INTRODUCTION
Reviews that estimate global or regional coastal metabolism and carbon fluxes due to primary producers (e.g. Smith, Reference Smith1981; Gattuso et al., Reference Gattuso, Frankignoulle and Wollast1998; Middelburg et al., Reference Middelburg, Duarte, Gattuso, del Giorgio and Williams2005) need to use first-hand biomass and productivity data of the different primary producers. However, data on macroalgae biomass are still few and consequently result in biased estimates: in the best case scenarios, Smith (Reference Smith1981) or Gattuso et al. (Reference Gattuso, Frankignoulle and Wollast1998) use an estimate of the surface cover area of macroalgae as an underestimate of the biomass per unit area that is then used in several subsequent reviews (Middelburg et al., Reference Middelburg, Duarte, Gattuso, del Giorgio and Williams2005). In the worst case scenario, Gazeau et al. (Reference Gazeau, Smith, Gentili, Frankignoulle and Gattuso2004) do not take into account primary production from macrophytes (seagrasses and macroalgae) in their regional scale estimates due to the lack of data on the production and surface area covered by macrophytes in Europe. The inadequacy of using percentage cover as a proxy for biomass (Masterson et al., Reference Masterson, Arenas, Thompson and Jenkins2008), and the lack of biomass estimates both compel for first-hand estimates of the dominant macroalgae of a region.
The fucale Ascophyllum nodosum (L.) Le Jolis is characteristic of the mid-intertidal zones of North Atlantic temperate sheltered rocky shores. This canopy-forming alga has a major role in the functioning of the zone. It acts as a foundation species (sensu Bruno & Bertness, Reference Bruno, Bertness, Bertness, Gaines and Hay2001) by regulating the community composition and richness of the associated algal and animal assemblages (Bertness et al., Reference Bertness, Leonard, Levine, Schmidt and Ingraham1999; Jenkins et al., Reference Jenkins, Hawkins and Norton1999a). In addition, it is responsible for the major carbon fluxes of the zone (Golléty et al., Reference Golléty, Migné and Davoult2008). This species is also economically important, since A. nodosum is being harvested in several countries of its distribution for its value as a fertilizer, animal fodder and its good alginic acid extraction yields. Recently, a long-term decline of fucoid canopies including A. nodosum has been reported in several European locations (including Brittany) in response to various climate change-driven factors (Le Roux, Reference Le Roux2005; Airoldi & Beck, Reference Airoldi and Beck2007; Davies et al., Reference Davies, Johnson and Maggs2007). The loss of A. nodosum stands often involves the replacement of the canopy with filter-feeders, thus altering the carbon flows within the ecosystem.
In France, the distribution of this macroalga is concentrated along the coasts of Brittany, which is a major site for A. nodosum harvesting along the European rocky shores (Airoldi & Beck, Reference Airoldi and Beck2007). In this area, the most recent biomass estimates, around 13,000 tons, have been calculated on the basis of the harvested amounts of algae received by the processing industries and are not easily available to the scientific community (Arzel & Véron, Reference Arzel and Véron2005). Yet, no studies have attempted to estimate the variability of the densities, lengths and biomasses of A. nodosum along this region. Given the long harvesting history taking place in Brittany, the proportion of canopy-covered shores along these coasts and the important functional role of this canopy for the community, it is even more surprising that such a study has never been performed in this area. The variability of the A. nodosum density and length over such an area could also affect the distribution of the associated algal and animal assemblages (Cervin et al., Reference Cervin, Lindegarth, Viejo and Aberg2004) and the ecosystem functioning. The present study thus aimed at: (1) estimating the variability of the A. nodosum lengths, biomasses and densities at the meso-scale (i.e. km to 100s of km); and (2) describing the animal and algal assemblages associated with the zone and their variability at the local scale (i.e. sampling site) and meso-scale. It will then provide a reference state for future studies on primary production estimates at regional scales and on the responses of rocky shores communities to environmental changes.
