Introduction
Cell adhesion and its regulation are essential for the formation of different organs and maintenance of multicellularity. It is particularly important for the developing brain with its myriad of synaptic connections between neurons, but also for the dynamic interactions of other cells with their target structures. Equally important is cellular deadhesion, for example, during cellular movement and for metastasis of malignant cells.
Currently, we know a number of proteins, which are of pivotal importance for cellular interactions between cells and with the extracellular matrix. These include cadherins, which are essential for interactions of epithelial cells (Takeichi, Reference Takeichi1977, Reference Takeichi1991), selectins, which bind to the carbohydrate motif sialyl LeX on glycoproteins and glycolipids on blood endothelia and hematopoietic cells (Phillips et al., Reference Phillips, Nudelman, Gaeta, Perez, Singhal, Hakomori and Paulson1990; Walz et al., Reference Walz, Aruffo, Kolanus, Bevilacqua and Seed1990; Polley et al., Reference Polley, Phillips, Wayner, Nudelman, Singhal, Hakomori and Paulson1991), and the integrins with their ligands. Integrins bind to extracellular ligands such as fibronectin, fibrinogen, and collagens, but also to cellular ligands such as the intercellular adhesion molecules (ICAMs) (Rothlein et al., Reference Rothlein, Dustin, Marlin and Springer1986; Patarroyo et al., Reference Patarroyo, Clark, Prieto, Kantor and Gahmberg1987; Gahmberg, Reference Gahmberg1997) and the vascular cell adhesion molecule-1 (VCAM-1).
Most integrins normally exist in a resting state and need some activation to become adhesive. This is particularly true for blood cell integrins, which must be strictly regulated in the activity. Otherwise cell aggregation and blood clotting would take place, a reduced immunological response would ensue, etc., resulting in dangerous complications.
Several reviews have been published on integrins and their structures and the integrin cellular ligands (Springer, Reference Springer1990; Ruoslahti, Reference Ruoslahti1991; Hynes, Reference Hynes1992; Schwartz et al., Reference Schwartz, Schaller and Ginsberg1995; Gahmberg, Reference Gahmberg1997; Gahmberg et al., Reference Gahmberg, Tolvanen and Kotovuori1997, Reference Gahmberg, Fagerholm, Nurmi, Chavakis, Marchesan and Grönholm2009; Hynes, Reference Hynes2002; Arnaout et al., Reference Arnaout, Mahalingam and Xiong2005; Luo et al., Reference Luo, Carman and Springer2007; Takada et al., Reference Takada, Ye and Simon2007; Bachmann et al., Reference Bachmann, Kukkurainen, Hytönen and Wehrle-Haller2019). In this review, we focus on the essential problem how integrin-mediated adhesion and signaling are regulated. Integrins can be activated by inside-out activation or by outside-in activation (Kim et al., Reference Kim, Carman and Springer2003; Abram and Lowell, Reference Abram and Lowell2009; Hu and Luo, Reference Hu and Luo2013). It is, however, well established that cytosolic proteins interacting with the integrin intracellular tails are of pivotal importance for integrin regulation. We will here describe the most important findings dealing with the integrin – cytoplasmic interactions, and try to obtain a general view of how the integrin activities may be regulated. It is apparent, however, that integrin regulation is unusually complex and still incompletely understood. Integrin/cytoplasmic protein interactions can in theory be regulated by protein expression and degradation, accessibility, structural changes either in the integrins or the interacting proteins, and by posttranslational modifications. There are few ways to alter protein–protein interactions in a fast, economical, and specific way. Phosphorylation is perhaps the most important one in many cell regulatory systems, and recent work has shown that specific phosphorylations play important roles in the regulation of adhesion. Due to the labile nature of phosphorylation and the potential expression of around 500 protein kinases and about 100 phosphatases in the mammalian genome, phosphorylation studies are challenging. In this review, we focus on what is currently known about the regulation of integrin activity. We focus on integrin regulation in leukocytes and platelets, because integrin regulation is best understood in these cells.
Discovery of integrins
In 1973, fibronectin was discovered (Gahmberg and Hakomori, Reference Gahmberg and Hakomori1973; Hynes, Reference Hynes1973). It was the first protein found that later turned out to be an adhesion protein. With the help of newly developed monoclonal antibodies, a leukocyte protein complex was identified that was involved in several functions. The functions were mapped to two leukocyte proteins named leukocyte function-associated antigen-1 (LFA-1) and macrophage-1 antigen (Mac-1) (Sanchez-Madrid et al., Reference Sanchez-Madrid, Simon, Thompson and Springer1983). A genetic deficiency named leukocyte adhesion deficiency (LAD) with impaired T cell functions, inability to synthesize immunoglobulins by B lymphocytes, and an increased susceptibility to life-threatening infections was later described, and the cells were shown to lack the leukocyte cell surface proteins mentioned above (Arnaout et al., Reference Arnaout, Spits, Terhorst, Pitt and Todd1984; Springer et al., Reference Springer, Thompson, Miller, Schmalstieg and Anderson1984). In 1982, Patarroyo et al. showed that phorbol esters induced leukocyte aggregation (Patarroyo et al., Reference Patarroyo, Yogeeswaran, Biberfeld, Klein and Klein1982). After screening with more than 100 monoclonal antibodies, reacting with leukocyte cell surfaces, the phorbol ester-induced leukocyte aggregation (adhesion) could be inhibited by a single monoclonal antibody (60.3) (Patarroyo et al., Reference Patarroyo, Beatty, Fabre and Gahmberg1985a, Reference Patarroyo, Beatty, Serhan and Gahmberg1985b). The adhesion receptors identified were named Leu-Cam, and they turned out to be the same protein complex described above to be absent in the genetic disease called leukocyte adhesion deficiency-I (LAD-I). Simultaneously, Ruoslahti and coworkers prepared fibronectin fragments, which replicated the activity of fibronectin (RGD) (Pierschbacher and Ruoslahti, Reference Pierschbacher and Ruoslahti1984), and used them to identify the cellular receptor (Pytela et al., Reference Pytela, Pierschbacher and Ruoslahti1985). Sequencing of the fibroblast and leukocyte receptors showed that they were related and the common name for them ‘integrin’ was coined by Hynes (Hynes, Reference Hynes1987). This name was chosen to illustrate the fact that the receptors integrate the cell surface with the interior cytoskeleton and vice versa. Later work then showed that the integrins form a receptor superfamily, and the integrins were grouped according to their β-polypeptides (Fig. 1).

Fig. 1. A schematic figure of the human integrin family. The integrins are grouped according to their β-chains. Note that some β-chains can associate with more than one α-chain.
Lower animals have few integrins and, for example, in the nematode Caenorhabditis elegans, there are only two. Most cells express several types of integrins reflecting their different binding specificities and functions. Absence of β1 integrins is lethal, whereas absence or defective functions of the β2 integrin chain results in LAD-I, with life-threatening infections in the childhood. Defects in the αIIbβ3 integrin may result in Glanzmann's thrombasthenia. However, many integrin gene knockouts in the mouse have only mild or no effects possibly due to compensatory effects of other members of the integrin family. Selective absence of leukocyte α4β1, LFA-1, and Mac-1 results in increased leukocytosis and susceptibility to microbial infections (Kanwar et al., Reference Kanwar, Smith, Shardonofsky and Burns2001; Scott et al., Reference Scott, Priestley and Papayannopolou2003; Ghosh et al., Reference Ghosh, Chackerian, Parker, Ballantyne and Behar2006; Chen and Sheppard, Reference Chen and Sheppard2007).
Integrin structure
The integrins are heterodimers formed by two membrane spanning polypeptides, α- and β-chains (Fig. 2). They are glycoproteins, and the structures of the β2-integrin N-glycosidic carbohydrate chains have been determined (Asada et al., Reference Asada, Furukawa, Kantor, Gahmberg and Kobata1991). Interestingly, they contain the sialyl LeX structure and bind E-selectin (Kotovuori et al., Reference Kotovuori, Tontti, Pigott, Shepherd, Kiso, Hasegawa, Renkonen, Nortamo, Altieri and Gahmberg1993).
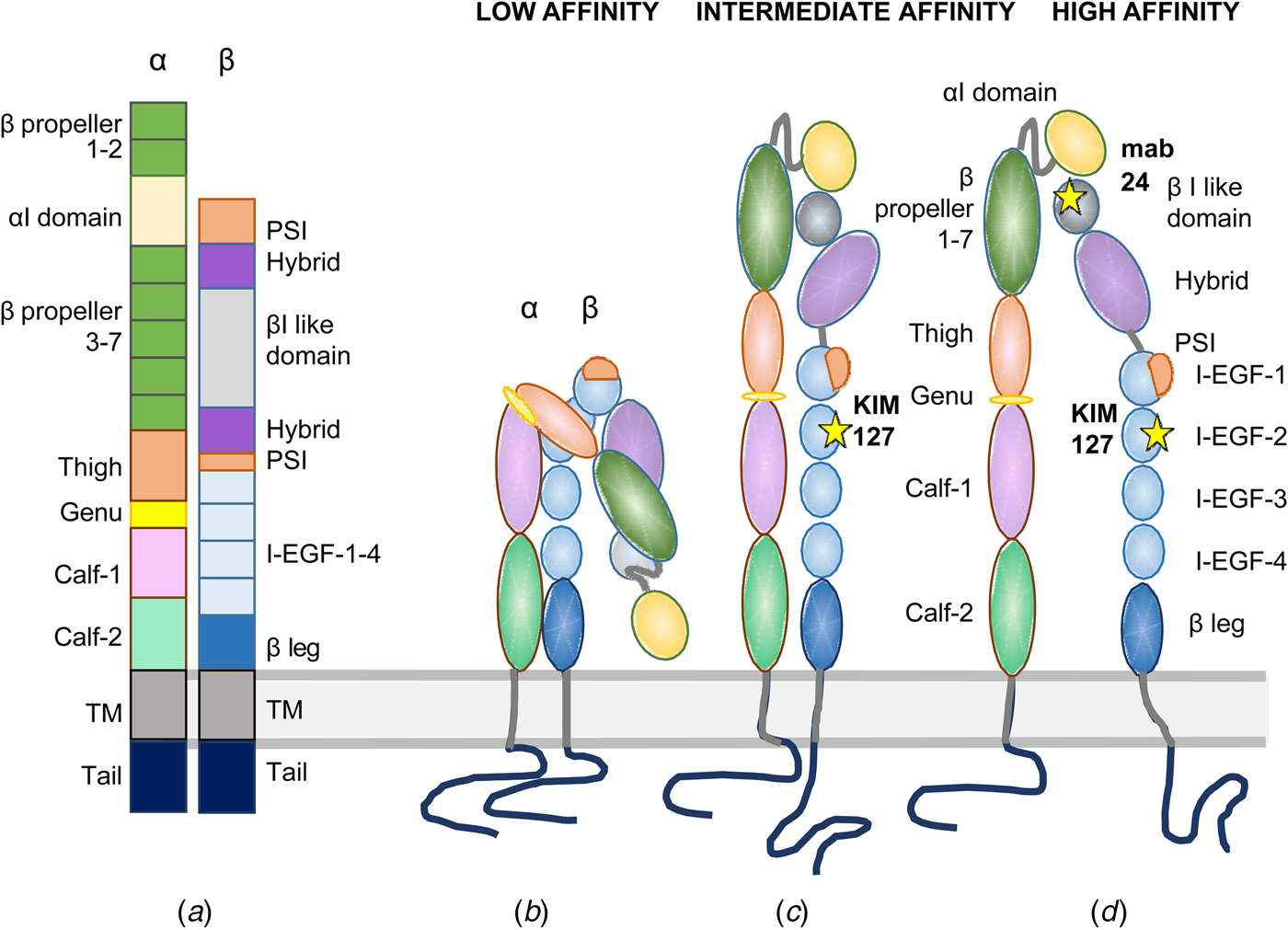
Fig. 2. Domain structures of I-domain integrins. In (a), the domains are depicted in a linear fashion. An inactive integrin is shown in (b) with the NH2-terminal binding site turned toward the lipid membrane. In (c), the integrin is extended with the external ligand binding site closed, and the cytoplasmic tails clasped. In (d), the integrin is fully active with the ligand binding site open and the cytoplasmic tails apart. The KIM127 epitope is available for antibody binding in (c) and (d). The mab24 binds only to the active integrin in (d).
No complete integrin has been crystallized, and the structure elucidated, but the structure of the external domains has been elucidated for a few integrins. The α-chains of nine integrins contain an inserted I-domain, the structure of which was first determined (Lee et al., Reference Lee, Rieu, Arnaout and Liddington1995). It contains a conserved metal ion-dependent adhesion site (MIDAS), which is needed for ligand binding. When the structure of the first integrin external part was determined (αVβ3), it came as a surprise that the NH2-terminal ligand binding region was turned back toward the membrane (Xiong et al., Reference Xiong, Stehle, Diefenbach, Zhang, Dunker, Scott, Joachimiak, Goodman and Arnaout2001). This structure does not bind ligands, or if so very weakly. Later, more integrin surface domains and parts of the transmembrane domains have been structurally determined (Xiong et al., Reference Xiong, Mahalingham, Alonso, Borrelli, Rui, Anand, Hyman, Rysiok, Muller-Pompalla, Goodman and Arnaout2009; Chen et al., Reference Chen, Xie, Nishida, Li, Walz and Springer2010, Reference Chen, Yu, Mi, Waltz and Springer2012; Xie et al., Reference Xie, Zhu, Chen, Mi, Nishida and Springer2010; Dong et al., Reference Dong, Mi, Zhu, Wang, Hu, Luo and Springer2012; Sen et al., Reference Sen, Yuki and Springer2013, Reference Sen, Koksal, Yuki, Wang and Springer2018; Springer and Sen, Reference Springer and Sen2016; Moore et al., Reference Moore, Aaron, Chew and Springer2018). It is now well established that integrins can exist in at least three different conformations: (1) bent toward the membrane, (2) extended, but with a closed ligand binding site, and (3) extended with the binding site open. An extended open integrin has more than 1000 times higher ligand affinity than the resting bent form (Li and Springer, Reference Li and Springer2017a, Reference Li and Springer2017b). The affinities of different integrins may, however, be different even when expressed in the same cell. Using negative staining and electron microscopy whole integrins has been visualized, and one can see the different conformational states of the integrins (Fig. 3). The binding sites of different monoclonal antibodies, used for studies on β2 integrin function, are shown in Fig. 4. The Kim127 antibody (extended closed) (Robinson et al., Reference Robinson, Andrew, Rosen, Brown, Ortlepp, Stephens and Butcher1992), Mab24 (extended open) (Dransfield et al., Reference Dransfield, Cabanas, Craig and Hogg1992), and the 7E4 adhesion inhibitory antibody for the leukocyte LFA-1 (Nortamo et al., Reference Nortamo, Patarroyo, Kantor, Suopanki and Gahmberg1988). The Pac1 antibody reacts with the extended open form of the platelet integrin αIIbβ3 (Shattil et al., Reference Shattil, Cunningham and Hoxie1987).

