1. Introduction
The ~1000 km long Pontide mountain belt is located between the Anatolian plateau and the Black Sea (Fig. 1) and formed part of the Eurasian continental margin of the Tethys ocean during Late Palaeozoic–Early Palaeogene time (Gregor & Zijderveld, Reference Gregor and Zijderveld1964; Haas, Reference Haas1968; Şengör & Yılmaz, Reference Şengör and Yilmaz1981; Adamia et al. Reference Adamia, Chkhotua, Kekelia, Lordkipanidze, Shavishvili and Zakariadze1981; Robertson & Dixon, Reference Robertson, Dixon, Dixon and Robertson1984; Ricou et al. Reference Ricou, Dercourt, Geyssant, Grandjacquet, Lepvrier and Biju-Duval1986; Sarıbudak, Sanver & Ponat, Reference Saribudak, Sanver and Ponat1989; Evans & Hall, Reference Evans and Hall1990; Dercourt et al. Reference Dercourt, Zonenshain, Ricou, Kazmin, LePichon, Knipper, Grandjacquet, Sbortshikov, Geyssant, Leprevier, Pechersky, Boulin, Sibuet, Savostin, Sorokhtin, Westphal, Bazhenov, Lauer and Biju-Duval1986, Reference Dercourt, Gaetani, Vrielynck, Barrier, Biju-Duval, Brunet, Cadet, Crasquin and Sandulescu2000). The Upper Cretaceous–Lower Palaeogene İzmir–Ankara–Erzincan Suture Zone is located along the southern edge of the Pontides (Ketin, Reference Ketin1966) and marks the boundary between the Pontides and the Gondwana-derived Tauride–Anatolide Platform to the south (Fig. 1; Bergougnan, Reference Bergougnan1975; Şengör & Yılmaz, Reference Şengör and Yilmaz1981). The suture zone records the closure of a northerly branch of the Mesozoic Tethys ocean (here termed Northern Neotethys; Şengör & Yılmaz, Reference Şengör and Yilmaz1981; Robertson & Dixon, Reference Robertson, Dixon, Dixon and Robertson1984; Dercourt et al. Reference Dercourt, Zonenshain, Ricou, Kazmin, LePichon, Knipper, Grandjacquet, Sbortshikov, Geyssant, Leprevier, Pechersky, Boulin, Sibuet, Savostin, Sorokhtin, Westphal, Bazhenov, Lauer and Biju-Duval1986, Reference Dercourt, Gaetani, Vrielynck, Barrier, Biju-Duval, Brunet, Cadet, Crasquin and Sandulescu2000; Okay, Tansel & Tüysüz, Reference Okay, Tansel and Tüysüz2001). A range of tectonic models exist for the suture zone concerning the polarity of subduction, ophiolite genesis and emplacement, and the timing of subduction, obduction and collision (Bergougnan, Reference Bergougnan1975; Şengör & Yılmaz, Reference Şengör and Yilmaz1981; Yılmaz, Reference Yilmaz1985; Okay & Şahintürk, Reference Okay, Şahintürk and Robinson1997a; Yılmaz et al. Reference Yilmaz, Tüysüz, Yiğitbaş, Genç, Şengör and Robinson1997; Ustaömer & Robertson, Reference Ustaömer, Robertson and Robinson1997; Yalınız, Floyd & Göncüoğlu, Reference Yaliniz, Floyd, Göncüoğlu, Bozkurt, Winchester and Piper2000; Sarifakioğlu, Özen & Winchester, Reference Sarifakioğlu, Özen and Winchester2009). Testing these alternatives is critical in order to constrain the Late Cretaceous–Early Palaeogene development of the Arabia–Eurasia collision zone. The Eastern Pontides is one of the few well-exposed areas of the > 1000 km long İzmir–Ankara–Erzincan Suture Zone in Turkey that includes all the units of an active continental margin related to the closure of the Northern Neotethys.

Figure 1. Map showing location of the study area in relation to the main tectonic elements referred to in the text. EAFZ – East Anatolian Fault Zone; IAESZ – Izmir–Ankara–Erzincan Suture Zone; NAFZ – North Anatolian Fault Zone; NEAF – Northeast Anatolian Fault.
In this paper we present a revised stratigraphy of the Upper Cretaceous–Early Eocene units of the suture zone in the Erzincan area (Figs 1–3; Table 1), supported by new palaeontological dating. Successive modifications to the stratigraphy of the area have resulted in a wide range of stratigraphic names, subdivisions, ages and tectonostratigraphic relationships, with little supporting palaeontological or geochronological data. The field data and revised stratigraphy presented here provide a sound basis for the interpretation of active margin processes including supra-subduction zone ophiolite formation, subduction/accretion and arc magmatism related to closure of Tethys.

Figure 2. Revised tectonostratigraphy of the Eastern Pontides. The units are arranged in a generalized way from structurally highest on the left to structurally lowest on the right (after Rice, Robertson & Ustaömer, Reference Rice, Robertson, Ustaömer, Robertson and Mountrakis2006).
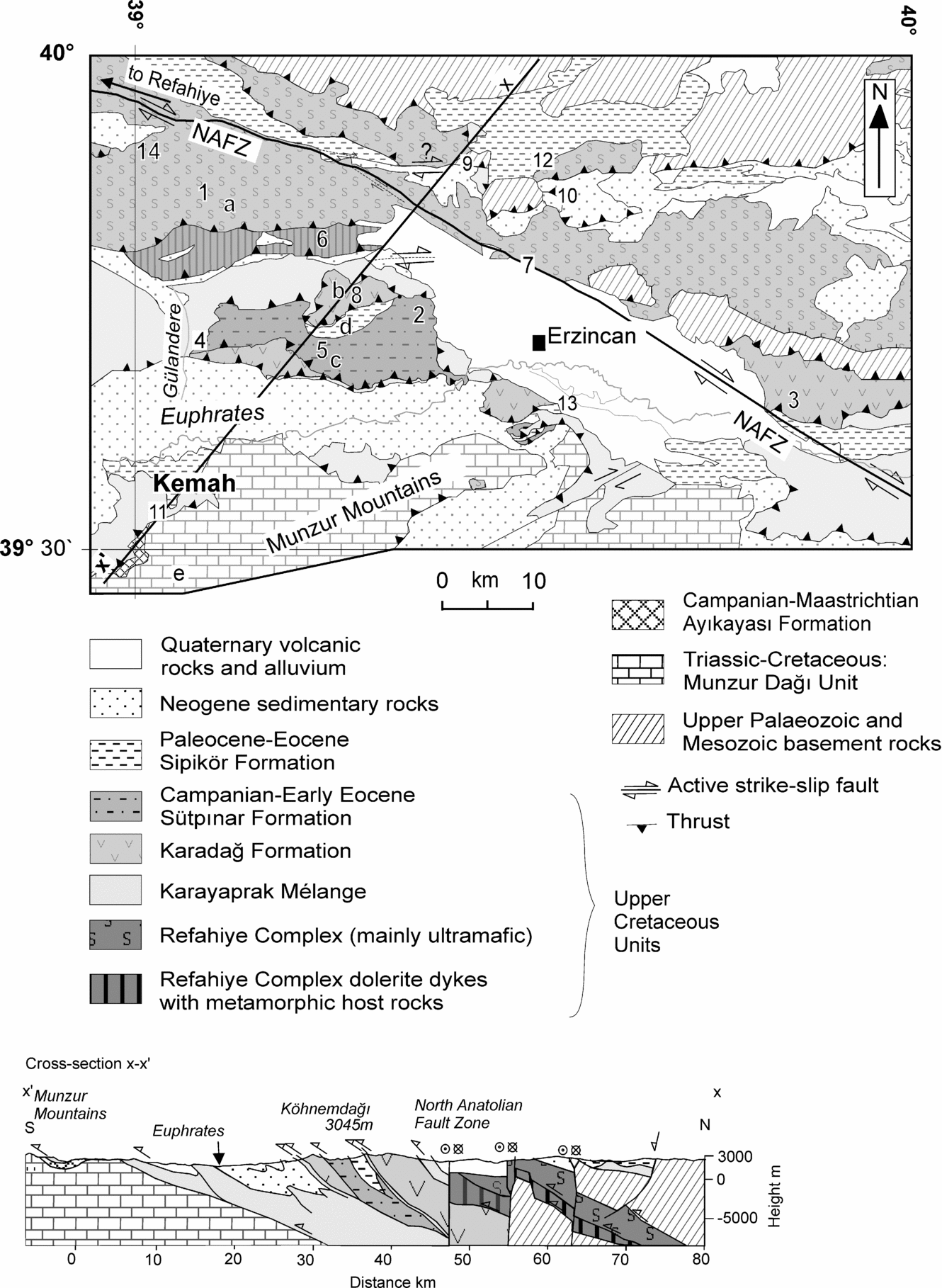
Figure 3. Simplified geological map of the study area (Erzincan) in the Eastern Pontides showing the outcrop of Upper Cretaceous–Lower Cenozoic units of the Izmir–Ankara–Erzincan Suture Zone; based on the Geological Map of Turkey (MTA, Reference İşiker2002). Numbers indicate locations described in the text; 1 – Akbudak; 2 – Ballı; 3 – Çardaklı; 4 – Doğanbeyli; 5 – Gökkaya; 6 – Göyne; 7 – Işıkpınar; 8 – Köhnemdağı; 9 – Kömür; 10 – Mecidiye; 11 – Muratboynu; 12 – Sipikör; 13 – Yaylabaşı; 14 – Yeniköy and Berişerif. Line of section x–x’ is shown. Letters a–e indicate locations of generalized logs (see Figure 4). Note: outcrop of the Ayıkayası Formation is shown schematically at this scale (after Rice, Robertson & Ustaömer, Reference Rice, Robertson, Ustaömer, Robertson and Mountrakis2006).
Table 1. Summary of the tectono-stratigraphic units identified within the İzmir–Ankara–Erzincan Suture Zone during this study

2. Regional setting
We will focus on units of Late Cretaceous–Early Eocene age that are exposed in the Erzincan area (Figs 1–3). We begin by summarizing the Late Palaeozoic–Early Palaeogene geology of the wider Eastern Pontide region to provide a context for the interpretation of the rocks in our study area.
