of two different taxa are preserved in the assemblage: (1) no specimens exhibit a transition in lateral venation from perpendicular to Z-shaped (or vice versa) along the length of a single leaf (as known for Taeniopteris abnormis, discussed above), which would be expected if the venation changed from base to apex; (2) all leaves assigned to Taeniopteris sp. cf. T. multinervia have parallel lateral margins regardless of preserved length, whereas most leaves assigned to Lesleya sp. indet. have slightly curved lateral margins that exhibit a degree of convergence; and (3) leaves of the Lesleya type have obtusely rounded apices (Figs. 6.3, 7), whereas the single example referred to Taeniopteris converges to an acute apex (Fig. 8.2). Separation of the two taxa is corroborated by running Mann-Whitney U tests (non-parametric, univariate test for equality of medians) on midvein width, angle of lateral veins at the midvein, midpoint and lateral margin, and venation density (Fig. 9; Table 3). With the exception of the angle at which lateral veins arise from the midvein, the test indicates that there are statistically significant differences in the median values of the attributes. Thus, based on both empirical and statistical criteria, there is evidence that two taxa with large non-compound leaves co-occur in the assemblage. Although morphologically similar, one plant conforms to the diagnosis of Taeniopteris (Brongniart, Reference Brongniart1828a), and the other to Lesleya (Lesquereux, 1880).
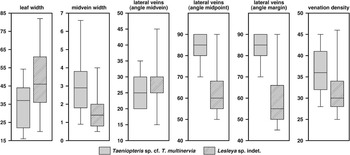
Figure 9 Box and whisker plots showing range, 25th and 75th percentiles, and median of morphological variables measured on specimens in dryland assemblage identified as Lesleya Lesquereux, 1879-80 (sp. indet.) and Taeniopteris sp. cf. T. multinervia Weiss, Reference Weiss1869. Mann-Whitney U tests indicate statistically significant differences in median values of all attributes except angle at which lateral veins arise from midvein (see Table 3), permitting segregation of two superficially similar taxa.
Table 3 Morphometric data from specimens in dryland assemblage identified as Lesleya Lesquereux, 1879-80 (sp. indet.) and Taeniopteris sp. cf. T. multinervia Weiss, Reference Weiss1869 (data presented graphically in Figure. 9). Mann-Whitney U tests indicate statistically significant differences in median values of all attributes except angle at which lateral veins arise from midvein.

Incertae sedis
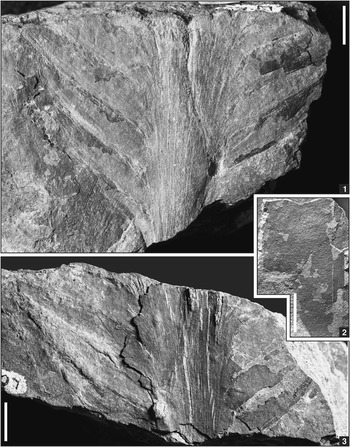
Figure 10 Photographs of specimens in dryland assemblage from heterolithic unit below Minshall Coal, Clay County, Indiana; scale bars=10 mm. (1, 3) Incertae sedis, part and counterpart of specimen of unknown affinity, with wide ‘rachis’ that exhibits coarse longitudinal striations and ‘cracks,’ and distichous, decurrent, and opposite ‘pinnules’ that dichotomize directly from (rather than clasp) rachis. Pinnule bases have acroscopic incision but merge with rachis on basiscopic side, and faint veins (upper left in 1) are widely spaced and parallel, USNM 606707. (2) Taeniopteris sp. cf. T. multinervia Weiss, Reference Weiss1869, abaxial leaf surface demonstrating pronounced, rigid midvein that is flattened and obscures most proximal part of perpendicular lateral veins, USNM 606697.
Material
One specimen.
