1. Introduction
During Precambrian time plankton communities were microbial, comprising the primary producing phytoplankton and the bacterial biomass that degraded dead phytoplankton and returned the nutrients to the water column to be recycled into the next generation of phytoplankton. This ecosystem changed dramatically through the late Proterozoic – earliest Palaeozoic interval with the evolution of metazoans, the colonization of the water column by zooplankton and the development of the complex marine ecosystems of the Phanerozoic Eon (Butterfield, Reference Butterfield2011). Arthropods were likely in the vanguard of early plankton colonization and have been a numerically important component of the ocean zooplanktonic ecosystem since Cambrian time (Signor & Vermeij, Reference Signor and Vermeij1994; Rigby & Milsom, Reference Rigby and Milsom2000; Peterson et al. Reference Peterson, Lyons, Nowak, Takacs, Wargo and McPeek2004; Vannier, Reference Vannier, Williams, Haywood, Gregory and Schmidt2007). In modern planktonic ecosystems, arthropod zooplankton are essential intermediates between the primary producers and secondary and tertiary consumers (Falkowski, Reference Falkowski2012). Arthropod zooplankton are important consumers of phytoplankton, bacterioplankton, marine snow and other zooplankton, repackaging carbon via their faecal pellets and delivering this to other parts of the water column, including to the seabed. They are fundamental to the transfer of energy, carbon and biomass through the marine ecosystem. They also provide a food source for organisms on higher trophic levels (Rigby & Milsom, Reference Rigby and Milsom2000).
Many groups of organisms that have colonized the water column have benthic antecedents, for example, ostracods (Siveter, Vannier & Palmer, Reference Siveter, Vannier and Palmer1991), foraminifera (Hart, Reference Hart and Hart2000; Hart et al. Reference Hart, Hylton, Oxford, Price, Hudson and Smart2003), hemichordates (Hou et al. Reference Hou, Aldridge, Siveter, Siveter, Williams, Zalasiewcz and Ma2011) and chitinozoans (Shen et al. Reference Shen, Aldridge, Williams, Vandenbroucke and Zhang2013). However, despite numerous groups of extant and fossil zooplankton (for a comprehensive summary see Rigby, Reference Rigby1997; Rigby & Milsom, Reference Rigby and Milsom2000), few studies have attempted to identify the environmental and anatomical feedbacks that facilitate a zooplanktonic lifestyle. Furthermore, although arthropods are a major component of modern marine zooplankton, the fossil record of arthropod zooplankton as a whole is scarce and evidence of this important component of marine ecosystems is largely absent. This is exemplified by copepods, numerically the most abundant component of modern arthropod zooplankton, but which have virtually no fossil record (see summary in Selden et al. Reference Selden, Huys, Stephenson, Heward and Taylor2010). Examples of arthropod zooplankton colonizations are fundamental to understanding the drivers of a zooplanktonic lifestyle and the likely modes of re-colonization of the water column post-dating major extinction events in the marine ecosystem.
Here we examine a component of the early zooplankton within a major group of Cambrian bivalved arthropods, the Bradoriida. Bradoriida were small as adults (typically 0.2–1 cm long) and a common component of shelly marine Cambrian assemblages (Williams et al. Reference Williams, Siveter, Popov and Vannier2007). They are numerically the most important component of the Burgess Shale (e.g. see Siveter & Williams, Reference Siveter and Williams1997) and Chengjiang Lagerstätten (e.g. see Hou et al. Reference Hou, Siveter, Williams and Feng2002) and are presumed important primary consumers in basal positions in many Cambrian ecosystems. Bradoriida were a globally distributed component of seabed and near-seabed marine shelf communities for about 20 million years during Cambrian epochs 2 and 3 (Williams et al. Reference Williams, Siveter, Popov and Vannier2007). Thereafter, their biodiversity apparently collapsed, with only a few taxa persisting into late Cambrian time (Furongian Epoch). This change may be linked to sea-level rise and the spread of anoxic conditions onto continental shelves (Williams et al. Reference Williams, Vannier, Corbari and Massabuau2011). Bradoriids are an ideal group with which to assess the mechanisms of a planktonic colonization in arthropods: there is abundant evidence of their geographical and lithofacies distribution, of their faunal associates through Cambrian space and time (Williams et al. Reference Williams, Siveter, Popov and Vannier2007, Reference Williams, Vannier, Corbari and Massabuau2011), of their soft-part anatomy (Hou et al. Reference Hou, Siveter, Williams, Walossek and Bergström1996, Reference Hou, Williams, Siveter, Siveter, Aldridge and Sansom2010; Shu et al. Reference Shu, Vannier, Luo, Chen, Zhang and Hu1999), and of their physiology (Vannier, Williams & Siveter, Reference Vannier, Williams and Siveter1997; Williams et al. Reference Williams, Vannier, Corbari and Massabuau2011). Geological and palaeobiological evidence supports a zooplanktonic lifestyle for two Anabarochilina bradoriid species (see also Collette, Hughes & Peng, Reference Collette, Hughes and Peng2011), namely Anabarochilina primordialis (Linnarsson) and Anabarochilina australis (Hinz-Schallreuter). We assess the possible biological and environmental drivers of this lifestyle and show how these may relate to other arthropod zooplankton colonizations elsewhere during Phanerozoic time.
