Introduction
Mycoplasma bovis (formerly Mycoplasma agalactiae subsp. bovis) was first identified in 1961 from a case of mastitis (Hale et al., Reference Hale, Hemboldt, Plastridge and Stula1962), and was described as a cause of respiratory disease in 1976 (Gourlay et al., Reference Gourlay, Thomas and Howard1976). Since then, M. bovis has emerged as an important cause of pneumonia in feedlot cattle and dairy calves. Other disease manifestations include arthritis and tenosynovitis (Singh et al., Reference Singh, Doig and Ruhnke1971; Gourlay et al., Reference Gourlay, Thomas and Howard1976; Ryan et al., Reference Ryan, Wyand, Hill, Tourtellotte and Yang1983; Adegboye et al., Reference Adegboye, Halbur, Nutsch, Kadlec and Rosenbusch1996; Pfutzner and Sachse (Reference Pfutzner and Sachse1996); Byrne et al., Reference Byrne, Fagan and McCormack2001a; Gagea et al., Reference Gagea, Bateman, Shanahan, van Dreumel, McEwen, Carman, Archambault and Caswell2006a), mastitis (Fox et al., Reference Fox, Kirk and Britten2005), otitis media in dairy calves and unweaned beef calves but uncommonly in feedlot cattle (Walz et al., Reference Walz, Mullaney, Render, Walker, Mosser and Baker1997; Maeda et al., Reference Maeda, Shibahara, Kimura, Wada, Sato, Imada, Ishikawa and Kadota2003; Lamm et al., Reference Lamm, Munson, Thurmond, Barr and George2004; Gagea et al., Reference Gagea, Bateman, Shanahan, van Dreumel, McEwen, Carman, Archambault and Caswell2006a), keratoconjunctivitis (Levisohn et al., Reference Levisohn, Garazi, Gerchman and Brenner2004; Alberti et al., Reference Alberti, Addis, Chessa, Cubeddu, Profiti, Rosati, Ruiu and Pittau2006), decubital abscesses (Kinde et al., Reference Kinde, Daft, Walker, Charlton and Petty1993), metritis, abortion and infertility, seminal vesiculitis (LaFaunce and McEntee, Reference LaFaunce and McEntee1982), and perhaps meningitis (Stipkovits et al., Reference Stipkovits, Rady and Glavits1993).
M. bovis has been the subject of considerable investigation in the past 40 years, yet gaps in knowledge limit our ability to control diseases resulting from infection with this pathogen. The challenges of isolating and manipulating mycoplasmas in vitro, the high prevalence of infection in some groups of healthy cattle, the discrepancies between experimentally induced and natural disease, and the lack of simple correlation between antibody titers and disease resistance are some factors that complicate the study of the role of M. bovis in pneumonia of cattle. Areas of substantial uncertainty include the clinical importance of the genotypic diversity of this pathogen, knowledge of virulence factors and how infection leads to tissue damage, mechanisms of immunity and the potential for development of effective vaccines, the specific role of co-pathogens and environmental factors in development of disease and the overall importance of this agent as a contributor to the bovine respiratory disease complex.
M. bovis is a member of the family Mycoplasmataceae in the class Mollicutes (‘soft skin’) (Waites, Reference Waites, Murray and Baron2007). The Mollicutes are prokaryotes of gram-positive lineage that evolved from Clostridium-like bacteria by gene deletion. The genus Mycoplasma comprises the smallest self-replicating life forms and includes over 100 species. Most mycoplasmas are facultative anaerobes, and all have a tri-layered cell membrane but lack the genetic ability to form a cell wall. The lack of a cell wall results in pleomorphic shapes, confers resistance to antimicrobials of the β-lactam group, and interferes with Gram staining. The small genome size of Mycoplasma species, ranging from 580 to 1380 kb, may explain their dependency on the host or complex media for essential nutrients, and their limited survival in the environment. The genome of M. bovis is 1080 kb with a low G+C ratio of 27.8–32.9 mol% (Hermann, Reference Hermann and Maniloff1992; Nicholas and Ayling, Reference Nicholas and Ayling2003). M. bovis and M. agalactiae are closely related, with 99% homology in 16S rRNA (Mattsson et al., Reference Mattsson, Guss and Johansson1994). M. bovis appears to be of no significance as a human pathogen, as there is only one report of its association with human disease: it was cultured from the sputum of a patient with lobar pneumonia and probable myocarditis, nephritis and hemolytic anemia (Madoff et al., Reference Madoff, Pixley, DelGiudice and Moellering1979).
Laboratory diagnosis
Specimens
Mycoplasmas are extremely sensitive microorganisms, so specimens must be kept refrigerated and delivered to a laboratory within 24 h after collection if immediate transportation to the laboratory is not possible. If shipment or storage times exceed 24 h, the specimen should be placed in transport medium and frozen at −70°C to prevent loss of viability of the mycoplasma. For examination, specimens should be thawed very rapidly in a water bath at 37°C (Murray and Baron, Reference Murray and Baron2003). Samples of choice for the various diseases caused by M. bovis have been reviewed (Nicholas and Ayling, Reference Nicholas and Ayling2003) and include bronchoalveolar lavage fluid, pneumonic lung tissue, synovial tissues or joint fluids, mastitic milk, semen, genital discharge, washings of the prepuce and eye swabs. Serum is the appropriate sample for antibody detection. If swabs are used, Dacron, calcium alginate or polyester swabs are preferred over wooden-shaft cotton swabs which are potentially inhibitory (Murray and Baron, Reference Murray and Baron2003). Swabs should be used thoroughly and vigorously to obtain many cells, as mycoplasmas are cell-associated. Swabs should be submitted in transport medium such as Stuart's and Eaton's media. Culture results from nasal swabs do not correlate well with those from the lower respiratory tract (Thomas et al., Reference Thomas, Dizier, Trolin, Mainil and Linden2002b), suggesting that bronchoalveolar lavage fluid is preferable as an indicator of lung infection.
Isolation
Samples for M. bovis isolation should always be thoroughly mixed before the inoculation of media. Fluids should be centrifuged and the pellet used for inoculation. If contaminants are suspected, samples should be passed through a 0.45 μm filter. To overcome the inhibitory effects of antibodies, antibiotics, bacteria and other inhibitors in the specimen, samples such as bronchoalveolar lavage fluid should be serially diluted in broth, with subculture of each dilution (Murray and Baron, Reference Murray and Baron2003; Arcangioli et al., Reference Arcangioli, Duet, Meyer, Dernburg, Bezille, Poumarat and Le Grand2007).
Mycoplasmas require sterols for growth in media, and these are supplied by the addition of serum. M. bovis can grow easily in various media such as Eaton's, supplemented pleuropneumonia-like organism (PPLO) medium, N-agar or broth, modified Friis medium, modified Hayflick medium and other commercially available mycoplasma culture media (Nicholas and Baker, Reference Nicholas, Baker, Miles and Nicholas1998). Broth media should contain yeast extract, tryptone, 20% horse serum and 500 μg/ml of ampicillin, and are incubated at 37°C for 3–10 days for recovery of M. bovis. The filtration-cloning technique must be used to obtain a pure culture of a field isolate, which involves filtration of the broth culture through a 220–450 nm pore-size membrane filter to remove microcolonies. The filtrate is then serially diluted (10−1 to 10−4) in broth medium and plated on agar, where M. bovis will usually produce visible colonies within 2–4 days. Single colonies are picked up with a Pasteur pipette or a needle for subcloning back into a series of broth cultures. The classical method recommends that the isolates be cloned three times by filtration to obtain a pure culture (Tully, Reference Tully, Tully and Razin1983), but this is sometimes not followed because of the risk of mutations associated with adaptation to in vitro growth.
Broths should be incubated at 37°C under atmospheric conditions but agar plates are incubated with 5–10% CO2 (Murray and Baron, Reference Murray and Baron2003); use of a candle jar may be satisfactory. Each new batch of medium and supplements should be tested with a laboratory control strain such as the M. bovis international reference strain PG45, to ensure that the medium sustains growth and does not contain any inhibitory substances. M. bovis isolation from chronically affected animals is reportedly more difficult due to inhibitory substances such as antibiotics and contaminants (Ayling et al., Reference Ayling, Nicholas, Hogg, Wessels, Scholes, Byrne, Hill, Moriarty and O'Brien2005). A pure culture of the mycoplasma should be obtained before carrying out identification procedures.
Identification
Colonies of M. bovis can be identified at the genus level based on their appearance with magnification, by use of Dienes' stain, and biochemical tests, but species-level identification of the isolates requires testing by ELISA or PCR. The agar plates are viewed under a stereomicroscope at 20–60× magnification. It usually requires 2–5 days for M. bovis to produce visible colonies, but this is very dependent on the quality of the media and growth in less than 20 h is reported as common to many field strains in appropriate media (Tully, Reference Tully, Tully and Razin1983; Nicholas and Ayling, Reference Nicholas and Ayling2003). Colonies of M. bovis often have a typical fried-egg appearance, but M. bovis produces films and spots in some media such as Eaton's medium (Nicholas and Baker, Reference Nicholas, Baker, Miles and Nicholas1998). Colonies can be small (0.1–0.5 mm), dark and lack halos (Stalheim and Proctor, Reference Stalheim and Proctor1976). Air bubbles, water or lipid droplets may be confused with mycoplasmal colonies. Dienes' stain, a commercially available methylene blue stain, is useful for easier visualization of colonies, and also aids in differentiating mycoplasmas from debris and bacterial L-form colonies. Mycoplasmal colonies stain blue with Dienes' stain and retain their color indefinitely, whereas bacterial L-form colonies are reported to decolorize. The denser center of the colonies, which grows down into the agar, will stain dark blue, whereas the less dense peripheral zone of surface growth stains light blue (Waites et al., Reference Waites, Crabb, Duffy and Cassell1997; Francoz et al., Reference Francoz, Fortin, Fecteau and Messier2005).
Mycoplasmas form coccoid cells of about 0.2–0.3 μm in diameter, which cannot be clearly seen by routine light microscopy. Lack of a cell wall prevents staining with the Gram stain, and although they do stain with Giemsa, interpretation is difficult because of their small size (Murray and Baron, Reference Murray and Baron2003). Acridine orange staining has proved to be useful in the recognition and enumeration of mycoplasma cells (Rosendal and Valdivieso-Garcia, Reference Rosendal and Valdivieso-Garcia1981; Jasper et al., Reference Jasper, Rosendal and Barnum1984) but this stain is not specific to mycoplasmas.
Biochemical tests such as glucose fermentation, arginine hydrolysis, phosphatase activity, urease activity, film production and reduction of tetrazolium can be used to differentiate M. bovis from other mycoplasmas or ureaplasmas (Poveda, Reference Poveda1998). Biochemical characteristics of M. bovis and other mycoplasmas have been reviewed (Nicholas and Ayling, Reference Nicholas and Ayling2003). Biochemical tests are of little value to differentiate M. bovis and M. agalactiae: neither species ferment glucose or hydrolyse arginine, and both use organic acids such as lactate and pyruvate, give an orange color to the broth with an absence of turbidity (Abu-Amero et al., Reference Abu-Amero, Abu-Groun, Halablab and Miles2000; Khan et al., Reference Khan, Loria, Ramirez, Nicholas, Miles and Fielder2005a), oxidize ethanol (Abu-Amero et al., Reference Abu-Amero, Abu-Groun, Halablab and Miles2000), and produce film and spot reactions in media as a result of lipolytic activity. Molecular tests are able to distinguish these two bovine pathogens (Subramaniam et al., Reference Subramaniam, Bergonier, Poumarat, Capaul, Schlatter, Nicolet and Frey1998; Thomas et al., Reference Thomas, Dizier, Linden, Mainil, Frey and Vilei2004a; Bashiruddin et al., Reference Bashiruddin, Frey, Königsson, Johansson, Hotzel, Diller, Santis, Botelho, Ayling, Nicholas, Thiaucourt and Sachse2005).
Antibody-based tests using hyperimmune rabbit serum have been used to identify M. bovis, including growth inhibition, film inhibition, fluorescent antibody and metabolic inhibition tests (Poveda and Nicholas, Reference Poveda and Nicholas1998). These techniques are relatively slow and labor-intensive because the antisera are not commercially available and must be produced in the laboratory. A sandwich ELISA using monoclonal antibodies (Ball et al., Reference Ball, Finlay and Reilly1994) is frequently used to confirm M. bovis identity and is available commercially (Bio X, Luxembourg). Mycoplasmas can also be identified by dot immunobinding on membrane filtration (MF-dot) using polyclonal antisera prepared against the reference strain PG45 (Poumarat et al., Reference Poumarat, Perrin and Longchambon1991; Arcangioli et al., Reference Arcangioli, Duet, Meyer, Dernburg, Bezille, Poumarat and Le Grand2007). Monoclonal antibody-based ELISA (Boothby et al., Reference Boothby, Mueller, Jasper and Thomas1986) and antigen-capture ELISA (Heller et al., Reference Heller, Berthold, Pfutzner, Leirer and Sachse1993) have been developed to detect M. bovis from milk samples. Other assays to identify M. bovis include immunoperoxidase labeling (Nielsen et al., Reference Nielsen, Stewart, Garcia and Eaglesome1987; Gourlay et al., Reference Gourlay, Thomas and Wyld1989), immunobinding assays (Infante Martinez et al., Reference Infante, Jasper, Stott, Cullor and Dellinger1990; Infante et al., Reference Infante, Infante and Flores-Gutierrez2002; Flores-Gutierrez et al., Reference Flores-Gutierrez, Infante, Salinas-Melendez, Thomas, Estrada-Bellmann and Briones-Encinia2004) and flow cytometry (Assuncao et al., Reference Assuncao, Rosales, Rifatbegovic, Antunes, de la Fe, Ruiz de Galarreta and Poveda2006). The sensitivity of many immunological techniques can be improved with an enrichment step (Boothby et al., Reference Boothby, Mueller, Jasper and Thomas1986; Infante Martinez et al., Reference Infante, Jasper, Stott, Cullor and Dellinger1990; Heller et al., Reference Heller, Berthold, Pfutzner, Leirer and Sachse1993). Conventional diagnostic methods for the detection of M. bovis have been compared previously (Sachse et al., Reference Sachse, Pfutzner, Hotzel, Demuth, Heller and Berthold1993b) and molecular tests for the identification of M. bovis are described below. Immunohistochemistry is an excellent method for detection of M. bovis antigen in formalin-fixed tissues and the distribution of antigen detected by this technique is discussed below (Haines and Chelack, Reference Haines and Chelack1991; Adegboye et al., Reference Adegboye, Rasberry, Halbur, Andrews and Rosenbusch1995a, Reference Adegboye, Hallbur, Cavanaugh, Werdin, Chase, Miskimins and Rosenbuschb; Rodriguez et al., Reference Rodriguez, Kennedy, Bryson, Fernandez, Rodriguez and Ball1996b, Reference Rodriguez, Sarradell, Poveda, Ball and Fernandez2000; Shahriar et al., Reference Shahriar, Clark, Janzen, West and Wobeser2002; Haines et al., Reference Haines, Moline, Sargent, Campbell, Myers and Doig2004; Khodakaram-Tafti and Lopez, Reference Khodakaram-Tafti and Lopez2004; Gagea et al., Reference Gagea, Bateman, Shanahan, van Dreumel, McEwen, Carman, Archambault and Caswell2006a).