The suture zone in the Ezincan area is well exposed within a ~40 km wide, dominantly south-vergent thrust belt that extends across northern Anatolia and the Lesser Caucasus. The rocks were initially described by Ketin & Erentöz (Reference Ketin and Erentöz1961) as mainly Mesozoic ophiolitic mélange. Later workers (Bergougnan, Reference Bergougnan1975; Yılmaz, Reference Yilmaz1985; Koçyiğit, Reference Koçyığızt1990; Aktimur et al. Reference Aktımur, Yurdakul, Sariaslan, Göksel, Keçer, Yildirim, Akkuş and Erler1995) identified discrete units within the suture zone, for example, the Sütpınar Formation, the Karadağ Complex, plus ‘Inner Tauride Mélange’ (Koçyiğit, Reference Koçyığızt1990), the Çerpaçindere Formation, the Karadağ Bazalt, the Gülandere Formation and the Senek Ophiolitic Complex (Aktimur et al. Reference Aktımur, Yurdakul, Sariaslan, Göksel, Keçer, Yildirim, Akkuş and Erler1995). In the northern part of the suture zone, in the Erzincan area, the Upper Cretaceous units are thrust-imbricated with earlier Mesozoic sedimentary rocks (the Kelkit and Hözbirikyayla formations: Okay & Şahintürk, Reference Okay and Şahintürk1997b). In the south, the suture zone structurally overlies the Tauride–Anatolide Platform, preserved in the Munzur Mountains within the study area (Fig. 1; Bergougnan, Reference Bergougnan1975; Yılmaz, Reference Yilmaz1985; Koçyiğit, Reference Koçyığızt1990).
The Late Cretaceous–Early Palaeogene tectonic evolution of the Eastern Pontides and the adjacent Lesser Caucasus involved the development of an active margin, including accretionary complex, forearc, volcanic arc and back-arc units (Adamia et al. Reference Adamia, Chkhotua, Kekelia, Lordkipanidze, Shavishvili and Zakariadze1981; Manetti et al. Reference Manetti, Peccerillo, Poli and Corsini1983; Philip et al. Reference Philip, Cisternas, Gvishiani and Gorshkov1989; Koçyiğit, Reference Koçyığızt1990; Ustaömer & Robertson, Reference Ustaömer, Robertson and Robinson1997; Okay & Şahintürk, Reference Okay, Şahintürk and Robinson1997a; Yılmaz et al. Reference Yilmaz, Adamia, Chabukiani, Chkhotua, Erdoğan, Tuzcu, Karabiyikoğlu, Bozkurt, Winchester and Piper2000; Yılmaz, Şen & Özgür, Reference Yilmaz, Şen and Özgür2003; Sosson et al. Reference Sosson, Rolland, Corsini, Danelian, Stephan, Avagyan, Melkonian, Jrbashyan, Melikian and Galoian2005; Rice, Robertson & Ustaömer, Reference Rice, Robertson, Ustaömer, Robertson and Mountrakis2006; Galoyan et al. Reference Galoyan, Rolland, Sosson, Corsini and Melkonyan2007; Ustaömer & Robertson, Reference Ustaömer and Robertson2009). However, there are contrasting interpretations of these units, their ages, and the timing and nature of major tectonic events, for example, the initiation of subduction, timing and causes of extension, volcanism, back-arc basin formation, and the onset of collision and suturing.
2.a. North Tethyan margin
Upper Palaeozoic Hercynian basement units in the Pontides, north of the İzmir–Ankara–Erzincan Suture Zone, are mainly composed of granitic rocks, schist and gneiss, with marble intercalations in some units (Tanyolu, Reference Tanyolu1988; Okay & Şayintürk, Reference Okay, Şahintürk and Robinson1997a; Topuz et al. Reference Topuz, Altherr, Satur and Schwartz2004). The oldest unmetamorphosed sedimentary unit in the area is a coherent Upper Carboniferous clastic succession (‘molasse’), which crops out ~50 km north of Erzincan in the Pulur region (Okay & Leven, Reference Okay and Leven1996). The Upper Palaeozoic units are regionally overlain by a mélange known as the Karakaya Complex that is widely interpreted as a Triassic accretionary complex related to northward subduction of Palaeotethys (Pickett & Robertson, Reference Pickett and Robertson1996, Reference Pickett and Robertson2004; Okay, Reference Okay, Bozkurt, Winchester and Piper2000), although other interpretations exist (see Okay & Göncüoğlu, Reference Okay and Göncüoğlu2004). All of the metamorphosed and deformed units are unconformably overlain by a Lower–Middle Jurassic succession that includes abundant volcanogenic rocks (e.g. Kelkit Formation; Akın, Reference Akin1978; Rojay, Reference Rojay1995; Robinson et al. Reference Robinson, Spadini, Cloetingh and Rudat1995; Yılmaz et al. Reference Yilmaz, Tüysüz, Yiğitbaş, Genç, Şengör and Robinson1997; Okay & Şahintürk, Reference Okay and Şahintürk1997b). This is overlain, with a localized unconformity, by shallow-marine carbonates up to ~2000 m thick that are interpreted as mainly carbonate platform and carbonate ramp facies (Koçyiğit & Altıner, Reference Koçyığıt and Altiner2002; Yılmaz & Kandemir, Reference Yilmaz and Kandemir2006). These rocks, in turn, pass stratigraphically upwards into a pelagic radiolarian biomicrite and calciturbidite succession of Late Jurassic–Early Cretaceous age that is well exposed in the south (e.g. Berdiga Formation: Koçyiğit & Altıner, Reference Koçyığıt and Altiner2002; Yılmaz & Kandemir, Reference Yilmaz and Kandemir2006; Dokuz & Tanyolu, Reference Dokuz and Tanyolu2006). Together, the Upper Jurassic–Lower Cretaceous units are interpreted as parts of a south-facing passive continental margin succession (Yılmaz, Reference Yilmaz1985; Okay & Şahintürk, Reference Okay, Şahintürk and Robinson1997a,Reference Okay and Şahintürkb; Okay et al. Reference Okay, Tüysüz, Satır, Özkan-Altıner, Altıner, Sherlock and Eren2006). However, the Jurassic succession in the Eastern Pontides locally includes calc-alkaline volcanic rocks (Yılmaz et al. Reference Yilmaz, Adamia, Chabukiani, Chkhotua, Erdoğan, Tuzcu, Karabiyikoğlu, Bozkurt, Winchester and Piper2000), and various authors envisage an active margin setting related to either southward subduction (Koçyiğit & Altıner, Reference Koçyığıt and Altiner2002), or northward subduction (Nikishin et al. Reference Nikishin, Korotaev, Ershov and Brunet2003; Şen, Reference Şen2007; Ustaömer & Robertson, Reference Ustaömer and Robertson2009). The continental margin sequence is bounded at the top by an angular unconformity of Late Cretaceous age which marks the beginning of compressional deformation that was associated with the emplacement of oceanic units (Okay & Şahintürk, Reference Okay, Şahintürk and Robinson1997a,Reference Okay and Şahintürkb).
In the Eastern Pontides, to the north of the suture zone, there is an Upper Cretaceous arc-related volcanic and volcaniclastic succession > 2 km thick, composed of andesitic, dacitic and rhyolitic rocks, associated with calk-alkaline granitic plutonism (Şengör & Yılmaz, Reference Şengör and Yilmaz1981; Robinson et al. Reference Robinson, Spadini, Cloetingh and Rudat1995; Okay & Şahintürk, Reference Okay, Şahintürk and Robinson1997a). The oldest post-Jurassic volcanic rocks of the Pontides are believed to be Turonian in age (93.5–89.3 Ma: Taner & Zaninetti, Reference Taner and Zaninetti1978). Granitic plutons from the Eastern Pontides have K/Ar radiometric ages of ~95–65 Ma (late Cenomanian–Maastrichtian: Taner, Reference Taner1977; Gedikoğlu, Reference Gedikoğlu1979; Moore, McKee & Akıncı, Reference Moore, McKee, Akincı, Jankovic and Sillitoe1980). Associated volcaniclastic rocks are interbedded with fossiliferous limestones of Coniacian–Santonian age (Yılmaz, Şen & Özgür, Reference Yilmaz, Şen and Özgür2003; Boztuğ et al. Reference Boztuğ, Joncheere, Wagner and Yeğıngıl2004, Reference Boztuğ, Erçın, Kuruçelık, Göç, Kömür and İskenderoğlu2006). The Eastern Pontide magmatic rocks are generally interpreted as an Upper Cretaceous continental margin arc formed above northward-subducting oceanic lithosphere (e.g. Şengör & Yılmaz, Reference Şengör and Yilmaz1981; Akınçi, Reference Akinçı, Dixon and Robertson1984; Yılmaz et al. Reference Yilmaz, Tüysüz, Yiğitbaş, Genç, Şengör and Robinson1997; Okay & Şahintürk, Reference Okay, Şahintürk and Robinson1997a,Reference Okay and Şahintürkb). However, the timing of onset of northward subduction is controversial. For example, Adamia et al. (Reference Adamia, Chkhotua, Kekelia, Lordkipanidze, Shavishvili and Zakariadze1981), Hess et al. (Reference Hess, Aretz, Gurbanov, Emmermann and Lippolt1995) and Nikishin et al. (Reference Nikishin, Korotaev, Ershov and Brunet2003) suggested that subduction took place continuously beneath Eurasia at least since Jurassic time, whereas Yılmaz et al. (Reference Yilmaz, Tüysüz, Yiğitbaş, Genç, Şengör and Robinson1997) and Okay & Şahintürk (Reference Okay, Şahintürk and Robinson1997a) proposed a Cenomanian–Turonian time for the onset of northward subduction. Recently, Okay et al. (Reference Okay, Tüysüz, Satır, Özkan-Altıner, Altıner, Sherlock and Eren2006) reported Albian crystallization ages for eclogites and blueschists in the Central Pontides, and interpreted this to indicate that northward subduction of Neotethys was already active during Early Cretaceous time. The timing of termination of subduction and the onset of continental collision is also debatable. Peccerillo & Taylor (Reference Peccerillo and Taylor1976), Şengör & Yılmaz (Reference Şengör and Yilmaz1981) and Robinson et al. (Reference Robinson, Spadini, Cloetingh and Rudat1995) envisaged that subduction continued into Eocene time. However, Yılmaz et al. (Reference Yilmaz, Tüysüz, Yiğitbaş, Genç, Şengör and Robinson1997) noted the existence of an angular unconformity between Upper Cretaceous volcanic rocks and Middle Eocene sedimentary rocks, while Okay & Şahintürk (Reference Okay, Şahintürk and Robinson1997a) suggested that subduction ended in Paleocene time and was followed by Eocene magmatism in a post-collisional setting.
