Introduction
Annual bluegrass (Poa annua L.) is a problematic weed that reduces the aesthetic and functionality of turfgrass. Compared with most turfgrasses, P. annua has light green color, coarse leaf texture, a shallow root system, and unsightly seed heads (Beard Reference Beard1970). Poa annua often creates unsightly patches in turfgrass due to prolific seed head production and poor tolerances to heat, disease, drought, and traffic stress (Beard Reference Beard1970; Lush Reference Lush1989). The decline of P. annua populations in spring reduces turf density, which can lead to the invasion of summer weeds. Turf managers reduce P. annua populations by adjusting fertilization programs, modifying irrigation regimens, and removing mower clippings after seed head formation (Gaussoin and Branham Reference Gaussoin and Branham1989; Lush Reference Lush1989). Cultural practices can reduce P. annua populations in turfgrass, but herbicides are often warranted to provide acceptable levels of control.
Herbicide resistance is “the inherited ability of a plant to survive and reproduce following exposure to a dose of herbicide normally lethal to the wild type. In a plant, resistance may be naturally occurring or induced by such techniques as genetic engineering or selection of variants produced by tissue culture or mutagenesis” (WSSA 1998). Herbicide resistance is an evolutionary process, and its dynamics and impact are influenced by multiple factors, such as the intensity of selection pressure by herbicides and the initial frequency of herbicide-resistant individuals in the population (Jasieniuk et al. Reference Jasieniuk, Brûlé-Babel and Morrison1996; Vencill et al. Reference Vencill, Nichols, Webster, Soteres, Mallory-Smith, Burgos, Johnson and McClelland2012). Resistance is commonly reported to be the result of high intensity of recurrent selection pressure, but the high initial frequency of resistant individuals in the population is unusual and rarely reported (Jasieniuk et al. Reference Jasieniuk, Brûlé-Babel and Morrison1996; Powles and Yu Reference Powles and Yu2010; Preston and Powles Reference Preston and Powles2002). In Australia, an Italian ryegrass [Lolium perenne (L.) ssp. multiflorum (Lam.) Husnot.] population evolved resistance to acetyl-CoA carboxylase– and acetolactate synthase (ALS)-inhibiting herbicides with as few as three applications of these herbicides (Powles et al. Reference Powles, Preston, Bryand and Jutsum1997; Preston and Powles Reference Preston and Powles2002; Tardif et al. Reference Tardif, Holtum and Powles1993). The high initial frequency of individuals resistant to ALS-inhibiting herbicides in this L. perenne population that had never been previously exposed to these herbicides explains the rapid evolution of herbicide resistance in this weed species once ALS-inhibiting herbicides were used (Preston and Powles Reference Preston and Powles2002). Naturally occurring herbicide-resistant P. annua has not been previously reported.
Currently, 28 cases of herbicide-resistant P. annua have been reported worldwide (Heap Reference Heap2018). Resistance has been confirmed to ethofumesate, glyphosate, sulfonylureas, pronamide, and triazines used for POST P. annua control in turfgrass (Binkholder et al. Reference Binkholder, Fresenburg, Teuton, Xiong and Smeda2011; Hutto et al. Reference Hutto, Coats and Taylor2004; Kelly et al. Reference Kelly, Coats and Luthe1999; McCullough et al. Reference McCullough, Yu and Czarnota2017; McElroy et al. Reference McElroy, Flessner, Wang, Dane, Walker and Wehtje2013). Poa annua has also developed resistance to dithiopyr, pendimethalin, and prodiamine used for PRE control (Isgrigg et al. Reference Isgrigg, Yelverton, Brownie and Warren2002; Cutulle et al. Reference Cutulle, McElroy, Millwood, Sorochan and Stewart2009). Herbicide resistance in P. annua is often conferred by a mutated target-site enzyme or protein that reduces affinity for the herbicide (Anthony et al. Reference Anthony, Waldin, Ray, Bright and Hussey1998; Cross et al. Reference Cross, McCarty, Tharayil, McElroy, Chen, McCullough, Powell and Bridges2015; McElroy et al. Reference McElroy, Flessner, Wang, Dane, Walker and Wehtje2013). Use of herbicides with different modes of action appears necessary for successful control of P. annua with resistance to these herbicides.