2. The distribution of Cambrian Bradoriida
The geographical and temporal distribution of Bradoriida is summarized by Williams et al. (Reference Williams, Siveter, Popov and Vannier2007, Reference Williams, Vannier, Corbari and Massabuau2011). The group occurs in many regions of the world during Cambrian epochs 2 and 3, but is best known from palaeocontinental Laurentia (e.g. Siveter & Williams, Reference Siveter and Williams1997), Baltica (e.g. Diez Álvarez et al. Reference Diez Álvarez, Gozalo, Cederström and Ahlberg2008), Avalonia (Williams & Siveter, Reference Williams and Siveter1998), South China (Hou et al. Reference Hou, Siveter, Williams and Feng2002; Zhang, Reference Zhang2007) and various regions of palaeocontinental Gondwana including Antarctica (e.g. Wrona, Reference Wrona2009), North Africa (e.g. Hinz-Schallreuter, Reference Hinz-Schallreuter1993) and especially Australia (e.g. Jones & Laurie, Reference Jones and Laurie2006; Topper et al. Reference Topper, Skovsted, Brock and Paterson2011). Bradoriid arthropods are interpreted as benthos and demersal swimmers and favoured well-oxygenated seas, as indicated by their lithofacies distribution (Williams et al. Reference Williams, Vannier, Corbari and Massabuau2011) and well-developed integumental circulatory systems (Vannier, Williams & Siveter, Reference Vannier, Williams and Siveter1997). In general, Bradoriida are absent where phosphatocopid arthropods (=labrophoran eucrustacea; see Siveter, Waloszek & Williams, Reference Siveter, Sutton, Briggs and Siveter2003) are common, in the black shale successions of the Avalonian and Baltic regions during the upper part of Cambrian Series 3 and the Furongian (Williams et al. Reference Williams, Vannier, Corbari and Massabuau2011). However, bradoriids and phosphatocopids occur together in the Monastery Creek Phosphorite Formation (Cambrian Stage 5, Series 3) of Australia (Jones & Laurie, Reference Jones and Laurie2006).
At the species level, and to some degree at the genus level, bradoriid arthropods are highly provincial and map out different regions of Cambrian palaeogeography (see Williams et al. Reference Williams, Siveter, Popov and Vannier2007). This suggests that the vast majority of bradoriid species lacked the capacity for intercontinental (trans-oceanic) distribution, and supports their interpretation as benthic organisms (see Schallreuter & Siveter, Reference Schallreuter and Siveter1985 for similar distribution patterns in Ordovician ostracods). This interpretation is also endorsed by rare instances of soft-part anatomy preservation in bradoriids, which suggest that their appendages were useful for locomotion but not specifically adapted for swimming or permanent occupation of the water column (see Hou et al. Reference Hou, Williams, Siveter, Siveter, Aldridge and Sansom2010). While this pattern of bradoriid provinciality persisted for much of Cambrian epochs 2 and 3, species of one bradoriid genus (namely Anabarochilina) have a markedly different palaeogeographical distribution (Fig. 1). Anabarochilina species (Fig. 2) are the most widespread of all bradoriids, with taxa recorded from North America (Siveter & Williams, Reference Siveter and Williams1997), southern Britain (Williams & Siveter, Reference Williams and Siveter1998), Scandinavia (Siveter et al. Reference Siveter, Williams, Abushik, Berg-Madsen and Melnikova1993), Siberia and Kazakhstan (Melnikova, Siveter & Williams, Reference Melnikova, Siveter and Williams1997; Melnikova, Reference Melnikova2003), India (Collette, Hughes & Peng, Reference Collette, Hughes and Peng2011) and Australia (Hinz-Schallreuter, Reference Hinz-Schallreuter1993; Jones & Laurie, Reference Jones and Laurie2006; Collette, Hughes & Peng, Reference Collette, Hughes and Peng2011). Two species in particular are widely distributed, A. australis at low palaeo-latitude, and A. primordialis from low to high palaeo-latitude during Cambrian Epoch 3 (Fig. 1).

Figure 1. Geographical distribution of Anabarochilina species plotted onto a Cambrian base map for 500 Ma (from Torsvik & Cocks, Reference Torsvik, Cocks, Harper and Servais2013). Occurrences are cross-referenced (by number) with the temporal occurrence of Anabarochilina species depicted in Figure 3. For locality references see text. Numbers 1 and 2 are Cambrian Epoch 2 occurrences; 3–10, 12, 13 are Epoch 3; 11 is uncertain (either Epoch 3 or Furongian); and 14 is Furongian. 1, Anabarochilina? sp. A of Williams & Siveter (Reference Williams and Siveter1998), Red Callavia Sandstone, Shropshire, England. 2, Anabarochilina rotundata, Elliptocephala asaphoides fauna, ‘upper Taconic’, New York, USA. 3, Anabarochilina australis, Monastery Creek Phosphorite Formation, Queensland, Australia. 4, Anabarochilina hicksii, Menevian Group of South Wales, and Abbey Shale Formation of Warwickshire, England, UK. 5, Anabarochilina corpulenta, Rushton Brook Bed, Shropshire, UK. 6, Anabarochilina primordialis, Mancetter Shale Formation, Warwickshire, England, UK. 7, Anabarochilina australis, Weeks and Marjum formations, Utah, USA. 8, Anabarochilina primordialis, Alum Shale Formation, Sweden. 9, Anabarochilina primordialis, vicinity of the River Kotui, East Siberia, Russia. 10, Anabarochilina australis, Kyr-Shabakty section, Maly Karatau, Kazakhstan. 11, Anabarochilina konevae, Edrei Beds, Agyrek Mountains, Kazakhstan. 12, Anabarochilina australis, Zanskar Valley, northern India. 13, Anabarochilina chummyensis, Mungerebar Limestone, Australia. 14, Undescribed Anabarochilina species are also known from the Outwoods Shale Formation, Furongian, southern Britain (Williams & Siveter, Reference Williams and Siveter1998).