2.b. South Tethyan margin
The southern margin of the Northern Neotethys in Eastern Anatolia is represented by part of the regional Tauride–Anatolide Platform (also known as the Menderes–Tauride Platform) in the Munzur Mountains (Fig. 1; Bergougnan, Reference Bergougnan1975; Şengör & Yılmaz, Reference Şengör and Yilmaz1981; Özgül & Turşucu, Reference Özgül, Turşucu, Tekeli and Göncüoğlu1984; Okay & Tüysüz, Reference Okay, Tüysüz, Durand, Jolivet, Horváth and Séranne1999). In contrast to the Eurasian basement of the Pontides, the Tauride–Anatolide Platform shows a similar stratigraphy and biostratigraphy to Northern Arabia and North Africa (Gondwana) at least from Late Jurassic time onwards (Şengör & Yılmaz, Reference Şengör and Yilmaz1981; Robertson & Dixon, Reference Robertson, Dixon, Dixon and Robertson1984; Dercourt et al. Reference Dercourt, Zonenshain, Ricou, Kazmin, LePichon, Knipper, Grandjacquet, Sbortshikov, Geyssant, Leprevier, Pechersky, Boulin, Sibuet, Savostin, Sorokhtin, Westphal, Bazhenov, Lauer and Biju-Duval1986, Reference Dercourt, Gaetani, Vrielynck, Barrier, Biju-Duval, Brunet, Cadet, Crasquin and Sandulescu2000). The succession in the Munzur Mountains comprises algal, stromatolitic and rudist-bearing shallow-water carbonates of Late Triassic–Late Cretaceous age (Munzur Dağı Formation; Özgül & Turşucu, Reference Özgül, Turşucu, Tekeli and Göncüoğlu1984) and is interpreted as a stable carbonate platform above a metamorphic basement (Yoncayolu Formation: Özgül & Turşucu, Reference Özgül, Turşucu, Tekeli and Göncüoğlu1984). The top of the succession exhibits a transition to deep-water facies of probable Campanian–Maastrichtian age (Ayıkayası Formation). The Munzur platform succession is tectonically overlain by ophiolitic mélange that is interpreted as having been derived from the north (Bergougnan, Reference Bergougnan1975; Özgül & Turşucu, Reference Özgül, Turşucu, Tekeli and Göncüoğlu1984; Temiz et al. Reference Temiz, Guezou, Poisson and Tutkun1993). The platform is inferred to have subsided ahead of advancing nappes of ophiolitic mélange during Campanian–Maastrichtian time (Kurtman, Reference Kurtman1961; Özgül & Turşucu, Reference Özgül, Turşucu, Tekeli and Göncüoğlu1984; Özer, Koç & Özsayar, Reference Özer, Koç and Özsayar2004).
3. Revised tectonostratigraphy
Our revised tectonostratigraphy of the Erzincan area is shown in Figure 2 and Table 1. Six main tectonostratigraphic units of Late Cretaceous to Early Eocene age are identified (S. P. Rice, unpub. Ph.D. thesis, Univ. Edinburgh, 2006; Rice, Robertson & Ustaömer, Reference Rice, Robertson, Ustaömer, Robertson and Mountrakis2006). We begin by discussing the foredeep succession in the Munzur Mountains to the south (Ayıkayası Formation). Then we summarize the ophiolitic rocks (Refahiye Complex), the accretionary mélange (Karayaprak Mélange) and the Upper Cretaceous arc-type rocks (Karadağ Formation). We then describe Upper Cretaceous and Lower Palaeogene mainly sedimentary rocks of fore-arc basin type (Sütpınar Formation). All of the above units exhibit north-vergent deformational structures. Lastly, we describe transgressive Upper Paleocene–Lower Eocene facies (Sipikör Formation). The Sipikör Formation lacks north-vergent structures. Further details of the structural geology of these units and the geochemistry of the ophiolitic and arc-related rocks are given in Rice, Robertson & Ustaömer (Reference Rice, Robertson, Ustaömer, Robertson and Mountrakis2006).
3.a. Campanian–Maastrichtian Ayıkayası Formation
3.a.1. Previous work
The Ayıkayası Formation (Özgül &Turşucu, Reference Özgül, Turşucu, Tekeli and Göncüoğlu1984) takes its name from a hill in the Munzur Mountains.
3.a.2. Lithology
The Ayıkayası Formation exhibits a coarsening-upward succession that begins with thinly bedded pelagic limestones interbedded with oolitic and bioclastic calcarenites and then passes stratigraphically upwards into coarse, matrix-supported, poorly sorted, normal-graded, breccia and conglomerate with scoured, erosive bed bases (Figs 4e, 5a,). In general, the clasts are mainly angular to sub-rounded neritic limestone, basic lava, red mudstone and chert. At one specific locality (Muratboynu; Figs 3, 4e, 5a) the formation locally begins with a unit of pink pelagic limestone, ~3 m thick. This is overlain by numerous thick-bedded, or massive (~2 m), matrix-supported conglomerates. The conglomerates contain large (< 50 cm) rounded, to sub-angular, boulders and pebbles of grey coarse biosparite and also angular to sub-angular pebbles and granules of red chert and red pelagic limestone. Individual beds tend to be dominated by either angular red pelagic clasts or rounded neritic limestone clasts.

Figure 4. Composite logs (a–f) of the Upper Cretaceous sequences in the İzmir–Ankara–Erzincan Suture Zone in the Eastern Pontides, arranged from left to right according to general structural position (a – highest; e – lowest). Note the differences in the scales of the individual logs (see Fig. 3 for locations). From Rice, Robertson & Ustaömer (Reference Rice, Robertson, Ustaömer, Robertson and Mountrakis2006).

Figure 5. Field photographs of the Ayıkayası Formation (a), Refahiye Ophiolitic Complex (b, c), Karayaprak Mélange (d), Karadağ Formation (e), Sütpınar Formation (f), Sipikör Formation (g) and Miocene cover (h). See Figure 3 for locations. (a) Globotruncana-bearing pelagic limestone and coarse conglomeratic debris-flow deposit within the Ayıkayası Formation, exposed ~3 km south of Muratböynü. (b) A thin screen of banded highly strained amphibolitic schistose country rock within a diabase dyke swarm within the Refahiye Ophiolitic Complex, exposed near Göyne. (c) 100% sheeted dykes in the Refahiye Ophiolitic Complex exposed north of Işıkpınar. (d) Typical lithological assemblage of the Karayaprak Mélange; trains of large (up to ~30 m long) fault-bounded lozenges of basaltic lava and neritic limestone within a sheared serpentinite matrix, exposed at Muratböynü. (e) Schistose metavolcaniclastic conglomerate within the Karadağ Formation exposed at Köhnemdağı. (f) Boudinaged beds of hemipelagic limestone with pelitic interbeds exhibiting a pervasive top-to-the-north shear fabric, exposed west of Ballı. (g) Matrix-supported polymict conglomerate typical of the Sipikör Formation, exposed north of Gökkaya. (h) Large (~1 m) fossilized algal mounds(?) within Miocene bedded oolitic bioclastic grainstones and packstones, exposed at Mercidiye.
3.a.3. Lower and upper boundaries
The unit lies above thick neritic limestone (Kabataş Member of the Munzur Dağı Formation) that dominates the Mesozoic Munzur carbonate platform to the south of the area studied. The Ayıkayası Formation begins with a sharp but conformable contact (Table 1, Fig. 2; Özgül &Turşucu, Reference Özgül, Turşucu, Tekeli and Göncüoğlu1984). Its upper stratigraphic boundary has been removed by south-vergent thrust faulting.
3.a.4. Thickness and lateral extent
The only known exposure of the Ayıkayası Formation that was accessible during this work is situated ~10 km south of Muratboynu (Fig. 3), where it reaches a thickness of ~25 m (Fig. 3). The outcrop there is limited to thin remnants of the uppermost part of the Munzur Dağı sequence that escaped erosion (Özgül & Turşucu, Reference Özgül, Turşucu, Tekeli and Göncüoğlu1984).
3.a.5. Age
The Ayıkayası Formation was previously dated as Turonian–Campanian, based on the Foraminifera Praeglobotruncana delrioensis diumn, Globotruncana alpina Bolli, Globotruncana renzi Gandolfi, Globotruncana linneiana (D'Orbigny) and Globotruncana helvetica Bolli (Özgül & Turşucu, Reference Özgül, Turşucu, Tekeli and Göncüoğlu1984). During this study, samples of limestone from the Ayıkayası Formation yielded only reworked Jurassic and Cretaceous Siphovalvulina sp., together with Charentia sp., Rotaliidae and echinoderm debris. However, comparable facies exposed at Köhnemdağı (Fig. 3), a section that is likely to correlate with the Ayıkayasi Formation, have yielded the Campanian–Maastrichtian pelagic foraminifera Globotruncana linneina (D'Orbigny). The Ayıkayası Formation is, therefore, believed to be of latest Cretaceous (Campanian–Maastrichtian) age, and also contains reworked older microfossils. Microfossil reworking is known to reflect erosion, for example, due to uplift or current activity (Van Gorsel, Reference Van Gorsel1988; Armentrout, Reference Armentrout1987).
3.a.6. Interpretation
The very coarse, poorly sorted texture of the some of the conglomerates suggests a relatively nearby source and local deposition by sediment gravity flows. Clast composition varies between beds, suggesting that different local sources existed. The internal texture of biosparite clasts in some of the conglomerates is identical to lithologies in the stratigraphically underlying Munzur Dağı Formation. These clasts were probably derived from the carbonate platform in response to faulting and tectonic subsidence. The red pelagic chert and pelagic limestone clasts have no potential source within the Munzur carbonate platform and were instead derived from the mélange (Karayaprak Mélange) that was overthrust from the north (see Section 3.c). Overall, the Ayıkayası Formation records the transition from a shallow-marine to a deep-marine environment accompanied by input of coarse, poorly sorted sediment gravity flows from multiple sources. Rapid tectonically triggered denudation of these source areas is consistent with the evidence of microfossil reworking. The Mesozoic carbonate platform is assumed to have flexurally upwarped and then collapsed, associated with the emplacement of oceanic units from the Northern Neotethys.
3.b. Refahiye Complex
3.b.1. Previous work
This unit was named by Aktimur et al. (Reference Aktımur, Yurdakul, Sariaslan, Göksel, Keçer, Yildirim, Akkuş and Erler1995) after a town located 70 km west of Erzincan (Fig. 3) and is well exposed along the Refahiye–Erzincan road. The Refahiye Complex has also been referred to, or mapped as, the Zone Intermédiaire des Péridotites d'Erzincan (Bergougnan, Reference Bergougnan1975), the Refahiye Karmaşığı/Erzincan Nappe (Yılmaz, Reference Yilmaz1985), the Karakaya Kompleksi (in part), Karadağ Kompleksi, and İç Toros Kompleksi (in part: Koçyiğit, Reference Koçyığızt1990), Ofiyolitli Melanj (in part: Okay & Şahintürk, Reference Okay, Şahintürk and Robinson1997a,Reference Okay and Şahintürkb), and the Uluyamaç Ophiolite (Özer, Koç & Özsayar, Reference Özer, Koç and Özsayar2004).
3.b.2. Lower and upper boundaries
The base of the ophiolitic Refahiye Complex is a north-dipping thrust, wherever observed. The boundaries are commonly characterized by serpentinitic shear zones displaying south-vergent shear fabrics and fluid alteration of the surrounding rocks. In places, the Refahiye Complex is unconformably overlain by the less deformed Upper Paleocene–Lower Eocene Sipikör Formation and younger sedimentary rocks (Figs 2, 3).