Description
The entire plant appears robust, stiff, and possibly succulent. The axis (hereafter ‘rachis’) has a preserved length of 60 mm, is ~18 mm wide, and exhibits coarse longitudinal striations and random ‘cracks’ that are lenticular and longitudinally elongate. Foliar structures (hereafter ‘pinnules’) are distichous, opposite to subopposite, contiguous or partly overlapping (due to slight imbrication, with part of the upper pinnule margin lying beneath the succeeding pinnule), and decurrent, arising directly from the rachis at an acute angle before arching and extending straight at ~40–50°. Pinnules do not clasp the rachis, but are confluent with it; the acroscopic margin is somewhat incised at the base, and the basiscopic margin merges into the rachis. Pinnules are 7–8 mm wide and may exceed 41 mm in length (the only ‘complete’ pinnule is 38 mm long, incomplete pinnules being longer), and become longer and better developed higher up the rachis. Acroscopic lateral margins are parallel for most of their length, whereas basiscopic margins are slightly convex, producing an asymmetrical pinnule that gradually narrows to an acutely(?) rounded, entire(?) apex. The presumed upper pinnule surface is smooth, with faint, densely spaced epidermal striations (~60–70 per cm). Veins, only expressed on the presumed lower pinnule surface, arise directly from the rachis, appear to branch more than once and, because the acroscopic margin of a pinnule is incised at its base, splay out slightly before running roughly parallel to the lateral margins. Venation density is 7–15 veins per cm. The mesophyll of the pinnules apparently decayed prior to burial, leaving a ‘hollow’ interior, manifest as a thin veneer of sediment enclosed between the upper and lower pinnule surfaces.
Remarks
The affinity of this peculiar plant is unknown, hence assignment to Incertae sedis. Comer (Reference Comer1992, pl. 15) also was perplexed and termed the specimen ‘Problematica,’ noting its resemblance to a modern cycad. The most conspicuous attribute is the fact that foliar structures dichotomize directly from the lateral margins of the axis, with little basal constriction or evidence of having clasped the axis. When alive, the entire organ must have been photosynthetic, the foliar elements representing appendicular extensions of a large pinnate leaf. As such, the plant superficially resembles a fern or palm frond fragment, rather than a branch on which leaves are borne, and thus the terms ‘rachis’ and ‘pinnule’ are used here in place of ‘stem’ and ‘leaf.’
The fossil initially was considered to be a leaf-bearing cordaitalean branch (e.g., Göppert, Reference Göppert1852, pl. 15; Grand’Eury, Reference Grand’Eury1877, pls. 19–25; Lesquereux, 1879, pl. 78, pl. 80, fig. 1). However, the rachis does not display the helically arranged, laterally elongated leaf bases typical of a cordaitalean branch (Cordaicladus Grand’Eury, Reference Grand’Eury1877), and although the pinnules have a longitudinal venation like Cordaites (albeit weakly developed), they are distichous, opposite, and dichotomize directly from the lateral margins of the axis, rather than clasp it.
The specimen also bears a superficial resemblance to the fossil-genus Aphlebia Presl in Sternberg, Reference Presl1838, which comprises myriad morphological variants and has been interpreted to represent protective leaf-like structures that surrounded juvenile fern fronds. The fragment shares some similarities with material that Zeiller (Reference Zeiller1888) described and illustrated under Aphlebia grossouvrei (p. 296–298, pl. 33, fig. 4) and Aphlebia rhizomorpha (p. 298–300, pl. 33, figs. 5, 6), both characterized by decurrent foliar structures that are broadly attached to an axis with coarse longitudinal striations. However, A. grossouvrei and A. rhizomorpha have an irregular appearance with segments that are lobed to varying degrees, whereas the specimen at hand has a more regimented organization.