Figure 2. Morphology of Anabarochilina species from Cambrian epochs 2 and 3, and comparison between the carapace anatomy of Anabarochilina primordialis and the Silurian myodocopid ostracod Parabolbozoe bohemica? (after Perrier, Vannier & Siveter, Reference Perrier, Vannier and Siveter2011). Both Anabarochilina australis (e), and A. primordialis (h, i) show the clear development of an anastomosing integumental circulatory system extending from the area of the adductor muscle attachment (see also Fig. 5). (a, b) Anabarochilina rotundata (Walcott), lateral view, internal mould of right valve and silicon rubber cast of right valve respectively, USNM 17446, 4.76 mm long. (c, d) Anabarochilina hicksii (Jones): (c) internal mould of carapace, right lateral view, NMW 80.34G.1095, 8.3 mm long; (d) lateral view of internal mould of flattened left valve, BGS Zs4582, 6.25 mm long. (e, f) Anabarochilina australis (Hinz-Schallreuter): (e) right valve, lateral view, PIN N4343/55, 8 mm long; (f) KUMIP 1129844, open carapace, 6.5 mm long. (g–j) Anabarochilina primordialis (Linnarsson); (g) internal mould of flattened right valve, BGS BDA2313, 8.3 mm long; (h, i) right valve, lateral view, and close-up of anterior features, SGU 8622, 8.89 mm long; (j) left valve, lateral view, PIN 4342/62, 10 mm long; (k, l) Parabolbozoe bohemica? (Barrande) internal mould of carapace, left lateral view and close up of anterior features, UMC-IP-VP 1, 8.51 mm long. USNM – United States National Museum, Washington DC; NMW – National Museum of Wales, Cardiff; BGS – British Geological Survey, Nottingham; PIN – Palaeontological Museum of the Palaeontological Institute, Russian Academy of Sciences; KUMIP – University of Kansas, Museum of Invertebrate Paleontology; SGU – Sveriges Geologiska Undersokning, Uppsala; UMC-IP-VP – University of Montpellier. (a, b) Washington County, New York, USA; (c) Porth-y-rhaw, South Wales, UK; (d) Nuneaton, Warwickshire, UK; (e) Maly Karatau, Kazakhstan; (f) Millard County, Utah, USA; (h, i) Vastergotland, Sweden; (j) East Siberia, Russia; (k, l) La Combe d’Yzarne, Montage Noire, France. Scale bars are 1 mm.
3. Temporal and biogeographical distribution of Anabarochilina
Species of Anabarochilina are present in strata of Cambrian series 2, 3 and the Furongian (Fig. 3). The biogeographical and temporal distribution of Anabarochilina species is summarized in Figures 1 and 3. Trilobite biozones referred to in the text are summarized in Figure 4.
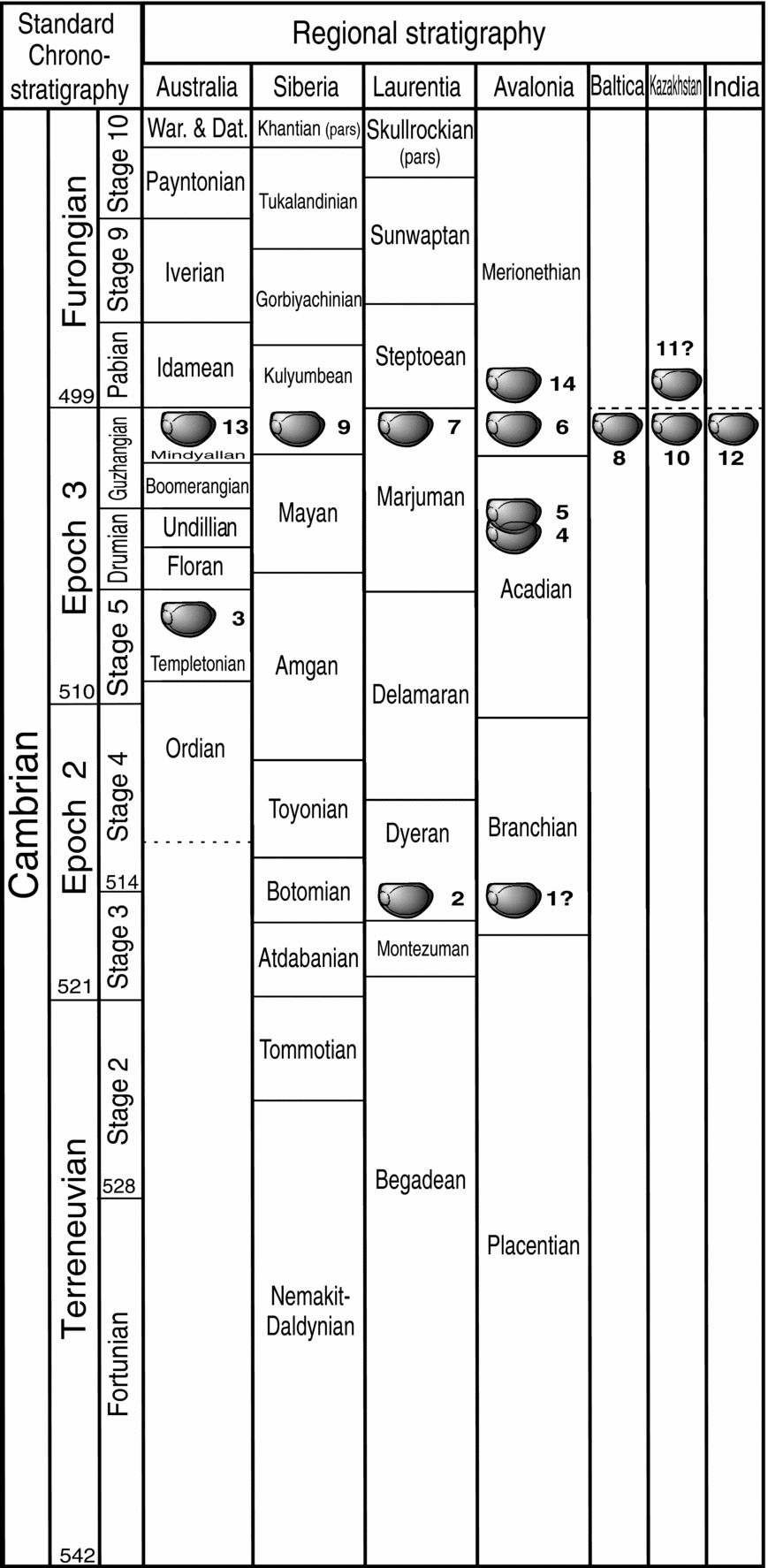
Figure 3. Temporal distribution of Anabarochilina species plotted against the international and selected regional chronostratigraphic subdivisions of the Cambrian (chronostratigraphy from Zhu, Babcock & Peng, Reference Zhu, Babcock and Peng2006). For geographical distribution of these Anabarochilina species and relevance of numbers adjacent icons see Figure 1. Note that ‘8’ represents the occurrence of A. primordialis in the Alum Shale Formation of Sweden, at the same level as the late Guzhangian occurrence of this species in Siberia and Avalonia.

Figure 4. Trilobite biozones referred to in the text and their correlation with the International and British regional stages of the Cambrian, and with available radiometric dates for the Cambrian (based on the detailed summary in Rushton et al. Reference Rushton, Brück, Molyneux, Williams and Woodcock2011, radiometric ages in brackets are inferred). Boundaries shown by dashed lines are of uncertain correlation with the standard.