3.b.3. Thickness and lateral extent
The apparent thickness of the Refahiye Complex is ~8 km. The ophiolite is very well exposed to the north and northwest of the Neotectonic Erzincan pull-apart basin and crops out as an ~8 km wide belt extending eastwards and westwards beyond the area studied (Fig. 3).
3.b.4. Age
A minimum age of Late Paleocene–Eocene for the formation and emplacement of the Refahiye Complex is given by the oldest unconformably overlying sediments (Sipikör Formation). Previous studies suggested a Late Cretaceous age based on this stratigraphic relationship (e.g. Bergougnan, Reference Bergougnan1975; Yılmaz, Reference Yilmaz1985; Koçyiğit, Reference Koçyığızt1990; Aktimur et al. Reference Aktımur, Yurdakul, Sariaslan, Göksel, Keçer, Yildirim, Akkuş and Erler1995; Okay & Şahintürk, Reference Okay, Şahintürk and Robinson1997a,Reference Okay and Şahintürkb), but no radiometric dating has yet been published on the Refahiye Complex. An alternative, Jurassic, age may also be possible in view of the radiometrically determined Jurassic ages of comparable ophiolitic rocks in the Caucasus to the east (Sosson et al. Reference Sosson, Rolland, Corsini, Danelian, Stephan, Avagyan, Melkonian, Jrbashyan, Melikian and Galoian2005) and in the Ankara Mélange to the west (Dilek & Thy, Reference Dilek and Thy2006).
3.b.5. Lithological variation
In the Erzincan area, the Refahiye Complex is mainly made up of the typical lithologies of the lower part of an ophiolitic pseudostratigraphy, that is, mantle tectonite, layered and isotropic gabbro and sheeted diabase (Fig. 4a; Table 1). Extrusive rocks and overlying deep-sea (‘epiophiolitic’) sedimentary rocks are, however, absent. Serpentinized peridotite dominates the central and northern parts of the outcrop and is tectonically imbricated with Pontide ‘basement’ to the north of Erzincan. The ophiolitic complex is composed of > 70% (by volume) serpentinized harzburgite, ~20% diabase and minor amounts of gabbro and trondhjemite/plagiogranite.
The serpentinized harzburgite exhibits a relict mineral foliation (S1), commonly overprinted by younger fabrics related to emplacement (Late Cretaceous/Early Palaeogene), or neotectonics (Miocene–Recent). Hydrothermal (metasomatic) alteration of the ultrabasic rocks has replaced the original mineralogy with serpentine group minerals to a varying degree. Cumulate rocks generally crop out between areas of sheeted dykes and minor gabbro to the south, and serpentinized harzburgite to the north (Fig. 3). A primary cumulate layering is locally preserved within relatively undeformed lenses, surrounded by fissile, sheared serpentinite. The layered cumulates are locally intruded by sub-vertical dykes of microgabbro (~5 m thick) and hornblende–phyric andesite (< 2 m thick), as seen at Işıkpınar (Fig. 3).
Isolated diabase dykes that cut the serpentinized peridotite are mainly fine-grained to medium-grained and contain primary plagioclase, whereas actinolite and minor amounts of bastite completely replace primary pyroxene. Plagiogranite (trondhjemite) dykes are less than 3 m thick and are relatively fine-grained, with occasional small (~1 mm) plagioclase phenocrysts set in a groundmass of plagioclase and secondary quartz. The isolated dykes commonly occur as trains of pull-apart blocks set in a matrix of fissile, sheared serpentinite. Isolated dykes also cut the metamorphic rocks described below (Fig. 5b).
A sheeted dyke complex is composed of basic diabase and microgabbro dykes and is well exposed west of Erzincan, towards Akbudak village (Fig. 3). In the north of this area the complex is composed of 100% dykes, with individual dykes ~1.5 m thick. North of Erzincan (e.g. Işıkpınar; Figs 3, 5c), swarms of multiple dykes occur as large blocks and shear pods (up to ~75 m long) within larger outcrops of sheared serpentinite. The dykes are commonly chloritized and altered to spilite, or uralite, indicating that hydrous greenschist-facies metamorphism has taken place.
An extraordinary feature, not seen in typical ophiolite dyke complexes, is the presence of screens of metamorphic rocks between dykes (Fig. 5b). This association is exposed particularly in the area west of Erzincan, along the road to Akbudak, where it is in tectonic contact with 100% sheeted dykes. The metamorphic rocks crop out in the southern part of the ophiolitic dyke complex and make up < 5% of the outcrop area of the Refahiye Complex as a whole. Individual dykes exhibit well-developed chilled margins against the metamorphic host rocks. The metamorphic rocks form screens, ranging in width from several centimetres between individual diabase dykes to more than 50 metres between thick swarms of dykes (Fig. 6a). The metamorphic lithologies include epidote–actinolite schist, metabasite, amphibole-bearing gneiss and massive marble. The screens of metamorphic rocks are cut by unmetamorphosed intrusive rocks including small dyke-like bodies of plagiogranite (trondhjemite), isolated diabase dykes and also rare andesite, rhyodacite and aplite dykes (0.15–10 m wide). The aplite dykes locally exhibit chilled margins against individual diabase and trondjhemite bodies, suggesting relatively late-stage intrusion. The metamorphic screens and cross-cutting dykes are commonly strongly sheared and, in places, entrained with ductile serpentinite.

Figure 6. Field sketches of the Refahiye Ophiolite (a), and Sütpınar Formation (b). See Figure 3 for locations. (a) Field sketch of the Refahiye Formation exposed at Göyne showing key intrusive relationships between metamorphic host rocks and successive intrusions. (b) Field sketch of the Sütpınar Formation exposed at Gökkaya showing top-to-the-north folds and thrusts cut by a top-to-the-south shear zone developed within a turbiditic shale, sandstone, conglomerate and limestone succession.
The Refahiye Complex exhibits a pervasive north-vergent shear-fabric, which suggests that the emplacement direction was from the south–southwest towards the north–northeast (Rice, Robertson & Ustaömer, Reference Rice, Robertson, Ustaömer, Robertson and Mountrakis2006).
3.b.6. Geochemical results
Five samples of peridotite from the ophiolitic Refahiye Complex were previously analysed using an electron microprobe to determine the composition of chrome spinels (S. P. Rice, unpub. Ph.D. thesis, Univ. Edinburgh, 2006). Four of these samples fall within, or close to, the compositional range of abyssal spinel-peridotites, whereas one sample exhibits a higher Cr number. Three peridotite samples collected along a 3 km transect within a single peridotite thrust sheet exhibit marked compositional variations, with each sample representing one of two petrogenetic types (abyssal- or Alpine-type peridotite: Dick & Bullen, Reference Dick and Bullen1984). Such variation is similar to the depletion and enrichment trends for chromites in peridotites of modern oceanic settings, including some spreading ridge segments and back-arc basins (Dietrich et al. Reference Dietrich, Emmermann, Oberhansli and Puchelt1978; Saunders & Tarney, Reference Saunders, Tarney, Kokelaar and Howells1984; Barker et al. Reference Barker, Christeson, Austin and Dalziel2003). In addition, the major- and trace-element chemical compositions of the diabase sheeted dykes and several isolated dykes have been analysed by X-ray fluorescence (Table 2; Rice, Robertson & Ustaömer, Reference Rice, Robertson, Ustaömer, Robertson and Mountrakis2006). The diabase sheeted dykes plot in the andesite–basalt field on the Zr/Ti–Nb/Y diagram (Fig. 7a). On MORB (mid-ocean ridge basalt)-normalized multi-element plots, the patterns of the sheeted and isolated dykes range from MORB-like with a slight Nb depletion to more depleted with a distinct negative Nb anomaly (Fig. 7b i and ii). The geochemical results suggest that the sheeted dykes and the isolated diabase dykes formed in an oceanic setting, influenced by subduction (Robertson, Parlak & Ustaömer, Reference Robertson, Parlak, Ustaömer, van Hinsbergen, Edwards and Govers2009).
Table 2. Geochemical analyses of igneous rocks from the Refahiye Complex in the Eastern Pontides


Figure 7. Whole-rock geochemistry of rocks from the Refahiye Complex: (a) Zr/Ti v. Nb/Y diagram; diamonds – isolated dykes intruding metamorphic host rocks, crosses – sheeted dykes; (b) MORB-normalized trace-element plots; (i) sheeted dykes, (ii) isolated dykes. Normalizing values: Sr, 120 ppm; K2O, 0.15%; Rb, 2.0 ppm; Ba, 20 ppm; Nb, 3.5 ppm; La, 3 ppm; Ce, 10 ppm; Nd, 8 ppm; P2O5, 0.12%; Zr, 90 ppm; TiO2, 1.5%; Y, 30 ppm; Sc, 40 ppm; Cr, 250 ppm (Pearce, Reference Pearce and Thorpe1982).
3.b.7. Interpretation
The Refahiye Complex is interpreted as part of an originally complete section of oceanic lithosphere, although the upper extrusive levels and associated deep-sea sediments are not preserved. Geochemical studies suggest that the ophiolite represents oceanic lithosphere that formed in a subduction-related tectonic setting, possibly a forearc, incipient arc or back-arc. Many of the harzburgitic ophiolites of the İzmir–Ankara–Erzican Suture Zone are cut by isolated diabase/micrograbbro dykes, and similar dykes also cut the Refahiye Complex harzburgite (e.g. northeast of Erzincan). Good examples of isolated dykes are also seen in the Mersin, Beyşehir, Lycian, Tekirova, Antalya ophiolites and the ‘Anatolide’ ophiolites (Parlak & Delaloye, Reference Parlak and Delaloye1996; Collins & Robertson, Reference Collins and Robertson1998; Dilek et al. Reference Dilek, Thy, Hacker, Grundvig and Anonymous1998; Andrew & Robertson, Reference Andrew and Robertson2002; Parlak, Höck & Delaloye, Reference Parlak, Höck, Delaloye, Bozkurt, Winchester and Piper2000; Robertson, Reference Robertson2002; Parlak & Robertson, Reference Parlak and Robertson2004; Robertson, Parlak & Ustaömer, Reference Robertson, Parlak, Ustaömer, van Hinsbergen, Edwards and Govers2009). Chemically, these dykes are all of supra-subduction zone (SSZ), island arc tholeiite (IAT) type and are widely interpreted as the products of incipient arc magmatism (Collins & Robertson, Reference Collins and Robertson1998; Dilek et al. Reference Dilek, Thy, Hacker, Grundvig and Anonymous1998; Parlak et al. Reference Parlak, Höck, Kozlu and Delaloye2004; Robertson, Parlak & Ustaömer, Reference Robertson, Parlak, Ustaömer, van Hinsbergen, Edwards and Govers2009). The rocks exhibit mainly north-vergent small- to meso-scale asymmetrical folds and thrust-duplexes associated with a pervasive shear-fabric, which suggests that the emplacement direction was from the south–southwest towards the north–northeast (Rice, Robertson & Ustaömer, Reference Rice, Robertson, Ustaömer, Robertson and Mountrakis2006). A Late Cretaceous age for the formation and emplacement of the Refahiye Complex is possible here because the oldest rocks stratigraphically overlying the Refahiye Complex belong to the Upper Paleocene–Eocene Sipikör Formation.