Fig. 3. Electron micrographs of negative stained αXβ2 integrin. In A is shown the inactive integrin with the ligand binding site closed. In B1 and B2 are shown extended, but not fully active integrins. B3 shows the active, open integrin. The figure was reprinted from Chen et al. (Reference Chen, Xie, Nishida, Li, Walz and Springer2010) with permission from the Proceedings of the US National Academy of Sciences.

Fig. 4. A schematic figure of the LFA-1 integrin with functionally important monoclonal antibody binding sites marked. See also Fig. 2.
The transmembrane domains form α-helices, and are evidently closely associated through GxxxG interactions in the transmembrane domains (Kim et al., Reference Kim, Lau, Ulmer and Ginsberg2009), similar to that found in red cell glycophorin (MacKenzie et al., Reference MacKenzie, Prestegard and Engelman1997).
The integrins have relatively short cytoplasmic tails, 20–60 amino acids long (an exception is the integrin β4 polypeptide), but they are nevertheless important for integrin function (Ylänne et al., Reference Ylänne, Huuskonen, O'Toole, Ginsberg, Virtanen and Gahmberg1995) (Fig. 5). A number of proteins bind to the integrin cytoplasmic domains, and this is particularly true for the integrin β-chains. Much fewer cytoplasmic proteins seem to bind to the α-chain cytoplasmic domains. On the other hand, integrin external ligands bind to the α-chain I (A)-domain or to a combinatory binding site formed by the α- and β-chains. As discussed below, both the α- and the β-chains have important regulatory roles.

Fig. 5. Human integrin cytoplasmic tail sequences. Known phosphorylation sites are marked in red.
It is obvious that only a few cytoplasmic proteins can simultaneously bind to the intracellular portions of the integrins. This means that the interactions must be both structurally and temporally regulated. These interactions are often short lived. For example, during cellular migration, there must be a continuous formation and release of bonds between integrins and external ligands. The same must be true for integrin cytosolic interactions. The integrin interactions with intracellular molecules must not be direct. A number of cytosolic proteins bind to integrins indirectly through the integrin-bound proteins. Such adhesion complexes may be very large as evident from proteomic studies (Zaidel-Bar et al., Reference Zaidel-Bar, itzkovitz, Ma àyan, Iyengar and Geiger2007; Byron et al., Reference Byron, Humphries, Bass, Knight and Humphries2011; Needham et al., Reference Needham, Parker, Burykin, James and Humphrey2019).
The cytoplasmic domains of the integrin β-chains are homologous with a proximal HDR(R/K)E sequence, two functionally important NXX(Y/F) motifs and a TTT/TTV/STF sequence between them. For the most part, the α-chain cytoplasmic sequences are diverse, with the exception of the well-preserved GFFKR motif in the proximal part of the domains (Fig. 5). A salt bridge is formed between the arginine residue of the GFFKR motif in the α-chains and aspartic acid (D731 in β2) in the β-chains (Hughes et al., Reference Hughes, Diaz-Gonzales, Leong, Wu, McDonald, Shattil and Ginsberg1996; Vinogradova et al., Reference Vinogradova, Velyvis, Velyviene, Hu, Haas, Plow and Qin2002).
Integrin external ligands
Integrins bind to a variety of ligands, which include cellular receptors, extracellular matrix proteins, soluble proteins in various body fluids, and microbial proteins and carbohydrates. The topic has been extensively reviewed, and the reader is advised to read the following reviews (Yamada and Olden, Reference Yamada and Olden1978; Hynes and Yamada, Reference Hynes and Yamada1982; Yamada, Reference Yamada1983; Ruoslahti, Reference Ruoslahti1988, Reference Ruoslahti1996; Gahmberg, Reference Gahmberg1997). Many β1-integrins, such as α5β1, bind to extracellular matrix proteins like fibronectin (Ruoslahti, Reference Ruoslahti1991; Hynes, Reference Hynes1992) and α1β1, α2β1, α10β1 to different types of collagens (Knight et al., Reference Knight, Morton, Peachey, Tuckwell, Farndale and Barnes2000; Zeltz and Gullberg, Reference Zeltz and Gullberg2016). Exceptions are the α4β1, α9β1, and α4β7 integrins, which also can bind to the membrane protein VCAM-1. The subset of β1 integrins binding various laminin isoforms includes α3β1, α6β1, and α7β1, but also α6β4 (Humphries et al., Reference Humphries, Byron and Humphries2006; Takada et al., Reference Takada, Ye and Simon2007). The platelet integrin αIIbβ3 interacts, for example, with proteins that are important in blood coagulation, such as fibrinogen. Several integrins recognize the arginine–glycine–aspartic acid (RGD) or the leucine–aspartic acid–valine (LDV) sequences in the ligands. For example, α4β1 and α9β1 bind to fibronectin via LDV, and α5β1 via RGD.
In contrast, the β2-integrins interact for the most part with cellular ligands. The ICAMs and VCAM-1 are type I membrane proteins belonging to the immunoglobulin superfamily. They span the cell membrane once, and their cytoplasmic domains interact with cytoskeletal proteins. Five ICAM molecules are present in mammals with different expressions. ICAM-1 and ICAM-3 have five immunoglobulin (Ig)-domains, ICAM-2 and ICAM-4 have two, and the most complex one, ICAM-5, has nine Ig-domains (Gahmberg, Reference Gahmberg1997). In most cases, the integrin binding sites are in the NH2-terminal Ig-domain with the exception of Mac-1, the binding site of which is in domain-3 of ICAM-1 (Diamond et al., Reference Diamond, Staunton, Marlin and Springer1991). The ICAM binding activity is regulated by expression. ICAM-1 is easily up-regulated by cytokines and during inflammation, whereas the other ones are more stably expressed. ICAM-1 and ICAM-2 are present in leukocytes and endothelial cells, and ICAM-3 in leukocytes. ICAM-4 is erythroid cell specific (Bailly et al., Reference Bailly, Tontti, Hermand, Cartron and Gahmberg1995), and ICAM-5 is confined to the dendrites and the cell bodies of central neurons (Yoshihara et al., Reference Yoshihara, Oka, Nemoto, Watanabe, Nagata, Kagamiyama and Mori1994; Tian et al., Reference Tian, Yoshihara, Mizuno, Mori and Gahmberg1997; Gahmberg et al., Reference Gahmberg, Tian, Ning and Nyman-Huttunen2008). It may suppress T cell activation (Tian et al., Reference Tian, Lappalainen, Autero, Hänninen, Rauvala and Gahmberg2008). LFA-1 binds to all ICAMs, but not to extracellular matrix molecules. The other β2-integrins, Mac-1, αXβ2, and αDβ2, are less restricted in binding and may bind to soluble host proteins, and also to microbial molecules.
Structural changes in integrins upon activation
In resting cells, the integrins have their NH2-terminal ligand-binding domains turned toward the membrane. Upon activation, the integrins extend, and may open their ligand binding site (Nishida et al., Reference Nishida, Xie, Shimaoka, Cheng, Walz and Springer2006). In the I-domain integrins, the binding site is present in the I-domain. Upon activation, the α7-helix moves down. In the other integrins, the binding site is formed by a combination of the β-propeller of the α-chains and the βI domain of the β-chains. Upon activation, the βI α7-helix moves toward the βI interface with the hybrid domain, resulting in an outward movement of the hybrid domain, and exposure of the binding site. The cytoplasmic domains also move apart enabling new interactions of cytoplasmic proteins with the integrin tails (Springer and Dustin, Reference Springer and Dustin2012). These findings show that there exists a closely regulated connection between the integrin external parts and the inner domains. How the allosteric structural alterations are generated remains incompletely understood.
The fact that cells express many different integrins shows that cells need them for several different functions (Schwartz et al., Reference Schwartz, Schaller and Ginsberg1995; Arnaout et al., Reference Arnaout, Mahalingam and Xiong2005; Luo et al., Reference Luo, Carman and Springer2007; Hogg et al., Reference Hogg, Patzak and Willenbrock2011; Huttenlocher and Horwitz, Reference Huttenlocher and Horwitz2011; Mitroulis et al., Reference Mitroulis, Alexaki, Kourtzelis, Ziogas, Hajishengallis and Chavakis2015; Kourtzelis et al., Reference Kourtzelis, Mitroulis, von Renesse, Hajishengallis and Chavakis2017; Fagerholm et al., Reference Fagerholm, Guenther, Asens, Savinko and Uotila2019). Their activity must be regulated, and often so in different ways. Therefore, results obtained for one integrin may not apply to another one. The cellular environment, including molecular neighbors, is certainly also important.
Experimental results indicate that the β-chains are most important for the regulation of integrin activity, but they also show that the α-chains closely cooperate with the β-chains, and reciprocally that the β-chains regulate the ligand binding activity of the α-chains.
The integrin cytoplasmic tails are relatively unstructured, and their conformations and movements are restricted by interactions with cytosolic and cytoskeletal proteins. Interactions with the inner leaflet of the lipid membrane are also important.
The integrin cytoplasmic domains contain numerous binding sites for intracellular proteins
The integrins are not only mere adhesion proteins, although their primary functions are based on their capacity to adhere. They are pivotal components of machineries for cellular movement, cell spreading, phagocytosis, metastasis of malignant cells, blood coagulation, brain and immune synapse formation, etc. Furthermore, they act, perhaps due to their ‘sticky nature’, as receptors for various microbes (Gbarah et al., Reference Gbarah, Gahmberg, Ofek, Jacobi and Sharon1991; Wickham et al., Reference Wickham, Mathias, Cheresh and Nemerow1993; Jackson et al., Reference Jackson, Sheppard, Denyer, Blakemore and King2000; Campadelli-Fiume, Reference Campadelli-Fiume, Collins-McMillen, Gianni and Yurochko2016). Their ability to signal in two directions across the plasma membrane makes them unique. The integrin β-chains are quite well conserved, which indicates that their functions are quite similar. The α-chains are much more diverse, enabling more specific functions. Rather few proteins have been found to bind to the α-chain cytoplasmic domains, and at least some of them act as negative regulators of integrin activity.
Figure 6 shows the sequence of the LFA-1 cytoplasmic tails, and the binding sites for some of the intracellular proteins that interact with αL or β2. The α-chain cytoplasmic domain contains about 60 amino acids, and the β-chain domain about 45. This means that the β-chain cytoplasmic segment is about 180 Å long if extended. In fact, some part of it forms an α-helix, and some parts are more extended. This means that at most 5–6 average size proteins could bind to it at the same time. Therefore, the interactions of cytoplasmic proteins with the β-chain must be strictly regulated. On the other hand, several proteins may indirectly bind to the integrins through the integrin tail binding proteins, and form adhesomes. In fact, it has been estimated by proteomics that hundreds of proteins can interact with the integrins (Zaidel-Bar et al., Reference Zaidel-Bar, itzkovitz, Ma àyan, Iyengar and Geiger2007; Byron et al., Reference Byron, Humphries, Bass, Knight and Humphries2011).
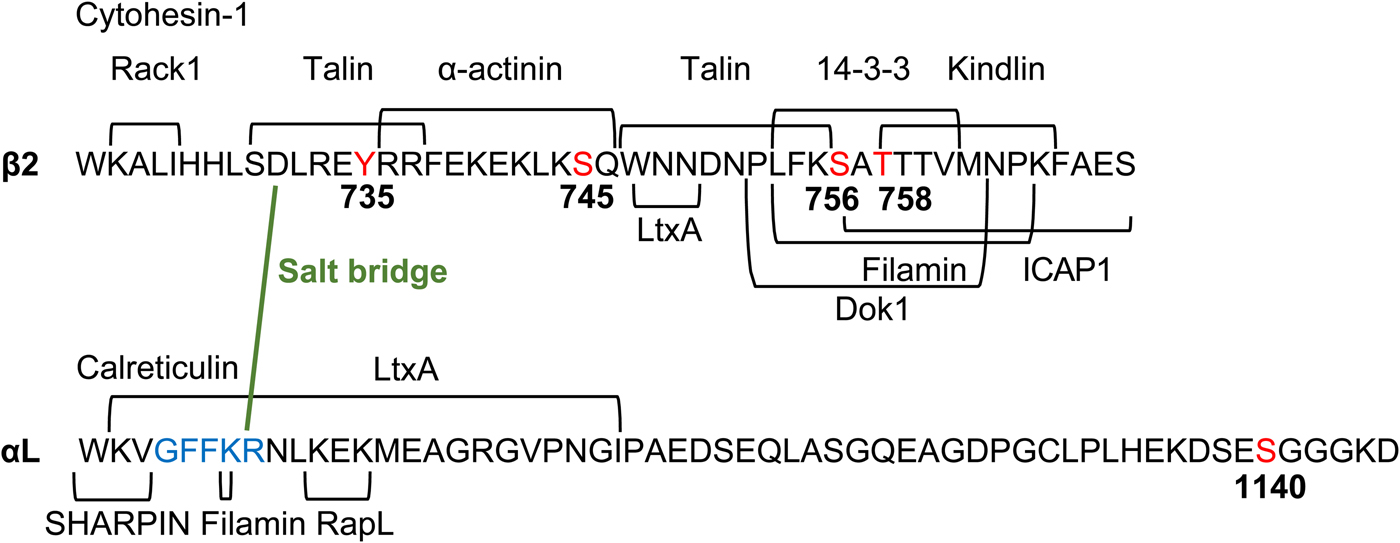
Fig. 6. Protein binding sites to the cytoplasmic tails of LFA-1. The phosphorylation sites are marked in red and the conserved α-tail GFKKR sequence in blue.
In the following part of the review, we briefly describe cytoplasmic proteins that are involved in the regulation of integrin activity. Most of these proteins have been subjects of previous reviews, and the reader is referred to them for additional information (Sampath et al., Reference Sampath, Gallagher and Pavalko1998; Kim et al., Reference Kim, Carman and Springer2003; Abram and Lowell, Reference Abram and Lowell2009; Moser et al., Reference Moser, Legate, Zent and Fässler2009b; Anthis and Campbell, Reference Anthis and Campbell2011; Calderwood et al., Reference Calderwood, Campbell and Critchley2013; Klapholz and Brown, Reference Klapholz and Brown2017). Thereafter, we discuss how the proteins, by interacting with the integrin cytoplasmic domains, may regulate integrin binding and signaling activity.