MATERIALS AND METHODS
The shores of Penmarc'h, Molène, Coulouarn, and Bréhat are distributed clockwise along a stretch of the Brittany coast (France) over 200 km long (Figure 1). The intertidal zone at Penmarc'h consists of large sheltered bedrock with large boulders. Because of the very weak slope of this shore, the Ascophyllum nodosum zone extends over several 100 m. The study site at Molène is located in the northern part of the Molène Island over a steeper and slightly less sheltered zone than Penmarc'h but also dominated by large granite boulders. The study site at Coulouarn is located on a small island made up of smaller boulders on which the A. nodosum zone only spreads over a few metres. This is probably the least sheltered of the five locations. Finally, the last two study sites are located on the Island of Bréhat, respectively on the west and the east coasts of the island. These two sites were the most sheltered ones, so much that the A. nodosum zone is found on a rocky zone slightly covered with fine sand and even some mud on the east coast. These five shores were chosen because they are representatives of the landscape variability of sheltered rocky shores along the Brittany coast. They are also shores where A. nodosum harvesting often takes place although we were careful in choosing study sites where no harvesting had taken place prior to the study.

Fig. 1. Location of the five study sites along the coast of Brittany, France. The sites of Penmarc'h and Coulouarn are located on the continent, while the two Bréhat sites are on the west and east coast of the Bréhat Island 1 km away from the continent and the Molène site is on the north-eastern coast of the Molène Island over 20 km away from the continent.
Sampling was performed in winter so that any influence of ongoing recruitment or post-recruitment mortality did not bias the composition of the algal and animal assemblages (Warner, Reference Warner and Gibbs1984). Sampling took place in early February 2005 on twelve 0.5 × 0.5 m quadrats distributed evenly on both sides of a 10 m transect line. Care was taken to place the transect perpendicularly to the tidal gradient and in the middle of the vertical distribution of the canopy zone of each site, so that the presence of other canopy species could not be accounted for by border effects with the neighbouring macroalgae belts. Traditionally, an Ascophyllum nodosum individual is defined as the assemblage of fronds or shoots arising directly or indirectly from a common holdfast (Baardseth, Reference Baardseth1955). However, holdfast fragmentation or holdfast formation from several zygotes is also possible (Aberg, Reference Aberg1989). The total length and maximum circumference of each A. nodosum was thus measured on individual plants, defined as all the shoots originating from touching holdfasts. On each quadrat, identification of all the macroalgae and macrofauna was recorded to the lowest possible taxon identifiable in the field. As this implied identification of different taxonomic levels, the algal and animal diversity are expressed as numbers of taxa per quadrat (taxa.quadrat−1). Densities (ind.m−2) were also estimated for each animal taxon, except for the colonial organisms and the polychaete Spirorbis sp., for which both densities and percentage cover are very difficult to obtain on three-dimensional substrates.
Ascophyllum nodosum density (ind.m−2) and biomass (kgDW.m−2) for each site were calculated from the mean number of A. nodosum individuals per quadrat and the mean A. nodosum dry weight per quadrat respectively. The A. nodosum dry weight of each quadrat is the sum of each A. nodosum plant dry weight (DW, expressed as gDW), estimated using the following allometric relationship (Aberg, Reference Aberg1990):

where l is the total length and c the maximum circumference of a single plant. A preliminary study, based on 77 A. nodosum plants collected at four different sites along the Brittany coastline, confirmed that this relationship could be used in our study. Indeed, there was a significant correlation between the estimated DW (x) based on the above relationship and the measured DW (y) (r = 0.981, N = 77, P < 0.001), which allowed calculating the following linear regression: y = 0.986·x − 1.78. The intercept of this regression was not significantly different from 0 (Student's t-test: t = 0.32, P = 0.375) while the slope was not significantly different from 1 (Student's t-test: t = 1.27, P = 0.104). Therefore, there is no significant difference between the measured and the estimated DW. The population structure of A. nodosum lengths was assessed at each site by establishing length–frequency histograms of the number of individuals per length-classes of 10 cm sizes smoothed using third degree weighted moving averages (Legendre & Legendre, Reference Legendre and Legendre1998). We compared how the percentage of fronds from the 10 cm size-classes was distributed among three groups of length-classes: (1) fronds below 20 cm, which is the French legal length for harvesting A. nodosum; (2) fronds above 70 cm, which is the mean length measured over the entire study; and (3) fronds between 20 cm and 70 cm.