There are three possible explanations of the metamorphic rock screens that are locally present within the swarms of diabase dykes. The first, considered by Rice, Robertson & Ustaömer (Reference Rice, Robertson, Ustaömer, Robertson and Mountrakis2006), is that they represent a fragment of continental ‘basement’ that rifted from the Pontide (Eurasian) margin and was incorporated into a sheeted dyke complex during seafloor spreading. The rocks (e.g. schist, marble and metabasite) between the dykes are generally comparable with some of the ‘basement’ rocks of the Pontides, notably the Karakaya Complex (locally termed the Doğankavak Unit) and the high-grade metamorphic rocks of the Pulur Massif (Topuz et al. Reference Topuz, Altherr, Satur and Schwartz2004). Material within the Karakaya Complex, and probably the Pulur Massif also, was accreted from Palaeotethys to the Eurasian margin before Early Jurassic time (Pickett & Robertson, Reference Pickett and Robertson1996, Reference Pickett and Robertson2004; Okay, Reference Okay, Bozkurt, Winchester and Piper2000). A second possibility is that the metamorphic rocks and the sheeted dykes, together, represent a fragment of Eurasian continental ‘basement’, unrelated to the ophiolitic rocks. Comparable swarms of sheeted dykes cut Upper Palaeozoic basement in the Artvin area where the host rocks are schist, gneiss and amphibolite and are cut by dykes that are locally dated as Jurassic in the Eastern Pontides (Ustaömer & Robertson, Reference Ustaömer and Robertson2009). However, the cross-cutting dyke swarms form part of a wider outcrop of 100% sheeted dykes within the Refahiye Complex and are, therefore, unlikely to be older ‘basement’.
A third possibility is that the metamorphic screens represent a type of metamorphic sole of the ophiolitic harzburgite exposed to the north (Fig. 2). The metamorphic rocks might have formed by the accretion of oceanic material to the base of the supra-subduction-zone-type Refahiye ophiolitic harzburgite, where they were metamorphosed and then cut by swarms of dykes. One difficulty, however, is that meta-chert is a common constituent of Upper Cretaceous metamorphic soles elsewhere in the İzmir–Ankara–Erzincan Suture Zone, but this lithology was not observed within the metamorphic screens. However, it is possible that a seamount capped by limestone was accreted to the overlying ophiolite, in which case meta-chert need not be present. Another difficulty is that the cross-cutting dykes appear to represent part of the sheeted dyke complex that formed much higher in the oceanic lithosphere than the mantle harzburgite.
Elsewhere, sub-ophiolite metamorphic soles include a range of amphibolite and greenschist-facies rocks (meta-basalt, marble, metachert, serpentinite). The metamorphic soles typically show an inverted metamorphic gradient with amphibolite-facies rocks above, and greenschist-facies rocks below. Although typically only a few tens of metres thick, metamorphic soles can be up to several hundred metres thick (e.g. in Oman: Searle & Malpas, Reference Searle and Malpas1980). Also, metamorphic soles are commonly displaced from overlying harzburgitic bodies during emplacement. Elsewhere in the İzmir–Ankara–Erzincan Suture Zone, ophiolite-related metamorphic soles are locally cut by basaltic dykes. For example, a range of depleted to enriched-composition dykes cut the metamorphic sole and the overlying ultramafic ophiolitic rocks of the Karsantı ophiolite that overlies the Tauride platform to the south of the İzmir–Ankara–Erzincan Suture Zone (Lytwyn & Casey, Reference Lytwyn and Casey1995; Çelik, Reference Çelik2007).
Having considered several alternatives, we consider that the previous interpretation of Rice, Robertson & Ustaömer (Reference Rice, Robertson, Ustaömer, Robertson and Mountrakis2006) is the most realistic, that is, that the metamorphic screens represent Tethyan material that was previously accreted to the Eurasian margin (of Karakaya Complex type) and was later detached and intruded by subduction-influenced dykes during formation of the Refahiye Complex. This interpretation implies that supra-subduction zone spreading to form the Refahiye ophiolite took place near the Eurasian continental margin.
3.c. Upper Cretaceous Karayaprak Mélange
3.c.1. Previous work
This unit has previously been referred to, in part, as the Karayaprak Napı of the Anadolu Kompleksi (Koçyiğit, Reference Koçyığızt1990).
3.c.2. Lithology
The Karayaprak Mélange is a variably tectonized mixture of blocks and slices, individually up to about one kilometre long and several hundred metres wide.
The most common blocks and slices within the mélange are pale-grey, massive, crystalline pelagic limestone (Fig. 5d). Blocks of hydrothermally altered basaltic pillow lava commonly crop out together with red radiolarian chert, pelagic limestone and mudstone. Serpentinite occurs as blocks and slices and also as highly deformed inclusions along shear zones. Volumetrically subordinate components of the mélange include diabase, volcaniclastic shale and sandstone, gabbro, recrystallized and deformed pink pelagic limestone, rare plagiogranite, amphibolite and greenschist metavolcanic rocks. Sedimentary matrix is generally absent and the blocks are tectonically mixed (tectonic mélange). However, matrix-supported debris-flow deposits (‘olistostromes’) crop out near Kömür village north of Erzincan, where metre-scale blocks are set in a muddy matrix.
Two common lithological associations are recognized in the mélange: first, serpentinite + basalt + radiolarite (Association 1), and second, serpentinite + massive limestone + lava + volcaniclastic sedimentary rocks (Association 2). Basalt is interbedded with and overlain by neritic limestone in Association 2. Association 2 also contains coarse matrix-supported debrites composed of basic lava and massive limestone (Rice, Robertson & Ustaömer, Reference Rice, Robertson, Ustaömer, Robertson and Mountrakis2006).
3.c.3. Lower and upper boundaries
The lower contact of the Karayaprak Mélange is a thrust wherever observed. North-dipping thrust faults imbricate the mélange with units of different age in the area, including pre-Liassic basement (Karakaya Complex), and Miocene and younger cover rocks (Kemah Unit). The Karayaprak Mélange is unconformably overlain by the Upper Paleocene–Eocene Sipikör Formation, as seen at Muratboynu village (Fig. 3). In addition, near the Bayburt–Erzurum road, outside the study area, the Karayaprak Mélange is reported to be unconformably overlain by Maastrichtian shallow-water limestone (Kapıkaya Limestone: Ketin, Reference Ketin1951; Okay & Şahintürk, Reference Okay and Şahintürk1997b).
3.c.4. Thickness and lateral extent
The Karayaprak Mélange forms discrete slices, up to ~4 km thick and tens of kilometres long, that crop out across a wide area, imbricated with other units. Accessible exposures are found along the main highway east of Erzincan (Fig. 3). The mélange also crops out between the Euphrates (Firat) River and the Munzur Mountains, southwest of the town of Kemah (Fig. 3). Large outcrops also exist outside the study area, notably between Ovacık and Kemaliye and in the Munzur Mountains (Özgül & Turşucu, Reference Özgül, Turşucu, Tekeli and Göncüoğlu1984; Aktimur et al. Reference Aktımur, Atalay, Ateş, Tekırlı and Yurdakul1988).
3.c.5. Geochemical results
To shed light on the possible tectonic setting of eruption, seven samples of relatively unaltered lava from the Karayaprak Mélange were analysed by X-ray fluorescence (XRF) at the School of GeoSciences, University of Edinburgh, using the method of Fitton et al. (Reference Fitton, Saunders, Larsen, Hardarson, Norry, Saunders, Larsen and Wise Jr1998). The samples are of rhyodacitic and trachytic composition (Fig. 8a; Table 3). Rocks of Association 1 (Fig. 8b i) exhibit near-MORB abundances of the relatively immobile trace elements, with the exception of Nb and Cr. The variable Cr abundances probably reflect fractionation, while the persistent Nb depletion indicates a subduction influence on the mantle source. Basalts associated with the neritic limestones of Association 2 (Fig. 8b ii) are generally of intermediate composition and exhibit no Nb depletion, suggesting that they are not influenced by subduction. Their enriched patterns are comparable to alkali basalts and enriched MORB that characterize oceanic seamounts.
Table 3. Geochemical analyses of igneous rocks from the Karayaprak Mélange in the Eastern Pontides


Figure 8. Whole-rock geochemistry of rocks from the Karayaprak Mélange: (a) Zr/Ti v. Nb/Y diagram; circles – Association 1, triangles – Association 2; (b) MORB-normalized trace-element plots; (i) Association 1, (ii) Association 2. Normalizing values: Sr, 120 ppm; K2O, 0.15%; Rb, 2.0 ppm; Ba, 20 ppm; Nb, 3.5 ppm; La, 3 ppm; Ce, 10 ppm; Nd, 8 ppm; P2O5, 0.12%; Zr, 90 ppm; TiO2, 1.5%; Y, 30 ppm; Sc, 40 ppm; Cr, 250 ppm (Pearce Reference Pearce and Thorpe1982).
3.c.6. Age
The youngest age previously reported for blocks in the mélange is Mid-Eocene (Aktimur et al. Reference Aktımur, Yurdakul, Sariaslan, Göksel, Keçer, Yildirim, Akkuş and Erler1995). However, because the Karayaprak Mélange is unconformably overlain by the Upper Paleocene–Eocene Sipikör Formation, the presence of Eocene inclusions in the mélange is considered to be the result of later tectonic imbrication.
During this study, pelagic carbonate blocks in the mélange have yielded the planktonic foraminifera Globotruncana linneiana (D'Orbigny) and Archaeoglobigerina sp. of Campanian–Maastrichtian age and also the calpionellids Calpionella alpina (Lorenz) and Calpionella elliptica (Cadish) of Early Cretaceous (Berriasian) age. Elsewhere in the İzmir–Ankara–Erzincan Suture Zone, ages of Late Triassic to Late Cretaceous have been reported from pelagic sediments that are depositionally associated with basaltic lavas (Göncüoğlu et al. Reference Göncüoğlu, Yaliniz and Tekin2006).
The Karayaprak Mélange tectonically overlies the Campanian–Maastrichtian Ayıkayası Formation. To the north of the area that we studied, ophiolitic mélange is unconformably overlain by Maastrichtian limestone (Kapıkaya Limestone: Okay & Şahintürk, Reference Okay, Şahintürk and Robinson1997a). However, further south in our study area the oldest cover is the Upper Paleocene–Eocene Sipikör Formation.