Integrin β-tail binding cytoplasmic proteins
Talin
Talin-1 and -2 are large cytosolic 270 kDa proteins consisting of a head region and a long rod (Burridge and Connell, Reference Burridge and Connell1983; Critchley, Reference Critchley2009; Calderwood et al., Reference Calderwood, Campbell and Critchley2013; Klapholz and Brown, Reference Klapholz and Brown2017). The two talins are functionally quite similar, but have different cellular distributions. The talin head is related to the FERM (4.1, ezrin, radixin, moesin) proteins, which are known to bind to several integral membrane proteins. The rod binds to the actin cytoskeleton. Talin can be cleaved by the Ca2+-activated protease calpain, and this reaction may be important for the inside-out activation of integrins (Carragher et al., Reference Carragher, Levkau, Ross and Raines1999; Glading et al., Reference Glading, Lauffenburger and Wells2002; Franco et al., Reference Franco, Rodgers, Perrin, Han, Bennin, Critchley and Huttenlocher2004; Franco and Huttenlocher, Reference Franco and Huttenlocher2005). The involvement of talin in cell adhesion has mainly been studied in platelets and leukocytes (Calderwood et al., Reference Calderwood, Zent, Grant, Rees, Hynes and Ginsberg1999, Reference Calderwood, Campbell and Critchley2013; Goult and Schwartz, Reference Goult and Schwartz2018; Sun et al., Reference Sun, Costell and Fässler2019). The talin head binds to the proximal NXXY/F sequence in the β-chains through its phosphotyrosine binding domain (PTB) in the F3 subdomain, but also to an α-helix in the proximal part of the cytoplasmic domain (Tadokoro et al., Reference Tadokoro, Shattil, Eto, Tai, Liddington, de Pereda, Ginsberg and Calderwood2003). Talin-1 knockout mice die early during embryonic development, which shows that the protein is essential. In vitro experiments show that the purified talin head can activate integrins by forcing the cytoplasmic parts apart, and it has been proposed to be the final component in the activation cascade (Kim et al., Reference Kim, Ye, Hu and Ginsberg2012). The talin rod may bind to several F-actin molecules, further increasing the clustering of integrins, and thus up-regulating adhesion by an increase in avidity. Talin interacts with phosphatidylinositol 4,5-bisphosphate at the inner surface of the plasma membrane, and this interaction is considered to be important for adhesion (Chinthalapudi et al., Reference Chinthalapudi, Rangarajan and Izard2018).
Kindlins
The kindlin family of proteins consists of three members (Ussar et al., Reference Ussar, Wang, Linder, Fässler and Moser2006). Kindlin-1 is mainly expressed in epithelia, kindlin-2 is ubiquitously expressed, and kindlin-3 expression is restricted to hematopoietic cells. Genetic absence of kindlin-1 results in a skin disease with blisters, an absence of kindlin-2 is lethal, and a deficiency of kindlin-3 results in the LAD III syndrome (Malinin et al., Reference Malinin, Zhang, Choi, Ciocea, Razorenova, Ma, Podrez, Tosi, Lennon, Caplan, Shurin, Plow and Byzova2009; Svensson et al., Reference Svensson, Howarth, McDowall, Patzak, Evans, Ussar, Moser, Metin, Fried, Tomlinson and Hogg2009; Moser et al., Reference Moser, Bauer, Schmid, Ruppert, Schmidt, Sixt, Wang, Sperandio and Fässler2009a). Like the talins, the kindlins contain a FERM domain, but they lack a rod domain. Kindlin-1 may bind to the proximal NPXY sequence (Gao et al., Reference Gao, Bao, Ge, Sun, Sun, Liu, Chen, Han, Cao, Qin, White, Xu and Ma2019), whereas the others bind to the distal integrin NPXY/F motif and the preceding TTT/TTV/STF sequence (Morrison et al., Reference Morrison, MacPherson, Savinko, Lek, Prescott and Fagerholm2013; Li et al., Reference Li, Deng, Sun, Yang, Liu, Wang, Zhang, Lin, Wu, Wei and Yu2017). Whereas the talins can directly activate integrins by binding to the proximal NXXY/F sequence, the kindlins cannot do that, but they have to cooperate with talins and other proteins (Moser et al., Reference Moser, Legate, Zent and Fässler2009b). Integrins cannot extend when kindlin is absent. Kindlin-3 is difficult to express and purify, and therefore kindlin-2 has been used for molecular studies. Structural analysis shows that when it is bound to integrin tails, it combines two binding motifs (Kammerer et al., Reference Kammerer, Aretz and Fässler2017; Li et al., Reference Li, Deng, Sun, Yang, Liu, Wang, Zhang, Lin, Wu, Wei and Yu2017). Importantly, kindlin-2 forms a dimer and this is necessary for cell adhesion (Ye et al., Reference Ye, Petrich, Anekal, Lefort, Kasirer-Friede, Shattil, Ruppert, Moser, Fässler and Ginsberg2013a). Kindlin-3 is phosphorylated on T482 or S484. Mutation of the phosphorylated residues to alanine decreased binding and cell spreading on fibrinogen through the αIIbβ3 integrin (Bialkowska et al., Reference Bialkowska, Byzova and Plow2015). Furthermore, kindlin-2 activated the small G protein Rac1, and recruited the cytoskeletal Arp2/3 complex, enabling the formation of an actin network (Böttcher et al., Reference Böttcher, Veelders, Rombaut, Faix, Theodosiou, Stradal, Rottner, Zent, Herzog and Fässler2017). Taken together, the functional basis of kindlin-mediated activation of integrins is quite different from that of talin. Talin and kindlins work together in regulating adhesion. Interestingly, the minimal amount of kindlin-3 needed for platelet and leukocyte adhesion is only 5% of the normal levels. A gradual increase in kindlin-3, however, improved the integrin avidity in leukocytes (Bennett, Reference Bennett2015; Klapproth et al., Reference Klapproth, Moretti, Zeiler, Ruppert, Breithaupt, Mueller, Haas, Mann, Sperandio, Fässler and Moser2015a).
Filamins
The filamin family consists of three members (Stossel et al., Reference Stossel, Condeelis, Cooley, Hartwig, Noegel, Schleicher and Shapiro2001). Filamin A is a large homodimeric protein of about 280 kDa, linked at the COOH-terminals making a V-shaped structure. The monomers contain 24 Ig-domain like β-sheet repeats. It has been shown that domain-21 binds to integrin β2-chains between the two NP(L/K)F motifs (Liu et al., Reference Liu, Das, Yang, Ithychanda, Yakubenko, Plow and Qin2015a). This means that its binding site overlaps with those of talin and 14-3-3 proteins (Kiema et al., Reference Kiema, Lad, Jiang, Oxley, Baldassarre, Wegener, Campbell, Ylänne and Calderwood2006; Takala et al., Reference Takala, Nurminen, Nurmi, Aatonen, Strandin, Takatalo, Kiema, Gahmberg, Ylänne and Fagerholm2008; Chatterjee et al., Reference Chatterjee, Zhiping, Tan and Bhattacharjya2018b). Filamin also has a binding site in the α-chains (Fig. 6) (Liu et al., Reference Liu, Wang, Thinn, Ma and Zhu2015b). The migfilin protein binds directly to filamin (Takafuta et al., Reference Takafuta, Sacki, Fujimoto, Fujimura and Shapiro2003; Tu et al., Reference Tu, Wu, Shi, Chen and Wu2003). Filamin is considered to be an integrin inhibitor, and migfilin displaced filamin from integrin β-chains resulting in the activation of β1, β2, and β3 integrins (Das et al., Reference Das, Ithychanda, Qin and Plow2011). Interestingly, filamin phosphorylation was induced by G protein-coupled receptors by protein kinase A (Tirupula et al., Reference Tirupula, Ithychanda, Mohan, Prasad, Qin and Karnik2015). The phosphorylation on S2152 affected talin and kindlin-3 binding (Ithychanda et al., Reference Ithychanda, Fang, Mohan, Zhu, Tirupula, Prasad, Wang, Karnik and Qin2015; Waldt et al., Reference Waldt, Seifert, Demiray, Devroe, Turk, Reichardt, Mix, Reinhold, Freund, Muller, Schraven, Stork and Kliche2018).
The 14-3-3 proteins
The 14-3-3 proteins got their peculiar name from their presence in chromatography fractions, obtained during their isolation. They are dimeric serine/threonine-phosphate binding proteins (Muslin et al., Reference Muslin, Tanner, Allen and Shaw1996), but may also bind to non-phospho-proteins, although more weakly (Petosa et al., Reference Petosa, Masters, Bankston, Pohl, Wang, Fu and Liddington1998). They bind with high affinity to the pTTT phosphorylated β2-chain. The binding was also influenced by the sequence surrounding the phosphorylation site (Campbell, Reference Campbell2013). The 14-3-3 proteins also interact with the β1- and β3-cytoplasmic sequences 776–790 in a phosphorylation-dependent manner (Han et al., Reference Han, Rodriguez and Guan2001).
α-Actinin
α-Actinin is a cytoskeletal protein formed by two identical 100 kDa polypeptides. It mediates the binding of proteins to the actin cytoskeleton, among them ICAMs and integrins. α-Actinin binds to the integrin β-chain upstream of the proximal NXXY/P sequence (Fig. 6) (Pavalko and LaRoche, Reference Pavalko and LaRoche1993). Using conformation-specific monoclonal antibodies, Stanley et al. showed that the extended, but closed LFA-1, which binds the monoclonal antibody KIM127 epitope, is at the leading edge of a migrating cell and bound to the cytoskeleton through α-actinin (Stanley et al., Reference Stanley, Smith, McDowall, Nicol, Zicha and Hogg2008).
Cytohesin-1
Cytohesin-1 is a G protein exchange factor. The binding site is at the WKA motif in the most proximal part of the β2 cytoplasmic chain. Expression of a β2-chain mutated at this position reduced adhesion and cell spreading in inside-out activation (Kolanus, Reference Kolanus2007). Outside-in signaling in Jurkat T cells, expressing this β2 mutant, resulted in threonine phosphorylation of the protein, whereas signaling through the T cell receptor (TCR) did not (Perez et al., Reference Perez, Mitchell, Jager, South, Murriel, McBride, Herzenberg, Kinoshita and Nolan2003).
Dok1
The adaptor protein Dok1 contains a pleckstrin domain and a PTB domain. It binds to the integrin β-chains through the proximal NXXY/F motif with a higher affinity than that of talin (Oxley et al., Reference Oxley, Anthis, Lowe, Vakonakis, Campbell and Wegener2008). The binding increased when Y747 in β3 was phosphorylated. Dok1 bound strongly to the phosphorylated S745 and S756 in β2, but poorly to pT758 (Gupta et al., Reference Gupta, Chit, Feng, Bhunia, Tan and Bhattacharjya2015).
ICAP-1
ICAP-1 is a β1 integrin binding protein, which binds to the valine residue close to the proximal NPIY sequence and competes with talin and kindling-3 for binding. It regulates the formation of focal adhesions (Chang et al., Reference Chang, Wong, Smith and Liu1997).
Integrin α-tail binding cytoplasmic proteins
Rather few cytosolic proteins have been shown to bind to integrin α-chains.
CD45 tyrosine phosphatase
The CD45 tyrosine phosphatase, first identified as ‘common leukocyte antigen’ (Gahmberg et al., Reference Gahmberg, Häyry and Andersson1976; Andersson and Gahmberg, Reference Andersson and Gahmberg1978; Trowbridge, Reference Trowbridge1978; Thomas, Reference Thomas1989), is a major cell surface glycoprotein in leukocytes, which due to alternative splicing of the cell surface part expresses several isoforms. It contains two phosphatase domains in the cytoplasm, but only the proximal one is catalytically active. CD45 has an important function in the dephosphorylation of the COOH-terminal tyrosine phosphate present in the cytoplasmic Src family tyrosine kinase family members including Src, Lck, and Fyn. The dephosphorylation activates the Src family kinases (Mustelin et al., Reference Mustelin, Coggeshall and Altman1989, Reference Mustelin, Pessa-Morikawa, Autero, Gassman, Gahmberg, Andersson and Burn1992). The effect of CD45 on the Src family kinases is, however, complicated. In Lck, Y394 is required for full activity, and the phosphate at this residue can also be removed by CD45 (Courtney et al., Reference Courtney, Amacher, Kadlecek, Mollenauer, Au-Yeung, Kuriyan and Weiss2017; Philipsen et al., Reference Philipsen, Reddycherla, Hartig, Gumz, Kästle, Kritikos, Poltorak, Prokazov, Turbin, Weber, Zuschratter, Schraven, Simeoni and Muller2017). Furthermore, Lck has a phosphosite at Y192, and mutation of this residue to alanine inhibited the association of CD45 with Lck. This resulted in an inability to dephosphorylate the COOH-terminal phosphate in the kinases (Courtney et al., Reference Courtney, Lo and Weiss2018). Therefore, there must exist a balance between the COOH-terminal inhibitory phosphorylation, the activity promoting one on Y394 and the phosphorylation on Y192. CD45 is also a component of the immunological synapse, where it is peripherally located (Johnson et al., Reference Johnson, Bromley, Dustin and Thomas2000; Chang et al., Reference Chang, Fernandes, Ganzinger, Lee, Siebold, McColl, Jönsson, Palayret, Harlos, Coles, Jones, Lui, Huang, Gilbert, Klenerman, Aricescu and Davis2016).
CD45 binds to the LFA-1 α-chain at the COOH-terminal amino acid sequence 1123–1145 (Geng et al., Reference Geng, Tang, Law and Tan2005). Importantly, it does not bind to αM or αX, the cytoplasmic domains of which are shorter, and quite different from the COOH-terminal domain of αL. Full length CD45 cytoplasmic domain bound relatively poorly to LFA-1, whereas the isolated membrane proximal part, including the first phosphatase domain, showed strong binding. The binding to αL did not affect the phosphatase activity. LFA-1 and CD45 may form a complex in the immunological synapse. Interestingly, a soluble cytoplasmic form of CD45 is found in plasma and it inhibits the activation of T cells (Puck et al., Reference Puck, Hopf, Modak, Majdic, Cejka, Blumi, Schmetterer, Arnold-Schrauf, Gerwien, Frederiksen, Thell, Leitner, Steinberger, Aigner, Seyerl-Jiresch, Zlabinger and Stöckl2017). It is derived from neutrophils and monocytes by proteolysis.