Strain typing
Typing systems are expected to reveal new insights in the epidemiology of M. bovis infection, both within herds and across larger populations. Conventional typing systems such as sodium dodecyl sulfate polyacrylamide gel electrophoresis have not revealed sufficient variability to differentiate between strains, although differences in the level of expression of 64–68, 55 and 26 kDa proteins were detected (Sachse et al., Reference Sachse, Grajetzki, Pfützner and Hass1992; Cerda et al., Reference Cerda, Xavier, Sansalone, de la and Rosenbush2000). Better discrimination is achieved using molecular methods and are currently recommended.
Random amplified polymorphic DNA analysis (RAPD) identified three groups (A–C) within 54 isolates of M. bovis from the United Kingdom (UK), containing 54, 35 and 11% of the isolates. Amplified fragment length polymorphism analysis (AFLP) showed more diversity, with two clusters (A and B) that included 35 banding profiles from 54 UK isolates (McAuliffe et al., Reference McAuliffe, Kokotovic, Ayling and Nicholas2004) and 18 profiles from 42 Danish isolates (Kusiluka et al., Reference Kusiluka, Kokotovic, Ojeniyi, Friis and Ahrens2000a). About 80–90% of isolates were assigned to the same group using AFLP and RAPD. For both techniques, the most prevalent group included the type strain PG45, and group A isolates were more diverse than those in group B. Ten isolates from the same herd had identical RAPD results, suggesting transmission between calves or a common source of infection. The banding patterns were considered reproducible, despite variation in the intensity of bands (McAuliffe et al., Reference McAuliffe, Kokotovic, Ayling and Nicholas2004). RAPD requires little in the way of specialized equipment, but gel analysis software is needed and careful technique is required to avoid poor reproducibility. In contrast, AFLP has high discriminatory power and better reproducibility, and may be more well suited to analysis of large numbers of samples in different laboratories.
Pulsed-field gel electrophoresis (PFGE) using SmaI revealed 23 different profiles from 43 isolates from the UK. The congruency of PFGE with RAPD and AFLP varied by genotype: the concordance between PGFE and RAPD/AFLP was 77–85% for group A isolates but less for group B isolates, although identical PGFE results were obtained for all 10 isolates from a single herd (McAuliffe et al., Reference McAuliffe, Kokotovic, Ayling and Nicholas2004). Vsp expression was apparently not related to any of the AFLP, RAPD or PFGE typing patterns, implying that recombination in the Vsp locus does not introduce variability to the molecular typing methods. Compared to RAPD and AFLP, PGFE has good discriminatory power but is time-consuming, laborious and cannot type all isolates (McAuliffe et al., Reference McAuliffe, Kokotovic, Ayling and Nicholas2004).
Arbitrarily primed polymerase chain reaction (AP-PCR) was used to identify seven banding patterns in 50 isolates from three herds. AP-PCR was considered rapid, simple and reproducible, with identical results on isolates obtained from the same animal on different dates (Butler et al., Reference Butler, Pinnow, Thomson, Levisohn and Rosenbusch2001). Analysis of 16S rRNA gene sequences revealed 12 polymorphisms among the eight isolates of M. bovis, which may have some value as epidemiologic markers but are not useful for differentiation of M. bovis and M. agalactiae (Konigsson et al., Reference Konigsson, Bolske and Johansson2002).
These typing data have been used to suggest that group A isolates from the UK may have been recently introduced from Europe at the time of increased importation of cattle following creation of the European Union, whereas group B isolates derived from those present in the UK prior to this time (McAuliffe et al., Reference McAuliffe, Kokotovic, Ayling and Nicholas2004). The absence of any relationship between molecular patterns, geographic origin of the isolates, or the year in which the UK isolates were obtained may reflect movement of cattle between herds (McAuliffe et al., Reference McAuliffe, Kokotovic, Ayling and Nicholas2004). In contrast, AFLP typing data have suggested greater genetic diversity in recent isolates compared to older Danish M. bovis isolates (Kusiluka et al., Reference Kusiluka, Kokotovic, Ojeniyi, Friis and Ahrens2000a). Finally, the AP-PCR studies showed high homology and stability over time among isolates obtained from a closed cow-calf beef herd and a 200-head feedlot, but a greater diversity with five banding patterns among isolates from a ranch receiving 20,000 dairy calves, consistent with the diverse sources of calves in this herd (Butler et al., Reference Butler, Pinnow, Thomson, Levisohn and Rosenbusch2001).
Insertion elements are mobile 0.7–2.5 kb fragments of DNA that transfer between bacteria, and their distribution and copy number give clues to the evolution of pathogenic mycoplasmas. A study suggested an older divergence of M. bovis and M. agalactiae, but more recent transfer of genetic elements from M. mycoides ssp. mycoides, perhaps from concurrent infection of a single animal by these two species (Thomas et al., Reference Thomas, Linden, Mainil, Bischof, Frey and Vilei2005b). A similar approach was used to identify variability between 49 UK isolates of M. bovis (Miles et al., Reference Miles, McAuliffe, Persson, Ayling and Nicholas2005).
Serology
Serology is effective in indicating exposure to M. bovis (Nicholas and Ayling, Reference Nicholas and Ayling2003), and may be more sensitive than culture in chronic cases or those treated with antibiotics, as these factors may interfere with the in vitro growth of M. bovis (Nicholas and Ayling, Reference Nicholas and Ayling2003). M. bovis has both lipid and protein antigens which can elicit antibody responses, and antibody levels remain high for many months (Nicholas and Ayling, Reference Nicholas and Ayling2003). Serology is useful to identify groups of cattle free of infection, but the high seroprevalence in many populations limits its usefulness for routine diagnosis, and antibody titers are not correlated with disease resistance.
Many serological tests have been developed, including indirect hemagglutination, film inhibition and indirect ELISA. Indirect ELISAs using whole cell or treated antigen are the principal methods used for serological testing. Commercial ELISA tests are also available (Biovet, Canada; Bommelli, Switzerland) (Nicholas and Ayling, Reference Nicholas and Ayling2003). The ELISA has been used to assess the seroprevalence of M. bovis infection (Grand et al., Reference Grand, Calavas, Brank, Citti, Rosengarten, Bézille and Poumarat2002) and to select M. bovis-free cattle for creation of disease-free herds (O'Farrell et al., Reference O'Farrell, Dillon, Mee, Crosse, Nolan, Byrne, Reidy, Flynn and Condon2001). Antibodies against M. bovis in milk samples have been detected by ELISA, making it possible to identify individual infected quarters (Byrne et al., Reference Byrne, Ball, Brice, McCormack, Baker, Ayling and Nicholas2000). A blocking ELISA, which used a monoclonal blocking antibody to an unknown antigen and whole-cell lysate of M. bovis as the target antigen, detected serum antibodies to M. bovis with high specificity, good sensitivity, low background and lack of cross-reaction (Ghadersohi et al., Reference Ghadersohi, Fayazi and Hirst2005).
Other ELISAs identify serum antibodies to specific M. bovis proteins, for example those using recombinant VspA as a target antigen (Brank et al., Reference Brank, Grand, Poumarat, Bezille, Rosengarten and Citti1999) or a membrane lipoprotein homologous to the P48 of M. agalactiae (Robino et al., Reference Robino, Alberti, Pittau, Chessa, Miciletta, Nebbia, Grand and Rosati2005). Antisera raised against the P48 protein identified all M. bovis isolates tested, but did not react with six other mycoplasma species of cattle, suggesting that the M. bovis P48 ELISA may be useful as a specific marker of infection (Robino et al., Reference Robino, Alberti, Pittau, Chessa, Miciletta, Nebbia, Grand and Rosati2005).
Molecular tests
Culture and serology have long been the mainstays for diagnosis of Mycoplasma infection. Molecular techniques do not eliminate the need for these traditional techniques, but have the advantage of readily available equipment and expertise, rapid sample processing, integration with testing for other pathogens, cost efficiency, and the potential for improved sensitivity and specificity. Molecular techniques may be particularly appropriate in situations that interfere with mycoplasma isolation, including samples treated with preservatives, contaminated with bacteria or containing antibodies, from animals treated with antibiotics, or from chronic lesions with tissue debris or autolysis (Pinnow et al., Reference Pinnow, Butler, Sachse, Hotzel, Timms and Rosenbusch2001; Cai et al., Reference Cai, Bell-Rogers, Parker and Prescott2005). The differentiation of M. bovis and M. agalactiae is an important goal of PCR assays, because distinguishing these two pathogens using conventional techniques is challenging (Bashiruddin et al., Reference Bashiruddin, Frey, Königsson, Johansson, Hotzel, Diller, Santis, Botelho, Ayling, Nicholas, Thiaucourt and Sachse2005).
Amplification of the 16S rRNA gene by PCR does not adequately distinguish M. bovis and M. agalactiae unless additional methods are used (Subramaniam et al., Reference Subramaniam, Bergonier, Poumarat, Capaul, Schlatter, Nicolet and Frey1998). One such adaptation is the use of real time PCR for amplification of a 16S rRNA gene fragment, with use of hybridization probes or melting temperature analysis of the product to identify and differentiate M. bovis and M. agalactiae in milk or lung tissue, in a sensitive, specific, rapid and cost-effective manner (Cai et al., Reference Cai, Bell-Rogers, Parker and Prescott2005). This RT-PCR is now offered routinely by some laboratories as a same-day diagnostic test for milk samples. Similarly, PCR-based amplification of a 16S rRNA gene fragment can be followed by denaturing gradient gel electrophoresis for rapid differentiation of these two species (McAuliffe et al., Reference McAuliffe, Ellis, Lawes, Ayling and Nicholas2005).
Other gene targets provide direct identification to the species level. PCR-based amplification of the uvrC gene is very useful for identification of M. bovis and differentiation from other mycoplasmas including M. agalactiae (Subramaniam et al., Reference Subramaniam, Bergonier, Poumarat, Capaul, Schlatter, Nicolet and Frey1998; Bíró et al., Reference Bíró, Erdei, Szathmáry and Stipkovits2004), and was recently modified to allow smaller sample and reaction volumes (Thomas et al., Reference Thomas, Dizier, Linden, Mainil, Frey and Vilei2004a). Similarly, PCR assays targeting the ATP-binding protein oppD/F (Hotzel et al., Reference Hotzel, Sachse and Pfutzner1996) or the DNA polymerase III gene (Marenda et al., Reference Marenda, Sagné, Poumarat and Citti2005) were able to identify and differentiate M. agalactiae and M. bovis. Amplification of membrane protein 81 using multiplex PCR is able to identify M. agalactiae and M. bovis, and restriction fragment length analysis differentiates these two species (Foddai et al., Reference Foddai, Idini, Fusco, Rosa, de la Fe, Zinellu, Corona and Tola2005).
Another M. bovis-specific PCR targets an unknown gene sequence (Ghadersohi et al., Reference Ghadersohi, Coelen and Hirst1997), and was adapted to a semi-nested PCR with improved sensitivity and reproducibility on milk and mucosal samples (Hayman and Hirst, Reference Hayman and Hirst2003). A modification of the primers was reported to improve performance of this assay (Tenk et al., Reference Tenk, Bálint, Stipkovits, Bíró and Dencso2006).
A study comparing PCR assays routinely used in five laboratories suggested that those targeting the uvc and oppD/F genes had the highest reliability, and the molecular methods identified four isolates with incorrect species identification by conventional diagnostic methods (Bashiruddin et al., Reference Bashiruddin, Frey, Königsson, Johansson, Hotzel, Diller, Santis, Botelho, Ayling, Nicholas, Thiaucourt and Sachse2005). Antigen capture (Hotzel et al., Reference Hotzel, Heller and Sachse1999) or DNA-binding filter membranes (Hotzel et al., Reference Hotzel, Sachse and Pfutzner1996) have been used to enhance the sensitivity of PCR for detection of M. bovis in milk samples.
Antimicrobial susceptibility testing
Many methods of antimicrobial susceptibility testing used for conventional bacteria have also been used for testing mycoplasmas. Agar dilution has been employed as a reference method (Bebear et al., Reference Bebear, Bove, Bebear and Renaudin1997). However, this technique is not practical to test small numbers of isolates. Agar disk diffusion is not useful for testing mycoplasma species because of the slow growth of these microorganisms and the absence of correlation between zone sizes and minimum inhibitory concentrations (MICs). The microbroth dilution is the most practical and widely used technique since it is economical and permits the testing of several antimicrobial agents in the same microtiter plate. However, this method is labor-intensive because antimicrobial dilutions must be prepared, and the end point tends to shift over time. The ‘Sensititer’ (Trek Diagnostics) version of the microbroth dilution method removes the labor-intensivity of the technique. The E test (AB BIODISK, Solna, Sweden) has the advantage of simplicity, minimal effect of inoculum size, stable end point and adaptativity for testing single isolates. These methods have all been used to determine the antimicrobial susceptibility of M. bovis strains or isolates (Kobayashi et al., Reference Kobayashi, Morozumi, Munthali, Mitani, Ito and Yamamoto1996; Hannan, Reference Hannan2000).