Because of the small sample size (12 quadrats per site), most of our data lacked the normality and homoscedasticity required to perform ANOVAs. Significant differences between A. nodosum lengths, biomasses and densities, between mean numbers of algal or animal taxa and between mean numbers of animal densities were thus tested using Kruskal–Wallis tests (Hc, the value corrected for tied ranks, SSI SYSTAT 11 software, SYSTAT Software, Inc., Richmond, CA, USA). Post hoc tests (equivalent to the Student–Newman–Keuls test of the ANOVA; Scherrer, Reference Scherrer1984) were also performed when needed.
In order to test for any algal and animal taxonomic composition and density differences between the assemblages of each site, cluster analyses based on the Bray–Curtis similarity index (Clarke & Warwick, Reference Clarke and Warwick2001) were performed on the presence/absence data of both the algal and animal diversity and on the animal abundances (PRIMER v6.1.6 software; Clarke & Gorley, Reference Clarke and Gorley2001). The differentiation between groups was tested using a ‘similarity profile’ permutation test (SIMPROF test given in PRIMER v6.1.6). The analyses were performed after elimination of the rare taxa, i.e. present in only one quadrat for the two qualitative analyses and having only one individual for the quantitative analysis. For the interpretation of these analyses, we first looked at taxa exclusively present at one or several sites from those that were ubiquitous. Then, we considered their ‘occurrence frequency’ depending on whether taxa were present at few or many quadrats within a given site.
RESULTS
Ascophyllum nodosum
Mean (±standard deviation, SD) individual lengths measured 56 ± 28 cm at Coulouarn, 64 ± 41 cm at Bréhat west, 70 ± 46 cm at Bréhat east, 79 ± 36 cm at Molène and 88 ± 53 cm at Penmarc'h (Figure 2A). Densities were 14 ± 9.3 ind.m−2 in Bréhat east, 16 ± 8.4 ind.m−2 in Penmarc'h, 21 ± 4.3 ind.m−2 in Molène, 21 ± 19.7 ind.m−2 in Coulouarn, 24 ± 9.8 ind.m−2 in Bréhat west (Figure 2B). Biomasses were 2.5 ± 1.99 kgDW.m−2 in Bréhat east, 2.6 ± 1.82 kgDW.m−2 in Penmarc'h, 2.7 ± 0.98 kgDW.m−2 in Molène, 2.9 ± 2.42 kgDW.m−2 in Coulouarn and 3.7 ± 2.47 kgDW.m−2 in Bréhat west (Figure 2C). Individual lengths were significantly lower at Coulouarn than at Penmarc'h and Molène only (Hc = 19.718, P < 0.001, N= 290). On the contrary, the biomasses and densities showed no significant differences (biomass: Hc = 2.605, P = 0.626, N = 60; density: Hc = 8.326, P = 0.080, N = 60). The class subdivision of the population structure showed that the majority of fronds measured between 20 cm and 70 cm in Coulouarn, Bréhat west and Bréhat east while it measured over 70 cm in Penmarc'h and Molène (Table 1; Figure 3).