Rap1
Rap1 is a small G protein important in T cell adhesion (Bivona et al., Reference Bivona, Wiener, Ahearn, Silletti, Chiu and Philips2004). Cellular activation results in the localization of the protein to the plasma membrane by attachment of a geranyl-geranyl lipid. Rap1 binds weakly to the F0 domain of talin, but the interaction is stabilized when Rap1b in platelets is membrane-anchored (Zhu et al., Reference Zhu, Yang, Bromberger, Holly, Lu, Liu, Sun, Klapproth, Hirbawi, Byzova, Plow, Moser and Qin2017). Rap1 has two downstream effectors. In leukocytes, Rap1 binds to RIAM (Rap1-interacting adapter molecule), which in turn binds to talin and activates adhesion through β2 integrins (Calderwood, Reference Calderwood2015; Su et al., Reference Su, Wynne, Pinheiro, Strazza, Mor, Montenont, Berger, Paul, Bergmeier, Gertler and Philips2015; Lagarrique et al., Reference Lagarrique, Kim and Ginsberg2016). RIAM is normally auto-inhibited by its N-terminal segment. The auto-inhibition can be released by phosphorylation of Y45 by FAK (focal adhesion kinase) (Chang et al., Reference Chang, Su, Cho, Zhang, Huang, Philips and Wu2019). Upon TCR activation in Jurkat cells, RIAM translocated to the plasma membrane, and this could be inhibited by FAK inhibitors. The Rap1 binding protein, RAPL, is implicated in leukocyte adhesion, and binds to lysines K1097 and K1099 in the αL chain. This binding is critical for LFA-1 conformational changes, and binding to ICAM-1 (Tohyama et al., Reference Tohyama, Katagiri, Pardi, Lu, Springer and Kinashi2003). In contrast, α4β1 binding to VCAM-1 was only weakly affected by RIAM deficiency (Klapproth et al., Reference Klapproth, Sperandio, Pinheiro, Prunster, Soehnlein, Gertler, Fässler and Moser2015b), and platelet adhesion was not affected by RIAM deficiency (Stritt et al., Reference Stritt, Wolf, Lorenz, Vogtle, Gupta, Bosl and Nieswandt2015; Bromberger et al., Reference Bromberger, Klapproth, Rohwedder, Zhu, Mittmann, Reichel, Sperandio, Qin and Moser2018). Recent work shows that Rap1 binds to the F1 domain in the talin head and activates the αIIbβ3 integrin. Interestingly, the F1 domain activity is due to the formation of a helix when bound to membrane lipids (Gingras et al., Reference Gingras, Lagarrique, Cuevas, Valadez, Zorovich, McLaughlin, Lopez-Ramirez, Seban, Ley, Kiosses and Ginsberg2019). Rap1 binds to RAPL and affects leukocyte adhesion, whereas no platelet effects have been reported (Katagiri et al., Reference Katagiri, Maeda, Shimonaka and Kinashi2003). RAPL acts as an integrin transporter, whereby LFA-1 localizes to the leading edge of migrating T cells.
The results show that different integrins and cells use Rap1, RIAM, RapL, and talin in different ways. Interestingly, the S/T Mst1 kinase is bound to RAPL, but its possible function is still not understood (Katagiri et al., Reference Katagiri, Imamura and Kinashi2006).
Calreticulin
The cytosolic protein calreticulin (Krause and Michalak, Reference Krause and Michalak1997) binds the KXGFFKR conserved motif found in the proximal part of integrin α-chains (Coppolino et al., Reference Coppolino, Leung-Hagesteijn, Dedhar and Wilkins1995). Treatment of the Jurkat T cell line with phorbol esters increased the calreticulin association to the α2β1 integrin. Integrins regulate Ca++ transport into cells, and crosslinking of the VLA-4 integrin with monoclonal antibodies induced Ca++ spikes, whereas knockout of calreticulin resulted in the abrogation of extracellular Ca++ transport into cells (Coppolino et al., Reference Coppolino, Woodside, Demaurex, Grinstein, St-Arnaud and Dedhar1997).
Paxillin
Paxillin binds to a sequence around E983 and Y991 in the integrin α4 chain. Mutational analysis of amino acid residues in this region showed that S998A mutation resulted in enhanced binding of paxillin, whereas the S988D mutation abrogated binding and cell migration, but increased cell spreading (Liu and Ginsberg, Reference Liu and Ginsberg2000). Very recent work indicates that kindlin-3 recruits leupaxin to podosomes and regulates paxillin phosphorylation and thereby podosome turnover (Klapproth et al., Reference Klapproth, Bromberger, Turk, Kruger and Moser2019).
SHARPIN
SHARPIN (Shank-Associated RH Domain Interactor) negatively regulates β1, β2, and β3 integrins and binds to the three amino acids N-terminal to the conserved GFFKR sequence in integrin α-chains (Rantala et al., Reference Rantala, Pouwels, Pellinen, Veltel, Laasola, Mattila, Potter, Duffy, Sundberg, Kallioniemi, Askari, Humphries, Parsons, Salmi and Ivaska2011; Pouwels et al., Reference Pouwels, de Franceschi, Mattila, Potter, Sundberg, Hogg, Gahmberg, Salmi and Ivaska2013). Recent work shows that it binds even more strongly to the β1 chain and more weakly to the β3 chain. Importantly, the binding site involves the proximal NPIY sequence. Talin binds to the same site and binding of the talin head domain was out-competed by SHARPIN (Gao et al., Reference Gao, Bao, Ge, Sun, Sun, Liu, Chen, Han, Cao, Qin, White, Xu and Ma2019). Furthermore, SHARPIN binds to kindlin-1, but not to kindlin-2 or -3. Importantly, SHARPIN may in this way bring kindlin-1 to the β1 binding site and inhibit integrin activation. SHARPIN interacted with activated Rap1 and R-Ras, and inhibited downstream signaling (Lilja et al., Reference Lilja, Zacharchenko, Georgiadou, Jacquemet, De Franceschi, Peuhu, Hamidi, Pouwels, Martens, Nia, Beifuss, Boeckers, Kreienkamp, Barsukov and Ivaska2017). SHARPIN is also involved in the formation of the ubiquitin chain complex, and it binds to the HOIP E3 ubiquitin ligase (Kasirer-Friede et al., Reference Kasirer-Friede, Tjahjono, Eto and Shattil2019).
Preferential binding of proteins to clasped integrin cytoplasmic domains
In contrast to most cytosolic proteins, binding preferentially to either integrin β- or α-chains, the L-plastin (LCP1) protein prefers binding to the interacting, clasped cytoplasmic integrin domains (Tseng et al., Reference Tseng, Samarelli, Kammerer, Scholze, Ziegler, Immier, Zent, Sperandio, Sanders, Fässler and Böttcher2018). It was shown to compete with talin for binding. Filamin is also known to bind to inactive, clasped integrins (Liu et al., Reference Liu, Das, Yang, Ithychanda, Yakubenko, Plow and Qin2015a).
Integrin activity is regulated by inside-out or outside-in signaling
Most experimental models on integrin activation are based either on T lymphocytes or platelets. In contrast to most cells, these cells are quiescent without activation, and therefore it is possible to observe changes in integrin-related functions after activating them. Lymphoblasts, fibroblasts, and glial cells, for example, are continuously dividing and their integrins already activated at least to some extent.
A description of integrin signaling, leading to the activation of adhesion, can be done in different ways. Here we first describe how cellular signaling takes place before reaching the integrins, how the integrins are modified, and the integrin–cytoplasmic interactions and signaling may take place downstream from the activated integrins.
In inside-out signaling, integrins become activated through the binding of agonists to non-integrin receptors (Giancotti and Ruoslahti, Reference Giancotti and Ruoslahti1999; Hynes, Reference Hynes2002; Gahmberg et al., Reference Gahmberg, Fagerholm, Nurmi, Chavakis, Marchesan and Grönholm2009; Hogg et al., Reference Hogg, Patzak and Willenbrock2011). These can be cell surface proteins, such as the TCR, or chemokine receptors, but it is also possible that activation takes place by binding of intracellular proteins due to changes in the environment, for example, high temperature. In outside-in signaling, ligands are bound directly to the integrins, which then signal into the cell. In integrin crosstalk, an activated or inhibited integrin communicates with other integrins in a given cell by intracellular signaling. Integrin inside-out activation, resulting in ligand binding, may subsequently be followed by outside-in signaling, due to interaction of external ligands with the integrins.
Interestingly, T cells in the blood stream can migrate either upstream or downstream depending on to which ligand they bind. When the cells bind to ICAM-1, they migrate upstream, but when bound to VCAM-1, they migrate downstream (Dominguez et al., Reference Dominguez, Anderson and Hammer2015). At higher shear rates, the cells migrate upstream even in the presence of small amounts of ICAM-1 and substantial levels of VCAM-1. The results show that outside-in signaling acts in different ways depending on which ligand–integrin interactions take place.
During the past few years, it has become increasingly apparent that integrins can be regulated by mechanical forces acting on integrins. In leukocyte trafficking, the integrins can form slip bonds, which may be broken by shear force, but also catch bonds, which in contrast become stronger under shear force (Sun et al., Reference Sun, Guo and Fässler2016). In both inside-out and outside-in signaling, conformational changes in the integrins occur, enabling them to become adhesive (Springer and Sen, Reference Springer and Sen2016). The distance from the ligand binding site to the lipid membrane is in the range of 200 Å for the extended integrins, and it is intriguing to speculate how the information flows between the adhesion sites and the cytoplasmic domains. In addition to the conformational changes, resulting in increased integrin affinity for ligands, an important feature is the clustering of the integrins in the plane of the membrane. This in turn will increase integrin avidity. In this case, the role of the actin cytoskeleton is of pivotal importance (Li and Springer, Reference Li and Springer2017a, Reference Li and Springer2017b). Obviously, a combination of both affinity and avidity increase results in highly efficient integrin binding.
A number of studies show that the hybrid domain in the external part of the β-chains swings out upon activation, concomitantly with a down-movement of the α7 helix in the α-chain I domain or in the β I-like domain in the integrins lacking I domains. The external integrin structure then opens, and this is coupled to a separation of the cytoplasmic domains of the α- and β-chains (Vinogradova et al., Reference Vinogradova, Velyvis, Velyviene, Hu, Haas, Plow and Qin2002; Kim et al., Reference Kim, Carman and Springer2003; Tng et al., Reference Tng, Tan, Ranganathan, Cheng and Law2004). Mn2+ ions can activate the binding capacity for external ligands by stabilizing the extended open conformation (Sen et al., Reference Sen, Koksal, Yuki, Wang and Springer2018), but the ion-induced activity is not seen inside the cell because of the separation of the cytoplasmic tails. These facts show that integrin signaling is unusually complex. Furthermore, we should realize that integrins are different, belonging to several subclasses. Therefore, the mechanisms regulating their functions are different, in spite of many common features.
The cytoplasmic domains of the integrin β polypeptides are homologous, indicating that their functions are relatively similar. Opposite to that, the α-chains are quite different in structure. This may reflect the fact that the α-chains are primarily responsible for the binding of different ligands, which needs specificity, whereas the β-chains are more involved in integrin activity regulation.
Below we try to describe what we currently know about integrin activity regulation, but we realize that we still are in the very beginning of an understanding of this fascinating, but very complex subject. The reader is encouraged to get familiar with the previous original work. However, during the past few years, we have seen a dramatic development in the understanding of cell adhesion and its multifaceted features.
Upstream events in integrin inside-out activation
T lymphocytes are excellent model cells for the study of integrin activity regulation. One reason is that we know a lot about T cells, due to their importance in immunology. Activation can take place through the TCR or chemokine receptors. Each T cell has a TCR of unique specificity due to the rearrangement of the DNA during development. When T cells encounter cells presenting antigen peptides bound to class I or class II transplantation antigens, the T cells must recognize whether the complex is self or novel. Further activation then needs the assembly of a TCR signaling complex for downstream signaling (Courtney et al., Reference Courtney, Lo and Weiss2018). Associated with the TCR are the CD3 molecules, which are pivotal for signaling. An efficient way to induce extensive signaling, and circumvent-specific T cell antigen-restricted signaling, is by crosslinking the CD3 proteins and the associated TCR with anti-CD3 antibodies (Fig. 7). The CD3 molecules contain in their cytoplasmic domains sequence motifs called Immune-receptor-Tyrosine-based-Activation-Motifs (ITAMs), which act as substrates for the cytoplasmic tyrosine kinase Lck. The phosphorylated ITAM then binds to the Zap70 tyrosine kinase through its SH2 domains. Zap70 becomes activated and phosphorylates the linker for the activation of T cells (LAT). This results in the binding of phospholipase Cϒ1. Then phosphatidylinositol-3 kinase is recruited and phosphorylates phosphatidylinositol 4,5-bisphosphate (PIP2) to yield PIP3. This brings in turn the ITK kinase to the complex, which phosphorylates PLCϒ1. PLCϒ1 then hydrolyzes PIP2 resulting in IP3 and diacylglycerol (DAG). IP3 then binds to its intracellular receptor resulting in the release of Ca++. Cellular Ca++ can also increase upon activation through the binding of the cytoplasmic protein calreticulin to the conserved KXFFKR motif in the proximal part of integrin α-chains. The Ca++ increase was indistinguishable in thapsigargin-treated wild type and calreticulin knockout cells (Coppolino et al., Reference Coppolino, Woodside, Demaurex, Grinstein, St-Arnaud and Dedhar1997). Because the drug releases Ca++ by inhibiting the endoplasmic reticulum Ca++-ATPase, the Ca++ must come from extracellular sources (Coppolino et al., Reference Coppolino, Woodside, Demaurex, Grinstein, St-Arnaud and Dedhar1997).

Fig. 7. LFA-1 activation through the TCR. Activation takes place by activation of the CD3-associated molecules through the Lck tyrosine kinase, followed by ZAP70 kinase activation, phosphorylation of LAT, and activation of PLCϒ1. This results in the generation of DAG and IP3. DAG activates PKC enzymes, which phosphorylate T758 in the β2 chain. IP3 activates Ca2+ release. Calcineurin is recruited to the TCR complex, where it may release the inhibitory phosphate on S59 in Lck, activate the kinase. In β1 and β3 integrins, calreticulin is known to bind to the α-chains, but this has not been shown for β2.
DAG activates protein kinase C enzymes (PKC) (Castagna et al., Reference Castagna, Takai, Kaibuchi, Sano, Kikkawa and Nishizuka1982; Nishizuka, Reference Nishizuka1984; Newton, Reference Newton1995), which phosphorylate the integrin β2-chain (Fagerholm et al., Reference Fagerholm, Morrice, Gahmberg and Cohen2002b). The above scheme is based on the studies of T cell activation, which obviously results in several molecular changes in the cells, among which integrin activation is only one.