3.c.7. Interpretation
The serpentinite + basalt + radiolarite (Association 1) is interpreted as accreted oceanic lithosphere. The radiolarian chert, micritic limestone and mudstone containing pelagic microfossils accumulated in a deep-marine pelagic/hemipelagic setting, comparable to modern abyssal plains (Iijima, Hein & Siever, Reference Iijima, Hein and Siever1983). The presence of the negative Nb anomaly in the basaltic lavas suggests a subduction influence (Pearce, Lippard & Roberts, Reference Pearce, Lippard, Roberts, Kokelaar and Howells1984), as in many Upper Cretaceous subduction-related ophiolitic basalts in Turkey and elsewhere (e.g. Robertson, Reference Robertson2002; Parlak et al. Reference Parlak, Höck, Kozlu and Delaloye2004). These basalts are similar to those of modern oceanic arcs (e.g. Tonga, Mariana: e.g. Pearce et al. Reference Pearce, Stern, Bloomer and Fryer2005). The serpentinite + massive limestone + lava + volcaniclastic rock association (Association 2) is interpreted as remnants of emplaced oceanic seamounts. These lavas are fractionated and lack an observable subduction influence. Elsewhere in the İzmir–Ankara–Erzincan Suture Zone, neritic limestones of Early Cretaceous age within the Ankara Mélange (central Anatolia) are depositionally associated with basalts of within-plate type that are interpreted as accreted oceanic seamounts (Rojay et al. Reference Rojay, Altıner, Özkan-Altıner, Önen, James and Thirlwall2004). The İzmir–Ankara–Erzincan Suture Zone as a whole is known to include basalts of mid-ocean ridge, seamount and subduction-influenced type (Göncüoğlu et al. Reference Göncüoğlu, Yaliniz and Tekin2006; Robertson, Parlak & Ustaömer, Reference Robertson, Parlak, Ustaömer, van Hinsbergen, Edwards and Govers2009). The basaltic rocks of the Karayaprak Mélange are likely to have erupted in a similar range of tectonic settings.
Other rock types, including deformed blocks of volcaniclastic and polymict sedimentary rocks could represent trench-fill deposits. For example, matrix-supported coarse debrites composed exclusively of basic lava and neritic limestone could have formed by mass wasting of a seamount as it approached a trench, prior to its tectonic accretion into the mélange.
The mélange is dominated by oceanic igneous rocks, together with pelagic/hemipelagic sedimentary rocks, with little matrix. Intersliced terrigenous sediments (e.g. turbidites) are absent. The evidence suggests that the accretionary wedge developed in an oceanic setting, away from any supply of continentally derived sediment. The Karayaprak Mélange is, therefore, interpreted as part of an emplaced Upper Cretaceous accretionary complex that formed at an appreciable distance from the Eurasian (Pontide) margin.
3.d. Campanian–Maastrichtian Karadağ Formation
3.d.1. Previous work
Karadağ means ‘black mountain’. There are several mountains in the Erzincan area named Karadağ; two of these correspond to outcrops of the Karadağ Formation, as defined here. The unit was previously mapped as the Refahiye Ophiolitic Complex (in part: Yılmaz, Reference Yilmaz1985), Karadağ Volkanitleri (Koçyiğit, Reference Koçyığızt1990), Karadağ basalt member (of the Çerpaçindere Formation: Aktimur et al. Reference Aktımur, Yurdakul, Sariaslan, Göksel, Keçer, Yildirim, Akkuş and Erler1995), or the Pazarcık Volkanics (Atalay, Reference Atalay1999).
3.d.2. Lithology
The Karadağ Formation comprises a thick succession (~3 km) of low-grade- metamorphosed hornblende- and plagioclase-phyric, basic to andesitic composition lavas, volcanogenic metasedimentary rocks and rare hemipelagic metacarbonate. The volcanogenic metasediments are mainly matrix-supported conglomerates, volcaniclastic sandstones, tuff and mudrocks (Table 1, Figs 4b, 5e). The composition of the clastic sediments is similar to the interbedded extrusive rocks. Schistosity is locally developed. The presence of a chlorite–epidote–albite–quartz assemblage indicates greenschist-facies metamorphism. Some sections are dominantly metasedimentary rocks, whereas others are mainly metavolcanic. Thick successions (> 3 km thick) of 100% andesite are observed on Karadağ, near Gökkaya, west of Erzincan and also at Karadağ, 10 km due south of Erzincan (Fig. 3).
The Karadağ Formation retains sedimentary structures and textures, especially in harder or coarser-grained rocks where the metamorphic fabric is less intense. The metasedimentary rocks are mainly thick-bedded (< 10 m), coarse-grained (< 40 cm), poorly-sorted, matrix-supported volcaniclastic conglomerates, which contain sub-rounded to sub-angular boulders and pebbles of feldspar-phyric, vesicular andesite and basalt. The conglomerates exhibit erosive bases and are interbedded with amalgamated, medium- to thick-bedded (< 5 m), coarse-grained (1 mm), texturally immature arkoses that contain ~30% quartz, ~30% feldspar and ~30% mafic grains. Thick (< 10 m), irregular lenses of pale yellowish massive tuff are interbedded with metalava flows and metaconglomerates in places.
3.d.3. Geochemical results
The whole-rock geochemistry of basaltic rocks from the Karadağ Formation, as reported by Rice, Robertson & Ustaömer (Reference Rice, Robertson, Ustaömer, Robertson and Mountrakis2006), is indicative of eruption in a volcanic arc setting.
3.d.4. Lower and upper boundaries
The lower contact of the Karadağ Formation is everywhere a north-dipping thrust. The upper boundary is commonly cut out by a thrust. Where intact, the unit is unconformably overlain by the Upper Paleocene–Eocene Sipikör Formation, as seen ~10 km south of Erzincan (near Yaylabaşı; Fig. 3), or by younger sedimentary rocks (Fig. 2).
3.d.5. Thickness and lateral extent
The Karadağ Formation occurs as discontinuous wedge-shaped thrust slices, up to 20 km long, with a maximum post-deformational thickness of ~3 km. A total outcrop area of 250 km2 is estimated in the Erzincan area. The best-exposed outcrop is at Köhnemdağı, a mountain 20 km west of Erzincan (Fig. 3). Other outcrops are located at Doğanbeyli, east of Erzincan near Çardaklı, and also south of the main road west of Erzincan, near Yeniköy and Berişerif (Fig. 3).
3.d.6. Age
Microfossils identified during this study from thin limestone interbeds within a dominantly volcanic rock succession exposed at Yaylabaşı (Fig. 3) include Globotruncana sp., suggesting a Late Cretaceous (Campanian–Maastrichtian) age for this unit.
3.d.7. Interpretation
The Karadağ Formation is interpreted as part of an Upper Cretaceous volcanic arc, dominated by andesitic volcanic rocks and volcaniclastic sedimentary rocks, with pelagic microfauna, but little or no terrigenous input. An arc edifice was constructed above the surrounding ocean floor and underwent mass wasting in a deep-sea setting to form volcanogenic debris flow deposits and turbidites. The presence of interbedded siliceous tuffs suggests that parts of the arc reached near or above sea level, although sedimentary evidence of shallow-water conditions was not observed. As no plutonic bodies were observed, it is inferred that only the upper part of the arc is preserved, possibly because the lower part of the arc was detached and subducted. Overall, the Karadağ Formation is interpreted as the higher levels of an immature oceanic arc.
3.e. Campanian–Lower Eocene Sütpınar Formation
3.e.1. Previous work
The Sütpınar Formation was first named by Koçyiğit (Reference Koçyığızt1990). The village of Sütpınar is located 12 km west–southwest of Erzincan. The unit was previously referred to as the Gözerek Formation (in part) and the Arıkaya Limestone (in part) (Özgül & Turşucu, Reference Özgül, Turşucu, Tekeli and Göncüoğlu1984), the Sütpınar Formation (Koçyiğit, Reference Koçyığızt1990), or the Çerpaçin member of the Çerpaçindere Formation (Aktimur et al. Reference Aktımur, Yurdakul, Sariaslan, Göksel, Keçer, Yildirim, Akkuş and Erler1995).
3.e.2. Lithology
The Sütpınar Formation consists of a ~1500 m thick coarsening-upward succession of mixed carbonate–siliciclastic sedimentary rocks and subordinate volcanogenic rocks (Fig. 4c). The formation interfingers with the Sipikör Formation in places (see Section 3.f). The lower part, ~800 m thick, is mainly medium-bedded calcarenite and quartzo-feldspathic sandstone. Individual beds are separated by laminated calcareous shaly partings. Bedded carbonate mudstones (wackestones) in the lower part of the Sütpınar Formation contain recrystallized radiolarians. Above, the succession is dominated by thick-bedded, pale calcarenite with rare andesitic lava flows and volcaniclastic matrix-supported conglomerate, together with thin-bedded, fine-grained, dark mudrock. The calcarenites are composed of calcite, volcanic quartz, and feldspar, together with oxide minerals and lithic clasts including basic lava, chert and meta-quartzite. The composition of the volcanic material in the sedimentary rocks is similar to that of the Karadağ Formation.
3.e.3. Lower and upper boundaries
The lower and upper boundaries of the Sütpınar Formation are commonly north-dipping thrusts. In the middle part of the suture zone, between Gökkaya village and Köhnemdağı (Fig. 3), the top of the Sütpınar Formation interfingers with the Sipikör Formation, as marked by the appearance of thick (up to 4 m) lenses of coarse polymict conglomerate and massive, texturally immature, compositionally varied sandstone. This lithostratigraphic transition roughly coincides with the disappearance of north-vergent structures (Fig. 6b).
3.e.4. Thickness and lateral extent
The present apparent thickness is ~8 km based on mapping. The succession exposed at Gökkaya measures ~1500 m (Fig. 4c). West of Erzincan, outcrop of the Sütpınar Formation forms a 25 km long belt that extends into mountains between the Euphrates River and the North Anatolian Fault Zone. Very good exposure is found in deep gorges that drain the southern, western and eastern slopes of Köhnemdağı. The best access is by foot from the village of Gökkaya (Fig. 3).
3.e.5. Age
Planktonic foraminifera (e.g. Globotruncana sp.) were identified during this study in the lower part of the succession and suggest a Late Cretaceous age. In addition, benthic foraminifera including Alveolina (Glomalveolina) sp. and Idalina sinjarica Grimsdale occur higher in the formation and indicate a Paleocene age (Fig. 9a, b). Benthic foraminifera (e.g. Alveolina sp.) were identified at even higher stratigraphic levels and are indicative of an Early Eocene age (Fig. 9a, b). The Sütpınar Formation is, therefore, assigned a Late Cretaceous to Early Eocene age.