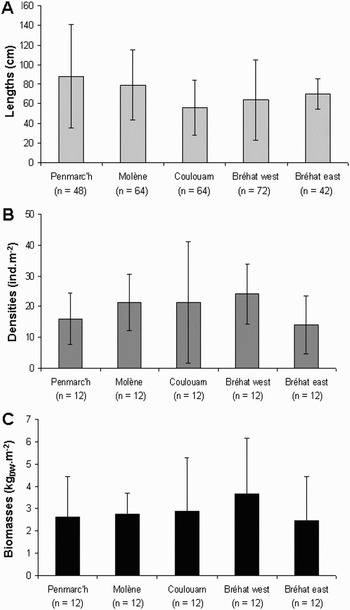
Fig. 2. Mean (±standard deviation) lengths (A), densities (B) and biomasses (C) of the Ascophyllum nodosum plants sampled during the study. Below the site names are given the number of A. nodosum individuals, for lengths, and quadrats, for densities and biomasses, used to estimate mean values.
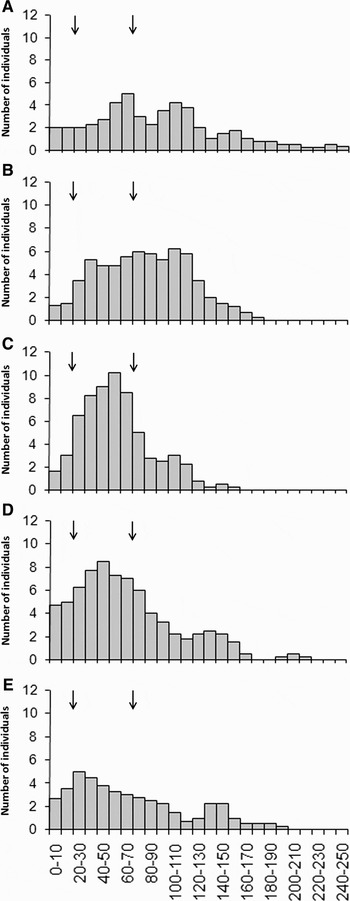
Fig. 3. Ascophyllum nodosum length–frequency histograms using third degree weighted moving averages over 10 cm size-classes at Penmarc'h (A), Molène (B), Coulouarn (C), Bréhat west (D) and Bréhat east (E). The size-classes are closed on the lower limit and opened on the higher limit. The arrows on the histograms indicate the 20 cm limit, which is the French legal length for hand harvesting, and the 70 cm limit, which is the mean length measured in the present study.
Table 1. Percentage of fronds grouped below 20 cm, which is the French legal length for hand harvesting, above 70 cm, which is the mean length measured in the present study, and between 20 cm et 70 cm.

Algal and animal diversity
ALGAL DIVERSITY
A total of 21 taxa were identified amongst the five sites (Table 2). The algal richness was significantly lower at Coulouarn than at all the other sites as well as significantly lower at Bréhat east than at Penmarc'h and Molène (Table 3) (Hc = 12.481, P = 0.014, N = 60).
Table 2. Presence of algal taxa at each site.
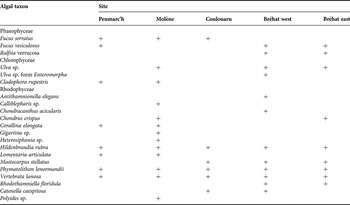
+, presence.
Table 3. Algal and macrofaunal taxa total number, mean (±standard deviation) number of taxa (taxa.quadrat−1) and densities of quantifiable macrofaunal taxa (ind.m−2) at each site.

N, total number; SD, standard deviation.
The cluster analysis based on the algal data was performed on a 57 quadrats × 18 taxa matrix after elimination of the uncommon taxa Calliblepharis sp., Chondracanthus acicularis and Polyides sp. and the removal of three quadrats that had no taxon present. The dendrogram resulted in the significant differentiation (π = 1.98, P = 0.018) between the group A, which isolated 10 of the 11 Bréhat east quadrats together with one-third of the Bréhat west quadrats in spite of some intragroup heterogeneity (similarity = 37.7%), and the group B (similarity = 39.2%), which included all the remaining quadrats (Figure 4). Within the group B, the differentiation between the group B1 (similarity = 44.6%) and the group B2 (similarity = 44.1%) was not significant (π = 2.03, P = 0.064) (Figure 4).