Irrespective of the method, there are no universally accepted guidelines for performing mycoplasma antimicrobial susceptibility tests. In the absence of accepted breakpoint values, susceptibilities to different antimicrobial agents are usually based on the Clinical Laboratory Standards Institute values for common veterinary pathogenic bacteria (CLSI, formerly National Committee for Clinical Laboratory Standards, 2002) or CLSI values for human pathogens (Francoz et al., Reference Francoz, Fortin, Fecteau and Messier2005). In Japan, the MICs are measured according to a standard method (Japanese Society of Chemotherapy, 1981), in which the breakpoints of MICs for tetracyclines, macrolides, aminoglycosides and fluoroquinolones are 1.0, 0.5–2.0, 2.0–4.0 and 1.0–2.0 μg ml−1, respectively (Japanese Society of Chemotherapy, 1994) based on values for human pathogens (Hirose et al., Reference Hirose, Kobayashi, Ito, Kawasaki, Zako, Kotani, Ogawa and Sato2003). In the absence of accepted breakpoint values, published MIC cut-off values for animal mycoplasmas as well as CLSI interpretive criteria can be used as a reference to define in vitro activity (Rosenbusch et al., Reference Rosenbusch, Kinyon, Apley, Funk, Smith and Hoffman2005).
Several methodologies have been used to report MIC values for M. bovis. In the agar dilution method (Kobayashi et al., Reference Kobayashi, Morozumi, Munthali, Mitani, Ito and Yamamoto1996; Hannan, Reference Hannan2000), antimicrobial agents were serially diluted in M-broth and mixed with M-agar; the initial MICs were read as soon as the colonies appeared during incubation, while final MICs were read 3 days later (Hirose et al., Reference Hirose, Kobayashi, Ito, Kawasaki, Zako, Kotani, Ogawa and Sato2003). A broth microdilution method is described (Rosenbusch et al., Reference Rosenbusch, Kinyon, Apley, Funk, Smith and Hoffman2005), using detection methods including redox indicator (ter Laak et al., Reference ter Laak, Noordergraaf and Verschure1993), acid production from pyruvate (Thomas et al., Reference Thomas, Nicolas, Dizier, Mainil and Linden2003a) and growth pellet formation (Ayling et al., Reference Ayling, Baker, Nicholas, Peek and Simon2000). Antimicrobial susceptibility test using alamarBlue is described (Rosenbusch et al., Reference Rosenbusch, Kinyon, Apley, Funk, Smith and Hoffman2005), in which diluted cultures were added to custom prepared ‘Sensititer’ plates, and growth inhibition was detected by changes in the redox status of the resazurin (alamarBlue) indicator dye (Rosenbusch et al., Reference Rosenbusch, Kinyon, Apley, Funk, Smith and Hoffman2005). Finally, in the E test method (Tanner and Wu, Reference Tanner and Wu1992; Hannan, Reference Hannan2000; Francoz et al., Reference Francoz, Fortin, Fecteau and Messier2005), commercially available E test strips impregnated with different antimicrobial agents are placed on agar plates that have been inoculated with a diluted culture of M. bovis. To date, there are no specified procedures to ensure an adequate inoculum size for performing E tests on M. bovis, but inocula of 105 CFU ml−1 have been recommended (Hannan, Reference Hannan2000; Francoz et al., Reference Francoz, Fortin, Fecteau and Messier2005). Growth inhibition, read with a stereomicroscope, is facilitated by staining with Dienes' stain (Waites et al., Reference Waites, Crabb, Duffy and Cassell1997).
Antimicrobial resistance (AMR)
The AMR of M. bovis isolates has been studied in Europe (Wachowski and Kirchhoff, Reference Wachowski and Kirchhoff1986; Poumarat and Martel, Reference Poumarat and Martel1989; ter Laak et al., Reference ter Laak, Noordergraaf and Verschure1993; Ayling et al., Reference Ayling, Baker, Nicholas, Peek and Simon2000; Poumarat et al., Reference Poumarat, Grand, Philippe, Calavas, Schelcher, Cabanié, Tessier and Navetat2001; Vogel et al., Reference Vogel, Nicolet, Martig, Tschudi and Meylan2001; Nicholas and Ayling, Reference Nicholas and Ayling2003), Canada (Francoz et al., Reference Francoz, Fortin, Fecteau and Messier2005), the United States (Rosenbusch, Reference Rosenbusch2000; Rosenbusch et al., Reference Rosenbusch, Kinyon, Apley, Funk, Smith and Hoffman2005), Italy (Mazzolini et al., Reference Mazzolini, Agnoletti and Friso1997) and Japan (Hirose et al., Reference Hirose, Kobayashi, Ito, Kawasaki, Zako, Kotani, Ogawa and Sato2003). Similar AMR profiles were obtained in Europe and the United States, with a high prevalence of resistance (high MIC) to tilmicosin, erythromycin, ampicillin and ceftiofur; intermediate occurrence of resistance to oxy- and chlor-tetracycline, and infrequent or no resistance (low MICs) to enrofloxacin, florfenicol and spectinomycin (Rosenbusch et al., Reference Rosenbusch, Kinyon, Apley, Funk, Smith and Hoffman2005). The main differences observed between isolates were in the high level of oxytetracycline and chlortetracycline resistance in Dutch and British isolates compared to American ones, and the isolation of florfenicol-resistant M. bovis isolates in the British study. In a Canadian study, susceptibility to enrofloxacin was also observed, as was the development of acquired resistance to tetracycline, spectinomycin, azythromycin and clindamycin in M. bovis. (Francoz et al., Reference Francoz, Fortin, Fecteau and Messier2005). In Japan, all M. bovis isolates tested showed a high susceptibility to tiamulin while erythromycin had no activity on any of the isolates. The M. bovis isolates were less susceptible than the type strains to spiramycin, oxytetracycline and tylosin, suggesting that resistance to these antimicrobials may be appearing in field isolates in Japan (Hirose et al., Reference Hirose, Kobayashi, Ito, Kawasaki, Zako, Kotani, Ogawa and Sato2003). MICs from this study were higher than those reported in a previous Japanese study (Katoh et al., Reference Katoh, Koya, Watanabe, Sakai, Ogata and Hikinuma1996).
Pulmonary infection with M. bovis
Virulence factors
Many mycoplasmas appear to be well adapted to survival on mucosal surfaces, where they are highly dependent on their hosts for provision of nutrients including amino acids, nucleotides, fatty acids and sterols. Although a better understanding of nutrient acquisition and biosynthesis by mycoplasmas is emerging, and the mechanisms by which these bacteria adhere to host cells and evade the immune response are becoming better defined, many other aspects of virulence remain unknown. This situation is particularly true for M. bovis, for which evidence of secreted toxins is limited or questionable, and other mechanisms whereby the infection causes injury to host tissues are not clear. This section describes the structural and regulatory aspects of M. bovis virulence factors, while their role in pathogenesis is considered below.
The variable surface lipoproteins are the best characterized virulence factors of M. bovis. They are a family of immunodominant surface antigens that contribute to phenotypic variability of the bacteria, play important roles in bacterial adherence to host cells and evasion of binding by antibody, and provide a fascinating model for understanding the regulation of protein expression in bacteria. In M. bovis, five Vsp proteins have been identified: VspA, VspB, VspC, VspF and VspO (Sachse et al., Reference Sachse, Helbig, Lysnyansky, Grajetzki, Müller, Jacobs and Yogev2000). High frequency variation in the phase (on/off expression) and size of the expressed proteins are characteristic features (Sachse et al., Reference Sachse, Helbig, Lysnyansky, Grajetzki, Müller, Jacobs and Yogev2000). Most or probably all strains of M. bovis express Vsp proteins; those most commonly expressed are VspB, VspO and VspF, while VspA and VspC expression is less frequent. Many combinations of Vsp protein expression are recorded; for example, VspBO, VspB and VspBF were the most common in one study (McAuliffe et al., Reference McAuliffe, Kokotovic, Ayling and Nicholas2004). However, it is important to recognize that this marked heterogeneity in Vsp expression, banding patterns and antigenicity is not a stable feature of the bacterial strain, but instead represents recombination and variable expression of the vsp genes (Behrens et al., Reference Behrens, Heller, Kirchhoff, Yogev and Rosengarten1994; Rosengarten et al., Reference Rosengarten, Behrens, Stetefeld, Heller, Ahrens, Sachse, Yogev and Kirchhoff1994). Indeed, in vitro passage results in altered Vsp expression (Thomas et al., Reference Thomas, Leprince, Dizier, Ball, Gevaert, Damme, Mainil and Linden2005a), and the profile of Vsp protein expression does not correlate with genotype (McAuliffe et al., Reference McAuliffe, Kokotovic, Ayling and Nicholas2004).
The variable surface lipoproteins are encoded by at least 13 distinct vsp genes. The N-terminus is conserved and functions in insertion of the protein into the bacterial membrane. In general, the Vsp proteins contain multiple domains, and many of these domains are formed by repeated sequences of 6–87 amino acid residues (Sachse et al., Reference Sachse, Helbig, Lysnyansky, Grajetzki, Müller, Jacobs and Yogev2000). The number of domains, the length of each repetitive sequence, and the prevalence of proline, glutamic acid and lysine residues are highly variable among the Vsp proteins. These repetitive sequences and their three-dimensional conformations are presumably critical to their immunogenicity, ligand binding and role in cytoadhesion (Sachse et al., Reference Sachse, Helbig, Lysnyansky, Grajetzki, Müller, Jacobs and Yogev2000).
The phenotypic variability in Vsp proteins may be explained by the variation in the repertoire of the vsp genes between isolates and recombination events in the vsp gene locus. Indeed, M. bovis isolates differ in their repertoire of expressed vsp genes (Poumarat et al., Reference Poumarat, Le Grand, Solsona, Rosengarten and Citti1999). In addition, recombination events within the vsp locus involving deletion and addition of repetitive sequences result in size and antigenic variability of the encoded protein, while site-specific DNA inversions mediate the characteristic phase variation and can lead to expression of novel chimeric Vsp proteins (Lysnyansky et al., Reference Lysnyansky, Rosengarten and Yogev1996, Reference Lysnyansky, Ron, Sachse and Yogev2001a, Reference Lysnyansky, Ron and Yogevb; Nussbaum et al., Reference Nussbaum, Lysnyansky, Sachse, Levisohn and Yogev2002; Flitman-Tene et al., Reference Flitman-Tene, Mudahi-Orenstein, Levisohn and Yogev2003). Further, the presence of antibody to specific Vsp proteins may either influence expression of Vsp proteins or more likely select for bacteria with specific patterns of Vsp expression (Le Grand et al., Reference Le Grand, Solsona, Rosengarten and Poumarat1996; Lysnyansky et al., Reference Lysnyansky, Ron and Yogev2001b). The various roles of the Vsp proteins in the development of disease, described in more detail below, include evasion of host antibody response (Le Grand et al., Reference Le Grand, Solsona, Rosengarten and Poumarat1996), colonization of mucosal surfaces and adhesion to host cells (Sachse et al., Reference Sachse, Helbig, Lysnyansky, Grajetzki, Müller, Jacobs and Yogev2000; Lysnyansky et al., Reference Lysnyansky, Ron, Sachse and Yogev2001a, b; Thomas et al., Reference Thomas, Sachse, Farnir, Dizier, Mainil and Linden2003b), and suppression of lymphocyte blastogenesis and cytokine secretion (Vanden Bush and Rosenbusch, Reference Vanden Bush and Rosenbusch2004).
Other surface proteins, unrelated to the Vsps, have also been shown to mediate adhesion to host cells. The pMB67 is an immunogenic protein recognized by antibodies that are produced during infection. Like the Vsps, it also undergoes a high rate of phase and size variation in vitro (Behrens et al., Reference Behrens, Poumarat, Grand, Heller and Rosengarten1996). The P26 antigen is a 32 kDa hydrophilic protein shown to be an important adhesin based on a competitive adherence assay. Neuraminidase treatment revealed the importance of sialyl moieties in adhesive interactions (Sachse et al., Reference Sachse, Grajetzki, Rosengarten, Hanel, Heller and Pfutzner1996). A protein of 28 kDa was reported as a factor involved in M. bovis adhesion to host cells (Sachse et al., Reference Sachse, Grajetzki, Rosengarten, Hanel, Heller and Pfutzner1996). A protein of 40 kDa was reported to be an adhesin in M. agalactiae but is a pseudogene in M. bovis (Thomas et al., Reference Thomas, Linden, Mainil, Dizier, Baseman, Kannan, Fleury, Frey and Vilei2004b). The role of these proteins in cytoadhesion and pathogenesis is discussed below.
Other virulence attributes, apart from those mediating adhesion, have received much less attention. These include a polysaccharide toxin, other polysaccharides, hydrogen peroxide, heat shock proteins and formation of biofilm. There is a single report of toxin production by M. bovis, a heat-stable, 73 kDa, complex polysaccharide which stimulated an inflammatory response following introduction into the mammary gland (Geary et al., Reference Geary, Tourtellotte and Cameron1981). A later study was not able to replicate this finding so the presence and importance of toxin production by M. bovis remains unconfirmed (Thomas et al., Reference Thomas, Howard, Stott and Parsons1986). M. bovis contains other polysaccharides, and although these are of potential diagnostic use, their role in pathogenesis is unknown (Brooks et al., Reference Brooks, Lutze-Wallace, Lu and Robertson2004).
Hydrogen peroxide is produced by some mycoplasmas and is thought to play a role in disease by reacting with iron to produce hydroxyl radicals that cause lipid peroxidation and oxidative damage to cell membranes. Hydrogen peroxide production is reported to be variable between strains of M. bovis and its production seems to be reduced by in vitro passage (Khan et al., Reference Khan, Miles and Nicholas2005b).
Heat shock responses occur in mycoplasmas, and the hsp60 genes of M. bovis, M. agalactiae, Mycoplasma arthritidis and Mycoplasma hyopneumoniae were recently identified. Sequence analysis revealed high homology between these genes. Investigations with western immunoblotting using recombinant Hsp60 fusion proteins and convalescent sera indicated that mycoplasmal Hsp60 is immunogenic in natural infection (Scherm et al., Reference Scherm, Gerlach and Runge2002), although there is no evidence that this response is protective or the proteins affect virulence.
Biofilms are usually associated with resistance to heat and desiccation as well as antimicrobial resistance. Biofilm formation has been identified in mycoplasmas, although they lack the genes commonly associated with biofilm formation in other bacterial species. Analysis of mycoplasma biofilms demonstrated that they form a highly differentiated structure with stacks and channels. For M. bovis, biofilm formation is reported to be strain-dependent and associated with certain Vsp profiles. For example, strains that expressed VspF showed poor biofilm formation while those expressing VspO or VspB formed abundant biofilms. Oxytetracycline inhibited biofilm formation in planktonic M. bovis cells. In an environmental setting, M. bovis was shown to survive over 30 h in a biofilm (McAuliffe et al., Reference McAuliffe, Ellis, Miles, Ayling and Nicholas2006).