The plant also shares features with the fossil-genus Taeniophyllum Lesquereux, Reference Lesquereux1878, which includes five fossil-species erected by Lesquereux (Reference Lesquereux1878, Reference Lesquereux1879-84) and White (Reference White1899). Salient characters include wide stems, from which arise a profusion of long, spirally arranged, decurrent, linear, and smooth leaves. Leaves narrow towards the base, with the point of attachment expressed as a tiny linear or acuminate scar (e.g., Lesquereux, 1879, pl. 81, fig. 1). A striking attribute of the leaves is that upper and lower leaf surfaces can envelop a thin wafer of sediment, leading Lesquereux (Reference Lesquereux1878, 1880) and White (Reference White1899) to infer that the leaves were inflated and somewhat fistular in life, with decay of the delicate or lacuneous inner leaf producing a cavity that filled during burial. Taeniophyllum initially was considered a gymnosperm (Lesquereux, Reference Lesquereux1878), although after the discovery of clusters of trilete megaspores within or along the leaves, Lesquereux (1880, 1884) speculated that the plant was a lycopsid, comparing it with Isoëtes Linnæus, Reference Linnæus1753. White (Reference White1899) was inclined towards an affinity with the (tree) ferns due to the association of Taeniophyllum leaves with axes bearing petiole scars (Caulopteris Lindley and Hutton, Reference Lindley and Hutton1833). The systematic position of Taeniophyllum remains unresolved.
Taeniophyllum and the study specimen share many attributes, particularly the wide axes and decurrent, linear, and smooth foliar structures that show evidence of sediment infilling during fossilization. However, Taeniophyllum consists of a true stem bearing helically arranged leaves, as demonstrated by the presence of leaf scars on the axis, whereas the specimen described here has a single row of appendicular pinnules on either side of the rachis. Based on these fundamental differences in architecture, the fossil is confidently excluded from Taeniophyllum.
The specimen recalls some members of an enigmatic group of plants that many authors include in the noeggarathialeans (progymnosperms?), which are characterized by elongate foliar segments with mostly parallel, dichotomous veins. Comparable Pennsylvanian and/or Permian fossil-genera include Noeggerathia, Plagiozamites, Charliea, Yuania (= Russellites Mamay, Reference Mamay1968), Tingia Halle, Reference Halle1925, and Paratingia Zhang, Reference Zhang1987. An overview of the morphological traits of these genera facilitates comparisons with the study specimen (Table 4). Debate persists whether the sterile components of these plants represent shoots bearing leaves, or are pinnate structures analogous to a rachis bearing pinnae or pinnules; to simplify comparisons, the terms ‘axis’ and ‘foliar elements’ are used below.
Table 4 Main morphological traits, affinities, and age ranges of fossil-genera with elongate foliar segments and mostly parallel, dichotomous veins, facilitating comparison with enigmatic specimen from dryland assemblage identified as Incertae sedis (illustrated in Fig. 10.1, 10.3). Characters in bold text shared with study specimen. Symbols: (a) earlier synonym of Russellites Mamay, Reference Mamay1968 (Wang and Chaney, Reference Wang and Chaney2010); (b) only known from Cathaysia; (c) not true Pterophyllum Brongniart, Reference Brongniart1824 (=Bennettitales), and best assigned to Dioonites Miquel, Reference Miquel1851 if epidermal features uncertain (cf. Pott et al., Reference Pott, McLoughlin and Lindström2010). See text for details.
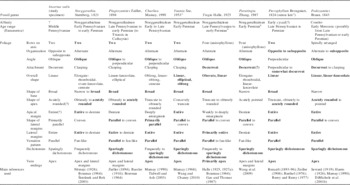
All of the examined noeggerathialean genera share some morphological commonalities with the foliar elements of the specimen (Table 4), including: (1) arrangement in a single row on either side of the axis (the exceptions are two anisophyllous rows in Tingia and Paratingia); (2) oblique attachment; (3) generally elongate overall shape; (4) broad bases (Paratingia and some Noeggerathia have narrow bases); (5) primarily parallel to slightly convex lateral margins (Noeggerathia only has convex margins); (6) entire lateral margins (Paratingia and some Noeggerathia and Plagiozamites have dentate margins); (7) parallel venation patterns (Noeggerathia and Paratingia, as well as some Plagiozamites and Tingia, exhibit a fan-like venation); and (8) sparingly dichotomized lateral veins (Noeggerathia and some Charliea and Tingia have more frequent branching). In contrast, the following morphological attributes of the noeggerathialean foliar elements differ from the study specimen (Table 4): (1) all arise alternately from the axis rather than having an opposite to subopposite arrangement (although this may reflect derivation of the study specimen from near the ‘frond’ base); (2) most have clasping bases (only Tingia and possibly Paratingia have decurrent bases); (3) apex shapes are variable (only some Noeggerathia and Plagiozamites have acutely rounded apices); (4) apical margins are variable (only Yuania has exclusively entire apices); and (5) veins terminate along the lateral margins and apex (only in Charliea and Yuania do veins exclusively end at apices). Whereas this synthesis is admittedly convoluted, the exercise yields a crucial conclusion: although the specimen displays characters known for several late Paleozoic noeggerathialeans, no single genus shares the full suite of morphological attributes exhibited by the fossil, thereby precluding assignment to any of them.