Flumioxazin inhibits protoporphyrinogen oxidase (PPO), a key enzyme in the chlorophyll and heme biosynthetic pathway. PPO inhibition initiates a chain reaction of lipid peroxidation, resulting in loss of chlorophyll and carotenoids and in leaky membranes that allow cells and cell organelles to dry and disintegrate rapidly (Duke et al. Reference Duke, Lydon, Becerril, Sherman, Lehnen and Matsumoto1991). Flumioxazin provides PRE and POST control of P. annua in bermudagrass [Cynodon dactylon (L.) Pers.] (Flessner et al. Reference Flessner, McElroy, Baird and Barnes2013). Other PPO inhibitors used in turfgrass include carfentrazone, oxadiazon, and sulfentrazone. However, these herbicides do not target POST P. annua at labeled use rates (Isgrigg et al. Reference Isgrigg, Yelverton, Brownie and Warren2002; Toler et al. Reference Toler, McCarty and Higingbottom2003). The efficacy of flumioxazin for POST P. annua control is influenced by plant maturity. Flessner et al. (Reference Flessner, McElroy, Baird and Barnes2013) reported that flumioxazin at 0.43 kg ai ha−1 controlled P. annua ≥95% up to a 2-tiller growth stage, but applications to plants with 4 to 6 tillers provided <50% control. Similar levels of POST P. annua control have been noted in field experiments with flumioxazin (Flessner et al. Reference Flessner, McElroy, Baird and Barnes2013; Reed et al. Reference Reed, McCullough, Grey, Czarnota, Vencill and Waltz2015). Moreover, there have been no reports of P. annua resistance to PPO inhibitors (Heap Reference Heap2018), and flumioxazin offers an alternative mechanism of action for POST control of biotypes with resistance to other herbicides.
A P. annua biotype with limited control by flumioxazin was identified on a golf course in Georgia. The golf course had a history of using sulfonylureas, triazines, and dinitroaniline herbicides for P. annua control. The efficacy of flumioxazin at 420 g ai ha−1 was screened on this P. annua population as an alternative to the aforementioned herbicides. In preliminary greenhouse experiments, flumioxazin reduced dry-shoot biomass of this biotype and a known susceptible biotype to 77% (±6.9 SE, n=10) and 20% (±6.3 SE, n=10) of the nontreated at 4 wk after treatment (WAT), respectively. The differential tolerance levels of P. annua biotypes to flumioxazin could limit the consistency of applications for controlling populations with resistance to other modes of action. The objective of this research was to evaluate the susceptibility levels of these P. annua biotypes to PPO inhibitors and characterize physiological response of putative resistant and susceptible biotypes.
Materials and Methods
Plant Material
Poa annua plants (hereafter delineated as “R-biotype”) were collected from the Willow Lakes Golf Course in Metter, GA, on April 24, 2015. Plants were collected for herbicide screening in the greenhouse due to erratic levels of control in field from previous frequent use of prodiamine and sulfonylureas. The plants were transplanted to separate plastic pots with 79-cm2 surface area and 10-cm depths filled with sand:peat moss medium (85:15 v/v). Pots were placed in a greenhouse set for 23/17 C (day/night) at the University of Georgia Griffin Campus and irrigated to prevent moisture deficiencies. Seeds were collected by hand and immediately scattered over greenhouse flats filled with the aforementioned potting medium. Seed from a susceptible (S)-biotype of P. annua indigenous to Griffin, GA, was collected by hand from greenhouse-grown plants and scattered over separate flats for establishment. The soil was irrigated daily and fertigated (MacroN 28-7-14™ Sprayable Fertilizer, LESCO, Cleveland, OH, 44114) twice per week. Multileaf plants of the biotypes were transplanted to separate pots with 3.8-cm diameters and 20-cm depths filled with sand:peat moss soil. Plants were irrigated and fertilized to promote growth and allowed to reach a 3- to 5-tiller growth stage before treatments.