Anabarochilina species first appear in the rock record of the upper part of Cambrian Stage 3 (Series 2). These early Anabarochilina occur in the low palaeo-latitude Taconic Allochthon succession of Laurentia and possibly in the high palaeo-latitude assemblages of peri-Gondwanan Avalonia (see Figs 1, 3). This material consists of Anabarochilina? sp. A of Williams & Siveter (Reference Williams and Siveter1998) from the Red Callavia Sandstone of Shropshire, UK (Callavia trilobite Biozone; see Williams & Siveter, Reference Williams and Siveter1998), and Anabarochilina rotundata (Walcott) from limestones interbedded with mudstones containing the Elliptocephala asaphoides fauna (level equivalent to the Olenellus trilobite Biozone) of New York State, USA (Siveter & Williams, Reference Siveter and Williams1997).
During Cambrian Epoch 3 the distribution of Anabarochilina species became global (Fig. 1). Anabarochilina australis has its earliest occurrence in strata of Cambrian Stage 5 (Ptychagnostus gibbus trilobite Subzone of the Ptychagnostus paradoxissimus trilobite Biozone, at c. 507 Ma) of Australia. A. australis is notable for a temporal range that may exceed four million years (see Collette, Hughes & Peng, Reference Collette, Hughes and Peng2011 for a summary distribution of this species). Its early occurrence is notable, because this species became pandemic in low palaeo-latitude regions within the Lejopyge laevigata trilobite Biozone of the Guzhangian Stage (c. 503 Ma; Collette, Hughes & Peng, Reference Collette, Hughes and Peng2011). Anabarochilina is represented in Avalonia in the Drumian Stage of Series 3 by Anabarochilina hicksii (Tomagnostus fissus to Hypagnostus parvifrons trilobite subzones of the P. paradoxissimus Biozone, see Fig. 4), being recorded from the Abbey Shale Formation of Warwickshire and from the Menevian Group of South Wales (Williams & Siveter, Reference Williams and Siveter1998; see also Fig. 2). Anabarochilina corpulenta occurs in the Rushton Brook Bed (formerly known as the Paradoxides forchhammeri Grits, see Rushton & Berg-Madsen, Reference Rushton and Berg-Madsen2002), late Drumian of Shropshire, UK (for stratigraphical context see Figs 3, 4).
In the upper part of the P. forchhammeri Biozone (through an interval equivalent to the L. laevigata trilobite Biozone of the Guzhangian Stage) species of Anabarochilina extended their range to become global (Fig. 1), occurring in the palaeocontinental areas of Avalonia, Baltica, Laurentia, Siberia, Kazakhstan and Gondwana (see Williams et al. Reference Williams, Siveter, Popov and Vannier2007; Collette, Hughes & Peng, Reference Collette, Hughes and Peng2011; see also Fig. 3). A. primordialis extended its latitudinal range from high palaeo-latitude Gondwana to palaeo-tropical Siberia (Fig. 1). At the same time, A. australis extended from western Laurentia, across the palaeo-tropics to Gondwana in the east (Fig. 1).
4. Palaeoenvironmental setting of Anabarochilina
Individual species of Anabarochilina traverse environments from platform carbonates to deep-shelf and deep-marine fan environments. Coupled with anatomical evidence from the carapace that suggests an active lifestyle (see Section 5), the lithofacies distribution of certain Anabarochilina species supports the interpretation that they had a zooplanktonic lifestyle.