Fig. 4. Dendrogram resulting from the cluster analysis performed on the macroalgal presence or absence data on a 57 quadrats × 18 taxa matrix using the S17 Bray–Curtis similarity index at the sites of Penmarc'h (P), Molène (M), Coulouarn (C), Bréhat west (Bw), and Bréhat east (Be).
ANIMAL DIVERSITY
A total of 29 taxa were identified amongst the five sites (Table 4). There were no significant differences in the number of animal taxa between sites (Table 3) (Hc = 9.365, P = 0.053, N = 60). The cluster analysis performed on the qualitative data was carried out on a 60 quadrats × 21 taxa matrix after elimination of the uncommon taxa Anemonia viridis, Achantochitona sp., Chthamalus sp., Lipophrys pholis, Botryllus schlosseri, Bunodactis verrucosa, Dendrodoa grossularia and Flustrellidra hispida. The resulting dendrogram showed that no faunal assemblages could be significantly differentiated by the SIMPROF test; there is thus no overall structuring between sites (Figure 5).

Fig. 5. Dendrogram resulting from the cluster analysis performed on the macrofauna presence or absence data on a 60 quadrats × 21 taxa matrix using the S17 Bray–Curtis similarity index at the sites of Penmarc'h (P), Molène (M), Coulouarn (C), Bréhat west (Bw), and Bréhat east (Be).
Table 4. Presence and numbers when possible of macrofaunal taxa at each site.

+, presence.
Among the 29 animal taxa, 20 could be enumerated. Their mean densities (±SD) at Molène (30 ± 15.8 ind.m−2) were significantly lower than at the other sites while those at Penmarc'h (114 ± 36.2 ind.m−2) were significantly higher than at the two Bréhat sites (Table 3) (Hc = 24.268, P < 0.001, N = 60). The cluster analysis performed on the quantitative data was carried out on a 60 quadrats × 19 taxa matrix after elimination of the rare taxon Achantochitona sp. Again, the dendrogram showed no structuring between sites (data not shown).
DISCUSSION
Ascophyllum nodosum
Few Ascophyllum nodosum lengths and densities estimates have been reported so far in the literature, essentially due to the difficulty to define A. nodosum individuals. The mean (±SD) A. nodosum lengths estimated to 69 ± 26.3 cm and 86 ± 2.7 cm in two Irish sites (Kelly et al., Reference Kelly, Collier, Costello, Diver, McGarvey, Kraan, Morrissey and Guiry2001) and to 74.5 ± 27.6 cm in New Brunswick, Canada (Ugarte et al., Reference Ugarte, Sharp and Moore2006) are in the same range as measured in the present study. Densities estimated to 50 ind.m−2 in Maine, USA (Bertness et al., Reference Bertness, Leonard, Levine, Schmidt and Ingraham1999) were two to over three times higher than those measured in the present study. By contrast, the biomasses calculated in the present study along the Brittany coasts are comparable to the highest ones measured along the A. nodosum distribution (Table 5).
Table 5. Biomass (kgDW.m−2), measured using both direct and indirect methods, of Ascophyllum nodosum stands along the distribution of the species.

SD, standard deviation.