Dose–Response Experiments
The response of the two P. annua biotypes was evaluated in rate-titration experiments with PPO inhibitors. In the first experiment (delineated Experiment 1), flumioxazin (SureGuard® 51WG, Valent, Walnut Creek, CA) was applied in a spray chamber calibrated to deliver 187 L ha−1 with a flat-fan nozzle (8002E, TeeJet Spraying Systems, Roswell, GA 30075). Flumioxazin was applied at 12.5, 25, 50, 100, 200, 400, 800, 1,600, or 3,200 g ha−1. These rates were chosen so labeled use rates for P. annua in dormant bermudagrass (200 to 400 g ha−1) were in the midrange of rates evaluated (Anonymous 2015). Treatments included a nonionic surfactant (CNI 80-20, mixture of alcohol ethoxylate, alkylphenol ethoxylate, 80%, CNI, Leesburg, GA 31763) at 0.25% v/v. Nontreated checks of the two biotypes were included. Plants were returned to the greenhouse at 1 h after treatment (HAT). Irrigation was withheld for 24 HAT, but was applied thereafter as needed to prevent moisture stress. Shoots were harvested with shears 2 WAT, oven-dried for 72 h at 60 C, and then weighed.
In a separate experiment (Experiment 2), the differential tolerance levels of the two biotypes were evaluated from rate titrations of two PPO inhibitors, flumioxazin and sulfentrazone. Both herbicides were applied separately at 100, 200, 400, 800, 1,600, 3,200, 6,400, or 12,800 g ai ha−1 with the aforementioned nonionic surfactant at 0.25% v/v. Shoot biomass was harvested at 2 WAT, oven-dried, and weighed as previously described.
Evaluation of P. annua Responses to Flumioxazin with Chlorpyrifos
In Experiment 3, flumioxazin was applied with an organophosphate insecticide, chlorpyrifos, to determine whether control of the R-biotype could be enhanced by the tank mixtures. This technique was previously used to evaluate cytochrome P450–associated metabolism-based resistance to PPO inhibitors in waterhemp [Amaranthus tuberculatus (Moq.) J.D. Sauer].; Shoup et al. Reference Shoup, Al-Khatib and Peterson2003). Organophosphates have competitive binding with cytochrome P450 enzymes associated with herbicide metabolism, and applications have been shown to reverse metabolism-based resistance to synthetic auxins, photosynthesis inhibitors, and mitotic inhibitors (Chang et al. Reference Chang, Smith and Stephenson1971; Siminszky Reference Siminszky2006). Therefore, experiments were conducted to determine whether metabolism was associated with differences in susceptibility of the P. annua biotypes to POST flumioxazin applications.
The R- and S-biotypes were established as previously described and treated with the factorial combination of nine by two rates of flumioxazin and chlorpyrifos, respectively. Flumioxazin at 0, 12.5, 50, 100, 200, 400, 800, 1,600, or 3,200 g ha−1 was applied with chlorpyrifos (Eraser™ Insecticide 4L, Independent Agribusiness Professionals, Fresno, CA 93710) at 0 or 2,240 g ai ha−1. All treatments, except chlorpyrifos alone, included a nonionic surfactant at 0.25% v/v. Plants were returned to the greenhouse at 1 HAT. Irrigation was withheld for 24 HAT, but was applied thereafter as needed to prevent moisture stress. Shoots were harvested with shears at 2 WAT, oven-dried for 72 h at 60 C, and then weighed.