Early occurrences of Anabarochilina are typically associated with benthic assemblages of trilobites and small shelly fossils. The Red Callavia Sandstone, Lower Comley Group, Shropshire (see Harvey et al. Reference Harvey, Williams, Condon, Wilby, Siveter, Rushton, Leng and Gabbott2011 for a description of these strata) was deposited in a shelf marine setting on the eastern margins of the early Palaeozoic Avalonian Welsh Basin. It contains the bradoriid Anabarochilina? sp. A of Williams & Siveter (Reference Williams and Siveter1998), which is known only from a single specimen that has an anterior node, well-developed continuous marginal rim and smooth and broadly convex valves (Williams & Siveter, Reference Williams and Siveter1998, p. 23). It occurs with the bradoriids Indiana lentiformis (Cobbold) and Liangshanella salopiensis (Cobbold), which are interpreted as a benthic association of species (Williams & Siveter, Reference Williams and Siveter1998), consistent with the overall fauna of the Red Callavia Sandstone. Anabarochilina species are absent from the contemporaneous sedimentary deposits of the open marine shelf Purley Shale Formation of Warwickshire on the Midland Platform (see Rushton et al. Reference Rushton, Brück, Molyneux, Williams and Woodcock2011; Williams et al. Reference Williams, Rushton, Cook, Zalasiewicz, Lewis, Condon and Winrow2013), which however contains the bradoriid Matthoria sp. (see Williams & Siveter, Reference Williams and Siveter1998). Matthoria forms an important faunal link between the southern British succession and the Elliptocephala asaphoides fauna of New York State (Olenellus trilobite Biozone), where Matthoria troyensis (Ford) is recorded from near the city of Troy, New York State (Ford, Reference Ford1873; Siveter & Williams, Reference Siveter and Williams1997). The Elliptocephala asaphoides fauna is a component of the lower Palaeozoic Taconic Allochthon succession of eastern New York State, considered to be an outboard and deep-marine setting along the Iapetus-facing margin of the Laurentia palaeocontinent (e.g. Landing & Bartowski, Reference Landing and Bartowski1996). The Elliptocephala asaphoides fauna has long been considered to contain a biogeographically mixed assemblage of organisms that reflect different environmental settings (e.g. Lochmann, Reference Lochmann1956). Other bradoriids within the ‘upper Taconic’ of the New York Cambrian succession include Anabarochilina rotundata (Walcott) and Indiana dermatoides (Walcott) from Washington County (Walcott, Reference Walcott1887; see Siveter & Williams, Reference Siveter and Williams1997). Collectively, the bradoriids suggest a significant faunal similarity at the genus level with Avalonia. There is no clear suggestion from these early palaeogeographical patterns that Anabarochilina species were zooplanktonic, and their occurrence with other benthic marine organisms (including other bradoriids that have been interpreted as benthic) would be consistent with a lifestyle at or near the seabed.
During Cambrian Epoch 3 the distribution of Anabarochilina species became global, extending westwards through the palaeo-tropics (Collette, Hughes & Peng, Reference Collette, Hughes and Peng2011). The earliest (Cambrian Age 5) occurrence of the widespread and long-lived (c. 4 Ma duration, see Fig. 4) A. australis is in offshore phosphatic limestones of the Monastery Creek Phosphorite Formation, Queensland, Australia (see Jones & Laurie, Reference Jones and Laurie2006), a region of palaeocontinental Gondwana (see Collette, Hughes & Peng, Reference Collette, Hughes and Peng2011). A. australis occurs with a diverse bradoriid fauna and with arthropods interpreted as endemic phosphatocopids (see Jones & Laurie, Reference Jones and Laurie2006). During the Guzhangian, A. australis is found both in platform carbonates and distal fan settings (Collette, Hughes & Peng, Reference Collette, Hughes and Peng2011), while its likely synonym ‘A. burkensis’ is recorded from carbonates (Devoncourt Limestone) in Queensland, Australia (see Jones & Laurie, Reference Jones and Laurie2006).
Anabarochilina hicksii occurs in the Drumian Stage of Series 3 (Figs 3, 4), in the Abbey Shale Formation of Warwickshire and from the Menevian Group of South Wales (Williams & Siveter, Reference Williams and Siveter1998; Fig. 2). The Abbey Shale Formation consists of pyritic mudstones that were probably deposited on a shallow shelf during a period of low seabed oxygenation, punctuated by intervals when the sea bed became colonized by a diversity of benthic trilobites (Rushton, Reference Rushton, Rushton, Owen, Owens and Prigmore1999). The mudstones of the Lower and Middle Menevian Group represent deposition below storm wave base, probably in low but fluctuating oxygen conditions (Prigmore & Rushton, Reference Prigmore, Rushton, Rushton, Owen, Owens and Prigmore1999). Anabarochilina corpulenta occurs in the (upper Drumian) Rushton Brook Bed of Shropshire (Williams & Siveter, Reference Williams and Siveter1998; see Rushton & Berg-Madsen, Reference Rushton and Berg-Madsen2002 for revised correlation of the Paradoxides forchhammeri Grit).