Survival, transmission and incubation period
Most mycoplasmas are host-specific commensals on mucosal surfaces, including the respiratory, intestinal and genital tracts and the bovine mammary gland. Survival in the environment is limited and probably of low significance to transmission. However, M. bovis survives in the environment for several days if protected from sunlight, remains viable at 4°C in sponges or milk for 2 months and for over 2 weeks in water, but is more sensitive to higher temperatures, with survival of 1–2 weeks at 20°C and 1 week at 37°C (Pfutzner, Reference Pfutzner1984). Survival on dry paper disks was 126 days at 4°C, 28 days at 30°C and 7 days outdoors (Nagatomo et al., Reference Nagatomo, Takegahara, Sonoda, Yamaguchi, Uemura, Hagiwara and Sueyoshi2001). Survival in various materials are reported as follows: manure, 37 days; cotton, 18 days; straw, 13 days; wood and stainless steel, 1–2 days (Ruffo et al., Reference Ruffo, Nani and Podesta1969). Heat treatment of 65°C for 2 min or 70°C for 1 min resulted in loss of viability (Butler et al., Reference Butler, Sickles, Johanns and Rosenbusch2000). M. bovis survived in liquid media for 59–185 days and the survival was not affected by temperatures ranging from 4 to 37°C (Nagatomo et al., Reference Nagatomo, Takegahara, Sonoda, Yamaguchi, Uemura, Hagiwara and Sueyoshi2001).
M. bovis infection is acquired by ingestion of infected milk and by close contact with infected calves (Pfützner, Reference Pfützner1990). Colonization of the nasal cavity is more common in calves from herds with M. bovis mastitis compared to herds free of the disease (Bennett and Jasper, Reference Bennett and Jasper1977). Aspiration of infected milk is thought to be of particular importance in the development of otitis media and pneumonia in young dairy calves. Contact with infected calves is a second major source of infection, and importation of infected calves is a likely method by which farms become infected (Nicholas, Reference Nicholas2004). When naïve calves are mingled with infected calves, nasal shedding of M. bovis has been detected in the naïve calves within 24 h, and is found in most calves by 7 days after contact (Pfützner, Reference Pfützner1990). As discussed below, there is evidence that stress, transport, or handling is associated with increased shedding of M. bovis in nasal secretions, suggesting these as possible risk factors for transmission (Boothby et al., Reference Boothby, Jasper, Zinkl, Thomas and Dellinger1983). Once established, infection of the respiratory tract is often persistent (Boothby et al., Reference Boothby, Jasper, Zinkl, Thomas and Dellinger1983; Allen et al., Reference Allen, Viel, Bateman and Rosendal1992), which presumably provides a source for infection of in-contact calves.
Defining the incubation period in natural cases is complicated, because it is usually uncertain if the onset of clinical respiratory disease is the result of M. bovis or some other unidentified pathogen that was cleared by the time of clinical diagnosis or death, such as viruses or antibiotic-sensitive bacteria. One naturally infected cohort of calves developed respiratory disease 2 weeks after contact with a diseased group of calves (Adegboye et al., Reference Adegboye, Halbur, Nutsch, Kadlec and Rosenbusch1996). In experimental infections, respiratory disease and arthritis are typically recorded at 8–10 days after infection (Stipkovits et al., Reference Stipkovits, Glávits, Ripley, Molnár, Tenk, Szeredi, Bergonier, Berthelot and Frey2000a), although respiratory disease in as little as 2–6 days is suggested (Pfützner, Reference Pfützner1990).
Immune response: experimental infection and natural disease
The immune response to M. bovis infection has been the subject of considerable interest because of the potential for vaccination in disease control. Most studies have focused on experimental challenge, and immune responses in naturally infected calves have not been adequately studied. When seronegative calves were introduced into a group of calves with endemic M. bovis pneumonia, the recently introduced calves remained seronegative for at least 29–35 days, then developed serum antibody titers to M. bovis by 59–63 days (Nagatomo et al., Reference Nagatomo, Shimizu, Higashiyama, Yano, Kuroki and Hamana1996). This finding indicates a relatively slow spread of infection within the herd, delayed development of a serum antibody response to infection, or both. Naturally infected calves have M. bovis-specific IgM and IgG in serum, and low but detectable IgA in serum; whereas nasal secretions and bronchoalveolar lavage fluid contain low levels of M. bovis-specific IgM and IgG and more IgA than in serum (Boothby et al., Reference Boothby, Jasper, Zinkl, Thomas and Dellinger1983). The half-life of antibodies to M. bovis is reported to be 20 days (Tschopp et al., Reference Tschopp, Bonnemain, Nicolet and Burnens2001). Naturally infected calves have minimal cell-mediated immune responses, including delayed type hypersensitivity reaction and suppression of M. bovis-induced proliferation of blood lymphocytes (Boothby et al., Reference Boothby, Jasper, Zinkl, Thomas and Dellinger1983), but cellular immunity has not been studied with more contemporary methods.
Immune responses are more completely characterized in experimental infection. Intratracheal and/or intranasal challenge of seronegative 12-week-old calves resulted in detectable serum IgG1 production by 7 days post-infection (dpi), but this response continued to increase until at least 63 dpi (Vanden Bush and Rosenbusch, Reference Vanden Bush and Rosenbusch2003). Similarly, another study found no antibody response at 5 dpi, based on ELISA, but antibody was detected at 12 dpi (Nicholas et al., Reference Nicholas, Ayling and Stipkovits2002). Following experimental challenge, serum IgM was consistently detected by 7 dpi and maximal at 14 dpi, serum IgG1 was detected in 50% of calves by 1 week and in 100% by 2 weeks, and serum IgG2 was detected in 0% of calves at 1 week and in 80% by 2 weeks after infection (Howard et al., Reference Howard, Parsons and Thomas1986). IgA was the predominant M. bovis-specific isotype in tracheobronchial wash fluid and was detected in 100% by 2 weeks after challenge, while IgG1 levels were not increased at 1 week but were increased at 2 weeks (Howard et al., Reference Howard, Parsons and Thomas1986).
The antigen specificity of the antibody response has been described. The variable surface lipoproteins are immunodominant antigens, and contain immunodominant epitopes of 3–7 amino acids (Behrens et al., Reference Behrens, Heller, Kirchhoff, Yogev and Rosengarten1994; Sachse et al., Reference Sachse, Helbig, Lysnyansky, Grajetzki, Müller, Jacobs and Yogev2000). Other surface proteins that are targets of the antibody response include pMB67, a phase- and size-variable protein distinct from the Vsp family (Behrens et al., Reference Behrens, Poumarat, Grand, Heller and Rosengarten1996), and the surface lipoprotein P48 (Robino et al., Reference Robino, Alberti, Pittau, Chessa, Miciletta, Nebbia, Grand and Rosati2005).
Experimentally infected cattle had activation of CD8+ T cells with a lower response by CD4+ and γδ-T-cells, based on lymphocyte blastogenesis and enhanced expression of the IL-2 receptor-α (CD25) (Vanden Bush and Rosenbusch, Reference Vanden Bush and Rosenbusch2003). IFN-γ-producing cells were present in similar numbers as IL-4-producing cells, and antigen-specific serum antibody responses showed increased amounts of IgG1 with minimal IgG2. These results suggest skewing of the immune response with a Th2 bias (Vanden Bush and Rosenbusch, Reference Vanden Bush and Rosenbusch2003).
The effector mechanisms necessary for immunity to M. bovis infection are poorly understood. Based on the extracellular location of the bacteria in the airways and alveoli and their association with airway epithelial cells, it is assumed that an effective antibody response might block attachment, opsonize the bacteria, or activate complement-mediated killing. M. bovis activated complement in bovine serum by the classical pathway in the presence of either IgG1 or IgG2 from an immunized donor, or in the presence of serum from conventionally reared cattle, and led to dose-dependent killing of M. bovis. IgG1 was more effective at complement-mediated killing than was IgG2. In contrast, M. bovis did not activate complement by the alternate pathway, and immunoglobulin alone did not kill the bacteria (Howard, Reference Howard1981).
Morphology and development of disease
Many studies have reported gross and histopathologic findings in experimentally induced and in natural infections. The literature on this topic suffers from a lack of critical analysis, in particular regarding the causal association of M. bovis infection and disease, and from inconsistent use of the terms coagulation and caseous necrosis. Experimental challenge studies carry the limitation that the lesions are often more mild or of different appearance than those seen in natural cases, perhaps because experimentally infected calves are usually examined within 2 weeks of challenge whereas natural disease is often more chronic, or because other factors are necessary for development of lesions. In contrast, in studies of natural cases there is often uncertainty in the causal relationship between M. bovis infection and the associated lesions because of the complicating factors described below. A critical analysis of the causal role of M. bovis in development of lung lesions will require consideration of both experimental and natural cases.
Cattle may be infected with M. bovis in the absence of detectable clinical signs or lesions. M. bovis can be isolated from the nasal cavity and bronchoalveolar lavage fluid from about half of feedlot cattle at the time of entry into the feedlot, and from nearly all calves at 12 days after arrival (Allen et al., Reference Allen, Viel, Bateman and Rosendal1992). Necropsy studies have identified M. bovis in the lung of 3% of healthy calves (ter Laak et al., Reference ter Laak, Noordergraaf and Boomsluiter1992), 25% of those lungs with no macroscopic lesions (Tenk et al., Reference Tenk, Stipkovits and Hufnagel2004), and from 46% of grossly and histologically normal lungs (Gagea et al., Reference Gagea, Bateman, van Dreumel, McEwen, Carman, Archambault, Shanahan and Caswell2006b). Bronchoalveolar lavage fluid samples infected with mycoplasmas did not differ in their leukocyte populations from fluid samples without detectable mycoplasmal infection (Boothby et al., Reference Boothby, Jasper, Zinkl, Thomas and Dellinger1983). Finally, seroepidemiology studies found no association (Martin et al., Reference Martin, Bateman, Shewen, Rosendal and Bohac1989, Reference Martin, Bateman, Shewen, Rosendal, Bohac and Thorburn1999) or an uncertain association (Martin et al., Reference Martin, Bateman, Shewen, Rosendal, Bohac and Thorburn1990) between seroconversion to M. bovis and the prevalence of respiratory disease. Thus, calves may remain healthy despite being infected with M. bovis, implying that M. bovis may not necessarily be the cause of pneumonia in infected calves, so it is essential to correlate the findings in natural and experimental infections to properly understand the spectrum of lesions caused by this pathogen.
Clinical and pathologic findings in natural infection and disease
The clinical signs of M. bovis pneumonia are usually indistinguishable from other causes of pneumonia in feedlot cattle and dairy calves (Pfützner, Reference Pfützner1990; Pollock et al., Reference Pollock, Campbell, Janzen, West, Colleen and Pollock2000). Non-specific clinical signs of bronchopneumonia include anorexia, depression, lethargy, fever, hyperpnea, respiratory distress and weight loss (Adegboye et al., Reference Adegboye, Halbur, Nutsch, Kadlec and Rosenbusch1996; Stipkovits et al., Reference Stipkovits, Glávits, Ripley, Molnár, Tenk, Szeredi, Bergonier, Berthelot and Frey2000a, Reference Stipkovits, Ripley, Varga and Pálfib). Features that specifically suggest M. bovis pneumonia are lameness, chronicity and failure to respond to antibiotic therapy, but even these signs are not specific for M. bovis: lameness can also be caused by Histophilus somni infection, and chronic non-responsive pneumonia can result from pulmonary abscessation, bronchiectasis and sequestration. Arthritis manifests clinically as lameness and pain in one or more joints, and some but not all cases have externally visible swelling of the affected joints. Stifle, hock, shoulder and elbow joints are most commonly affected in the disease.
Chronicity and failure to respond to antibiotic treatment is a common feature in cases that have postmortem lesions of caseonecrotic bronchopneumonia (Pollock et al., Reference Pollock, Campbell, Janzen, West, Colleen and Pollock2000; Shahriar et al., Reference Shahriar, Clark, Janzen, West and Wobeser2002). However, it is unknown whether other cases of M. bovis pneumonia recover, because M. bovis would not be recognized as the cause of such cases of pneumonia unless arthritis was present. Feedlot calves that have postmortem lesions of caseonecrotic bronchopneumonia attributed to M. bovis infection were first treated at a mean of 14–15 days after arrival, and died at a mean of 44 days after arrival (Pollock et al., Reference Pollock, Campbell, Janzen, West, Colleen and Pollock2000; Gagea et al., Reference Gagea, Bateman, van Dreumel, McEwen, Carman, Archambault, Shanahan and Caswell2006b). This duration of illness was longer than for other forms of bronchopneumonia, reflecting the chronicity of disease. However, the interval between arrival in the feedlot and first treatment was similar to that for other forms of bronchopneumonia, reinforcing the idea that the early stages of M. bovis pneumonia and pneumonic pasteurellosis are usually indistinguishable. Most death losses from chronic pneumonia were the result of euthanasia, and timely euthanasia is an important welfare consideration to prevent unnecessary suffering (Pollock et al., Reference Pollock, Campbell, Janzen, West, Colleen and Pollock2000).
M. bovis infection is associated with four main patterns of lesions: caseonecrotic bronchopneumonia, bronchopneumonia with foci of coagulation necrosis, suppurative bronchopneumonia without necrosis and chronic bronchopneumonia with abscessation. Caseonecrotic bronchopneumonia is a distinctive lesion and there is a strong evidence that M. bovis plays a causal role, as discussed below. The lesions most consistently affect the cranial and middle lung lobes, but severe cases may have involvement of over 80% of the lung tissue, with only a thin band of unaffected lung in the dorsocaudal aspect of the caudal lobes. The affected areas of lung contain nodules of caseous necrosis separated by areas of reddened and collapsed or consolidated lung. The nodules vary from pinpoint to several centimeters in diameter, and are generally circular, white, dry, crumbly and bulging from the pleural or cut surfaces of the lung (Fig. 1 and 2) (Rodriguez et al., Reference Rodriguez, Bryson, Ball and Forster1996a; Shahriar et al., Reference Shahriar, Clark, Janzen, West and Wobeser2002; Khodakaram-Tafti and Lopez, Reference Khodakaram-Tafti and Lopez2004; Gagea et al., Reference Gagea, Bateman, Shanahan, van Dreumel, McEwen, Carman, Archambault and Caswell2006a). These caseous foci may develop into sequestra of necrotic tissue that occupy the entire lobe (Shahriar et al., Reference Shahriar, Clark, Janzen, West and Wobeser2002; Gagea et al., Reference Gagea, Bateman, Shanahan, van Dreumel, McEwen, Carman, Archambault and Caswell2006a), and bronchiectasis may be present (Khodakaram-Tafti and Lopez, Reference Khodakaram-Tafti and Lopez2004). In other calves, presumably when the lesions are co-infected with Arcanobacterium pyogenes or other bacteria, the foci of caseous necrosis develop into abscesses with liquid pus instead of dry friable caseous material. Rare cases have unilateral lesions affecting the middle and caudal lobes, and contagious bovine pleuropneumonia is an important differential diagnosis in such cases with unilateral lesions (Bashiruddin et al., Reference Bashiruddin, De Santis, Varga and Stipkovits2001).