Efficacy of PRE applications of PPO Inhibitors
In Experiment 4, PRE applications of PPO inhibitors were evaluated for controlling the two biotypes from seed. Flumioxazin and oxadiazon were chosen for these experiments, because they are the only PPO inhibitors used for PRE control of P. annua in turfgrass. Pots with 79-cm2 surface area and 10-cm depths were filled with the aforementioned sand:peat moss soil. Treatments included flumioxazin at 140 or 420 g ha−1, and oxadiazon (Ronstar® 50WSP, Bayer Environmental Science, Research Triangle Park, NC, 27709) at 3,360 g ai ha−1. Applications were made with the spray chamber at 187 L ha−1. Nontreated checks of both biotypes were included. The pots were returned to the greenhouse immediately after treatments. Seed of the two biotypes was uniformly scattered over separate pots. Irrigation was applied as needed to prevent soil moisture deficiencies and promote seedling growth. Plants were counted per pot at 3 and 6 WAT.
Electroconductivity Assay
In Experiment 5, the differential levels of electrolyte leakage in the R- and S-biotypes were evaluated using a modified methodology from experiments described by Falk et al. (Reference Falk, Al-Khatib and Peterson2006). Electrolyte leakage is indicative of cellular membrane damage and can be used to describe the response levels to PPO inhibitors. Leaves were harvested and sectioned into 1.5-cm segments. Samples (0.15 g) were placed on plastic plates and sprayed with flumioxazin at 0, 88, 175, 350, or 700 g ha−1. Herbicide treatments were made with a CO2-pressurized sprayer calibrated to deliver a volume of 187 L ha−1. A nonionic surfactant at 0.25% (v/v) was included in the spray solution.
At ~15 min after treatment, leaves were transferred with tweezers to moist filter paper in 100-cm-diameter by 15-mm-deep petri dishes containing 5 ml of deionized water. The petri dishes were then covered with clear plastic film, and the samples were placed in an incubator set for 25 C with 380 mol m−2 s−1 of light for 15 h. After incubation, plant leaves were removed from petri dishes with tweezers and placed in test tubes filled with 20 ml of deionized water. Electroconductivity of the incubation medium in test tubes was measured with a conductivity meter before the addition of leaf samples (AR60, Accumet Research, 300 Industry Drive, Pittsburgh, PA 15275). Samples were incubated in darkness for 3 h and vortexed three times at 1-h intervals. After incubation in darkness, conductance of the medium was recorded (CI). Samples were placed in an ethylene glycol bath (Thermo NESLAB, RTE 740, 25 Nimble Hill Road, Newington, NH 03801) at 90 C for 50 min and then cooled to 23 C using a water bath (Branson 5510, Branson Ultrasonics, 41 Eagle Road, Danbury, CT 06810), and the final conductance was measured to determine the total electrolyte leakage (Cmax). Percent electrolyte leakage was determined using the equation (CI/Cmax) ×100.
Malondialdehyde (MDA) Measurement
Experiments were conducted to evaluate the differences in lipid peroxidation following flumioxazin treatments in the two P. annua biotypes. Lipid peroxidation was quantified by measuring MDA content in leaves using a modified methodology described by Zhang and Huang (Reference Zhang and Huang2013). Grasses were grown in the greenhouse as previously described and treated with flumioxazin at 0, 280, and 560 g ha−1. Herbicide treatments were applied using a CO2-pressurized sprayer calibrated to deliver 374 L ha−1 with a 9504E flat-fan nozzle. Leaves were harvested at 24, 48, or 72 HAT.