During Guzhangian time, the lithofacies distribution of Anabarochilina species includes deeper shelf mudstones. A. australis occurs in both shelf and more deepwater marine lithofacies. A. primordialis (synonyms: A. ventriangulosa and A. ventriarcuata, see Siveter et al. Reference Siveter, Williams, Abushik, Berg-Madsen and Melnikova1993) occurs in a range of lithofacies that include the Mancetter Shale Formation (formerly the Mancetter Grits and Shales) of Warwickshire (Bridge et al. Reference Bridge, Carney, Lawley and Rushton1998), and also in low palaeo-latitude fine-grained and laminated limestones in Siberia (Melnikova, Siveter & Williams, Reference Melnikova, Siveter and Williams1997; see Fig. 2). Undescribed Anabarochilina species are also present in the succeeding Outwoods Shale Formation of Warwickshire, in strata that comprise black pyritic mudstones with a fauna of putative chemoautotrophic olenid trilobites (Fortey, Reference Fortey2001), possible pelagic agnostid trilobites and phosphatocopid arthropods. In particular, these levels of the Outwoods Shale Formation record seabed conditions that were, at least intermittently, anoxic (see Woods et al. Reference Woods, Wilby, Leng, Rushton and Williams2011). A. primordialis is also preserved in the Alum Shale Formation of Scandinavia (Berg-Madsen, Reference Berg-Madsen1985), a unit which is also associated with probable dysoxic seabed conditions (Ahlberg et al. Reference Ahlberg, Axheimer, Babcock, Eriksson, Schmitz and Terfelt2009): here it occurs with the trilobites Tomagnostella exsculpta and L. laevigata. The late Guzhangian (Mindyallan) Anabarochilina chummyensis is recorded from a carbonate setting in Australia and may yet prove to be conspecific with A. australis. Anabarochilina karatauensis Melnikova was considered conspecific with A. australis by Collette, Hughes & Peng (Reference Collette, Hughes and Peng2011), and occurs in fine-grained bedded limestones in the Kyr-Shabakty section, Maly Karatau, Kazakhstan. This setting represents a marine environment at the edge of a carbonate platform, located on an isolated submarine mount (L. Melnikova, pers. comm., November 2014).
Anabarochilina konevae occurs in a block of limestone within a succession dated as Furongian Epoch (regional Aksayan Stage) of the Agyrek Mountains, Kazakhstan, but is sourced from an olistolith (Williams et al. Reference Williams, Siveter, Popov and Vannier2007) and may represent material reworked from an older horizon.
5. Possible pre-adaptations of Anabarochilina for life in the water column
There is no known soft-part anatomy for species of Anabarochilina, and inferences about functional morphology are based on the carapace morphology. Nevertheless, certain anatomical characteristics of the carapace suggest that Anabarochilina possessed a well-developed integument circulatory system (see Figs 2e, h, i, 5a–c) that suggests an active mode of life and occupation of an oxygenated water column. That Anabarochilina was restricted to environmental settings characterized by oxygenated waters is also suggested by species in shelf successions with typical Cambrian marine benthic faunas (e.g. in the Rushton Brook Bed). An integumental circulatory system was noted in the earliest description of A. rotundata from the Cambrian Taconic Allochthon (Walcott, Reference Walcott1887, pl. 1, fig. 9) and is well developed in A. primordialis, with anastomosing structures extending across the entire lobal area of the carapace and converging on an ovoid lobe immediately posterior of the pronounced mid-anterior lobe (Figs 2e, h, i, 5a–c). This is analogous to structures in myodocopid ostracods and leptostracan phyllocarids where hemolymph enters the anastomosing circulatory network of both valves around the adductor muscles (Fig. 5d, e; Vannier & Abe, Reference Vannier and Abe1995). It is reasonable to suppose that provision of an integumental circulatory system facilitated an active metabolism and aided swimming.

Figure 5. Anabarochilina primordialis (Linnarsson). (a–c) Right valve, lateral view, of specimen SGU 8622, 8.89 mm long (the same specimen as Fig. 2h, i); (b) is shown with elevated contrast to pick out the morphology of the anastomosing structures while (c) is a camera lucida drawing of these structures showing their convergence on the area interpreted as an adductor muscle scar (arrowed). (d, e) Recent myodocope, Azygocypridina sp. (muscle scar arrowed) from New Caledonia, Université Claude-Bernard Lyon-1 collections. As with A. primordialis, this animal has a well-developed integumental circulatory system imprinted on the carapace that converges on the adductor muscle area. All scale bars are 1 mm.
The convergence of the anastomosing structures onto a lobal structure in Anabarochilina and the composition of this lobe from numerous small tubercles (Figs 2e, h, i, 5b–c) suggest that this is the site of adductor muscle attachment in Anabarochilina. The strongly inflated mid-anterior lobe (already present in A. rotundata, Fig. 2a, b) lying immediately anterior of these adductor muscles might, by analogy with the dorsal bulb of Silurian myodocopid ostracods (Siveter, Vannier & Palmer, Reference Siveter, Vannier and Palmer1991; Fig. 2k, l), have accommodated a well-developed basipodite of strong swimming appendages of the second antenna. If this interpretation is correct, Anabarochilina may have had well-developed swimming abilities. However, we note that in the Chengjiang bradoriid Kunmingella douvillei (Hou et al. Reference Hou, Williams, Siveter, Siveter, Aldridge and Sansom2010), all of the post-antennal appendages (barring the trailing terminal appendage pair) have identical biramous morphology (Hou et al. Reference Hou, Williams, Siveter, Siveter, Aldridge and Sansom2010) with no specifically adapted swimming appendages.
The preservation of many benthic bradoriid arthropods in butterfly orientation (e.g. Hou et al. Reference Hou, Siveter, Williams and Feng2002) and the general absence of muscle scars across all the main groups of bradoriids suggests that most (possibly nearly all) of these arthropods lacked the ability to articulate and close their valves tightly. However, A. primordialis and possibly other Anabarochilina species may have possessed adductor muscles and may have had the ability to articulate and possibly close the carapace. This would have provided an advantage to an organism occupying the water column, where predation would be a constant threat.