Differences in population structures could be observed between sites, going from a broad distribution over a wide range of lengths to a skewed distribution towards relatively long lengths. Both Bréhat sites displayed the greatest percentage of short lengths (i.e. fronds below 20 cm) and equal percentages of fronds over the rest of the distribution (i.e. fronds between 20 cm and 70 cm and above 70 cm) whereas Coulouarn was dominated by fronds between 20 cm and 70 cm. Finally, Molène, and even more so Penmarc'h, displayed a skewed distribution towards long lengths (i.e. above 70 cm). These different population structures reveal the important length heterogeneity that can be found among A. nodosum stands. Indeed, A. nodosum length can greatly vary either within or between sites, both at the sampling and at the site scale, due to the variability in: (1) grazing pressure (e.g. Viejo & Aberg, Reference Viejo and Aberg2003); (2) environmental forces such as wave action (e.g. Keser et al., Reference Keser, Swenarton and Foertch2005); or (3) harvesting impact (e.g. Ang et al., Reference Ang, Sharp and Semple1996). However, in our case, neither the differences in site exposure nor in grazer densities seem to support the observed population structures. More detailed information on the variability of these factors at the local scale as well as long term differences in recruitment intensity or changes in the survival of existing plants, which has proven to influence population growth rates (Aberg, Reference Aberg1992), could potentially explain the observed differences in population structures (Davies et al., Reference Davies, Johnson and Maggs2007). Unfortunately, these data are not available at our study sites or only available at the scale of the Brittany coast in the case of the wave regimes. In spite of these differences, the absence of significant differences in biomass (Figure 2C) suggests that the stimulated growth and production of new laterals on cut or basal shoots compensated for the lost biomass (Lazo & Chapman, Reference Lazo and Chapman1996; Ugarte et al., Reference Ugarte, Sharp and Moore2006).
Algal and animal diversity
The algal and animal community observed in the present study form a common assemblage typical of Ascophyllum nodosum zones. This assemblage is essentially dominated by the algae Hildenbrandia rubra, Phymatolithon lenormandii, Vertebrata lanosa and Ulva sp., and the invertebrates Carcinus maenas, Dynamena pumila, Gibbula umbilicalis, Nucella lapillus, Littorina obtusata, Patella vulgata and Spirorbis sp., also characteristic of most A. nodosum zones of other geographical areas (e.g. Boaden & Dring, Reference Boaden and Dring1980; Connor et al., Reference Connor, Brazier, Hill and Northern1997; Kelly et al., Reference Kelly, Collier, Costello, Diver, McGarvey, Kraan, Morrissey and Guiry2001; Cervin et al., Reference Cervin, Lindegarth, Viejo and Aberg2004).
ALGAL DIVERSITY
The significant difference in the number of algal taxa suggests a heterogeneous distribution of the taxonomic diversity at the meso-scale. Indeed, Coulouarn displayed a significantly lower mean number of taxa than at all the other sites due to the absence of any green taxa and that of the two-thirds of the red taxa identified over the study. Bréhat west had a significantly lower mean number of taxa than Penmarc'h and Molène due to the overall lower occurrence frequencies at the sampling scale. The structuring between sites evidenced by the cluster analysis reveals a homogeneous distribution of the algal identity composition at the sampling scale but with a heterogeneous distribution between sites. In particular, the two groups evidenced by the dendrogram discriminated sites essentially upon the presence of either Fucus vesiculosus, almost exclusively present in the Bréhat sites from the A group, or Fucus serratus, frequently present on the other sites and absent from Bréhat. The Bréhat sites exhibited high biomasses distributed over short individuals, probably enabling more light to reach the substrate. This could allow F. vesiculosus, apparently more competitive among sparser A. nodosum canopies, to develop faster than F. serratus (Jenkins et al., Reference Jenkins, Norton and Hawkins1999b). On the contrary, F. serratus will take over under the more developed or dense A. nodosum canopies of Penmarc'h, Molène and Coulouarn.
ANIMAL DIVERSITY
Contrary to the algal richness, there is an absence of significant difference in the number of taxa, which suggests a homogeneous distribution of the animal taxonomic richness at the meso-scale. The absence of strong structuring between sites evidenced by the cluster analysis reveals on the contrary a heterogeneous distribution of the animal identity composition at the sampling scale. This heterogeneity at the sampling scale prevents characterizing the animal identity composition at the meso-scale.