Figure 9. Photomicrographs of selected microfossils from the Sütpınar Formation (a, b) and Sipikör Formation (c–i). (a) Coskinolina (Coskinon) rajkae Hottinger and Drobne, oblique transverse section, Thanetian, sample PO03 99. (b) Globotruncana arca (Cushman), axial section, Campanian–Maastrichtian, sample PO03 30. (c) Alveolina (Glomalveolina) sp. (a, axial section) and Idalina sinjarica Grimsdale (b, oblique section), Thanetian, sample PO03 99. Scale bars = 0.1 mm. (d) Morozovella subbotinae (Morozova), axial section, Lower Eocene, sample PO01 81. (e) Gyroidinella magna (Le Calvez), subequatorial section, Lower(?) Eocene, sample PO01 79. (f) Operculina complanata (Defrance), subaxial section, Lutetian, sample PO03 51. (g) Sphaerogypsina globula (Reuss), equatorial section, Lower Eocene, sample PO01 81. (h) Asterigerina rotula Kaufmann, axial section, Lower Eocene, sample PO01 81. (i) Orbitoclypeus sp., axial section, Lutetian, sample PO03 51.
3.e.6. Interpretation
The Sütpınar Formation is interpreted as a forearc basin that was located adjacent to an Upper Cretaceous active magmatic arc represented by the Karadağ Formation. Neritic carbonate and volcanic arc material was supplied to the forearc basin as a prograding submarine fan. The reduction of textural maturity upwards suggests an increase in depositional energy and source proximity with time.
The presence of siliceous radiolarians within pelagic carbonates of Late Cretaceous age in the lower part of the succession indicates an initially deep-water, open-marine setting above the CCD. These lower beds are interpreted as deep-water carbonate turbidites, comparable to deposits on the distal parts of calcareous submarine fans (e.g. Crati fan in Italy: Leeder, Reference Leeder1982; Ricci Lucchi et al. Reference Ricci Lucchi, Colella, Gabianelli, Rossi and Normark1984). The localized lenses of volcaniclastic conglomerate, coarse calcarenite and associated amalgamated sandstone are likely to represent channellized debris-flow deposits, whereas the shale and fine-grained sandstone are interpreted as overbank and inter-channel deposits.
The facies association in the middle part of the Formation (Paleocene–Lower Eocene) is suggestive of a mid-fan setting. Carbonates were redeposited from a marine shelf setting, rich in Nummulites sp., into a deep-water, open-marine environment. The sediments were transported by turbidity currents and debris flows within submarine channels. The commonly volcaniclastic composition of this part of the succession suggests derivation from a volcanic arc, presumably the Karadağ Formation. Rare metamorphic quartzite grains and rare mica within the calcarenites could represent metamorphosed siliceous volcanogenic material (e.g. from arc basement that is not exposed), or material derived from the adjacent Pontide continental margin. The presence of a single andesitic lava flow within the uppermost (Eocene) part of the succession suggests that the basin was volcanically active, perhaps not long before regional continental collision took place.
In summary, an overall regressive sequence developed in response to the progradation of a submarine fan, possibly also influenced by tectonics and sea-level change. The volcaniclastic lithologies are similar to those of the Karadağ arc unit and it is likely that the two were originally intergradational.
3.f. Upper Paleocene–Eocene Sipikör Formation
3.f.1. Previous work
This unit was named by Bergougnan (Reference Bergougnan1975) after the village of Sipikör, 20 km north of Erzincan (Fig. 3). It has also been referred to as the Köroğlu Formasyonu (Koçyiğit, Reference Koçyığızt1990), and the Gülandere Formation (Aktimur et al. Reference Aktımur, Atalay, Ateş, Tekırlı and Yurdakul1988, Reference Aktımur, Yurdakul, Sariaslan, Göksel, Keçer, Yildirim, Akkuş and Erler1995; Atalay, Reference Atalay1999).
3.f.2. Lithology
The Sipikör Formation is composed of a variety of siliciclastic and carbonate sedimentary rocks that exhibit complex lateral and vertical stratigraphic relationships. In places, the Sipikör Formation is transitional to the underlying Sütpınar Formation, as observed south of Köhnemdağı (Fig. 3). It begins with polymict matrix-supported conglomerates (Fig. 5g), together with homogeneous, massive, fine-grained limestone containing Nummulites sp. The conglomerate and the limestone both form thick (40 m) discontinuous lenses. The conglomerate is associated with amalgamated, thick-bedded, massive calcareous sandstone and calcarenite. Individual conglomerate lenses fine upwards to cross-bedded sandstone and litharenite with laminated tops. Thick beds of massive, cross-bedded, coarse-grained sandstone contain both angular and rounded quartz grains. Other grains include feldspar, calcite, metamorphic lithoclasts and chert. The sandstone also contains rare pebbles of recrystallized limestone, red chert and basalt. Rare thin beds of pelagic limestone, shale and siltstone are interbedded with sandstone and gravel. The top of the formation has been removed by a north-dipping thrust fault in the Köhnemdağı area.
The Sipikör Formation exhibits an overall fining-upward succession > 80 m thick, as seen near Yaylabaşı (Figs 3, 4f). In this area, and at Muratbounu (Fig. 3), the formation contains bivalves. The unit lies unconformably on the Karadağ Formation and the Karayaprak Mélange. Elsewhere, it is tectonically imbricated with Miocene sedimentary rocks. The first 30 m of the succession contain coarse angular lithoclasts. Conglomerate and sandstone gradually give way upwards to mudrocks interbedded with nummulitic limestone. Locally, the Sipikör Formation is composed of impure nodular micritic limestone with abundant Nummulites sp. (~1 cm in diameter) and coarse shell-rich partings (at Muratboynu; Fig. 3).
A > 50 m thick succession of dark grey, medium-grained litharenite and shale is exposed ~20 km north of Erzincan, near Kömür village (Fig. 3). This sandstone contains mainly angular mafic lithoclasts, feldspar, red chert and benthic foraminifera and is interbedded with dark grey, coarse-, medium- and fine-grained sandstone. The sandstone is well-sorted, and medium to thinly bedded.
3.f.3. Lower and upper boundaries
The Sipikör Formation rests unconformably on the Karayaprak Mélange, or on the Refahiye Complex in different areas (Figs 2, 3). However, the base of the Sipikör Formation appears to be conformable with the underlying Sütpınar Formation in a well-exposed section between Gökkaya village and Köhnemdağı. There, the base of the Sipikör Formation is marked by the appearance of lenses of polymict conglomerate (~4 m thick), interbedded with thick (~40 m) lenses of massive fine-grained nummulitic limestone. The Sipikör Formation is unconformably overlain by Miocene and younger sedimentary and volcanic rocks (e.g. near Kemah; Fig. 3). However, the exposed upper contact at many localities is a north-dipping thrust fault.
3.f.4. Thickness and lateral extent
The thickness of the Sipikör Formation is estimated as > 500 m in the Köhnemdağı area where an almost complete relatively undeformed section crops out.
3.f.5. Age
In agreement with previous studies (Okay & Şahintürk, Reference Okay, Şahintürk and Robinson1997a; Topuz et al. Reference Topuz, Altherr, Satur and Schwartz2004), the Sipikör Formation is dated as Late Paleocene–Eocene, based on planktonic and benthic foraminifera. During this study the following fossils were identified: Alveolina ellipsoidalis Schwager, Alveolina pasticillata Schwager, Assilina sp., Asterigerina rotula Kaufmann, Alveolina (Glomalveolina) sp., Coskinolina (Coskinon) rajkae Hottinger & Drobne, Discocyclina sp., Gyrodinella magna (Le Calves), Idalina sinjarica Grimsdale, Lockhartia haimei Davies, Nummulites millecaput Boubee, Nummulites striatus (Bruguiere), Operculina sp., Ophthalmidium sp., Rotalia trochidiformis Lamarck, Sphaerogypsina carteri Silvestri, Sphaerogypsina globula (Reuss), Spirolina sp., as well as the coral Litharaeopsis subepithecata (Oppenheim). Age-diagnostic micofossils are shown in Figure 9c–i.
In addition, calcisphere Pithonella ovalis (Kaufmann) of Albo-Cenomanian–Turonian age and planktonic foraminifera Rugoglobigerina sp. of Campanian–Maastrichtian age were identified in the Sipikör Formation, but these are inferred to be reworked.
3.f.6. Interpretation
The Sipikör Formation mainly accumulated in a high-energy shallow-marine environment, in which generally poor sorting suggests reworking, mainly by currents. The Sipikör Formation is the oldest unit within the suture zone that contains abundant terrigenous material derived from the Pontide margin as well as from all the underlying mainly Upper Cretaceous units.
In different areas, the Sipikör Formation rests unconformably on the Upper Cretaceous Refahiye Complex, the Karadağ Formation and the Karayaprak Mélange. This unit lacks the north-vergent structures that are seen in the structurally underlying Upper Cretaceous units. The Formation is, therefore, interpreted as a transgressive cover deposited after northwards tectonic emplacement of the Upper Cretaceous units during Late Cretaceous time. Together with the underlying units, the formation was thrust southwards during post-Early Eocene time. Further north, near the Bayburt–Erzurum road, facies equivalents of the Sipikör Formation unconformably overlie Maastrichtian neritic limestones of the Kapıkaya Formation, which is not exposed in our study area (Okay & Şahintürk, Reference Okay and Şahintürk1997b).
3.g. Miocene cover units
Miocene and younger cover rocks crop out extensively in the Sivas Basin to the west of Erzincan and also in the Pontides to the north (e.g. Aktimur, Tekirli & Yurdakul, Reference Aktımur, Tekirli and Yurdakul1990). These sedimentary rock units comprise a variety of shallow-marine to terrestrial clastic, carbonate and evaporitic sedimentary rocks (Fig. 5h) that unconformably overlie Lower Palaeogene and older rock units. The rocks post-date the closure of the Northern Neotethys and formation of the İzmir–Ankara–Erzincan Suture Zone and will not be considered further here.
4. Alternative tectonic models
Alternative tectonic models for the closure of the Northern Neotethys and formation of the İzmir–Ankara–Erzincan Suture Zone are discussed in Rice, Robertson & Ustaömer (Reference Rice, Robertson, Ustaömer, Robertson and Mountrakis2006). One possible model is shown in Figure 10. The overall tectono-stratigraphy of the Upper Cretaceous units across the suture zone (Fig. 2; Table 1) suggests that northward-dipping subduction occurred beneath the Pontide continental margin during Late Cretaceous time. The inferred accretionary complex (Karayaprak Mélange) and the forearc basin (Sütpınar Formation) are located at structurally lower levels to the south of the volcanic arc unit (Karadağ Formation), whereas the supra-subduction zone ophiolite (Refahiye Complex) is located further north and at a higher structural level. Structural restoration of the suture zone would indicate that in latest Cretaceous time the Pontide (Eurasian) margin was located to the north, followed southwards by a supra-subduction zone ophiolite, then by an Upper Cretaceous volcanic arc and forearc basin. All of these units were emplaced southwards over the Munzur carbonate platform (Ayıkayası Formation). Ophiolitic mélange crops out both to the north and the south of the arc and the ophiolite.