Leaf tissue (0.1 g) was placed in centrifuge tubes and ground into powder with liquid nitrogen. Tubes were then filled with 1 ml 0.1% (1 g L−1) trichloroacetic acid (TCA), and samples were vortexed for 3 s (Vortex-Genie 2, 80 Orville Drive, Suite 102, Bohemia, NY 11716), sonicated for 5 min (Branson 5510 Ultrasonic Cleaners, Danbury, CT 60813), and then centrifuged at 10,000× g for 10 min. A 0.5-ml supernatant was transferred to separate tubes filled with 2 ml 20% TCA containing 0.5% thiobarbituric acid (TBA). The mixture was boiled at 95 C for 15 min (RTE 740, Thermo Fisher Scientific, 25 Nimble Hill Road, Newington, NH 03801) and quickly cooled in an ice bath to stop the reaction. After cooling, samples were centrifuged at 10,000×g for 10 min, and the optical density of supernatant was read at 532 nm using a spectrophotometer (UV-2700 Spectrophotometer, Shimadzu, Japan). The reaction of MDA with TBA catalyzed a red coloration of the sample, and MDA content was calculated from a standard curve.
Experimental Design and Data Analysis
The design for greenhouse experiments was a randomized complete block with four replications. This was chosen to reduce variability of greenhouse location on plant responses to treatments. The design for the laboratory experiments was completely randomized with four replications. Three experimental runs were conducted for Experiments 1 and 4. All other experiments were conducted twice.
Data were subjected to ANOVA with the PROC GLM in SAS (SAS v. 9.3, SAS Institute, Cary, NC). Levene’s test for homogeneity of variances was conducted before ANOVA. For the dose–response experiments, data were analyzed with the PROC NLIN in SAS. Means were plotted on figures and regressed against Equation 1 for the R- and S-biotypes: <COMP: Please set equation numbers flush right on type area.>

where β0 is the upper asymptote, β1 is the lower asymptote, β2 is the slope, and x is the application rate (g ha−1). This equation was chosen because it best described the relationship of rate with plant responses. For the electroconductivity assay, data were regressed against the following linear response equation:

where β0 is the intercept, β1 is the slope, and x is the flumioxazin rate. Regression analyses were used to identify the herbicide rate that caused 50% reductions in dry-shoot biomass from the nontreated (SR50) or 50% electrolyte leakage (EL50) before boiling. The 95% confidence limits for these values were calculated in SigmaPlot (v. 11.2, Systat Software, San Jose, CA) to separate biotypes. Experiment by treatment interactions were not detected, and thus results were pooled over experimental runs.
Results and Discussion
Growth Responses following Applications of PPO Inhibitors
In Experiment 1, the SR50 values for the R- and S-biotypes following flumioxazin treatments were >3,200 and 122 g ha−1, respectively (Figure 1; Table 1). In Experiment 2, the SR50 values for the S-biotype for flumioxazin and sulfentrazone were 232 and 472 g ha−1, respectively, while SR50 values for the R-biotype were 3,365 and 4,895 g ha−1, respectively (Figure 2; Table 1). The resistance factors for the R-biotype to POST applications of flumioxazin and sulfentrazone were >14.5 and 10.4, respectively.

Figure 1 Dry-shoot biomass of two Poa annua biotypes at 14 d after flumioxazin treatment in three greenhouse experiments. Results were pooled over experimental runs. Vertical bars represent standard error of the mean (n=12).