Table 4 also summarizes the morphological traits of the fossil-genus Pterophyllum, which superficially resembles the study specimen. It is emphasized that Pterophyllum is being used in a broad sense, to include fossils of cycad-like foliage from Upper Pennsylvanian and Permian strata that have traditionally been assigned to the genus (see Pott et al., Reference Pott, McLoughlin and Lindström2010, table 1); Pterophyllum sensu stricto only refers to Late Triassic to Jurassic bennettitalean foliage with specific macromorphological and epidermal characters (Pott et al., Reference Pott, van Konijnenburg-van Cittert, Kerp and Krings2007, Reference Pott, McLoughlin and Lindström2010; Pott and McLoughlin, Reference Pott and McLoughlin2009). Like the specimen at hand, Pterophyllum sensu lato has distichous foliar elements that arise (sub)oppositely, are broadly attached to the axis, are linear with parallel and entire lateral margins, and have sparingly dichotomous parallel veins that terminate at an entire apex. In fact, the only obvious differences are that foliar segments of Pterophyllum arise and extend perpendicular to the axis (as opposed to arising decurrently and extending obliquely), and that axes in Pterophyllum are far narrower. Of the late Paleozoic species of Pterophyllum from Euramerica listed by Pott et al. (Reference Pott, McLoughlin and Lindström2010, table 1), the study specimen is most similar to illustrations of Pterophyllum cottaeanum Gutbier, Reference Gutbier1835 (Barthel, Reference Barthel1976, pl. 38, fig. 1) and Pterophyllum blechnoides Sandberger, Reference Sandberger1864, (Remy and Remy, Reference Remy and Remy1977, text-figs. 70a, b), both with lateral veins that arise decurrently from the central axis. However, P. cottaeanum has a much denser venation, and both have relatively thin axes, making identity with P. blechnoides equally unlikely.
A final comparison is with the fossil-genus Podozamites, a broad-leaved conifer best known from the early Mesozoic and characterized by thin shoots that bear spirally inserted, simple, linear to linear-lanceolate leaves with parallel entire lateral margins and parallel venation (Table 4; Seward, Reference Seward1919; Harris, Reference Harris1926). That Podozamites consists of a leafy shoot precludes identity with the specimen, but the superficial similarities are striking. Although once considered a Mesozoic genus, specimens from the Late Pennsylvanian of Texas and New Mexico were compared with Podozamites (Mamay, Reference Mamay1990, fig. 10, 11, under Genus A and Genus B, respectively; Mamay and Mapes, Reference Mamay and Mapes1992, fig. 9f), as were examples with thick axes from the early Permian of Texas (DiMichele et al., Reference DiMichele, Mamay, Chaney, Hook and Nelson2001b, fig. 3.3, under Podozamites sp.). The decurrent attachment, oblique angle, and parallel venation of the linear leaves illustrated by these authors clearly resemble the enigmatic study specimen, but identity cannot be confirmed.
Acknowledgements
A Postdoctoral Fellowship from the Natural Sciences and Engineering Research Council of Canada to ARB is gratefully acknowledged. Appreciation is given to the following for correspondence about systematics, repositories, type localities, and stratigraphic information: B.M. Blake, C.J. Cleal, R.A. Gastaldo, O. Johansson, S.C. Johnson, H. Kerp, S. Mcloughlin, R.F. Miller, J.C. Pashin, and J. Schneider. Dan Chaney is thanked for his advice with illustrations. The constructive reviews of H. Kerp and B.R. Pratt (Editor) improved the manuscript.