Eyes are recorded in the Chengjiang bradoriid Kunmingella douvillei (Shu et al. Reference Shu, Vannier, Luo, Chen, Zhang and Hu1999; Hou et al. Reference Hou, Williams, Siveter, Siveter, Aldridge and Sansom2010), and are situated in a dorsal position. In A. primordialis the area dorsal of the mid-anterior lobe is clearly inflated, with a specific ‘node’ at its anterior extremity (Figs 2g–j, 5a–c) that might have accommodated eyes with excellent forward vision. K. douvillei also provides evidence that bradoriids brooded their eggs (Duan et al. Reference Duan, Han, Fu, Zhang, Yang, Komiya and Shu2014), and such a strategy would have been highly beneficial if Anabarochilina species reproduced in the water column.
In summary, Anabarochilina species have an integumental circulatory system that suggests an active metabolism. They may have had well-developed anterior swimming appendages and good vision. Their ability to close the carapace via adductor muscles would have protected swimming appendages and possibly eggs, and would have been useful anatomical adaptations to facilitate a life in the water column.
6. Environmental drivers of a widespread bradoriid zooplankton
Anabarochilina species reveal a distribution pattern with three phases: (1) early (Cambrian Epoch 2) distribution focuses on the Iapetus-facing Taconic Allochthon of Laurentia and possibly in Avalonia, and these Anabarochilina co-occur with essentially benthic assemblages of trilobites and small shelly fossils; (2) early–middle Cambrian Epoch 3 (Age 5 and Drumian) species of Anabarochilina (e.g. A. hicksii) are found in a wider spectrum of lithofacies, some of which suggest dysoxic sea beds but no species is cosmopolitan; and (3) during the Guzhangian Age (late Epoch 3, L. laevigata trilobite Biozone) two species of Anabarochilina became widely distributed, occurring in both inner and outer shelf settings, some of these environments being characterized by dysoxic sea beds. During phase 3, individual species had trans-oceanic (A. australis) and trans-latitude (A. primordialis) distribution. These patterns suggest that post-Drumian Anabarochilina species were zooplanktonic.
The earliest Anabarochilina species may have been distributed between Avalonia and Taconic Laurentia (Fig. 1), suggesting that Anabarochilina species could disperse between continental areas separated by oceans. However, there is no firm evidence that these earliest Anabarochilina were zooplanktonic.
The geographically widespread A. australis occurs in Cambrian Stage 5 limestones of Australia (Jones & Laurie, Reference Jones and Laurie2006), and approximately 4 million years later this taxon extended its range through the palaeo-tropics (Fig. 1). Based on its geographical and lithofacies distribution (Collette, Hughes & Peng, Reference Collette, Hughes and Peng2011) and on the morphology of Anabarochilina species in general, A. australis is considered to be a zooplanktonic arthropod. Given this interpretation, why did A. australis remain exclusively Gondwanan until the Guzhangian Age of Cambrian Epoch 3, approximately 4 million years after its first occurrence? The absence of A. australis from other regions during the interval of mid Epoch 3 might represent a collection bias, as in general specimens of Anabarochilina are rare. However, bradoriids are recorded from successions of this age in other palaeocontinental regions and include Anabarochilina species (e.g. A. hicksii and A. corpulenta in Avalonia; see Williams & Siveter, Reference Williams and Siveter1998).
Both A. hicksii and A. primordialis occur in sedimentary successions that have intermittent levels with pyritic mudstones, suggesting that seabed conditions were sometimes characterized by low oxygen. The spread of black shales onto the shelf during early Guzhangian time is associated with the development of phosphatocopid assemblages in Avalonia and Baltica that were probably adapted for dysoxic seabed conditions (Williams et al. Reference Williams, Vannier, Corbari and Massabuau2011) and which are characteristic of the Alum Shale Formation of Sweden (e.g. Ahlberg et al. Reference Ahlberg, Axheimer, Babcock, Eriksson, Schmitz and Terfelt2009) and the Outwoods Shale Formation of England (e.g. Williams et al. Reference Williams, Siveter, Rushton and Berg-Madsen1994; Williams & Siveter, Reference Williams and Siveter1998). The Drumian–Guzhangian boundary signals the demise of much of the benthic bradoriid fauna that occupied well-oxygenated shelf marine settings (Williams et al. Reference Williams, Vannier, Corbari and Massabuau2011). Anabarochilina hicksii is recorded from Drumian age strata in Avalonia that are assignable to the T. fissus and H. parvifrons subzones of the P. paradoxissimus Biozone (Figs 3, 4). By comparison with the carbon isotope curve of the Alum Shale Formation of Scandinavia (Ahlberg et al. Reference Ahlberg, Axheimer, Babcock, Eriksson, Schmitz and Terfelt2009, fig. 4), this equates to the interval immediately following the peak negative excursion of the Drumian Carbon Isotope Excursion (DICE) event, when carbon isotope values were rising with a positive trend. A. primordialis is characteristic of the (Guzhangian Stage) L. laevigata Biozone. Its first occurrence in the Scandinavian succession occurs at the inflection point of the carbon isotope curve as it begins to rise towards the Steptoean Positive Carbon Isotope Excursion (SPICE) event (Ahlberg et al. Reference Ahlberg, Axheimer, Babcock, Eriksson, Schmitz and Terfelt2009, figs 2, 4): A. primordialis characterizes the same interval in the Avalonian succession (Williams & Siveter, Reference Williams and Siveter1998). The pattern of distribution of Drumian and Guzhangian Anabarochilina species, which includes the dispersal of A. australis to Kazakhstan and Laurentia (Fig. 1), suggests that Anabarochilina species may have been pre-adapted to cope with sea-level rise and the spread of intermittent seabed anoxia onto the shelf, and that this was an important driver of their widespread distribution.