The significant difference in animal densities suggests a heterogeneous distribution of the overall densities at the meso-scale. The observed site ranking, resulting from significantly lower densities at Molène than at the other sites and significantly higher densities at Penmarc'h than at Bréhat west and east, is mostly due to density differences of Littorina obtusata and Gibbula umbilicalis, the most abundant taxa of the Ascophyllum nodosum zone studied here. Indeed, these two taxa showed lowest densities at Molène, intermediate at the two Bréhat sites and highest at Penmarc'h. In addition, excluding the densities of L. obtusata, G. umbilicalis, Patella vulgata, Osilinus lineatus and Balanus perforatus from the analysis resulted in no significant difference between sites (Hc = 4.679, P = 0.322, N = 60). This emphasizes how A. nodosum zones are characterized by the dominance of a few typical macrofaunal taxa. Although the dominance of L. obtusata and G. umbilicalis among A. nodosum stands might seem evident, previous studies have concentrated on the first species only. The A. nodosum zone is recognized as L. obtusata preferred habitat compared to other fucoid zones (e.g. Underwood, Reference Underwood1973) and this species is the dominant periwinkle of most European A. nodosum shores (e.g. Watson & Norton, Reference Watson and Norton1987; Williams, Reference Williams1995). On the contrary, the zonation of G. umbilicalis has been rarely dealt with (Underwood, Reference Underwood1973) and a wide range of intertidal habitats, more or less covered with macroalgae, seems to suit this prosobranch (Bode et al., Reference Bode, Lombas and Anadon1986; Gaudèncio & Guerra, Reference Gaudèncio and Guerra1986; Kendall & Lewis, Reference Kendall and Lewis1986). At the sampling scale, the absence of structuring between sites evidenced by the cluster analysis reveals a heterogeneous distribution of the taxonomic densities.
The A. nodosum zone is thus characterized by: (1) a homogeneous taxonomic richness of the macrofaunal assemblage at the meso-scale, typical of these shores; (2) an important assemblage of accessory taxa responsible for a taxonomic composition heterogeneity at the sampling site; (3) a heterogeneous distribution of the overall macrofauna densities at the meso-scale, due to the density differences of the most abundant taxa; and (4) a heterogeneous distribution of the taxonomic densities at the sampling scale due to a great variability in the densities of the accessory taxa.
CONCLUSION
The present study established that both the algal and animal assemblages are typical of Ascophyllum nodosum zones at the meso-scale studied here. Differences between sites in environmental forces, such as light penetration through the canopy, but probably also wave action and sediment accumulation as observed in the Bréhat sites, and substrate heterogeneity, might explain the major differences in algal diversity. More detailed characteristics of these forces at the local scale would however need to be obtained to validate these hypotheses. The animal assemblage was taxonomically homogeneous at the meso-scale. Yet, the differences in densities of the most abundant taxa could not be accounted for in the present study. A detailed analysis of the zonation and distribution of these species as well as the environmental forces and coastal hydrodynamic differences between sites (Menge et al., Reference Menge, Daley, Wheeler, Dahlhoff, Sanford and Strub1997) would probably improve our understanding of the regional diversity variations observed in the present study.
The A. nodosum zones studied along the coast of Brittany display comparable mean lengths to previously reported values from other regions over the distribution of the species. Unlike the mean lengths, the estimated biomasses are amongst the highest measured along the species range. The differences in population structures allowed emphasizing the capacity of A. nodosum to rapidly compensate for lost biomasses through vegetative growth. Finally, the biomasses estimated at the meso-scale complete the rare estimates available for macroalgae in this region. The lack of significant biomass variability at the meso-scale suggests that these data could be used together with regional cartography studies of A. nodosum stands to establish good estimates of the A. nodosum biomasses present around Brittany.
ACKNOWLEDGEMENTS
The authors wish to thank P. Arzel, C. Broudin, C. Daguin-Thiébaut, C. Destombe, C. Engel, F. Gentil, D. Knoch, E. Lacut, L. Lévèque, D. Muths, F. Rigal, I. Smyczynski and M. Voisin for their great help in sampling, N. Simon for her help with algal identification, R. Cousens for sharing his knowledge on the biology of A. nodosum, J. Trigui for her help using the PRIMER software, and R. Araújo for collecting and measuring the A. nodosum individuals for the preliminary study on the allometric relationship. This work was part of PhD thesis of C.G., which was financially supported by the Brittany region.