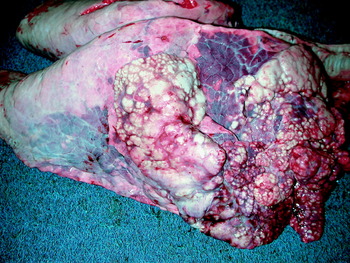
Fig. 1. Gross lesions of M. bovis pneumonia. Multifocal white nodules are present in the cranioventral areas of lung, and the intervening tissue in these areas is atelectatic and reddened. Reprinted from (Gagea et al., Reference Gagea, Bateman, Shanahan, van Dreumel, McEwen, Carman, Archambault and Caswell2006a) with permission of the American Association of Veterinary Laboratory Diagnosticians.

Fig. 2. Gross lesions of M. bovis pneumonia. On section, the lung contains multiple circular raised nodules containing white friable or crumbly caseous material. Reprinted from (Gagea et al., Reference Gagea, Bateman, Shanahan, van Dreumel, McEwen, Carman, Archambault and Caswell2006a) with permission of the AAVLD.
Histologically, the foci of caseous necrosis originate in small bronchioles, alveoli and interlobar septa. In the most mild and probably earliest lesion, leukocytes fill the lumen of bronchioles or alveoli, but undergo a distinctive form of necrosis in which they retain their ghost-like cellular outlines and have hypereosinophilic cytoplasm and inapparent or fragmented nuclei (Fig. 3). In contrast to lesions caused by Mannheimia haemolytica, the streaming chromatin of necrotic leukocytes (‘oat cells’) is not expected. More advanced lesions contain a coagulum of eosinophilic material with ghost-like remnants of necrotic leukocytes at the periphery, erosion of bronchiolar epithelium, and peripheral layers of necrotic cells with pyknotic nuclei, macrophages and lymphocytes and fibrosis (Rodriguez et al., Reference Rodriguez, Bryson, Ball and Forster1996a; Shahriar et al., Reference Shahriar, Clark, Janzen, West and Wobeser2002; Khodakaram-Tafti and Lopez, Reference Khodakaram-Tafti and Lopez2004; Gagea et al., Reference Gagea, Bateman, Shanahan, van Dreumel, McEwen, Carman, Archambault and Caswell2006a). Lymphocytes infiltrating these lesions include CD4+ and CD8+ positive T cells and IgG-producing plasma cells (Howard et al., Reference Howard, Thomas and Parsons1987). Descriptions of this lesion in the literature are confusing because different reports describe the same lesion as coagulation or as caseous necrosis. Although the histologic appearance of ghost-like remnants of necrotic leukocytes within the eosinophilic cellular debris gives credence to the term ‘coagulative’, ‘caseous’ is considered more appropriate because of the gross appearance of dry friable material and the histologically evident loss of alveolar architecture. The situation is further complicated because both lesions may co-exist, in which a central mass of lung tissue that has undergone coagulation necrosis (in which the bronchiolar and alveolar architecture remains visible) is surrounded by caseous eosinophilic cellular debris (Gagea et al., Reference Gagea, Bateman, Shanahan, van Dreumel, McEwen, Carman, Archambault and Caswell2006a).

Fig. 3. Histologic lesions of caseonecrotic bronchopneumonia caused by M. bovis. (A) A focal area of caseous necrosis in the lung tissue, with loss of the alveolar architecture. 40×. (B) The focus of necrosis contains faintly visible outlines of necrotic eosinophilic cells, and is delineated by necrotic neutrophils containing pyknotic nuclei. 200×.
Other pulmonary lesions associated with M. bovis infection include bronchopneumonia containing foci of coagulation necrosis. The caseonecrotic foci described above differ in their gross appearance from these foci of coagulation necrosis, which are irregular in shape, red-tan, non-friable, and not raised, and on histologic examination the architecture of the coagulated alveolar and bronchiolar tissue remains visible. Such lesions are often infected with M. bovis, but are indistinguishable from those caused by M. haemolytica. The possible role of M. bovis in their development is discussed below. Lung abscesses are frequently infected with M. bovis and with other bacteria; the abscesses are filled with liquid pus that differs from the dry friable content of the caseonecrotic foci.
Clinical and pathologic findings resulting from experimental infection
Several reports describe the lesions that result from inoculation of M. bovis into the trachea or bronchi of calves that had undetectable serum antibody to M. bovis and no history of M. bovis infection in the source herds (Gourlay et al., Reference Gourlay, Thomas and Howard1976; Martin et al., Reference Martin, Bochlisch, Pfutzner and Zepezauer1983; Lopez et al., Reference Lopez, Maxie, Ruhnke, Savan and Thomson1986; Thomas et al., Reference Thomas, Howard, Stott and Parsons1986; Rodriguez et al., Reference Rodriguez, Bryson, Ball and Forster1996a). In some, experimentally challenged animals showed no clinical signs (Rodriguez et al., Reference Rodriguez, Bryson, Ball and Forster1996a), while others had fever, lethargy and lameness with increased amount of joint fluid. Inconsistently reported signs included nasal discharge, cough, tachypnea and inappetance (Gourlay et al., Reference Gourlay, Thomas and Howard1976; Rodriguez et al., Reference Rodriguez, Bryson, Ball and Forster1996a). In one study, the clinical signs were transient and lasted only 6–9 days (Gourlay et al., Reference Gourlay, Thomas and Howard1976).
In calves euthanized at 7 or 14 days after challenge, gross lesions were minimal (Lopez et al., Reference Lopez, Maxie, Ruhnke, Savan and Thomson1986), or affected up to 25% of the lung with consolidation and reddening in the cranial and middle lobes, increased prominence of interlobular septa and enlarged lymph nodes (Gourlay et al., Reference Gourlay, Thomas and Howard1976; Lopez et al., Reference Lopez, Maxie, Ruhnke, Savan and Thomson1986; Thomas et al., Reference Thomas, Howard, Stott and Parsons1986; Rodriguez et al., Reference Rodriguez, Bryson, Ball and Forster1996a). Histologic lesions were usually restricted to peribronchiolar aggregates of mononuclear cells, neutrophils filling the lumen of bronchioles, infrequent attenuation of bronchiolar epithelium, and increased numbers of macrophages and few neutrophils in the alveoli (Lopez et al., Reference Lopez, Maxie, Ruhnke, Savan and Thomson1986; Thomas et al., Reference Thomas, Howard, Stott and Parsons1986; Rodriguez et al., Reference Rodriguez, Bryson, Ball and Forster1996a). Necrotic debris in alveoli was occasionally reported (Gourlay et al., Reference Gourlay, Thomas and Howard1976). Although atelectasis was prominent, accumulation of leukocytes in alveoli was not (Thomas et al., Reference Thomas, Howard, Stott and Parsons1986), similar to the situation in natural cases.
It is notable that lesions of caseous necrosis were not described in these studies of less than 2 weeks duration, whereas lesions of multifocal necrosis are described in two more chronic studies. Two gnotobiotic calves euthanized at 35 and 42 days after intratracheal challenge with M. bovis had grossly apparent raised white nodules in the cranial lung. Histologic lesions in these calves included focal coagulative necrosis in which the necrotic alveolar tissue remained visible, as well as foci in which pale amorphous eosinophilic material was surrounded by an inner band of pyknotic cells, a layer of macrophages and plasma cells, and an outer rim of fibroblast proliferation. In contrast to lesions caused by M. haemolytica, the foci of coagulation necrosis resulting from M. bovis challenge were less severe and less frequent, did not cross the interlobular septa, and had few neutrophils, little fibrin, no thrombosis of lymphatics, and lacked the leukocyte necrosis with fusiform streaming of nuclear chromatin (oat cells). M. bovis was isolated from the lung of these two calves, but other pathogens were not (Thomas et al., Reference Thomas, Howard, Stott and Parsons1986). In a second study, naïve calves were challenged by aerosol with M. bovis, and lesions at 18 and 19 days after infection included foci of necrosis consisting of amorphous eosinophilic debris surrounded by a layer of pyknotic cells and an outer layer of macrophages (Stipkovits et al., Reference Stipkovits, Glávits, Ripley, Molnár, Tenk, Szeredi, Bergonier, Berthelot and Frey2000a). Although these foci were described as coagulative, the description is identical to the caseonecrotic lesions described in natural cases (Shahriar et al., Reference Shahriar, Clark, Janzen, West and Wobeser2002; Khodakaram-Tafti and Lopez, Reference Khodakaram-Tafti and Lopez2004; Gagea et al., Reference Gagea, Bateman, Shanahan, van Dreumel, McEwen, Carman, Archambault and Caswell2006a), and probably reflects inconsistent use of the terms coagulation and caseous necrosis in the literature. Although these descriptions are based on only four calves, these lesions in chronically infected calves are noteworthy for their association with M. bovis and their similarity to lesions in natural cases described below.
Pathogenesis and localization of antigen
The distribution of M. bovis antigen has been investigated using immunohistochemistry. In the lesions of caseonecrotic bronchopneumonia, M. bovis antigen is identified throughout the foci of caseous necrosis but most prominently at their periphery, and is both within the cytoplasm of macrophages and free in the necrotic material. Antigen was similarly present in the bronchiolar lumen, within the eosinophilic cellular debris and between and within leukocytes (Thomas et al., Reference Thomas, Howard, Stott and Parsons1986; Rodriguez et al., Reference Rodriguez, Kennedy, Bryson, Fernandez, Rodriguez and Ball1996b; Khodakaram-Tafti and Lopez, Reference Khodakaram-Tafti and Lopez2004; Gagea et al., Reference Gagea, Bateman, Shanahan, van Dreumel, McEwen, Carman, Archambault and Caswell2006a).
In lesions of fibrinosuppurative bronchopneumonia with foci of coagulation necrosis, M. bovis antigen was mainly localized at the periphery of the foci of coagulation necrosis, and also in the cytoplasm of necrotic neutrophils and macrophages, occasionally within non-necrotic neutrophils, and free in edematous alveoli (Khodakaram-Tafti and Lopez, Reference Khodakaram-Tafti and Lopez2004; Gagea et al., Reference Gagea, Bateman, Shanahan, van Dreumel, McEwen, Carman, Archambault and Caswell2006a).
M. bovis antigen has been clearly identified in the cytoplasm of macrophages in natural lesions (Rodriguez et al., Reference Rodriguez, Kennedy, Bryson, Fernandez, Rodriguez and Ball1996b; Khodakaram-Tafti and Lopez, Reference Khodakaram-Tafti and Lopez2004; Gagea et al., Reference Gagea, Bateman, Shanahan, van Dreumel, McEwen, Carman, Archambault and Caswell2006a) and within mononuclear cells in lymphoid follicles (Khodakaram-Tafti and Lopez, Reference Khodakaram-Tafti and Lopez2004). However, it remains unknown whether this intracellular immunolabeling represents M. bovis bacteria that have been phagocytosed and killed by macrophages, or survival of these bacteria in macrophages as a strategy for evasion of the immune response.
M. bovis is able to adhere to and invade between tracheal and bronchial epithelial cells. Antigen was identified, albeit infrequently, among ciliated epithelial cells of the trachea and bronchi in natural and experimental infections (Rodriguez et al., Reference Rodriguez, Kennedy, Bryson, Fernandez, Rodriguez and Ball1996b; Gagea et al., Reference Gagea, Bateman, Shanahan, van Dreumel, McEwen, Carman, Archambault and Caswell2006a). When tracheal explants were infected with M. bovis, bacteria and bacterial antigen were detected on the epithelial cell surface and between epithelial cells, which may be a portal for dissemination via the blood (Thomas et al., Reference Thomas, Howard, Parsons and Anger1987). The association appears to represent specific adhesion of M. bovis to bovine bronchial epithelial cells, and is partially mediated by the variable surface proteins (Sachse et al., Reference Sachse, Helbig, Lysnyansky, Grajetzki, Müller, Jacobs and Yogev2000; Thomas et al., Reference Thomas, Sachse, Farnir, Dizier, Mainil and Linden2003b, 2005a) and protein P26 (Sachse et al., Reference Sachse, Pfutzner, Heller and Hanel1993a, Reference Sachse, Grajetzki, Rosengarten, Hanel, Heller and Pfutzner1996). Adhesion to epithelial cells is temperature-dependent, abrogated by trypsin and by in vitro passage, and may be associated with more highly pathogenic strains of the bacterium (Sachse et al., Reference Sachse, Grajetzki, Rosengarten, Hanel, Heller and Pfutzner1996; Thomas et al., Reference Thomas, Sachse, Farnir, Dizier, Mainil and Linden2003b, Reference Thomas, Leprince, Dizier, Ball, Gevaert, Damme, Mainil and Linden2005a). Attachment of M. bovis to ciliated epithelial cells has no effect on ciliary activity (Thomas et al., Reference Thomas, Howard, Parsons and Anger1987), nor does experimental challenge with M. bovis alter pulmonary clearance of M. haemolytica (Lopez et al., Reference Lopez, Maxie, Savan, Ruhnke, Thomson, Barnum and Geissinger1982).