On the external face of the TCR, the immunological synapse is formed (Fig. 8) (Grakoui et al., Reference Grakoui, Bromley, Sumen, Davis, Shaw, Allen and Dustin1999; Bromley et al., Reference Bromley, Burack, Johnson, Somersalo, Sims, Sumen, Davis, Shaw, Allen and Dustin2001). The TCR is located in the center with LFA-1 in the periphery. PKCθ localizes to the central supramolecular activation cluster, where it mediates effector T cell activation (Kong and Altman, Reference Kong and Altman2013). CD45 is more peripherally located. CD45 activates Lck and the related tyrosine kinases by releasing the COOH-terminal tyrosine phosphate. The Csk kinase in turn inactivates Lck by phosphorylating the COOH-terminal of Lck, resulting in an intramolecular loop between the SH2 domain of Lck and its COOH-terminal. On the other hand, phosphorylation of Y394 in Lck is needed for Lck activation and also this phosphate can be released by CD45. Most probably, the accessibility of CD45 to Lck is important in the regulation of the synapse and the subsequent influence on integrin activity (Fagerholm et al., Reference Fagerholm, Hilden and Gahmberg2002a).

Fig. 8. The immunological synapse. The synapse is formed between T cells and antigen presenting cells. The specific interaction is due to TCR and MHC-peptide recognition in the center. The synapse is strengthened by LFA-1/ICAM-1 interaction in the peripheral part. The CD45 tyrosine phosphatase must be close to Lck to be able to regulate its activity.
Calcineurin is an important serine/threonine phosphatase known to act on T cell NFAT transcription factors. It is activated by calmodulin, which is up-regulated by cytoplasmic Ca++ (Ye et al., Reference Ye, Feng, Yin, Faucher, Currie, Rahman, Jin, Li, Wei and Jia2013b). Calmodulin activates leukocyte adhesion (Fagerholm et al., Reference Fagerholm, Prescott, Cohen and Gahmberg2001). Intracellular Ca++ is important in the regulation of integrin activity, and interestingly, the intracellular Ca++ was increased by leukocyte integrin activation (Altieri et al., Reference Altieri, Stamnes and Gahmberg1992). Further studies showed that the β-chain of LFA-1 is critical for Ca++ regulation. The phenylalanine in the distal NPKF sequence was required for Ca++ signaling and subsequent integrin activation (Sirim et al., Reference Sirim, Zeitlmann, Kellersch, Falk, Schendel and Kolanus2001). Inhibition of calcineurin by cyclosporine A or FK506 is widely used in the treatment of transplantation rejection. It was recently reported that calcineurin is in fact recruited to the TCR complex, where it released the inhibitory phosphate on S59 in Lck (Dutta et al., Reference Dutta, Barr, Akpan, Mittelstadt, Singha, Samelson and Ashwell2017). The treatment inhibited LFA-1, which indicates that the effects of the drug are at least in part due to the inhibition of T cell adhesion.
Chemokines, leukotrienes, and kallikreins are deposited on endothelial cells lining the vasculature and trigger the activation of rolling leukocytes, including T cells (Alon and Feigelson, Reference Alon and Feigelson2012; Vestweber, Reference Vestweber2015) (Fig. 9). The chemokine receptors are G-protein-coupled receptors and induce signaling by activating PLCs, which results in the generation of inositol-1,4,5-trisphosphate and DAG (Zarbock et al., Reference Zarbock, Ley, McEver and Hidalgo2011; Strazza et al., Reference Strazza, Azoulay-Alfaguter, Peled, Smrcka, Skolnik, Srivastava and Mor2017). The former compound releases Ca++ from intracellular stores and DAG activates PKCs. Chemokines are also stored within endothelial cells and are released during leukocyte migration through the vessel walls (Shulman et al., Reference Shulman, Cohen, Roediger, Kalchenko, Jain, Grabovsky, Klein, Shinder, Stoler-Barak, Feigelson, Nurmi, Goldstein, Hartley, Gahmberg, Etzioni, Weninger, Ben-Baruch and Alon2012).

Fig. 9. Activation of LFA-1 through chemokines. After binding of chemokines to the receptors, PLCs become activated and generate DAG and IP3. DAG activates PKCs, which phosphorylate T758 in β2. On the other hand, the Cal-DAG G protein exchange factor activates Rap1. Rap1-GTP binds RapL, which binds to the αL-chain. In addition, RIAM binds to talin and the β2-chain.
The small G protein Rap1 is activated by its exchange factors such as CALDAG-GEFI by TCR or chemokine-induced signaling cascades. Rap1 is brought to the membrane by RapL and in migrating cells to the leading edge (Hogg et al., Reference Hogg, Patzak and Willenbrock2011). Activated Rap1 activates LFA-1, whereas its down-regulation inhibits T cell adhesion. Importantly, inhibition of the interaction with RIAM, and in RIAM depleted mice, the T and B lymphocytes could not traffic to peripheral lymph nodes, due to down regulation of LFA-1 and VLA-4 activities. In contrast, macrophages showed no defect in adhesion or spreading. The results show that different blood cells differently regulate their integrins.
The Del-1 (developmental endothelial locus-1) protein is a natural inhibitor of leukocyte/endothelial interactions. It is a soluble protein that becomes attached to blood vessel endothelial proteoglycans or the αVβ3 integrin through its RGD sequence (Choi et al., Reference Choi, Chavakis, Czabanka, Langer, Fraemohs, Economopoulou, Kundu, Orlandi, Zheng, Prieto, Ballantyne, Constant, Aird, Papayannopoulou, Gahmberg, Udey, Vajkoczy, Quartermous, Dimmeler, Weber and Chavakis2008a; Schurpf et al., Reference Schurpf, Chen, Liu, Wang, Springer and Wang2012; Mitroulis et al., Reference Mitroulis, Kang, Gahmberg, Siegert, Hajishengallis, Chavakis and Choi2014). It competes with ICAMs for the binding to LFA-1 and Mac-1 and therefore inhibits leukocyte binding to the ligands. Del-1 is strongly expressed in the brain and lung, but almost absent from the liver.
Upstream events in integrin outside-in activation
Outside-in activation of integrins takes place through agonist binding to the external domains of integrins (Abram and Lowell, Reference Abram and Lowell2009). It may also be a consequence of inside-out signaling, and it is then difficult to distinguish the two types of signaling. The two types of signaling may even take place at the same time. Mn2+ is an efficient activator of adhesion if it binds to the MIDAS site on the external aspect of cells (Gailit and Ruoslahti, Reference Gailit and Ruoslahti1988), but it is probably, however, not physiologically important for integrin activity. Soluble ligands, such as recombinant ICAM-2 lacking the transmembrane and cytoplasmic domains (Kotovuori et al., Reference Kotovuori, Pessa-Morikawa, Kotovuori, Nortamo and Gahmberg1999), integrin binding peptides (Li et al., Reference Li, Nortamo, Kantor, Kovanen, Timonen and Gahmberg1993; Koivunen et al., Reference Koivunen, Ranta, Annila, Taube, Uppala, Jokinen, van Willigen, Ihanus and Gahmberg2001), integrin antibodies, and immobilized fibrinogen, have been used to demonstrate outside-in signaling in leukocytes and platelets (Shattil et al., Reference Shattil, Kashiwagi and Pampori1998; Tan et al., Reference Tan, Robinson, Drbal, van Kooyk, Shaw and Law2001). Both integrin ligand binding activating and inhibitory antibodies can induce outside-in signaling, whereas antibodies binding to integrins, but which do not affect ligand binding, do not induce signaling (Grönholm et al., Reference Grönholm, Jahan, Bryushkova, Madhavan, Aglialoro, Hinojosa, Uotila and Gahmberg2016).
Outside-in signaling is in part different from that of inside-out signaling. The most studied integrin in this respect is the platelet integrin αIIbβ3 (Fig. 10). A number of ligands, including fibrinogen, von Willebrand factor, and fibronectin, bind to the integrin through the classical arginine–glycine–aspartic acid (RGD) sequence (Durrant et al., Reference Durrant, van den Bosch and Hers2017). Fibrinogen also binds through the KQAGDV sequence in the ɤ-chain (Humphries et al., Reference Humphries, Byron and Humphries2006). Using peptides interacting with the αIIb transmembrane domain, Fong et al. (Reference Fong, Zhu, Span, Moore, Yoon, Tamura, Yin, DeGrado and Bennett2016) induced activation by the activation of Src and Syk. The integrin became clustered through the signaling. Importantly, Glanzmann's thrombasthenia is due to mutations in this integrin. An early event in αIIbβ3 activation is the up-regulation of Src tyrosine kinase activity. Src is highly expressed in platelets and binds by the SH3 domain to the β3 cytoplasmic domain COOH-terminal RGT motif (Wu et al., Reference Wu, Span, Nygren, Zhu, Moore, Cheng, Roder, DeGrado and Bennett2015). Src phosphorylates Y747 and Y759 in the NXXY sequences of β3 (Jenkins et al., Reference Jenkins, Nannizzi-Alaimo, Silver, Sellers, Ginsberg, Law and Phillips1998). Incidentally, the corresponding residues in β2 are phenylalanines, which cannot be phosphorylated. Lck may have a similar function as Src in lymphocytes, but its target sites must be different. Y735 in β2 is a potential phosphorylation site, and a Y735A mutation resulted in decreased internalization of LFA-1 in transfected cells (Bleijs et al., Reference Bleijs, van Duijnhoven, van Vliet, Thijssen, Figdor and van Kooyk2001). Src activation results in the phosphorylation of the Syk tyrosine kinase and its binding to the β3 polypeptide (Obergfell et al., Reference Obergfell, Eto, Mocsai, Buensuceso, Moores, Brugge, Lowell and Shattil2002). Src also activates PLCϒ2 (Wonerow et al., Reference Wonerow, Pearce, Vaux and Watson2003), and its activation can also be supported by the Bruton's tyrosine kinase (Shattil and Newman, Reference Shattil and Newman2004). PLCϒ2 catalyzes the formation of IP3 and DAG, resulting in an increase of intracellular Ca++ and activation of PKCs.

Fig. 10. A simplified view of αIIbβ3 and LFA-1 activation by outside-in signaling. Ligands such as fibrinogen bind to the outside and activate Src family kinases, which in turn activate focal adhesion kinase and Syk by tyrosine phosphorylation. In LFA-1, the binding of external ligands induces the phosphorylation of T758 in the β-chain followed by binding of 14-3-3 proteins, Tiam1, and activation of Rac1. It remains unclear when talin and kindlin interact with the integrin, but results support the proposal that it is important in the final activation of integrins.
FAK is also activated upon αIIbβ3 activation, initially by autophosphorylation of Y397, which provides a docking site for Src, which then phosphorylates FAK on multiple sites and promotes its activity. The homolog Pyk2 may have similar effects in cells lacking FAK, such as macrophages (Okigaki et al., Reference Okigaki, Davis, Falasca, Harroch, Felsenfeld, Sheetz and Schlesinger2003). FAK may be important in integrin outside-in activation, and in colon cancer epithelial cells, FAK inhibition decreased metastasis through integrins (Downey-Biechler et al., Reference Downey-Biechler, Craig, More and Basson2019).
Protein interactions at the integrin cytoplasmic domains are pivotal for cell adhesion and adhesion-dependent functions
Integrins are involved in a number of different cellular functions, but they are all associated with cell adhesion. This is true for cell movement, cellular cytotoxicity, phagocytosis, antibody production, synaptic transmission, and as receptors for microbes. Most if not all of these functions involve signaling across the plasma membrane. The integrin cytoplasmic domains are 20–60 amino acids long (an exception is β4, which is longer), and they have no enzymatic activity. Therefore, to assert functions, it is necessary to assemble complexes of different signaling and interacting proteins at their cytoplasmic domains. Mammals have up to 24 integrins with different cellular distributions. A given cell may contain quite a few different integrins making it possible on one hand to express several different functions, but on the other hand, it has also become necessary to regulate the integrins in a useful way. Furthermore, the integrin activities must be coordinated temporally in order to function in a beneficial manner. One such example is cell migration. If some integrin activates movement, whereas others at the same time would act as brakes, the cell would be unable to move.
Currently, we are aware of many important interactions between integrins and cytoplasmic proteins, but we are still far from understanding how the regulatory mechanisms work. A lot of excellent science has been done on this topic, but one should emphasize that much of what currently is known has been done with different cells, different integrins, and under different conditions. Certainly, integrins are individuals, and facts applying to one integrin or integrin subfamily may not be true for others.
In the following, we focus on the role of β2-integrins in leukocyte adhesion, and when appropriate to some extent on other integrins. Leukocyte integrins are essential for a normal life, which is evident from the leukocyte adhesion deficiency syndromes. LAD-I is due to the absence of inhibitory mutations in the β2-polypeptide, LAD-II is due to deficiency in selectin function, and in LAD-III the integrin-binding protein, kindlin-3, is malfunctioning. Proteomic studies of integrin interacting proteins have identified a large number of proteins (Zaidel-Bar et al., Reference Zaidel-Bar, itzkovitz, Ma àyan, Iyengar and Geiger2007; Byron et al., Reference Byron, Humphries, Bass, Knight and Humphries2011; Needham et al., Reference Needham, Parker, Burykin, James and Humphrey2019). Several of these are members of adhesion complexes, adhesomes, and not directly interacting with integrins. In Fig. 6, the best characterized interacting proteins with LFA-1 are shown, and their binding sites indicated. A number of reviews are available on integrins and their cytoplasmic interactions, but recent progress has been substantial, and this fact merits an up-to-date review.
Integrin phosphorylation regulates integrin–cytoplasmic protein interactions
In early studies, Nishizuka and coworkers identified a group of enzymes named PKCs, which were found to be important for cell growth, morphology, and lipid metabolism. The group also found that phorbol esters strongly activate the kinases and have a similar role as the physiological lipid DAG (Castagna et al., Reference Castagna, Takai, Kaibuchi, Sano, Kikkawa and Nishizuka1982; Nishizuka, Reference Nishizuka1984). A total of 11 PKCs were identified and they could be divided into three groups. PKCs α, βI, βII, and ϒ are regulated by Ca++. The second group includes PKCs δ, ɛ, η, θ, and μ, and lack the C2 domain, which is thought to bind Ca++. The atypical PKCs are ξ and λ. The last two do not respond to phorbol esters (Newton, Reference Newton1995).
PKCs were first considered to be oncoproteins, because of their activation by phorbol esters, which act as tumor promoters. Surprisingly, more recent work indicates that they are in fact tumor suppressors, because they are commonly inactivated in tumors (Newton and Brognard, Reference Newton and Brognard2017). We will below discuss the possibility that restoration of PKC activity may in fact be beneficial in cancer treatment due to their agonist functions on integrins.