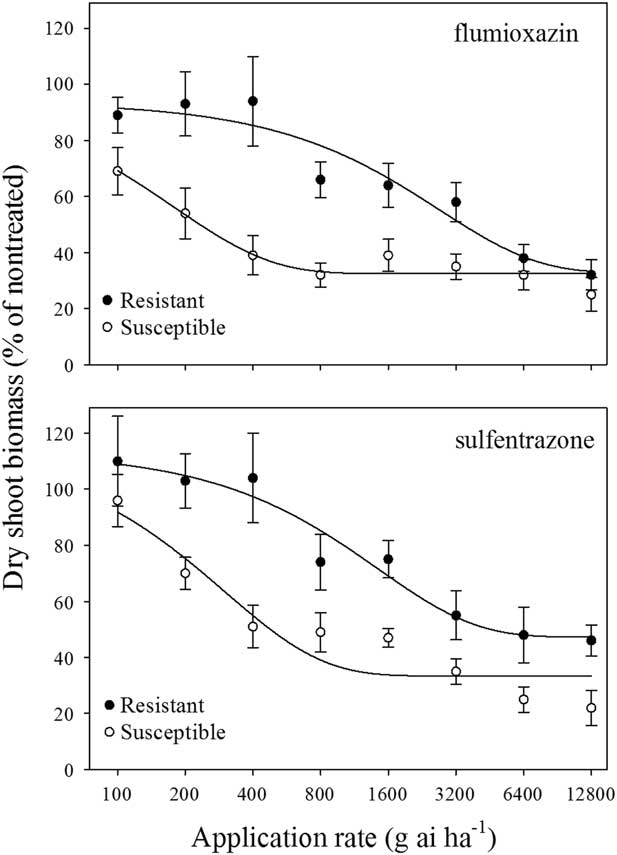
Figure 2 Dry-shoot biomass of two Poa annua biotypes at 14 d after treatment with flumioxazin or sulfentrazone in three greenhouse experiments. Results were pooled over experimental runs. Vertical bars represent standard error of the mean (n=12).
Table 1 Regression equations for data presented in figures.Footnote a

a CI, confidence interval; SR50, 50% reductions in dry-shoot biomass.
b Number in parenthesis is standard error of estimate.
c Data were regressed against the equation, y=β0+β1 * exp(−β2 * x), where β0 is the upper asymptote, β1 is the lower asymptote, and β2 is the slope, and x is the application rate in g ha−1.
d Data were regressed against the following linear response equation, y=β0+β1 * x, where β0 is the intercept, β1 is the slope, and x is the flumioxazin rate.
The first case of PPO-inhibitor resistance in a weed species was confirmed in a A. tuberculatus biotype from Kansas (Shoup et al. Reference Shoup, Al-Khatib and Peterson2003). This biotype was 34, 82, 8, and 4 times more resistant than a susceptible biotype to acifluorfen, lactofen, fomesafen, and sulfentrazone, respectively (Shoup et al. Reference Shoup, Al-Khatib and Peterson2003). There have since been many reports of PPO-resistant biotypes of A. tuberculatus found in Missouri and Illinois (Li et al. Reference Li, Smeda, Nelson and Dayan2004). The range of resistance levels to POST applications of PPO inhibitors has shown to vary from different populations. Patzoldt et al. (Reference Patzoldt, Tranel and Hager2005) reported A. tuberculatus from Illinois had resistance factors ranging from 2.2- to 23-fold for PPO inhibitors, with the value varying depending on the herbicide being evaluated.
In Experiment 3, the efficacy of PPO inhibitors for PRE control of the R- and S-biotypes was evaluated. Treatment by biotype interactions were detected for plant count reductions relative to the nontreated at 6 WAT. Plant counts for the nontreated averaged 9 (±1.7 standard error of the mean) and 6 (±1.5 standard error of the mean) per pot for the R- and S-biotypes, respectively. Flumioxazin and oxadiazon completely controlled the emergence of both biotypes at 6 WAT (unpublished data).
Researchers have reported that A. tuberculatus with resistance to POST applications of PPO inhibitors was susceptible to PRE applications. Shoup and Al-Khatib (Reference Shoup and Al-Khatib2004) found POST applications of acifluorfen and lactofen controlled PPO-resistant A. tuberculatus 40% at 8 WAT. However, PRE applications of sulfentrazone and flumioxazin controlled this biotype >85%. Similarly, PRE applications of flumioxazin, isoxaflutole, and sulfentrazone controlled an R-biotype of A. tuberculatus >90%, while POST applications of other PPO inhibitors provided <60% control (Harder et al. Reference Harder, Nelson and Smeda2012). It was also found that the majority of herbicide activity from POST flumioxazin, fomesafen, acifluorfen, and lactofen was from foliar placement, but control was less than 40% at all placements.