The issue of whether M. bovis infects airway epithelial cells is controversial. Some studies have identified M. bovis antigen within the cytoplasm of bronchiolar epithelial cells (Adegboye et al., Reference Adegboye, Hallbur, Cavanaugh, Werdin, Chase, Miskimins and Rosenbusch1995b; Rodriguez et al., Reference Rodriguez, Kennedy, Bryson, Fernandez, Rodriguez and Ball1996b), whereas this was specifically addressed but found to be absent (Gagea et al., Reference Gagea, Bateman, Shanahan, van Dreumel, McEwen, Carman, Archambault and Caswell2006a) or sparse (Khodakaram-Tafti and Lopez, Reference Khodakaram-Tafti and Lopez2004) in other investigations. It has been suggested that this invasion across the epithelium occurs within 7 days of infection and may not be detected in more chronic stages (Thomas et al., Reference Thomas, Howard, Stott and Parsons1986). Infection of tracheal explants with M. bovis did not reveal cytoplasmic localization of the bacteria (Thomas et al., Reference Thomas, Howard, Parsons and Anger1987).
Endothelial cells are susceptible to infection with M. bovis in vitro (Lu and Rosenbusch, Reference Lu and Rosenbusch2004), although localization of antigen in vascular walls is not reported in natural or experimental cases so the significance of this infection may be limited. Infected endothelial cells became activated, with induced surface expression of the adhesion molecule VCAM-1 and transcription of several cytokines, but there was minimal or no necrosis of infected endothelial cells (Lu and Rosenbusch, Reference Lu and Rosenbusch2004).
The mechanisms by which M. bovis infection incites inflammation and the characteristic lesion of coagulation necrosis remain unknown. M. bovis induces activation of pulmonary alveolar macrophages and production of tumor necrosis factor-α (Jungi et al., Reference Jungi, Krampe, Sileghem, Groit and Nicolet1996); it is not known whether membrane lipoproteins are responsible for this effect as has been shown for other mycoplasmas (Herbelin et al., Reference Herbelin, Ruuth, Delorme, Michel-Herbelin and Praz1994). A polysaccharide toxin is described and is reported to stimulate an inflammatory response characterized by increased vascular permeability, complement activation and infiltration of eosinophils and neutrophils (Geary et al., Reference Geary, Tourtellotte and Cameron1981). However, others have been unable to replicate these findings or induce a similar response using culture filtrate (Thomas et al., Reference Thomas, Howard, Stott and Parsons1986). Some strains of M. bovis produce hydrogen peroxide, and it is suggested that peroxide may react with iron to produce hydroxyl radicals that cause lipid peroxidation and tissue injury (Khan et al., Reference Khan, Miles and Nicholas2005b), as a possible explanation for the characteristic appearance of necrotic leukocytes within the lesions of caseous necrosis.
Hematogenous dissemination from the lung to other tissues
Bacteremia results from infection of the respiratory tract with M. bovis. Following intranasal and intratracheal challenge, M. bovis can be recovered by culture from blood, up to 9 days after infection but not at later times (Thomas et al., Reference Thomas, Howard, Stott and Parsons1986). However, infection of other tissues persists, and M. bovis can be recovered at 21 days after infection from the spleen, liver and kidney of experimentally challenged calves (Stipkovits et al., Reference Stipkovits, Ripley, Tenk, Glávits, Molnár and Fodor2005). M. bovis antigen has been localized by immunohistochemistry to macrophages in many tissues, hepatocyte cytoplasm, bile ductules, renal tubules and rarely axons in facial nerve (Maeda et al., Reference Maeda, Shibahara, Kimura, Wada, Sato, Imada, Ishikawa and Kadota2003). Thus, bacteremia is a rapid sequel to challenge of the respiratory tract with M. bovis. In vitro findings and immunohistochemical examination of lesions suggests that bacteremia may result from invasion across the airway or alveolar epithelium, or by lymphatic drainage from alveoli through the interlobular septa (Thomas et al., Reference Thomas, Howard, Parsons and Anger1987; Adegboye et al., Reference Adegboye, Hallbur, Cavanaugh, Werdin, Chase, Miskimins and Rosenbusch1995b; Rodriguez et al., Reference Rodriguez, Kennedy, Bryson, Fernandez, Rodriguez and Ball1996b; Gagea et al., Reference Gagea, Bateman, Shanahan, van Dreumel, McEwen, Carman, Archambault and Caswell2006a).
Arthritis is mainly the result of pulmonary infection. M. bovis arthritis in the absence of pneumonia is rare in feedlot cattle (Gagea et al., Reference Gagea, Bateman, Shanahan, van Dreumel, McEwen, Carman, Archambault and Caswell2006a), and clinical signs of pneumonia and arthritis often occur concurrently (Byrne et al., Reference Byrne, Fagan and McCormack2001a). Clinical signs and lesions of arthritis developed in 14–66% of calves following intranasal or intratracheal challenge with M. bovis, and M. bovis was identified by culture or immunohistochemistry in the affected joints (Gourlay et al., Reference Gourlay, Thomas and Howard1976; Thomas et al., Reference Thomas, Howard, Stott and Parsons1986; Stipkovits et al., Reference Stipkovits, Ripley, Tenk, Glávits, Molnár and Fodor2005). These findings imply that infection of the lung with M. bovis leads to hematogenous dissemination to the joints, and subsequent development of arthritis.
Mechanisms of evasion of the immune response
The observation that M. bovis infection of the lung is often chronic in natural cases (Allen et al., Reference Allen, Viel, Bateman and Rosendal1992; Shahriar et al., Reference Shahriar, Clark, Janzen, West and Wobeser2002; Gagea et al., Reference Gagea, Bateman, Shanahan, van Dreumel, McEwen, Carman, Archambault and Caswell2006a) and experimental infection (Thomas et al., Reference Thomas, Howard, Stott and Parsons1986; Stipkovits et al., Reference Stipkovits, Glávits, Ripley, Molnár, Tenk, Szeredi, Bergonier, Berthelot and Frey2000a, Reference Stipkovits, Ripley, Tenk, Glávits, Molnár and Fodor2005) implies that the immune response is ineffective in eliminating infection. The effects on innate immunity in the lung have not been adequately investigated. Although M. bovis antigen is often identified within alveolar macrophages, this seems to induce macrophage activation and cytokine secretion, and not suppression of the macrophage functions studied to date (Jungi et al., Reference Jungi, Krampe, Sileghem, Groit and Nicolet1996). M. bovis adheres to neutrophils and inhibits oxidative burst, chemiluminescence and degranulation in these cells (Finch and Howard, Reference Finch, Howard, Stanek, Cassell, Tully and Whitcomb1990; Thomas et al., Reference Thomas, Van Ess, Wolfgram, Riebe, Sharp and Schultz1991). Although impairment of neutrophil function might predispose to other bacterial infections, this probably has little effect on perpetuating the mycoplasmal infection. The association of M. bovis with ciliated epithelial cells has not been shown to affect ciliary beating or clearance of bacteria from the lung (Lopez et al., Reference Lopez, Maxie, Savan, Ruhnke, Thomson, Barnum and Geissinger1982; Thomas et al., Reference Thomas, Howard, Parsons and Anger1987). Although other infectious agents are capable of impairing antimicrobial peptide expression in airways (Al-Haddawi et al., Reference Al-Haddawi, Mitchell, Clark, Wood and Caswell2007), it is unknown whether M. bovis has a similar effect.
Variable expression of immunodominant proteins on the bacterial surface is probably a major mechanism by which M. bovis evades the developing humoral immune response. Incubation of M. bovis bacteria with serum that contained antibody to M. bovis resulted in altered expression of Vsp and reduced expression of pMB67 surface antigen. Further, incubation with Vsp-specific monoclonal antibodies reduced expression of the corresponding Vsp, which returned following incubation in media containing no antibody. These findings suggest that Vsp are a major target of the humoral immune response, but high frequency mutation of Vsp as well as antibody-driven alteration in Vsp expression allows the bacterium to evade this antibody response (Le Grand et al., Reference Le Grand, Solsona, Rosengarten and Poumarat1996).
M. bovis is also able to suppress the in vitro proliferative response of lymphocytes to mitogens (Finch and Howard, Reference Finch, Howard, Stanek, Cassell, Tully and Whitcomb1990; Thomas et al., Reference Thomas, Mettler, Sharp, Jensen-Kostenbader and Schultz1990) and induce apoptosis of lymphocytes in vitro (Vanden Bush and Rosenbusch, Reference Vanden and Rosenbusch2002). The inhibition of mitogen-induced lymphocyte function is mediated by a C-terminal fragment of Vsp-L, termed M. bovis lympho-inhibitory peptide (Mb-LIP) (Vanden Bush and Rosenbusch, Reference Vanden Bush and Rosenbusch2004). The impact of lymphocyte dysfunction and apoptosis on the magnitude, timing, or character of the immune response has not been investigated.
Role of co-infection with other pathogens
Most calves with M. bovis-associated pneumonia are also infected with other pathogens, which complicates the interpretation of the role that M. bovis plays in development of these lesions. M. bovis was the sole pathogen isolated from the lung in 34% of cases of fatal pneumonia (Byrne et al., Reference Byrne, McCormack, Brice, Egan, Markey and Ball2001b). In contrast, many cases of pneumonia are infected not only with M. bovis, but also with M. haemolytica, Pasteurella multocida, A. pyogenes, or Mycoplasma arginini, and less frequently with H. somni, bovine herpesvirus-1, bovine respiratory syncytial virus, or parainfluenza-3 virus (Byrne et al., Reference Byrne, McCormack, Brice, Egan, Markey and Ball2001b; Shahriar et al., Reference Shahriar, Clark, Janzen, West and Wobeser2002; Thomas et al., Reference Thomas, Ball, Dizier, Trolin, Bell, Mainil and Linden2002a; Khodakaram-Tafti and Lopez, Reference Khodakaram-Tafti and Lopez2004; Gagea et al., Reference Gagea, Bateman, van Dreumel, McEwen, Carman, Archambault, Shanahan and Caswell2006b; Arcangioli et al., Reference Arcangioli, Duet, Meyer, Dernburg, Bezille, Poumarat and Le Grand2007). Co-infection with bovine diarrhea virus is of particular interest, as it is identified in 30–40% of cases of caseonecrotic bronchopneumonia (Haines et al., Reference Haines, Martin, Clark, Jim and Janzen2001; Gagea et al., Reference Gagea, Bateman, Shanahan, van Dreumel, McEwen, Carman, Archambault and Caswell2006a) and 62% of cases of chronic pneumonia (Shahriar et al., Reference Shahriar, Clark, Janzen, West and Wobeser2002). However, since bovine viral diarrhea virus (BVDV) infection was also identified at a similar prevalence in calves with fibrinosuppurative bronchopneumonia typical of M. haemolytica infection, it was suggested that BVDV may impair innate immune responses in the lung and thereby predispose to bacterial pneumonia or enhance its severity, but not preferentially predispose to M. bovis pneumonia (Gagea et al., Reference Gagea, Bateman, Shanahan, van Dreumel, McEwen, Carman, Archambault and Caswell2006a; Al-Haddawi et al., Reference Al-Haddawi, Mitchell, Clark, Wood and Caswell2007).
Several studies have investigated whether co-infection of M. bovis and other agents results in exacerbation of disease. Infection with both M. bovis and M. haemolytica appears to result in more severe clinical disease and lesions than either infection alone (Houghton and Gourlay, Reference Houghton and Gourlay1983), particularly when M. bovis infection preceded M. haemolytica infection by 1 or 2 days (Gourlay and Houghton, Reference Gourlay and Houghton1985). In contrast, in calves with natural M. bovis infection, challenge with P. multocida induced only mild lesions (Martinez et al., Reference Martinez, Aznar, Vina and Martinez1982). Although one study found higher mortality in calves that were naturally infected with both M. bovis and bovine respiratory syncytical virus (BRSV) compared to BRSV infection alone (Howard et al., Reference Howard, Stott, Thomas, Gourlay and Taylor1990), experimental studies found no exacerbation of illness in co-infected calves (Thomas et al., Reference Thomas, Howard, Stott and Parsons1986).
Caseonecrotic bronchopneumonia is the result of chronic M. bovis infection, and it has been suggested that this lesion may arise from lesions initiated by M. haemolytica (Gagea et al., Reference Gagea, Bateman, Shanahan, van Dreumel, McEwen, Carman, Archambault and Caswell2006a). This hypothesis is based on several observations: the early stages of these two infections are clinically indistinguishable and are first detected at similar times after calves arrive in the feedlot, suggesting that they might begin as the same disease; M. bovis and M. haemolytica often co-infect the areas of coagulation necrosis (Fig. 4); some lesions have a central area of coagulation necrosis encircled by a peripheral area of caseous necrosis, perhaps representing caseation at the edge of an earlier lesion of coagulation necrosis; and the caseonecrotic lesions are more chronic than those of coagulation necrosis, consistent with the former being a complication of the latter (Gagea et al., Reference Gagea, Bateman, Shanahan, van Dreumel, McEwen, Carman, Archambault and Caswell2006a). Arguing against this hypothesis is the observation that more severe lesions develop when experimental M. bovis infection is followed by M. haemolytica challenge than the converse, and fact that caseonecrotic lesions were not described in the only study in which calves were sequentially infected with M. bovis and later M. haemolytica (Gourlay and Houghton, Reference Gourlay and Houghton1985). Thus, it remains unresolved whether these severe caseonecrotic lesions are a direct consequence of M. bovis infection, or whether co-infection or prior infection with M. haemolytica, M. arginini, or other factors contribute to the development of severe disease. Nevertheless, these are important considerations, because they dictate whether disease prevention might best be accomplished by control of virulent strains of M. bovis, or whether a better strategy is to control co-infections, underlying diseases, or other factors that enhance the disease resulting from M. bovis infection.

Fig. 4. Bronchopneumonia and coagulation necrosis associated with M. bovis and Mannheimia haemolytica infection. (A, B) A lesion of bronchopneumonia contains focal coagulation necrosis. The neutrophils at the periphery undergo necrosis with streaming of chromatin (oat cells), typical of that caused by M. haemolytica infection. Haematoxylin and eosin stain. (C, D) M. bovis antigen is present at the periphery of the focus of necrosis, and to a lesser extent within this lesion. Immunohistochemistry for M. bovis, DAB chromogen, haematoxylin counterstain. (E, F) M. haemolytica antigen is present throughout the focus of necrosis. Immunohistochemistry for M. haemolytica, DAB chromogen, haematoxylin counterstain. A, C, E: 100×; B, D, F: 400×.