Because phorbol esters could induce leukocyte aggregation, and PKCs were known to act as receptors for these compounds, several research groups thought that it would be important to study the possibility that the adhesion receptors are phosphorylated. Using 32P-labelled leukocytes, it was shown that the α-chain of LFA-1 was phosphorylated in resting cells, whereas the β-chain was not (Chatila et al., Reference Chatila, Geha and Arnaout1989; Buyon et al., Reference Buyon, Slade, Reibman, Abramson, Philips, Weissmann and Winchester1990; Valmu et al., Reference Valmu, Autero, Siljander, Patarroyo and Gahmberg1991) (Fig. 5). After activation of 32P-labelled cells with phorbol esters, the β2-chain became phosphorylated. The major phosphorylated residue was S756. However, the mutation S756A did not affect leukocyte adhesion, whereas mutations of the threonine triplet T758-760 to alanines abrogated adhesion (Hibbs et al., Reference Hibbs, Jakes, Stacker, Wallace and Springer1991). However, no phosphorylation of T758-760 was observed, and the possible role of phosphorylation of LFA-1 remained enigmatic. We then found that upon activation of T cells, there is in fact strong threonine phosphorylation on the β2-chain, which was observed after the use of the phosphatase inhibitor okadaic acid (Valmu and Gahmberg, Reference Valmu and Gahmberg1995). The only threonines in the cytoplasmic domain of the β2-chain are T758, T759, and T760, and these must therefore be the targets of phosphate labeling.
We then used the integrin β2 cytoplasmic tail as bait, and PKCβ, PKCβII, and PKCδ were identified as phosphorylating enzymes (Fagerholm et al., Reference Fagerholm, Morrice, Gahmberg and Cohen2002b). With purified PKCs, the most efficient enzymes phosphorylating β2 peptides were PKCα, PKCη, PKCβI, PKCβII, and PKCδ. Most of them phosphorylated both serine and threonine residues in β2, and S745, S756, T758, T759, and T760 became phosphorylated.
Detailed studies in vivo showed that the β2-chain is phosphorylated on S745, S756, T758, and weakly on T759. Y735 may also be phosphorylated, but direct proof for this is not available. T758 was found to be most strongly labeled (Hilden et al., Reference Hilden, Valmu, Kärkkäinen and Gahmberg2003). The RACK1 protein acts as a receptor for PKCs (Lilienthal and Chang, Reference Lilienthal and Chang1998), and it binds to the β2-chain proximal region (Fig. 5). This could be a mechanism for bringing PKCs close to the integrins and phosphorylate them.
The phosphorylation of T758 in T cell LFA-1 resulted in the binding of the dimeric 14-3-3 protein, followed by the binding of the adaptor protein Tiam1, activation of the small G-protein Rac1, activation of cell adhesion, and increased expression of the activation marker CD69 (Fagerholm et al., Reference Fagerholm, Morrice, Gahmberg and Cohen2002b, Reference Fagerholm, Hilden, Nurmi and Gahmberg2005; Nurmi et al., Reference Nurmi, Autero, Raunio, Gahmberg and Fagerholm2007; Grönholm et al., Reference Grönholm, Jahan, Marchesan, Karvonen, Aatonen, Narumanchi and Gahmberg2011). The activation induced increased the binding of LFA-1 to the actin cytoskeleton (Valmu et al., Reference Valmu, Fagerholm, Suila and Gahmberg1999).
The α-chain phosphorylations were not considered to be important, because they were constitutively phosphorylated in resting cells, and the phosphorylation did not seem to change upon activation. There is, however, a dynamic phosphorylation and dephosphorylation of the α-chains, because radioactive phosphate labeling of αL was stronger in cells treated with okadaic acid than in its absence (Valmu and Gahmberg, Reference Valmu and Gahmberg1995). We determined the phosphorylation site in the αL-chain, and it turned out to be S1140. Surprisingly, mutation of this residue to alanine completely abrogated cell adhesion (Fagerholm et al., Reference Fagerholm, Hilden, Nurmi and Gahmberg2005). Corresponding mutations gave the same result for αM and αX (Fagerholm et al., Reference Fagerholm, Varis, Stefanidakis, Hilden and Gahmberg2006; Uotila et al., Reference Uotila, Aatonen and Gahmberg2013). αD has not been studied in this respect. We tried to find proteins interacting with αL in a phosphorylation-dependent manner, but were not able to find any. We then made the exciting discovery that in LFA-1 and αXβ2, the T758 phosphorylation on the β2-chain depends on α-chain phosphorylation (Jahan et al., Reference Jahan, Madhavan, Rolova, Viazmina, Grönholm and Gahmberg2018). Mutations of αL S1140A and αX S1158A inhibited the important β2-T758 phosphorylation, which is necessary for integrin activation. The mutation S1140D had a similar effect on T758 phosphorylation as the αL wt chain, indicating that the negative charge is important.
Phosphorylation of other integrins has been less studied. T788 and T789 in the β1 chain, which correspond to T758-T59 in β2, are likewise necessary for ligand binding, because mutations to alanine reduced binding to fibronectin, whereas mutations to aspartic acid behaved as wt β1. It induced, however, more focal contacts and less migration (Nilsson et al., Reference Nilsson, Kaniowska, Brakebusch, Fässler and Johansson2006). In the αVβ5 integrin, the S759 and S762 residues were phosphorylated by the p21-activated kinase 4. Mutation of these residues abolished cell migration (Li et al., Reference Li, Zhang, Lundin, Thullberg, Liu, Wang, Claesson-Welsh and Strömblad2010).
The VLA-4 (α4β1) integrin is an important leukocyte integrin, but it is also expressed in many other cells. It is phosphorylated on S988 in the α-chain and the S988A mutation inhibited cell spreading, but increased cell migration. The S988A mutation prevented the binding of paxillin, whereas the S988D mutation restored the activity to resemble that of wt integrin (Han et al., Reference Han, Rose, Woodside, Goldfinger and Ginsberg2003). The phosphorylated α4 in VLA-4 localized to the leading edge of migrating cells (Lim et al., Reference Lim, Kain, Tkachenko, Goldfinger, Gutierrez, Allen, Groisman, Zhang and Ginsberg2008).
In the platelet αIIbβ3 integrin, the β3 chain is phosphorylated on T753, which is the last threonine residue in the TST triplet between the NPXY sequences. It was phosphorylated upon treatment with the phosphatase inhibitor calyculin A, and the phosphorylation inhibited platelet adhesion upon activation by outside-in activation with fibrinogen (Lerea et al., Reference Lerea, Cordero, Sakariassen, Kirk and Fried1999; Kirk et al., Reference Kirk, Sanderson and Lerea2000).
The effect of outside-in activation on integrin phosphorylation of αIIbβ3 has shown that the tyrosines in the NPXY sequences become phosphorylated in platelets by the Src kinase (Jenkins et al., Reference Jenkins, Nannizzi-Alaimo, Silver, Sellers, Ginsberg, Law and Phillips1998; Law et al., Reference Law, DeGuzman, Heiser, Ministri-Madrid, Killeen and Phillips1999; Wu et al., Reference Wu, Span, Nygren, Zhu, Moore, Cheng, Roder, DeGrado and Bennett2015; Dutta et al., Reference Dutta, Barr, Akpan, Mittelstadt, Singha, Samelson and Ashwell2017).
In addition to integrins, a number of other cellular proteins become phosphorylated upon cellular activation including several cytoplasmic proteins, which are important for cell adhesion. These include talin (Ratnikov et al., Reference Ratnikov, Ptak, Han, Shabanowitz, Hunt and Ginsberg2005), filamin (Pons et al., Reference Pons, Izquierdo, Andreu-Carbo, Garrido, Planaguma, Muriel, del Pozo, Geli and Aragay2017), Rap1 (Takahashi et al., Reference Takahashi, Dillon, Liu, Kariya, Wang and Stork2013), paxillin (Klapproth et al., Reference Klapproth, Bromberger, Turk, Kruger and Moser2019), and kindlin-3 (Bialkowska et al., Reference Bialkowska, Byzova and Plow2015). The filamin domain-21 is poorly phosphorylated on S2152 in vitro by protein kinase A, but upon ligand binding, the filamin phosphorylation strongly increased (Ithychanda et al., Reference Ithychanda, Fang, Mohan, Zhu, Tirupula, Prasad, Wang, Karnik and Qin2015; Tirupula et al., Reference Tirupula, Ithychanda, Mohan, Prasad, Qin and Karnik2015). The possible importance of these phosphorylations remains poorly understood.
Cytoplasmic proteins compete for binding to integrin cytoplasmic tails
Both inside-out and outside-in signaling are accompanied by changes in cell adhesion, and it is well established that the intracellular integrin domains are of pivotal importance. In order to describe how integrins are regulated, we focus on LFA-1.
Figure 6 shows the binding sites of cytoplasmic proteins known to bind to the α- and β-chains of LFA-1, and which affect integrin functions. The binding sites in the cytoplasmic domains have been determined by deleting the parts of the chains or by mutational analysis. The β2 cytoplasmic domain is about 50 amino acids long, and several of the potential binding proteins are relatively large proteins. The interacting proteins have distinct functions needed for the cells. Therefore, regulation of binding must be important. One way of regulating protein binding to the β-chain is by phosphorylation, which strongly affects the binding of cytosolic proteins (Takala et al., Reference Takala, Nurminen, Nurmi, Aatonen, Strandin, Takatalo, Kiema, Gahmberg, Ylänne and Fagerholm2008; Gupta et al., Reference Gupta, Chit, Feng, Bhunia, Tan and Bhattacharjya2015; Chatterjee et al., Reference Chatterjee, Zhiping, Tan and Bhattacharjya2016, Reference Chatterjee, D'Souza, Zhang, Bin, Tan and Bhattacharjya2018a). Another way is accessibility. In the resting state, there is a salt bridge between D731 in the β2-chain and R995 in the αL chain. Deletion of the distal part of α-chains, which makes the salt bridge impossible, activates integrins (Liu et al., Reference Liu, Wang, Thinn, Ma and Zhu2015b). Phosphorylation on serine has been shown to compete with salt bridge formation in various protein–protein interactions (Skinner et al., Reference Skinner, Wang, Lee, Ong, Sommese, Sivaramakrishnan, Koelmel, Hirschbeck, Schindelin, Kisker, Lorenz, Sosnick and Rosner2017), and could be a potential mechanism to change integrin cytoplasmic interactions.
In resting cells, the cytoplasmic tails are closely associated with restricting protein binding. Some proteins, however, interact well with the β2-chain in resting cells. Filamin has been shown to bind to L753-P764 in the β2-chain (Kiema et al., Reference Kiema, Lad, Jiang, Oxley, Baldassarre, Wegener, Campbell, Ylänne and Calderwood2006; Takala et al., Reference Takala, Nurminen, Nurmi, Aatonen, Strandin, Takatalo, Kiema, Gahmberg, Ylänne and Fagerholm2008; Chatterjee et al., Reference Chatterjee, Zhiping, Tan and Bhattacharjya2018b). Interestingly, it also binds to integrin α-chains (Liu et al., Reference Liu, Das, Yang, Ithychanda, Yakubenko, Plow and Qin2015a). Filamin could in this way form an integrin cytoplasmic domain clasp. Recent work shows that filamin is phosphorylated by the Ndr2 protein kinase on S2152 in vivo after activation of the TCR (Waldt et al., Reference Waldt, Seifert, Demiray, Devroe, Turk, Reichardt, Mix, Reinhold, Freund, Muller, Schraven, Stork and Kliche2018). The activation through the TCR results in the release of filamin from LFA-1. Structural analysis showed how the integrin binding motif in filamin domain-21 interacts with the β2-cytoplasmic domain (Takala et al., Reference Takala, Nurminen, Nurmi, Aatonen, Strandin, Takatalo, Kiema, Gahmberg, Ylänne and Fagerholm2008). When T758 is phosphorylated, the phosphorylated peptide cannot be accommodated within the filamin pocket and it is released. Filamin is an integrin inhibitor, because it occupies sites important for the binding of activating proteins. It should not, however, be considered only as an inhibitor. Its interactions may also organize the integrin cytoplasmic tails into a structure facilitating activation when appropriate.
Dok1 is a cytosolic protein binding in the resting state to the proximal NPLY sequence in β3 (Oxley et al., Reference Oxley, Anthis, Lowe, Vakonakis, Campbell and Wegener2008). The binding region partially overlaps with that of talin. Talin bound more strongly to the unphosphorylated sequence than Dok1, but upon activation and tyrosine phosphorylation of the NPLY sequence, the binding of Dok1 strongly increased (Oxley et al., Reference Oxley, Anthis, Lowe, Vakonakis, Campbell and Wegener2008). Dok1 bound weakly to β2 integrin cytoplasmic peptides, but when the S745 and S756 residues were phosphorylated, the binding increased (Gupta et al., Reference Gupta, Chit, Feng, Bhunia, Tan and Bhattacharjya2015). When cells were activated through the TCR or chemokine receptors, the high affinity of 14-3-3 proteins for the phosphorylated site outcompeted talin binding from its distal binding site, and enabled a molecular switch. In β3, the binding from the phosphorylated tyrosine in the NPLY sequence switched to a complex of β3/14-3-3/Dok1. In β2, when S756 was phosphorylated in β2, the complex β2/14-3-3/Dok1 was formed (Chatterjee et al., Reference Chatterjee, Zhiping, Tan and Bhattacharjya2016, Reference Chatterjee, D'Souza, Zhang, Bin, Tan and Bhattacharjya2018a). Activation through the TCR does not result in the phosphorylation of S756, but it becomes phosphorylated by phorbol ester treatment. In the β2 integrin Mac-1, S756 phosphorylation regulates Rap1 activity, which is important for phagocytosis (Lim et al., Reference Lim, Hotchin and Caron2011).