The efficacy of PPO inhibitors for POST weed control has been previously attributed to growth stage. Flessner et al. (Reference Flessner, McElroy, Baird and Barnes2013) reported that flumioxazin at 0.4 kg ha−1 controlled P. annua ≥95% up to a 2-tiller growth stage, but applications to plants with 4 to 6 tillers provided <50% control. Falk et al. (Reference Falk, Al-Khatib and Peterson2006) reported acifluorfen and fomesafen at 420 g ai ha−1 controlled a PPO-resistant biotype of A. tuberculatus greater than 85% at the 2-leaf and 4- to 6-leaf growth stage. However, applications to R-biotype plants at the 8- to 10-leaf growth stage ranged from 54% to 75%. Similarly, the tolerance of cotton (Gossypium hirsutum L.) and peanut (Arachis hypogaea L.) to flumioxazin applications has shown to increase at mature growth stage as compared with immature growth stages (Price et al. Reference Price, Pline, Wilcut, Cranmer and Danehower2004a; Thomas et al. Reference Thomas, Troxler, Smith, Fisher and Wilcut2005). Researchers have also shown that growth stage influences control of grassy weeds from other herbicides, including glufosinate, glyphosate, quinclorac, and mesotrione (Ahmadi et al. Reference Ahmadi, Haderlie and Wicks1980; Chism et al. Reference Chism, Birch and Bingham1992; Steckel et al. Reference Steckel, Wax, Simmons and Phillips1997; Yu and McCullough Reference Yu and McCullough2016). Thus, tolerance to PPO inhibitors at recommended application rates may become prevalent after R-biotypes reach mature growth stages.
Poa annua is likely susceptible to PRE treatments of flumioxazin and oxadiazon due to herbicide concentrations absorbed by seedlings. The differences in POST control between these biotypes suggest that physiological tolerance mechanisms are associated with plant maturity. Further research may be needed to quantify levels of control at various timings to determine the growth stage at which segregation may occur among biotypes from field applications. These findings support the results reported by Flessner et al. (Reference Flessner, McElroy, Baird and Barnes2013) regarding the importance of flumioxazin application timing for POST P. annua control. However, differential tolerance levels among biotypes could require earlier POST timings, such as at the pre-tiller stage, to maximize the efficacy and consistency of flumioxazin applications for P. annua control.
Electroconductivity, MDA Accumulation, and Metabolism Assays
Cellular leakage and lipid peroxidation levels are biochemical reactions used to measure the herbicidal effects of PPO inhibitors in rice (Oryza sativa L.) and soybean [Glycine max (L.) Merr.] (Dayan et al. Reference Dayan, Armstrong and Weete1998; Jung and Kuk Reference Jung and Kuk2007). Electrolyte leakage of the two biotypes linearly increased as flumioxazin rate increased (Figure 3; Table 1). The R-biotype averaged 27% less electrolyte leakage compared with the S-biotype after flumioxazin treatments.

Figure 3 Electrolyte leakage of two Poa annua biotypes following broadcast applications of flumioxazin. Results were pooled over experimental runs. Vertical bars represent standard error of the mean (n=8).
In further evaluations of lipid peroxidation, MDA content was measured following broadcast applications of flumioxazin at 280 and 560 g ha−1. The MDA concentrations (nm mg−1 fresh weight) of the nontreated R- and S-biotypes were similar at 24, 48, and 72 HAT (Table 2). The MDA concentrations of flumioxazin-treated plants of both biotypes increased within the first 48 HAT. There was also a rate response in both biotypes that was detected at 48 HAT. However, the R-biotype treated with both rates of flumioxazin averaged 25% less MDA accumulation by 72 HAT.
Table 2 Malondialdehyde (MDA) concentrations following broadcast flumioxazin applications to two Poa annua biotypes.Footnote a

a Results were pooled over two experimental runs.
b HAT, hours after treatment.