Figure 10. One possible model for the assembly of the Izmir–Ankara–Erzincan Suture Zone in the Eastern Pontides based on the tectonostratigraphy outlined in this paper. See text for explanation. SSZ – supra-subduction zone. For other abbreviations, see Figure 1.
The oldest Cretaceous subduction-related volcanic rocks in the Eastern Pontides are Turonian in age and are exposed in the Black Sea coastal area (Taner & Zaninetti, Reference Taner and Zaninetti1978). The regionally extensive Eastern Pontide volcanic arc is assumed to have formed related to northward subduction of Northern Neotethys (Yılmaz et al. Reference Yilmaz, Tüysüz, Yiğitbaş, Genç, Şengör and Robinson1997; Okay & Şahintürk, Reference Okay, Şahintürk and Robinson1997a).
The allochthonous units exposed in the Erzincan area also document an Upper Cretaceous (Campanian–Maastrichtian) volcanic arc (Karadağ Formation). It is assumed that this was bordered to the north by a supra-subduction zone-type harzburgitic ophiolite (Refahiye Complex) and to the south by an accretionary prism (Karayaprak Mélange) and related forearc basin (Sütpınar Formation).
There are three main options to explain the Late Mesozoic tectonic development of the Eastern Pontides:
First, a single, north-dipping subduction zone (relatively fixed in position) was activated beneath the southern margin of Eurasia, and in time generated all of the Upper Cretaceous magmatic arc rocks, including those overlying the Pontide continental basement in the north and those now within the suture zone to the south (e.g. Erzincan area). The main problems are to explain the width of the arc magmatic zone (several hundred kilometres), the means of generating the supra-subduction-type ophiolite and the lack of a continental margin trench-accretionary assemblage rich in terrigenous material.
Second, two north-dipping subduction zones could have been activated, one beneath the Pontide margin, as above, to create the Pontide arc and a second intra-oceanic subduction zone within Northern Neotethys to the south to generate the observed accretionary prism, volcanic arc and supra-subduction zone ophiolite. The two subduction zones would have collided and merged during final Mid-Eocene continental collision. The main problems with this interpretation are the absence of two contrasting trench-accretionary complexes, one continental margin and the other oceanic. However, this option cannot be excluded, especially as accretionary mélange is present in several different parts of the suture zone.
Third, a single north-dipping subduction zone was activated beneath the Eurasian margin. The Eastern Pontide arc developed prior to Campanian time. The subduction zone then migrated (rolled back) southwards, generating arc magmatism in a (marginal) oceanic setting. Two such settings can be envisaged. In one, a marginal basin (Refahiye Complex) opened behind an arc (Karadağ Formation), as suggested by Rice, Robertson & Ustaömer (Reference Rice, Robertson, Ustaömer, Robertson and Mountrakis2006). The screens of metamorphic rocks within sheeted diabase dykes could then represent a rifted fragment of Pontide ‘basement’ (e.g. older accretionary material). The main problem with this model is that the marginal basin should have formed by splitting of an arc. However, the arc-related intrusive rocks (e.g. isolated diabase dykes, plagiogranite dykes; rhyo-dacitic dykes and small aplitic intrusions) cutting the harzburgitic Refahiye ophiolite suggest that arc magmatism followed the formation of the ophiolite. This is explicable if the ophiolite first formed during roll-back of the subducting oceanic plate away from the Pontide continental margin, followed by the construction of a (marginal) oceanic arc (Karadağ Formation; Fig. 10a, b). Some of the arc-related magmas (diabase and plagiogranite dykes) then intruded the already formed surpra-subduction zone oceanic lithosphere (Fig. 10b). The screens of metamorphic rocks within the sheeted dykes could again represent rifted continental material, but might also have accreted contemporaneously and then been intruded by swarms of dykes related to the construction of the arc. In this interpretation, the subduction zone might have later rolled forward as subduction was impeded by the approaching Tauride margin (Fig. 10c). This could have caused loss of material (subduction erosion) and compression, leading to the observed early stage of northward thrusting as best developed in the north of the area, and also the emplacement of oceanic material onto the Pontide margin as documented north of the study area.
In any of the above three scenarios, the Tauride passive margin, represented by the Munzur Dağ Formation, migrated generally northwards until it reached the subduction trench, causing rapid flexural subsidence of the margin during Campanian–Maastrichtian time (Fig. 10c). The accretionary complex (Karayaprak Mélange) and all of the Upper Cretaceous units further north (forearc, arc, ophiolite) were then thrust southwards over the foundered Munzur platform, causing the second-stage southward thrusting mainly seen in the south of the area.
By the end of Cretaceous time, the suture zone was loosely assembled but oceanic remnants remained more regionally. Compressional deformation apparently ceased during Late Paleocene–Early Eocene time when remnant oceanic lithosphere subducted beneath Eurasia. This allowed the Early Palaeogene facies of the Sipikör Formation to transgress all of the Upper Cretaceous suture zone units. The Upper Cretaceous forearc basin (Sütpınar Formation) shallowed upwards and evolved into a syn-collisional basin by Early Eocene time (Sipikör Formation), during which compositionally and texturally immature siliciclastic sediments were deposited in a shallow-marine to non-marine environment with a substantial terrigenous input.
During Late Paleocene–Early Eocene time, forearc-type basins developed across much of Anatolia in response to late-stage subduction (e.g. Central Anatolian Basins: Görür, Tüysüz & Şengör, Reference Görür, Tüysüz and Şengör1998). Several of these basins include volcanic rocks that are attributed to transtension and local adjustments between loosely assembled microcontinental blocks (e.g. the Ulukışla Basin: Clark & Robertson, Reference Clark and Robertson2002). Further east, the Talysh unit of Azerbaijan may reflect a similar tectonic setting (Vincent et al. Reference Vincent, Allen, Ismail-Zadeh, Flecker, Foland and Simmons2005). This phase of suture assembly continued until Mid-Eocene time when regional ‘hard’ collision of Arabian and Eurasian continental crust took place. This resulted in large-scale, mainly south-vergent folding and thrusting of both the Upper Cretaceous and Lower Palaeogene units with the Erzincan area (Fig. 10d). Further north, the Mesozoic Pontide continental margin was delaminated and emplaced northwards as regional-scale thrust sheets. Suture tightening, which involved mainly southward thrusting in the Erzincan area, took place after the Miocene post-collisional cover began to accumulate and this, in turn, was followed by neotectonic strike-slip deformation (Fig. 10e).
5. Conclusions
(1) The revised stratigraphy presented here clarifies the age and stratigraphic context of Tethyan units exposed in the Eastern Pontides. Six important formations of Mesozoic–Early Palaeogene age are identified: the Ayıkayası Formation, the Refahiye Complex, the Karayaprak Mélange, the Karadağ Formation, the Sütpınar Formation and the Sipikör Formation.
(2) The Ayikayasi Formation, of Campanian–Maastrichtian age, lies conformably on the Tauride passive margin sequence in the Munzur Mountains in the south and is interpreted as an Upper Cretaceous flexural foredeep basin. Clast compositions within debrites of the Ayıkayası Formation indicate that this basin developed during emplacement of oceanic material (accretionary prism, ophiolite and arc volcanics) over the collapsed northern margin of the Tauride platform (Gondwana related).
(3) The Refahiye Complex, of possible Late Cretaceous age, comprises the deeper parts of an ophiolite pseudostratigraphy including harzburgite tectonites, layered cumulates, gabbroic rocks and diabase dykes. The occurrence of rare aplite and plagiogranite intrusions, together with the composition of chrome spinels in harzburgite, and of immobile trace elements in the diabase dykes suggests that the ophiolitic rocks formed in a supra-subduction zone setting, possibly in a forearc, arc, or back-arc position.
(4) Local screens of metamorphic rocks between sheeted dykes (e.g. marble, schist, phyllite, gneiss, serpentinite) are likely to have rifted from the adjacent Pontide continental margin. A less likely alternative is that contemporaneous oceanic material accreted to the sole of the Refahiye ophiolitic harzburgite and was detached and emplaced within higher-level crustal units.
(5) The Karayaprak Mélange developed during Late Cretaceous time as an oceanic accretionary prism and exhibits two lithological associations, interpreted as subduction-related volcanics (oceanic arc) and dismembered oceanic seamount(s).
(6) The Karadağ Formation is interpreted as an emplaced oceanic volcanic arc of Campanian–Maastrichtian age.
(7) The Sütpınar Formation is inferred to be an emplaced Campanian–Maastrichtian regressive forearc basin succession.
(8) North-vergent deformation affected all of the above units prior to Maastrichtian time, especially in the north of the area. In addition, particularly in the south, units were affected by south-vergent deformation that was probably related to collision of the subduction trench with the Tauride passive continental margin.
(9) The Sipikör Formation, of Late Paleocene–Eocene age, is the oldest unit unconformably overlying the deformed Upper Cretaceous units in the Erzincan area. North of the study area, Maastrichtian shallow-marine limestones unconformably overlie ophiolitic mélange and constrain initial northward emplacement of oceanic units onto the Eurasian continental margin as pre- or syn-Maastrichtian in this area.
(10) Transgressive shallow-marine sediments of the Sipikör Formation accumulated during Early Paleocene–Early Eocene time, supplied from all of the older suture zone units and the Pontide continental margin.
(11) Tectonic models involving one or two northward-dipping subduction zones beneath Eurasia are considered. A possible alternative is that a single subduction zone generated the Eastern Pontide continental margin arc (pre-Campanian). Roll-back of the subducting slab then allowed genesis of the supra-subduction-type harzburgitic Refahiye ophiolite and the oceanic Karadağ arc. These units were then dismembered during north-directed thrusting, possibly in response to slab roll-to (subduction erosion). This deformation and emplacement was approximately contemporaneous with the arrival of the leading edge of the Tauride passive margin (Munzur carbonate platform) at the subduction trench. Units were also emplaced southwards over the Munzur carbonate platform at this time. Any remaining oceanic crust in the region subducted during Early Palaeogene time, followed by forceful collision of the Eurasian and Tauride plates and mainly southward thrusting prior to Late Eocene time (40 Ma).
Acknowledgements
This project was funded by a NERC Ph.D. studentship. S. R. acknowledges Ö. Karslıoğlu, L. Meston and Y. Aydar for their assistance in the field. The manuscript benefited from the comments of Y. Dilek and an anonymous reviewer.