Kindlin-3 is confined to hematopoietic cells, and its genetic absence results in the LAD-III syndrome due to defects in adhesion (Malinin et al., Reference Malinin, Zhang, Choi, Ciocea, Razorenova, Ma, Podrez, Tosi, Lennon, Caplan, Shurin, Plow and Byzova2009; Svensson et al., Reference Svensson, Howarth, McDowall, Patzak, Evans, Ussar, Moser, Metin, Fried, Tomlinson and Hogg2009; Moser et al., Reference Moser, Bauer, Schmid, Ruppert, Schmidt, Sixt, Wang, Sperandio and Fässler2009a, Reference Moser, Legate, Zent and Fässler2009b). Studies on kindlin-2 show that its expression is regulated by the Smurf1 ubiquitin kinase (Wei et al., Reference Wei, Wang, Zhan, Chen, Fang, Zhang and Zhang2017), and kindlin-3 expression may also be regulated by the same mechanism. Like talin, the kindlins contain a FERM domain. Kindlins-2 and -3 function as coactivators of integrins together with talin, but how the cooperation takes place has remained incompletely understood (Calderwood et al., Reference Calderwood, Campbell and Critchley2013; Kondo et al., Reference Kondo, Ueda, Kita, Ozawa, Tomiyama, Yasuda, Lim and Kinashi2017). Kindlin-1 may be inhibitory. The recent finding that kindlins form dimers may favor integrin cluster formation, resulting in increased avidity (Kammerer et al., Reference Kammerer, Aretz and Fässler2017; Li et al., Reference Li, Deng, Sun, Yang, Liu, Wang, Zhang, Lin, Wu, Wei and Yu2017). Talin cannot do that, but may form dimers in an antiparallel way through its COOH-terminals. Kindlin-2 is most easily expressed and purified of the kindlins. It has therefore been used for studies on kindlin structure and interactions. It directly binds to actin, which could explain that clustering occurs (Bledzka et al., Reference Bledzka, Bialkowska, Sossey-Alaoui, Vaynberg, Pluskota, Qin and Plow2016). In addition, kindlin-2 binds paxillin and the Arp2/3 complex (Böttcher et al., Reference Böttcher, Veelders, Rombaut, Faix, Theodosiou, Stradal, Rottner, Zent, Herzog and Fässler2017). Paxillin is known to bind to integrin α-chains, and this fact indicates that kindlins could inhibit the formation of clasped integrin α- and β-chains. Through the paxillin and Arp2/3 interactions, kindlins could activate Rac1, and in this way induce membrane protrusions. This could also happen through the phosphorylation of the β2-chain on T758, which results in high affinity 14-3-3 binding and release of filamin. This in turn is followed by binding of the adaptor protein Tiam1 and activation of Rac1 (Grönholm et al., Reference Grönholm, Jahan, Marchesan, Karvonen, Aatonen, Narumanchi and Gahmberg2011; O’Toole et al., Reference O'Toole, Bialkowska, Li and Fox2011) (Fig. 11).
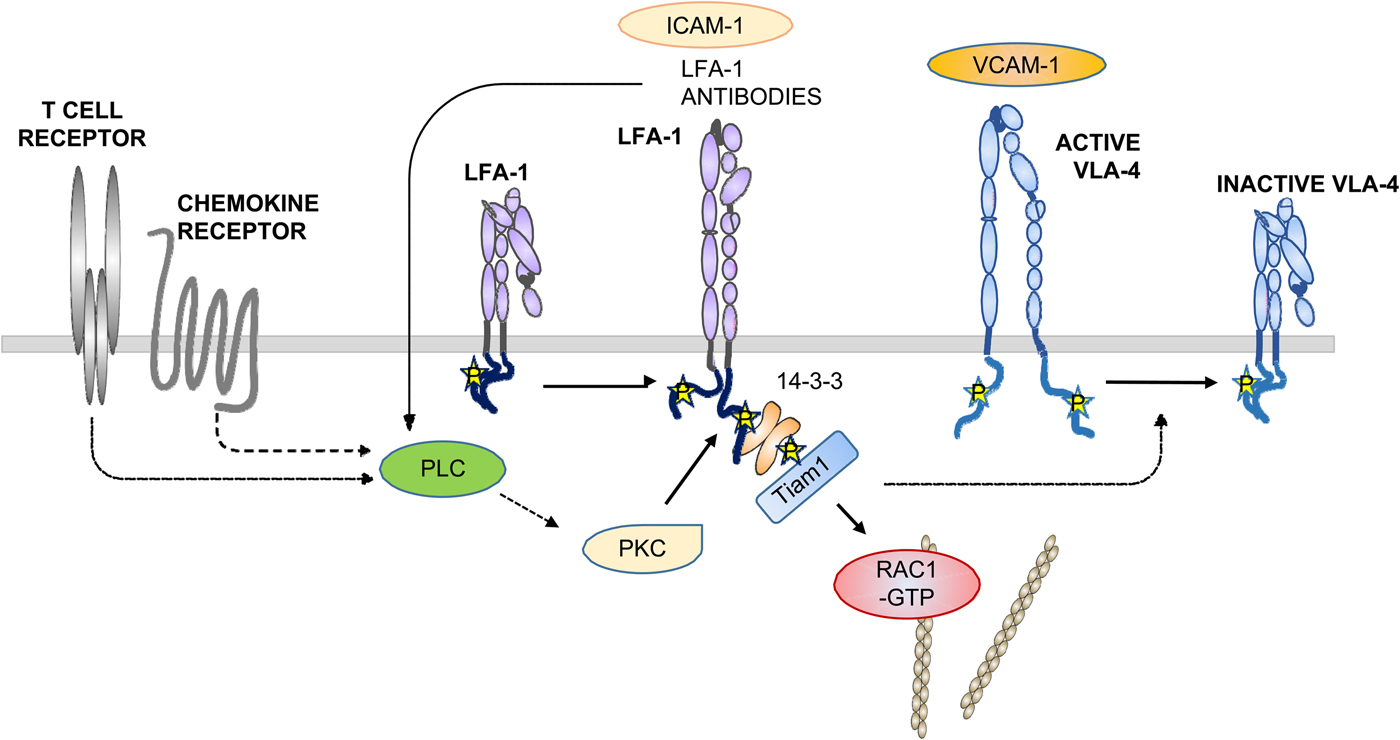
Fig. 11. Integrin crosstalk. Activation through the TCR or chemokine receptors activates LFA-1. This may also happen through antibody binding to LFA-1 or by outside-in activation by ICAMs. Then signaling through the β2 T758 route results in dephosphorylation of the β1 chain in VLA-4 resulting in its inactivation.
α-Actinin binds to the β2-chain between the two talin binding sites. It is an essential component of the actin cytoskeleton. Recent studies have shown that when LFA-1 and αXβ2 are activated, phosphorylation of the α-chains is needed to enable the phosphorylation of T758 on the β2-chain (Jahan et al., Reference Jahan, Madhavan, Rolova, Viazmina, Grönholm and Gahmberg2018). When both integrin chains were phosphorylated, α-actinin got access to the β-chain and bound more efficiently. The mutation S1140A in LFA-1 inhibited α-actinin binding to the β-chain and cell adhesion. Interestingly, the S1140D mutation restored α-actinin binding. The results indicate that when both the α- and β-chains are phosphorylated, the cytoplasmic clasp opens, perhaps due to repulsion between the cytoplasmic tails.
The Cbl-b protein is an adaptor protein, but it is also a ubiquitin ligase, and it affects cell adhesion. Deficiency of the protein resulted in increased phosphorylation of T758 in β2. This resulted in increased binding of 14-3-3 proteins and up-regulation of adhesion (Choi et al., Reference Choi, Orlova, Fagerholm, Nurmi, Zhang, Ballantyne, Gahmberg and Chavakis2008b). It is possible that SHARPIN and Cbl-b interact, because Cbl-b is known to bind the ubiquitin ligase HOIP (Zhou et al., Reference Zhou, Gao, Zhou, Chang, Hong, Song, Lin and Hu2008).
The Crk proteins, Crk and CrkL, are small adaptor proteins containing SH2 and SH3 domains and they are known to be involved in T cell trafficking (Roy et al., Reference Roy, MacKay, Robertson, Hammer and Burkhardt2018). They are active in outside-in signaling, because T cells with a knockout of Crk and CrkL adhered poorly to coated ICAM-1. Under shear flow, there was no difference in cell arrest in wt cells, but knockout cells did not spread. The leading edge was mobile in wt cells, whereas cells lacking the proteins did not form a leading edge. The Crk proteins promoted integrin-mediated PI3K signaling, but AKT phosphorylation was abrogated in knockout cells. Furthermore, the phosphorylation of the interacting C-cbl and Cbl-b proteins decreased. Possibly, the Crk proteins promote phosphorylation by facilitating contacts with tyrosine kinases.
The cytohesins are GEFs for the ARF family of small G proteins (Kolanus, Reference Kolanus2007). Cytohesin-1 is known to bind to the N-terminal cytoplasmic sequence (WKA) in the integrin β2-chain. This results in the activation of LFA-1. Cytohesin-3 resembles cytohesin-1 in inducing cell spreading, and cytohesin-2 regulates cell movement (Davies et al., Reference Davies, Tamaddon-Jahromi, Jannoo and Kanamalapudi2014). Interestingly, the binding motif for cytohesin-1 in the β2-chain resembles that of SHARPIN (WKV) for the α-chain.
Recently, it was found that the Aggregatibacter actinomycetemcomitans leukotoxin (LtxA) activates LFA-1 (Nygren et al., Reference Nygren, Balashova, Brown, Kieba, Dhingra, Boesze-Battaglia and Lally2018). The toxin binds to the LFA-1 external part, but surprisingly it also binds to the LFA-1 α- and β-chain cytoplasmic domains with high affinity. The affinities for αM and αX are considerably lower. The binding site in the α-chain cytoplasmic domain is in the proximal region (KVGFFKRNLKEKMEAGRGVPNGI), whereas the binding to the β2-chain is in the middle region, because the sequence W747NN increased the binding as compared to the β2 NH2 terminal W723-Q746 sequence. Treatment with phorbol esters decreased FRET of LFA-1 αL-CFP and β2-YFR as expected, but treatment with the toxin increased FRET, which indicates that the cytoplasmic domains became more close to each other. Possibly, the toxin interfered with the formation of the salt bridge between the αL and β2-chains, and therefore phorbol ester treatment of toxin-exposed cells no longer resulted in integrin phosphorylation. LtxA also prevented Rap1 and the formation of the Rap1/RapL complex. The lysines K998 and K1000 in the α-chain of LFA-1 are important for the binding of RapL, and this site overlaps with that of LtxA.
L-plastin (LCP1) is an actin binding protein related to α-actinin, which binds to the clasped αMβ2 and keeps the integrin inactive (Tseng et al., Reference Tseng, Samarelli, Kammerer, Scholze, Ziegler, Immier, Zent, Sperandio, Sanders, Fässler and Böttcher2018). It bound less efficiently to isolated αM and β2 polypeptides. Interestingly, the increased adhesion of LCP1 depleted cells was inhibited by treatment with phorbol esters. LCP1 may also affect integrin trafficking, because the amount of surface-expressed αMβ2 increased in LCP1 depleted cells, although the total amount of αMβ2 remained unchanged.
Cell adhesion is promoted by integrin-mediated mechanotransduction
Cells and organs live in an environment of extracellular matrix, and this is also true for blood cells at early stages of differentiation. Integrins bind to extracellular ligands, and during cellular movement in the blood stream or in extracellular tissues, there may arise mechanical tension between integrin-bound external molecules and the intracellular actin cytoskeleton. This integrin-mediated force induces important effects on cellular signaling and molecular changes in the interacting cells (Nordenfelt et al., Reference Nordenfelt, Elliott and Springer2016; Sun et al., Reference Sun, Guo and Fässler2016; Huse, Reference Huse2017).
In migrating cells, various integrin ligands, such as the ICAMs, VCAM-1, and fibronectin, form nascent adhesion sites at the leading edge through ‘catch bonds’. Here the integrins are active and induce focal adhesion or corresponding sites, and F-actin bundles force the cell further. The mechanosensitive proteins talin and vinculin are important and ‘slip bonds’ are generated. Whereas the talin head binds to the integrin β-chains, the actin binding sites in the talin rod are important, and by stretching the rod, vinculin binding sites become exposed. Kindlins and α-actinin are also important in mechanotransduction, but they have been less studied in this connection. Kindlin-3 may be important for the α4β1-mediated adhesion under shear flow (Lu et al., Reference Lu, Lin, Yan, Wang, Zhang, Wang, Wang, Liu and Chen2016).
In leukocytes, catch bonds are formed initially between E-selectin on endothelial cells and sialyl LeX oligosaccharides on leukocyte glycoconjugates. L-selectin on leukocytes may also bind to endothelial sialyl LeX ligands (Gong et al., Reference Gong, Zhang, Feng, Liu, Lu and Long2017). The rolling leukocytes then engage endothelial chemokines, which induce inside-out activation of integrins and strong adhesion due to catch bonds (Morikis et al., Reference Morikis, Chase, Wun, Chaikof, Magnani and Simon2017). During the selectin/ligand-induced rolling, the LFA-1 molecules extend, but remain closed. Due to the selectin catch bonds and chemokine activation, inside-out activation occurs, and the integrins become fully active with an open binding site. The leukocytes then move into tissues either between endothelial cells or through them. After that, leukocytes utilize local chemokines to migrate further (Shulman et al., Reference Shulman, Cohen, Roediger, Kalchenko, Jain, Grabovsky, Klein, Shinder, Stoler-Barak, Feigelson, Nurmi, Goldstein, Hartley, Gahmberg, Etzioni, Weninger, Ben-Baruch and Alon2012). Actually, the TCR interaction with peptide-bound transplantation antigens also involves catch bonds (Huse, Reference Huse2017). Interestingly, by studying single integrin attachment to a fibronectin substrate, Strohmeyer et al. (Strohmeyer et al., Reference Strohmeyer, Bharadwaj, Costell, Fässler and Muller2017) found that α5β1 sensed load to induce adhesion within a second, and after disrupting the bond, a slower adhesion reaction followed. This was due to catch bond formation.
Recent studies have shown that leukocytes can move upstream on ICAM-1 coated surfaces in fluid flow, and downstream when coated with VCAM-1 (Buffone et al., Reference Buffone, Anderson and Hammer2018). LFA-1 is the key receptor responsible for the upstream movement. Interestingly, the VLA-4/VCAM-1 interaction did not need any stimulation, whereas the LFA-1/ICAM-1 interaction must be activated. Possibly, there is a crosstalk between the integrins with LFA-1 as the dominating molecule (see below).
Integrin crosstalk
Due to the fact that most cells express several integrins, it is important that they can be regulated in a useful manner for the function of the cells. Communication between integrins is needed, for example, when cells migrate.
Porter and Hogg (Reference Porter and Hogg1997) observed that activation of LFA-1 in T cells down-regulated VLA-4 (α4β1), and α5β1 to a smaller extent. Activation through the TCR or with phorbol esters resulted in decreased binding to VCAM-1 and fibronectin. Then it was shown that ligation of αVβ3 inhibited α5β1 (Blystone et al., Reference Blystone, Slater, Williams, Crow and Brown1999). The treatment prevented the activation of the calcium/calmodulin-dependent protein kinase II (CamKII), and up-regulation of CamKII overcame the αVβ3-mediated suppression. Later work showed that β1 blocking antibodies inhibited αVβ3-mediated binding to α4 laminin. The treatment increased β3 phosphorylation on S752. PKA does not phosphorylate β3 on this site, but may act on the phosphatase inhibitor-1, which inhibits the phosphatase PP1 (Gonzales et al., Reference Gonzales, Claiborne and Jones2008).