Resistance to PPO inhibitors has been measured from levels of membrane disruption, tissue leakage, and accumulation of the protoporphyrin IX substrate in chlorophyll biosynthesis. Transgenic rice with PPO resistance accumulated 15- to 21-fold higher protoporphyrin IX than a wild type (Jung et al. Reference Jung, Lee, Yang, Lee, Jang, Ha and Back2004). Li et al. (Reference Li, Smeda, Nelson and Dayan2004) reported that levels of protoporphyrin IX accumulated in leaf disks exposed to acifluorfen were lower in an R-biotype compared with an S-biotype. The electrolyte leakage differences in two biotypes of P. annua are associated with the intensity of membrane degradation levels by flumioxazin. However, other biochemical factors warrant further investigation to help characterize the mechanism of tolerance to PPO inhibitors in this P. annua biotype.
Chlorpyrifos by flumioxazin rate interactions were not detected, and the main effect of chlorpyrifos was not significant. Thus, results were pooled over chlorpyrifos rates and analyzed by flumioxazin rate. The SR50 for the R-biotype was 17 times greater than that of the S-biotype in these experiments (unpublished data). There was insufficient evidence to suggest metabolism is associated with flumioxazin tolerance levels. Organophosphate insecticides have been previously used to analyze the role of metabolism with PPO-inhibitor resistance in other species. Shoup et al. (Reference Shoup, Al-Khatib and Peterson2003) found no differences between a PPO-resistant biotype and an S-biotype of A. tuberculatus treated with acifluorfen or lactofen. Similar findings were reported by Shoup and Al-Khatib (Reference Shoup and Al-Khatib2005) in A. tuberculatus biotypes. Price et al. (Reference Price, Pline, Wilcut, Cranmer and Danehower2004a) reported that cotton at the 12-leaf growth stage had sevenfold greater metabolism of flumioxazin than plants at the 4- or 8-leaf growth stage.
Researchers have noted that the susceptibility of weeds such as ivyleaf morningglory (Ipomoea hederacea Jacq.), pitted morningglory (Ipomoea lacunosa L.), and prickly sida (Sida spinosa L.) to flumioxazin was associated with slower metabolism than found in tolerant species (Price et al. Reference Price, Wilcut and Cranmer2004b). Oxidative degradation of PPO inhibitors through plant metabolism can lead to detoxification of the parent herbicide. However, metabolites of PPO inhibitors may also have herbicidal properties that increase phytotoxicity. Dayan et al. (Reference Dayan, Armstrong and Weete1998) found the 3-hydroxymethyl derivative of sulfentrazone inhibited the PPO enzyme of soybean to a greater extent than the parent herbicide. The fate of flumioxazin and other PPO inhibitors warrants further investigation to better understand the physiological behavior associated with P. annua control.
Implications for Resistance Management
Poa annua resistance to PRE and POST herbicides is a major limitation for successful control in turfgrass. Flumioxazin has the potential to enhance control of populations in turf with resistance to other mechanisms of action. This research confirms that P. annua biotypes may exhibit differential tolerance levels to POST applications of flumioxazin despite limited selection pressure from previous use of PPO inhibitors. These findings suggest that this population of P. annua may comprise a high initial frequency of resistant individuals, leading to herbicide resistance once herbicides are used. They also suggest that differences in biochemical reactions among P. annua biotypes may be associated with inconsistencies in flumioxazin efficacy for POST control. Further research is needed to sequence the genes for the target-site enzymes to help further characterize the genetic basis of susceptibility to PPO inhibitors in various P. annua biotypes.
Acknowledgments
This research received no specific grant from any funding agency or commercial or not-for-profit sectors. No conflicts of interest have been declared. The authors would like to acknowledge Seth Williams, Bill Nutt, and V. J. Mantripragada for technical assistance with this research.