M. bovis and pneumonia: is the association causal?
As described above, naturally occurring cases of M. bovis infection are associated with four main patterns of lesions: caseonecrotic bronchopneumonia, bronchopneumonia with foci of coagulation necrosis, suppurative bronchopneumonia without necrosis and chronic bronchopneumonia with abscessation. Caseonecrotic bronchopneumonia is a characteristic lesion that is almost always infected with M. bovis (Shahriar et al., Reference Shahriar, Clark, Janzen, West and Wobeser2002; Khodakaram-Tafti and Lopez, Reference Khodakaram-Tafti and Lopez2004; Gagea et al., Reference Gagea, Bateman, Shanahan, van Dreumel, McEwen, Carman, Archambault and Caswell2006a). Experimental studies confirm the causality of this association: the same lesion develops following experimental challenge, and M. bovis but not other respiratory pathogens are isolated from the ensuing lesions (Thomas et al., Reference Thomas, Howard, Stott and Parsons1986; Stipkovits et al., Reference Stipkovits, Glávits, Ripley, Molnár, Tenk, Szeredi, Bergonier, Berthelot and Frey2000a).
Less certain is the role of M. bovis in other forms of bronchopneumonia. A critical interpretation of the causal role of M. bovis in development of pneumonia in feedlot cattle is complicated by several factors outlined in Table 1. Calves with M. bovis infection detected by nasal culture were more likely to have a fever than uninfected calves (Wiggins et al., Reference Wiggins, Woolums, Sanchez, Hurley, Cole, Ensley and Pence2007), and M. bovis was isolated more commonly from the lung of calves with suppurative bronchopneumonia (even in the absence of caseous necrosis) than from healthy lungs or those with viral pneumonia (Gagea et al., Reference Gagea, Bateman, Shanahan, van Dreumel, McEwen, Carman, Archambault and Caswell2006a). Further, experimental challenge with M. bovis results in neutrophil infiltration of alveoli and bronchioles, with caseonecrotic lesions developing in only a minority of infected calves (Thomas et al., Reference Thomas, Howard, Stott and Parsons1986; Rodriguez et al., Reference Rodriguez, Kennedy, Bryson, Fernandez, Rodriguez and Ball1996b; Stipkovits et al., Reference Stipkovits, Glávits, Ripley, Molnár, Tenk, Szeredi, Bergonier, Berthelot and Frey2000a). Thus, it is clear that M. bovis is capable of inducing suppurative or catarrhal bronchopneumonia, and that the lesions may not progress to caseous necrosis.
Table 1. Challenges in interpreting the role of Mycoplasma bovis in individual cases of bronchopneumonia

The question of whether M. bovis is an important cause of pneumonia at the herd level is distinct from whether this pathogen is capable of causing bronchopneumonia. The question is complicated by the lack of measures to specifically prevent the disease, which would otherwise allow an estimate of the attributable fraction – the proportion of pneumonia that would not occur if M. bovis were absent from the herd. However, the following suggest that M. bovis infection does indeed contribute significantly to the prevalence of pneumonia in cattle herds. Calves that seroconverted to M. bovis were more likely to be treated for pneumonia than those that did not seroconvert (Rosendal and Martin, Reference Rosendal and Martin1986; Martin et al., Reference Martin, Bateman, Shewen, Rosendal, Bohac and Thorburn1990), although this association was not repeated in other studies (Martin et al., Reference Martin, Bateman, Shewen, Rosendal and Bohac1989, Reference Martin, Nagy, Armstrong and Rosendal1999). The mortality rate from pneumonia increased from 10 to 36 deaths per year following introduction of M. bovis infection to a single farm, for which laboratory data were also available prior to introduction of this pathogen (Gourlay et al., Reference Gourlay, Thomas and Wyld1989). Finally, the timing of infection is appropriate, as initial M. bovis infection followed by challenge with M. haemolytica results in more severe disease than the reverse order (Gourlay and Houghton, Reference Gourlay and Houghton1985). Thus, the available evidence indicates that M. bovis is a cause of caseonecrotic bronchopneumonia, but that M. bovis infection is also capable of causing and is probably an important contributor to other forms of bronchopneumonia in cattle.
Prevalence and economic impact
Prevalence of M. bovis infection
M. bovis infection has been reported in the United States, Canada and most European countries. Recent reports suggesting an absence of this pathogen from any country are rare, apart from a single study in Finland (Härtel et al., Reference Härtel, Nikunen, Neuvonen, Tanskanen, Kivelä, Aho, Soveri and Saloniemi2004). Northern Ireland and the Republic of Ireland were thought to be free of M. bovis until it was detected in 1993 and 1994, probably from importation of infected cattle associated with relaxation of trade barriers (Reilly et al., Reference Reilly, Ball, Cassidy and Bryson1993; Doherty et al., Reference Doherty, McElroy, Markey, Carter and Ball1994).
Estimates of the prevalence of infection vary widely between reported studies, and may depend on the amount of mixing of calves from different sources, the prevalence of pneumonia of any cause, the animal management system and presence of recent stresses, the samples analyzed, and the sensitivity and specificity of methods used for identification of the pathogen. Prevalences reported in older studies are generally lower than from more recent studies (Springer et al., Reference Springer, Fulton, Hagstad, Nicholson and Garton1982; Schulz and Umlauft, Reference Schulz and Umlauft1987; Schulz et al., Reference Schulz, Blohm, Umlauft and Hajesch1990; Kusiluka et al., Reference Kusiluka, Ojeniyi and Friis2000b), but there are examples of high prevalence in older studies (Muenster et al., Reference Muenster, Ose and Matsuoka1979; Allen et al., Reference Allen, Viel, Bateman and Rosendal1992). One report mentioned that M. bovis was more frequently isolated from bronchoalveolar lavage fluid than from nasopharyngeal swabs (Allen et al., Reference Allen, Viel, Bateman and Rosendal1992).
Many herds have serologic evidence of M. bovis exposure, but the prevalence of seropositive herds varies substantially (from 28 to 90%) between studies and between geographic regions in the same study (Grand et al., Reference Grand, Calavas, Brank, Citti, Rosengarten, Bézille and Poumarat2002; Tenk et al., Reference Tenk, Stipkovits and Hufnagel2004; Ghadersohi et al., Reference Ghadersohi, Fayazi and Hirst2005). The prevalence of seropositivity within herds is also not uniform: many groups of pre-weaned beef calves have <20% prevalence while others have >40% seropositivity (Grand et al., Reference Grand, Calavas, Brank, Citti, Rosengarten, Bézille and Poumarat2002), and the seroprevalence in dairy cows at slaughter was also variable between herds (Tenk et al., Reference Tenk, Stipkovits and Hufnagel2004). Although calves with high serum antibody titers to M. bovis are at increased risk of having pneumonia (relative risk=1.7), many calves with high titers do not have chronic pneumonia (Pollock et al., Reference Pollock, Campbell, Janzen, West, Colleen and Pollock2000).
Most studies have found only 0–7% prevalence of M. bovis infection in the lung of healthy calves that were not recently transported or introduced to feedlots (ter Laak et al., Reference ter Laak, Noordergraaf and Boomsluiter1992; Grand et al., Reference Grand, Calavas, Brank, Citti, Rosengarten, Bézille and Poumarat2002; Thomas et al., Reference Thomas, Ball, Dizier, Trolin, Bell, Mainil and Linden2002a; Hirose et al., Reference Hirose, Kobayashi, Ito, Kawasaki, Zako, Kotani, Ogawa and Sato2003; Rifatbegovic et al., Reference Rifatbegovic, Assunção, Poveda and Pasic2007; Wiggins et al., Reference Wiggins, Woolums, Sanchez, Hurley, Cole, Ensley and Pence2007). When healthy calves of different ages were compared in the same study, differences in prevalence were not found (Vogel et al., Reference Vogel, Nicolet, Martig, Tschudi and Meylan2001).
In contrast, several studies indicate that the prevalence of M. bovis infection is higher in feedlot calves and in calves that have been recently transported or co-mingled, even when these calves have no evidence of pneumonia. The prevalence of infection in healthy beef cattle was 40–60% on arrival in feedlots and increased to nearly 100% by day 12 after arrival (Allen et al., Reference Allen, Viel, Bateman, Rosendal, Shewen and Physick-Sheard1991, Reference Allen, Viel, Bateman and Rosendal1992); and a different study reported that 55% of calves seroconverted to M. bovis during the first 7 weeks in the feedlot (Tschopp et al., Reference Tschopp, Bonnemain, Nicolet and Burnens2001). Similarly, M. bovis was isolated from bronchoalveolar lavage fluid of 79% of healthy veal calves in feedlots, at the onset of respiratory disease outbreaks (Arcangioli et al., Reference Arcangioli, Duet, Meyer, Dernburg, Bezille, Poumarat and Le Grand2007). In contrast, the prevalence of M. bovis in nasal swabs was only 2% in calves sampled within 10 days of arrival in stocker and backgrounding operations (Wiggins et al., Reference Wiggins, Woolums, Sanchez, Hurley, Cole, Ensley and Pence2007). Of calves dying within 2 months of introduction to a feedlot, M. bovis was isolated from 46% of those lungs that had no gross or histologic evidence of inflammation (Gagea et al., Reference Gagea, Bateman, van Dreumel, McEwen, Carman, Archambault, Shanahan and Caswell2006b). Infection of feedlot cattle is persistent: studies involving sequential sampling show that when M. bovis is isolated from bronchoalveolar lavage fluid, subsequent samples from the same calf are likely to also be positive (Allen et al., Reference Allen, Viel, Bateman and Rosendal1992). Thus, pulmonary infection with M. bovis is uncommon in most groups of healthy non-transported calves, but becomes much more prevalent and persists after transport, co-mingling, or introduction to a feedlot.
Possible explanations for the higher prevalence of M. bovis infection in feedlot cattle include increased exposure both to M. bovis and to other pathogens as a result of co-mingling of animals from different farms or different age groups, or stress or altered respiratory defenses as a result of transportation and re-ordering of social groups. Epidemiologic assessment identified the following risk factors that were significantly associated with seroconversion to M. bovis in feedlot cattle: mixing calves of different ages and groups, contact with a seropositive animal and prophylactic antibiotic treatment (Tschopp et al., Reference Tschopp, Bonnemain, Nicolet and Burnens2001). In a group of 30 seronegative calves from a single facility in which M. bovis could not be isolated from nasal swabs prior to transportation, this bacterium was isolated from nasal swabs and bronchoalveolar lavage fluid in 20 and 47% of the calves respectively after transportation to a research facility (Boothby et al., Reference Boothby, Jasper, Zinkl, Thomas and Dellinger1983). This finding suggests that transportation stress or exposure to a new environment may enhance proliferation or spread of M. bovis, independent of assembly of groups from different sources. A recent study found M. bovis infection in a recently purchased group of heifers as well as in a fattening herd, but not in 20 other groups of cattle (Rifatbegovic et al., Reference Rifatbegovic, Assunção, Poveda and Pasic2007), indicating an effect of transportation or mixing of groups of calves independent of the feedlot environment itself. Thus, mixing and transporting animals increases the risk of M. bovis infection, although the precise predisposing factors and mechanisms are unknown. It is notable that similar factors increase the risk for pneumonic pasteurellosis in feedlot cattle.
M. bovis infection is more prevalent in the lungs of calves with pneumonia than in healthy lungs, with estimated prevalences ranging from 20 to 90% (Allen et al., Reference Allen, Viel, Bateman, Rosendal, Shewen and Physick-Sheard1991, Reference Allen, Viel, Bateman and Rosendal1992; Kusiluka et al., Reference Kusiluka, Ojeniyi and Friis2000b; Byrne et al., Reference Byrne, McCormack, Brice, Egan, Markey and Ball2001b; Vogel et al., Reference Vogel, Nicolet, Martig, Tschudi and Meylan2001; Thomas et al., Reference Thomas, Ball, Dizier, Trolin, Bell, Mainil and Linden2002a; Tenk et al., Reference Tenk, Stipkovits and Hufnagel2004; Gagea et al., Reference Gagea, Bateman, van Dreumel, McEwen, Carman, Archambault, Shanahan and Caswell2006b; Godinho et al., Reference Godinho, Sarasola, Renoult, Tilt, Keane, Windsor, Rowan and Sunderland2007). The infection is particularly common in the lungs of cattle with chronic pneumonia (71%) (Haines et al., Reference Haines, Martin, Clark, Jim and Janzen2001) and particularly those with chronic caseonecrotic bronchopneumonia (98%), a lesion that was identified in 54% of calves dying in beef feedlots in the first 2 months after arrival and was considered an important contributor to death in 36% (Gagea et al., Reference Gagea, Bateman, van Dreumel, McEwen, Carman, Archambault, Shanahan and Caswell2006b). The prevalence of chronic pneumonia varies substantially among feedlots and pens, but an overall prevalence of 1.3% is typical (Pollock et al., Reference Pollock, Campbell, Janzen, West, Colleen and Pollock2000).
It has been hypothesized that the recent emergence of M. bovis pneumonia in feedlot cattle might be a consequence of the routine use of metaphylactic antibiotic treatment, and perhaps a tendency for producers to monitor at-risk cattle less intensively if they are treated with antibiotics. Although there is currently little published evidence to support this idea (Tschopp et al., Reference Tschopp, Bonnemain, Nicolet and Burnens2001), it certainly merits further investigation. Alternatively, since M. bovis pneumonia seems to have been under-recognized in the past (Gagea et al., Reference Gagea, Bateman, Shanahan, van Dreumel, McEwen, Carman, Archambault and Caswell2006a), increased awareness of the disease by clinical veterinarians and diagnosticians may also have contributed to its apparent emergence.
Estimated economic impact
The economic costs of M. bovis infection are likely to include reduced weight gain or feed efficiency, pharmaceutical and labor costs for treatment of ill animals, death losses, and a portion of the cost of preventative measures such as conditioning. However, it is not possible to estimate the economic impact of M. bovis with any accuracy, because the proportion of acute pneumonia attributed to this pathogen is not known. In particular, the clinical signs in the early stages of M. bovis pneumonia cannot be distinguished from those in other forms of bacterial pneumonia, unless arthritis is also present, and many cases of pneumonia involve interactions between more than one pathogen. These factors make it impossible to reliably attribute economic losses to a single pathogen, and it is not yet possible to estimate the economic advantage of eliminating M. bovis from a herd. Thus, caution is warranted in interpreting estimates of the economic impact of this disease. In one study, calves that seroconverted to M. bovis experienced a 7.6% reduction in average weight gain during the first 7 weeks in the feedlot, and had about 2 times more antibiotics prescribed than calves that remained seronegative (Tschopp et al., Reference Tschopp, Bonnemain, Nicolet and Burnens2001). Whether M. bovis infection was the cause of this reduced weight gain is unknown, as it is plausible that stress or other predisposing factors may have enhanced infection with and seroconversion to M. bovis. Within these limitations, M. bovis infection has been estimated to cost €144 million per year for the European cattle industry and $32 million per year in the United States (Nicholas and Ayling, Reference Nicholas and Ayling2003), and €25 for calves of milking cattle and €58 per veal calf (Gevaert, Reference Gevaert2006). Finally, the non-economic costs are highly significant: chronic pneumonia is an important welfare issue, and prolonged treatment of affected animals with a variety of antibiotics contributes to development of antimicrobial resistance in other pathogens.