We studied how the activation of β2 integrins in T cells results in the inactivation of VLA-4. Jurkat T cells lacking LFA-1 bound strongly to the VLA-4 ligand VCAM-1. When the cells were transfected with αL, the cells bound well, but when LFA-1 was activated using the SDF-1 chemokine, the binding to VCAM-1 was inhibited (Uotila et al., Reference Uotila, Jahan, Hinojosa, Melandri, Grönholm and Gahmberg2014). Phosphorylation of S1140 on αL was necessary, as the mutation S1140A prevented the crosstalk. Likewise, phosphorylation of T758 was essential. It was further shown that the signaling from β2-pT758 through the 14-3-3/Tiam1/Rac1 signaling route (Fig. 11) was necessary for the crosstalk. The signaling resulted in the dephosphorylation of T788/789 on β1.
Monoclonal antibodies are potentially useful for the treatment of T cell-mediated functions. We studied whether such antibodies affect integrin crosstalk in T cells. It turned out that the treatment of cells with LFA-1-activating antibodies, and some inhibitory antibodies, which induce T758 phosphorylation on β2, resulted in the dephosphorylation of T788/789 on the β1 chain of VLA-4 (see Fig. 4). The signaling took place through the 14-3-3/Tiam1/Rac1 pathway. By these antibody treatments, it became possible: (1) to activate LFA-1 and inhibit VLA-4, (2) inhibit both LFA-1 and VLA-4, (3) inhibit LFA-1 but not VLA-4, or (4) bind to LFA-1, but without any effect on either LFA-1 or VLA-4 activity (Grönholm et al., Reference Grönholm, Jahan, Bryushkova, Madhavan, Aglialoro, Hinojosa, Uotila and Gahmberg2016).
The results show that intracellular signaling between integrins takes place through specific integrin phosphorylations. Presently, we do not know how extensive the crosstalk is, or whether other mechanisms exist to mediate communication between integrins.
Sleep and fever affect leukocyte integrins
Drugs such as isoproterenol and histaminergic receptor agonists are known to affect integrins. These may act through Gαs-coupled agonists such as epinephrine, norepinephrine, prostaglandins E2 and D2, or adenosine to inhibit integrin activation (Chigaev et al., Reference Chigaev, Waller, Amit and Sklar2008, Reference Chigaev, Smagley and Sklar2011, Reference Chigaev, Smagley and Sklar2014). During sleep, the levels of Gαs agonists such as serotonin and catecholamines decrease, resulting in the activation of T cell adhesion (Dimitrov et al., Reference Dimitrov, Lange, Gouttefangeas, Jensen, Szczepanski, Lehnnolz, Soekadar, Rammensee, Born and Besedovsky2019).
It is a well-known fact that fever promotes survival during infection. Incubation of T cells at 40 °C induced the heat shock protein 90 (Hsp90), resulting in T cell integrin activation (Lin et al., Reference Lin, Zhang, Zhang, Zheng, Lu, Chang, Yang, Yang, Wan, Wang, Yuan, Yan, Zhang, He, Ge, Wu and Chen2019). Hsp90 was shown to bind to the α4 polypeptide through the ENRRDSWSY(991) motif (Fig. 5) and mutation to alanine of R985, W989, or Y991 abrogated Hsp90 binding. The α4β7 integrin was up-regulated, resulting in increased adhesion to VCAM-1. β2 integrins were not affected. Thermal stress increased the binding of talin and kindlin-3 to α4 integrins. When mice were infected with Salmonella typhi murium, the body temperature rose to 40 °C, and the mice with the R985A/R985A mutation in α4 integrins showed higher lethality than wt mice. There were much fewer T cells in the small intestines of the mutated mice, which shows that the recruitment of cells was inhibited (Lin et al., Reference Lin, Zhang, Zhang, Zheng, Lu, Chang, Yang, Yang, Wan, Wang, Yuan, Yan, Zhang, He, Ge, Wu and Chen2019).
Integrins are potential targets for therapy
Monoclonal antibodies to leukocyte integrins are potentially useful for the treatment of chronic inflammatory diseases such as psoriasis, rheumatoid arthritis, ischemia/reperfusion injury, contact dermatitis, and asthma (Mitroulis et al., Reference Mitroulis, Alexaki, Kourtzelis, Ziogas, Hajishengallis and Chavakis2015). A positive clinical response is often seen, for example, in the treatment of psoriasis (Krueger et al., Reference Krueger, Gottlieb, Miller, Dedrick, Garovoy and Walicke2000). A problem is that harmful side effects may appear due to wipe out of important leukocyte functions. The statins form an interesting group of drugs. These drugs are commonly used for the treatment of hypercholesterolemia (Corsini et al., Reference Corsini, Maggi and Catapano1995). The statins inhibit the 3-hydroxy-3-methylglutaryl coenzyme-A reductase, which is a key enzyme in the biosynthesis of cholesterol. The lovastatin and simvastatin drugs blocked the interaction of LFA-1 with ICAM-1 by binding to the I-domain (Frenette, Reference Frenette2001; Weitz-Schmidt et al., Reference Weitz-Schmidt, Welzenbach, Brinkmann, Kamata, Kallen, Bruns, Cottens, Takada and Hommel2001). Because the statins are relatively safe without appreciable side effects, they are potentially useful in clinical practice where inhibition of leukocyte functions is wanted. It could also be important to develop drugs that activate leukocyte adhesion. For the treatment of malignant diseases, an up-regulation of T cell and natural killer cell functions could be important. Activation could be achieved with integrin-activating antibodies or β2-integrin-activating peptides (Li et al., Reference Li, Nortamo, Kantor, Kovanen, Timonen and Gahmberg1993; Tng et al., Reference Tng, Tan, Ranganathan, Cheng and Law2004; Grönholm et al., Reference Grönholm, Jahan, Bryushkova, Madhavan, Aglialoro, Hinojosa, Uotila and Gahmberg2016).
In the future, it could become possible to further improve cancer treatment by immune checkpoint therapy (Sharma and Allison, Reference Sharma and Allison2015). The CTLA-4 and PD-1T cell molecules induce inhibitory pathways in T cells. Their normal functions are to keep T cells unreactive to self-tissues. CTLA-4 and PD-1 inhibit T cell killing of malignant tumors due to T cell-mediated suppression. Whereas PD-1 inhibits Akt phosphorylation by preventing CD28-mediated activation of PI3K (Parry et al., Reference Parry, Chemnitz, Frauwirth, Lanfranco, Braunstein, Kobayashi, Linsley, Thompson and Riley2005; Buchbinder and Desai, Reference Buchbinder and Desai2016), the PP2A phosphatase is important for the CTLA-4-mediated effect. By use of antibodies to inhibit the inhibitory molecules, excellent results have been obtained in the treatment of melanoma. Promising results have also been obtained with lung cancer and kidney cancer. Interestingly, engaging CTLA-4 with antibodies up-regulated LFA-1, resulting in increased adhesion and integrin capping. The effect was even stronger using a combination of CD3 and CTLA-4 antibodies. The treatments induced signaling and activation of the small G protein Rap1 (Gatta et al., Reference Gatta, Calviello, di Nicuolo, Pace, Ubaldi, Doria and Pioli2002; Schneider et al., Reference Schneider, Valk, da Rocha Dias, Wei and Rudd2005).
A model of cell adhesion and deadhesion
So far we have reviewed the topic of cell adhesion and integrins with a focus on T lymphocytes, but also to some extent discussed integrins in platelets and other cells.
It is certainly evident from the above text that we currently know quite a lot about integrin structure and allosteric changes during activation, and how dominant integrins, such as LFA-1, may regulate other integrins. We can distinguish between the unique abilities of integrins to signal both inside-out and outside-in across the cell plasma membrane. We are also aware of the fact that a large number of cytoplasmic proteins interact with the intracellular domains of integrins, and the binding sites have been mapped with detailed precision. Proteomic studies show that there are still an enormous number of proteins, binding either directly or indirectly to integrin cytoplasmic domains, and certainly some of them may turn out to be essential for function. The ability to bind several different proteins to the cytoplasmic domains provides means to regulate functions in a number of ways in different cells, within subdomains in the same cell and at different times. This ability is quite different from that of most other type I membrane proteins, which have more restricted functions, such as transport through the membrane or membrane spanning tyrosine kinases or phosphatases.
Knockout experiments and mutational analyses show that most of the cytoplasmic proteins dealt with above are important for integrin functions and, likewise, several different integrin cytoplasmic motifs are of pivotal importance.
Furthermore, some interacting proteins are essential for adhesion and allosteric signaling through the integrin external domains to activate or inhibit binding, whereas others may be important for integrin localization, cellular movement, and intracellular signaling. Because the integrins have many different functions, it is important that they can switch interactions, and in this way cellular functions.
After describing what currently is known, let us now look at how T cell integrins could become activated using LFA-1 as a model (Fig. 12). In inside-out activation, the LFA-1 molecule is activated either through the TCR or through chemokine receptors. After proximal signaling, talin is associated with the β2 polypeptide, PKCs become activated and phosphorylate T758 in the β2-chain. At this point, a large proportion of S1140 in the α-chain is phosphorylated. There is a turnover of the α-chain phosphorylation at S1140, which is observed by treatment with okadaic acid (Valmu and Gahmberg, Reference Valmu and Gahmberg1995). If the α-chain phosphorylation site is without phosphate, the β-chain cannot be phosphorylated, and the integrin remains inactive (Jahan et al., Reference Jahan, Madhavan, Rolova, Viazmina, Grönholm and Gahmberg2018). When both chains are phosphorylated, the cytoplasmic part of the clasped integrin opens up and filamin is released due to high affinity 14-3-3 binding. Structural work showed that the phosphorylated β-chain cannot be accommodated within the filamin pocket (Takala et al., Reference Takala, Nurminen, Nurmi, Aatonen, Strandin, Takatalo, Kiema, Gahmberg, Ylänne and Fagerholm2008). The 14-3-3 proteins bind to the phosphorylated chain with a Kd of ~5 µM (Chatterjee et al., Reference Chatterjee, Zhiping, Tan and Bhattacharjya2016). The open cytoplasmic domain is now able to bind α-actinin (Jahan et al., Reference Jahan, Madhavan, Rolova, Viazmina, Grönholm and Gahmberg2018). Talin is then released from the NPLF site, but remains associated with the β-chain through the proximal binding site, and by interaction with the lipid membrane (Gingras et al., Reference Gingras, Lagarrique, Cuevas, Valadez, Zorovich, McLaughlin, Lopez-Ramirez, Seban, Ley, Kiosses and Ginsberg2019). Dok-1 is bound to the other half of the 14-3-3 dimer, and makes a complex of pT758/14-3-3/Dok-1/kindlin-3 (Chatterjee et al., Reference Chatterjee, D'Souza, Zhang, Bin, Tan and Bhattacharjya2018a). Both 14-3-3 and kindlin-3 are dimers and the proteins bind to the actin cytoskeleton. This results in the clustering of integrins leading to increased avidity. Furthermore, signaling through the pT758/14-3-3/Tiam1/Rac1(GTP) route activates the cytoskeleton allowing protrusions at the plasma membrane (Grönholm et al., Reference Grönholm, Jahan, Marchesan, Karvonen, Aatonen, Narumanchi and Gahmberg2011). Simultaneously, the bound talin directly activates the integrin by inducing an allosteric structural alteration transmitted through the membrane resulting in an increase of the integrin affinity for external ligands such as ICAM-1. The cycle of phosphorylation and dephosphorylation of S1140, combined with an activation signal through the TCR or chemokine receptors, in turn enables T758 phosphorylation and dephosphorylation of the β-chain and an integrin activation cycle.

Fig. 12. An integrated view of LFA-1 activation. In the resting integrin, the α-chain is phosphorylated, and filamin and Dok1 are associated with the β-chain. Upon activation, T758 in the β-chain is phosphorylated resulting in the release of filamin, whereas kindlin-3, Dok1, and 14-3-3 form a complex bound to the β-chain. The phosphorylations on both the α- and β-chains open the cytoplasmic domain, and α-actinin binds to the β-chain, and connect the integrin to the actin cytoskeleton. Talin remains associated with the β2-chain, and may induce a transmembrane allosteric activation of the LFA-1 external ligand binding site. Tiam1 activates Rac1 to the GTP form, inducing reorganization of the actin cytoskeleton and facilitating binding to the integrin. The 14-3-3, kindlin-3, and α-actinin proteins form dimers, enabling clustering of the integrins, which results in increased avidity for the ligands such as ICAM-1.
It should be pointed out that integrins differ particularly in their cytoplasmic parts, and the same regulatory mechanisms certainly do not apply to all. The αMβ2 integrin has a relatively short α-chain cytoplasmic domain as compared to the others in the integrin subfamily (Fig. 5). This fact may be the reason that S756, which is phosphorylated in phorbol ester-treated cells, is more accessible to β-chain interacting cytoplasmic proteins. Rap1 interacts with the phosphorylated S756 in αMβ2, recruits talin, and positions it for subsequent functions such as phagocytosis in myeloid cells (Lim et al., Reference Lim, Hotchin and Caron2011).
In outside-in activation, the beginning of activation is different involving early integrin phosphorylation by tyrosine kinases. In leukocytes, Lck is important, whereas Src is involved in αIIbβ3 activation. Further signaling then results in integrin phosphorylation as described above.
In the resting state, the integrins are clasped by inhibitory cytosolic proteins such as filamin, SHARPIN, and others. These are outcompeted early during activation due to lower affinity for integrin binding, ~ 0.5 mM for filamin (Chatterjee et al., Reference Chatterjee, Zhiping, Tan and Bhattacharjya2018b), as compared to that of 14-3-3 and subsequent activators.
With the exception of PKCs involved in T758 phosphorylation of the β2-chain, little is currently known about which protein kinases and phosphatases are involved in integrin regulation, and this subject certainly needs much further effort.
The outlined scheme is for some part hypothetical and needs further experimental work to become more complete. However, we now have a way to proceed to enable us to get a deeper understanding of this exciting and important subject in cell biology and medicine.
Financial support
The current studies were supported by the Finnish Medical Society, the Magnus Ehrnrooth Foundation, the Liv och hälsa Foundation, the Finnish Society of Science and Letters, and the Wilhelm och Else Stockmann Foundation.