The wide latitudinal range of A. primordialis during the interval of the L. laevigata trilobite Biozone is remarkable. It either suggests an organism with tolerance of a range of surface water temperatures (across nearly 70° of southern latitude), a low thermal gradient from the Cambrian tropics to the poles (not suggested by the apparent restriction of A. australis to the palaeo-tropics) or the possible extension of warm surface water currents to Avalonia. Alternatively, A. primordialis may have occupied deeper-water settings at the level of the thermocline and was therefore not subject to surface temperature gradients.
7. Comparison with other early putative arthropod zooplankton colonizations
The Cambrian (epochs 2 and 3) bivalved arthropod Isoxys had a global distribution in the palaeo-tropics (Williams, Siveter & Peel, Reference Williams, Siveter and Peel1996; Vannier & Chen, Reference Vannier and Chen2000), often occurring in black shale deposits such as those of the Sirius Passet Lagerstätte of Greenland (e.g. Williams, Siveter & Peel, Reference Williams, Siveter and Peel1996). However, Stein et al. (Reference Stein, Peel, Siveter and Williams2010) have noted that no single species of Isoxys has a cosmopolitan distribution; at present, a zooplanktonic mode of life for Isoxys is therefore speculative. Similarly, the Ordovician bivalved arthropod Caryocaris (Vannier et al. Reference Vannier, Racheboeuf, Brussa, Williams, Rushton, Servais and Siveter2003) has been interpreted as a zooplanktonic phyllocarid. It may have occupied the water column during Early Ordovician time (see Vannier et al. Reference Vannier, Racheboeuf, Brussa, Williams, Rushton, Servais and Siveter2003). It occurs in graptolite-bearing black mudstones but like Isoxys no single species has a cosmopolitan distribution, in contrast to contemporaneous macro-zooplanktonic graptolites.
A more direct comparison with zooplanktonic Anabarochilina is afforded by Silurian myodocopid ostracods. The early (Llandovery and Wenlock) myodocopid genus Pauline is recorded from England, Greenland and China (Siveter et al. Reference Siveter, Briggs, Siveter, Sutton and Joomun2013; Perrier et al. Reference Perrier, Siveter, Williams and Lane2014; unpub. data). However, no single species is palaeogeographically widespread. Later during Silurian time, myodocopids show a well-documented plankton colonization following the middle Homerian biotic crisis (Siveter, Vannier & Palmer, Reference Siveter, Vannier and Palmer1991) with transoceanic distribution at the species level (Perrier, Vannier & Siveter, Reference Perrier, Vannier and Siveter2007, Reference Perrier, Vannier and Siveter2011). This colonization event may be associated with sea-level change, the spread of ocean anoxia onto the continental shelf and the restoration of the planktonic ecosystem (including zooplanktonic graptolites) post-dating the middle Homerian extinction (Siveter, Vannier & Palmer, Reference Siveter, Vannier and Palmer1991; Porebska, Kozlowska-Dawidzuik & Masiak, Reference Porebska, Kozlowska-Dawidzuik and Masiak2004; Cramer et al. Reference Cramer, Condon, Söderlund, Marshall, Worton, Thomas, Calner, Ray, Perrier, Boomer, Patchett and Jeppsson2012). Extrinsic environmental drivers may have acted on physiological and anatomical pre-adaptations already present in myodocopids from Llandovery time (or possibly earlier; Siveter et al. Reference Siveter, Tanaka, Farrell, Martin, Siveter and Briggs2014) that included an active metabolism and well-developed swimming appendages, the latter evident in exceptionally preserved ostracods from the Wenlock Herefordshire Lagerstätte of England (e.g. Siveter et al. Reference Siveter, Sutton, Briggs and Siveter2003, Reference Siveter, Siveter, Briggs and Sutton2007, Reference Siveter, Briggs, Siveter and Sutton2010, Reference Siveter, Briggs, Siveter, Sutton and Joomun2013). Parallels with Anabarochilina include biological pre-adaptations acted upon by environmental drivers that include sea-level rise and the spread of anoxia onto the marine shelf. Although the record of arthropod zooplankton is scarce in the fossil record, similarities between the context and nature of Cambrian and Silurian arthropod zooplankton suggest that similar processes may have acted across time, and may have been important following major extinction events for the restoration of an arthropod zooplanktonic component of marine ecosystems.
Acknowledgements
MW, VP and DJS thank The Leverhulme Trust for funding this work (grant RPG-324, Pioneer ostracod zooplankton). TRAV and TS thank the Agence National de la Recherche, France as part of the RALI project (Rise of Animal Life; reference ANR-11-BS56-0025). MW thanks Lille 1 University for a visiting Professorship undertaken during autumn 2012. We are indebted to Adrian Rushton and Tonu Meidla for their insightful comments and suggestions regarding our manuscript. This is a contribution to IGCP 591, “the Early to Mid Paleozoic revolution”. We thank Leonid Popov (Cardiff), Ludmila Melnikova (Moscow) and Ed Landing (Albany) for discussion on the stratigraphic context of Anabarochilina species from Kazakhstan and New York State.
Declaration of interest
None.