Control of the disease
Treatment of affected cattle
Studies that investigate the treatment of M. bovis pneumonia address three disease conditions: ‘enzootic’ pneumonia in young dairy and veal calves, in which viruses, M. bovis, and other bacteria contribute to the disease complex; acute shipping fever pneumonia of feedlot cattle, in which the role of M. bovis is poorly defined; and chronic pneumonia and arthritis syndrome of feedlot cattle, in which M. bovis plays an important and causal role. In a western Canadian feedlot, 40% of calves with chronic pneumonia and arthritis syndrome were euthanized or died in the chronic hospital pen. The 60% that responded adequately enough to treatment to return to their home pen required on average 4 weeks of treatment and weighed 58 kg less than their cohorts (Pollock et al., Reference Pollock, Campbell, Janzen, West, Colleen and Pollock2000). No reports have described the efficacy of antibiotics compared to placebo for treatment of chronic M. bovis pneumonia of feedlot cattle, although most such calves are treated with a succession of different antibiotics.
This poor response to treatment in cases of chronic pneumonia and arthritis syndrome should not be taken as proof that other forms of M. bovis pneumonia have a similarly grim prognosis. The difficulty in clinical diagnosis is a major problem in designing and evaluating trials that address the treatment efficacy in acute M. bovis pneumonia: the clinical signs of acute M. bovis pneumonia are indistinguishable from those of pneumonic pasteurellosis except for cases in which arthritis is also present, isolation of M. bovis is of limited significance because of its prevalence in clinically normal calves, and the lesions of caseonecrotic bronchopneumonia can only be detected at necropsy and presumably resolve in calves that respond to therapy. Thus, it may be that some cases of acute M. bovis pneumonia respond well to antibiotic treatment, but the etiologic role of M. bovis in these cases cannot be easily determined. Experimental studies have the advantages of a more standardized exposure to M. bovis and improved control of other pathogens compared to field cases, but the experimental disease may not resemble that seen in naturally occurring cases. In the reported efficacy studies, the experimental challenge models induce acute disease that would be indistinguishable from other causes of acute bacterial pneumonia, but do not reliably induce the chronic disease, arthritis, or caseonecrotic bronchopneumonia that typify those cases recognized clinically and pathologically as chronic M. bovis pneumonia.
In an experimental challenge model, spectinomycin had no effect on clinical signs and a small effect on isolation of M. bovis from lung tissue (Poumarat et al., Reference Poumarat, Grand, Philippe, Calavas, Schelcher, Cabanié, Tessier and Navetat2001). Treatment with enrofloxacin had no effect on development of arthritis that was experimentally induced by intra-articular inoculation of M. bovis (Belli et al., Reference Belli, Poumarat, Perrin and Martel1993). Treatment of arthritis with tylosin is reported to be effective if administered early in the course of disease (Henderson and Ball, Reference Henderson and Ball1999), but there is no indication of its effectiveness in field cases.
The use of valnemulin and tulathromycin have been more extensively investigated. One study involved intratracheal M. bovis challenge of 3–9 weeks old dairy calves, followed 4 days later by treatment with tulathromycin or saline. Tulathromycin-treated calves had a significantly lower prevalence of severe disease, lower lung lesion scores, lower rectal temperatures, and greater body weight gains compared to saline-treated calves (Godinho et al., Reference Godinho, Rae, Windsor, Tilt, Rowan and Sunderland2005a). In this study, the experimental challenge resulted in consolidation of the lung, but there was no mention of the caseonecrotic lesions typical of that seen in field cases, nor was arthritis described. These experimental studies are complemented by others showing that both tulathromycin and tilmicosin are effective treatments for naturally occurring acute bovine respiratory disease. Although the disease in these studies was associated with infection by M. bovis, M. haemolytica and other bacteria, it is unknown whether elimination of M. bovis was the reason for treatment efficacy in these cases because these antibiotics are also expected to be effective against bacteria of the family Pasteurellaceae (Godinho et al., Reference Godinho, Wolf, Sherington, Rowan, Sunderland and Evans2005b; Skogerboe et al., Reference Skogerboe, Rooney, Nutsch, Weigel, Gajewski and Kilgore2005).
The efficacy of valnemulin has been evaluated in an experimental challenge model. Seronegative 10–35 days old dairy calves were challenged by aerosol, then valnemulin or enrofloxacin therapy was initiated 10 days later. Treatment with either drug resulted in improvement in clinical signs, appetite, weight gain and reduced number of M. bovis bacteria isolated from the lung (Stipkovits et al., Reference Stipkovits, Ripley, Tenk, Glávits, Molnár and Fodor2005). Although lameness and arthritis were detected and lesions of bronchopneumonia were described, the lung lesions lacked the caseonecrotic foci seen in natural disease. The effectiveness of valnemulin therapy was also reported in a group of dairy calves in which M. bovis and P. multocida were the major bacterial pathogens isolated from calves with enzootic pneumonia. Treatment was associated with reduced prevalence of depression, respiratory signs and arthritis. M. bovis was isolated less frequently in nasal swabs but was identified with similar frequency by PCR, in treated and control calves (Stipkovits et al., Reference Stipkovits, Ripley, Varga and Palfi2001).
Early intervention with antibiotic therapy is critical for successful treatment of pneumonic pasteurellosis. There are no published studies to address the importance of early treatment for M. bovis pneumonia, but it is highly likely that delayed treatment reduces the response to therapy, in part because the caseonecrotic lung lesions are probably poorly penetrated by many antibiotics and by the host leukocyte response.
Prevention
Metaphylactic antibiotic treatment
Metaphylactic treatment with antibiotics reduces morbidity and mortality from respiratory disease in beef feedlots (Vogel et al., Reference Vogel, Laudert, Zimmermann, Guthrie, Mechor and Moore1998; Duff and Galyean, Reference Duff and Galyean2007), although the impact on the prevalence of chronic pneumonia and arthritis is unknown. Similarly, in 3 weeks to 12 months old calves experiencing outbreaks of febrile respiratory disease, treatment of clinically normal in-contact calves with tulathromycin compared to saline was associated with reduced prevalence of disease and reduced number of relapses following treatment. However, the contribution of M. bovis to disease in these calves is unknown, and would be difficult to reliably assess (Godinho et al., Reference Godinho, Wolf, Sherington, Rowan, Sunderland and Evans2005b).
Vaccination
Because of the poor therapeutic response in cases of severe chronic M. bovis pneumonia, and concerns surrounding the preventative use of antibiotics in beef production, vaccination is an attractive method for control of M. bovis pneumonia. This approach has proven problematic because of a lack of knowledge of whether and how calves develop immunity to pulmonary infection with this pathogen. As described above, antibody responses to the immunodominant antigens, the variable surface proteins, are thought to be non-protective because they stimulate a shift in antigen expression by the bacteria. It remains unknown whether this evasion of the immune response can be overcome in naturally infected cattle or in vaccinates, as a result of limitations in the variability of this Vsp system, humoral immune responses to other antigens, or other mechanisms of innate or acquired immunity. Two vaccines for M. bovis, both bacterins, are currently licensed by the United States Department of Agriculture for an aid in prevention of pneumonia in cattle: Pulmo-GuardTM MpB (Boehringer Ingelheim Vetmedica, Inc.) and Myco-BacTM B (Texas Vet Lab, Inc.). A third vaccine, MycomuneTM (Biomune Co.), is licensed for control of mastitis (USDA-APHIS, 2007), and autologous vaccines are also in use (Thomson and White, Reference Thomson and White2006).
Many studies have shown that vaccination is capable of stimulating a measurable antibody response, including development of M. bovis-specific IgM, IgG1, IgG2, and IgA in serum, and IgA in the lung lining fluid (Boothby et al., Reference Boothby, Jasper, Zinkl, Thomas and Dellinger1983; Urbaneck et al., Reference Urbaneck, Liebig, Forbrig and Stache2000; Boehringer Ingelheim Vetmedica, Inc., 2003). In one study, titers were detectable in vaccinates within 16 days and remained for at least 6 months (Nicholas et al., Reference Nicholas, Ayling and Stipkovits2002). However, it is clear that serum and local antibody titers do not necessarily correlate with protection based on the prevalence of titers in cattle soon after arrival in feedlots (Allen et al., Reference Allen, Viel, Bateman, Rosendal, Shewen and Physick-Sheard1991, Reference Allen, Viel, Bateman and Rosendal1992; Tschopp et al., Reference Tschopp, Bonnemain, Nicolet and Burnens2001) and in calves dying of M. bovis pneumonia (Gagea et al., Reference Gagea, Bateman, van Dreumel, McEwen, Carman, Archambault, Shanahan and Caswell2006b).
Vaccines have shown promise in some experimental challenge studies, whereas other reports have suggested an increased level of disease in vaccinates (Nicholas et al., Reference Nicholas, Ayling and Stipkovits2002). Use of a vaccine based on saponin-inactivated M. bovis bacteria was reported to be safe in 3–4 week old dairy calves. Following aerosol challenge with M. bovis, vaccinates had a lower prevalence of M. bovis detected in lung and joints, reduced severity of fever and clinical signs of respiratory disease, and less extensive and severe pulmonary lesions at necropsy (Nicholas et al., Reference Nicholas, Ayling and Stipkovits2002). Lesions were described mainly as interstitial pneumonia and lymphohistiocytic bronchitis with less frequent catarrhal bronchopneumonia, so the relevance of this model to severe field cases may be questionable. Similarly, when calves were vaccinated with formalin-inactivated M. bovis and later challenged by intratracheal inoculation of M. bovis, vaccinates had reduced colonization of the lung at 3 weeks after infection. Although these findings suggest a role for local immune responses in protection against experimental challenge, the relevance of these findings to control of natural disease is unknown (Howard et al., Reference Howard, Gourlay and Taylor1977). One commercially available vaccine is claimed to have resulted in 29–89% reduction in lung lesions following experimental challenge, but detailed data are not available and the results are not published in the peer-reviewed literature (Boehringer Ingelheim Vetmedica, Inc., 2003).
Field studies of vaccines share design challenges with the antibiotic efficacy studies described above, because of the difficulty in establishing the precise role of M. bovis in field cases of pneumonia. An autologous formalin-inactivated vaccine is reported to have reduced both infection and mortality in a herd with ongoing respiratory disease caused by M. bovis and M. haemolytica (Urbaneck et al., Reference Urbaneck, Liebig, Forbrig and Stache2000). A quadrivalent inactivated vaccine containing respiratory syncytial virus, parainfluenza type 3, Mycoplasma dispar and M. bovis conferred an approximately 45% reduction in 4 of 7 groups of calves, and this effect was greater than that of the BRSV vaccine alone (Howard et al., Reference Howard, Stott, Thomas, Gourlay and Taylor1990). In contrast, in a field trial in a herd that suffered up to 25% mortality attributed in part to M. bovis, calves vaccinated on arrival but receiving no antibiotics had higher prevalence of severe disease than non-vaccinated calves that were treated with in-feed antibiotics (Nicholas et al., Reference Nicholas, Ayling, Woodger, Wessells and Houlihan2006). There are no published reports of field studies describing the effectiveness of M. bovis vaccines in preventing pneumonia of beef feedlot cattle.
Other disease control strategies
Conditioning of beef calves prior to their transport to feedlots is an effective method to prevent pneumonic pasteurellosis, and requires that calves be castrated and dehorned at an early age, and weaned and introduced to roughage and concentrated feed at least 30 days before transport to the feedlot. Some conditioning programs also require prior vaccination against respiratory viruses, BVDV, M. haemolytica and/or H. somni (Macartney et al., Reference Macartney, Bateman and Ribble2003; Duff and Galyean, Reference Duff and Galyean2007). Anecdotal evidence suggests that a similar strategy may minimize the prevalence or severity of M. bovis pneumonia, but objective published data to support this approach are lacking. Similarly, prevention of M. bovis pneumonia in dairy calves is often feasible by ensuring adequate ventilation and stocking densities, and segregating calves of different ages or different sources (Nicholas and Ayling, Reference Nicholas and Ayling2003).
Eradication is a theoretically possible control strategy because of limited survival of M. bovis in the environment, but is not currently practical in many herds because of the high prevalence of infection. Several reports describe eradication of M. bovis from dairy herds. Strategies include depopulation followed by repopulation with pregnant or weanling heifers that were from seronegative herd status, isolation on arrival, and on-arrival antibiotic treatment (O'Farrell et al., Reference O'Farrell, Dillon, Mee, Crosse, Nolan, Byrne, Reidy, Flynn and Condon2001); or by identification of infected cows using serology and culture of mammary gland or nasal cavity, followed by segregation then culling of cows that tested positive (Bicknell et al., Reference Bicknell, Gunning, Jackson, Boughton and Wilson1983; Byrne et al., Reference Byrne, Ball, McCormack and Brice1998). Herds were monitored by serologic testing and culture of imported cattle, or by a program of bulk tank milk culture (Bicknell et al., Reference Bicknell, Gunning, Jackson, Boughton and Wilson1983; O'Farrell et al., Reference O'Farrell, Dillon, Mee, Crosse, Nolan, Byrne, Reidy, Flynn and Condon2001).
Conclusion
M. bovis has emerged as an important cause of pneumonia, both in young dairy and veal calves and in feedlot cattle. Recent investigations have yielded important insights into the biology of this pathogen, the host response to infection and the role of M. bovis in disease. However, important questions still remain regarding genotypic diversity, the role of co-pathogens and other risk factors, disease mechanisms and immunity. Addressing these questions will be important in the development of improved strategies to control this disease.
Acknowledgments
We thank Drs Ken Bateman, Fernanda Castillo, Ted Clark, Mihai Gagea, Gord Mitchell and Tony van Dreumel for insightful discussions of this disease. Mycoplasma research by the authors is supported by the Ontario Cattlemen's Association, Canadian Cattlemen's Association and the Natural Sciences and Engineering Research Council of Canada.