Introduction
The Bucephalidae Poche, 1907 (Platyhelminthes: Digenea) is a cosmopolitan family comprising many parasitic species of marine and freshwater fish. Most representatives of the family have been identified to the species level based on the morphology of mature worms. Molecular data are only available for relatively few species and do not yet provide a basis for the objective validation of worm species and analysis of the phylogenetic interrelationships of the family (Corner et al., Reference Corner, Cribb and Cutmore2020). In our study, morphological and molecular data were obtained for four representatives of the family Bucephalidae, including three from the subfamily Bucephalinae Poche, 1907 and one species of the subfamily Prosorhynchinae Nicoll, 1914 from freshwater and marine fish species of East Asia.
Materials and methods
Collection of trematodes
Trematodes were obtained from the following fish species: Huso dauricus (Georgi, 1775) Siniperca chuatsi (Basilewsky, 1855) of the Amur River (53°7′N, 140°40′E), Myoxocephalus tuberculatus Soldatov et Pavlenko, 1922, Myoxocephalus ochotensis Schmidt, 1929 and Myoxocephalus polyacanthocephalus (Pallas, 1814) of the Okhotsk Sea (54°14′N, 142°11′E). The above-mentioned fish species were collected during a scientific control catch conducted by the Khabarovsk branch of the Russian Federal Research Institute of Fisheries and Oceanography. Adult worms were also obtained from Strongylura strongylura (van Hasselt, 1823) fish in the coastal waters of Cat Ba Island, Vietnam (20°84ʹN, 106°59ʹE). Worms were rinsed in distilled water for a very short time, killed in hot distilled water, and preserved in 70% ethanol. Worms for molecular analysis were placed in 96% ethanol after fixation. Whole mounts for adult descriptions were made by staining the specimens with alum carmine, dehydrating the worms in a graded ethanol series and clearing in clove oil, followed by mounting in Canada balsam under a coverslip on a slide. All measurements are given in micrometres.
This material is held in the parasitological collection of the Zoological Museum (Federal Scientific Center of the East Asia Terrestrial Biodiversity, Far East Branch of the Russian Academy of Sciences, Vladivostok, Russia; e-mail: petrova@biosoil.ru). It was deposited on 20 November 2020.
DNA extraction, amplification and sequencing
Adult specimens of bucephalid trematodes preserved in 96% ethanol were used for molecular analysis (Table 1). Total DNA was extracted from individual flukes using a ‘hot shot’ technique (Truett, Reference Truett and Kieleczawa2006).
Table 1. List of Bucephalidae species and outgroup taxa used for 28S rDNA-based phylogenetic analysis

28S ribosomal DNA (rDNA) was amplified with the primers DIGl2 (5′-AAG CAT ATC ACT AAG CGG-3′) and 1500R (5′-GCT ATC CTG AGG GAA ACT TCG-3′) (Tkach et al., Reference Tkach, Littlewood, Olson, Kinsella and Swiderski2003). The ribosomal ITS1-5.8S-ITS2 fragment was amplified with the primers ITSF (5′-CGC CCG TCG CTA CTA CCG ATT G-3′) (Andres et al., Reference Andres, Pulis, Cribb and Overstreet2014) and S4R (5′-TAT GCT TAA ATT CAG CGG GT-3′) (Besprozvannykh et al., Reference Besprozvannykh, Tatonova and Shumenko2019). Initial PCR reaction was performed in a total volume of 25 μL containing 0.25 mm of each primer pair, 25 ng of total DNA in water, 12.5 μL GoTaq Green Master mix (Promega) Amplification of a 1200-bp fragment of 28S rRNA gene was performed in a GeneAmp 9700, Applied Biosystems, with a 5-min denaturation at 96°C, 35 cycles of 1 min at 96°C, 20 s at 55°C and 2 min 30 s at 72°C, and a 7-min extension at 72°C. Negative and positive controls using both primers were used. PCR products were directly sequenced using an ABI Big Dye Terminator v.3.1 Cycle Sequencing Kit (Applied Biosystems, USA), as recommended by the manufacturer, with the internal sequencing primers described by Tkach et al. (Reference Tkach, Littlewood, Olson, Kinsella and Swiderski2003) for 28S rDNA and by Luton et al. (Reference Luton, Walker and Blair1992) for ITS2 rDNA. PCR product sequences were analysed using an ABI 3130 genetic analyser at the Federal Scientific Center of the East Asia Terrestrial Biodiversity FEB RAS. Sequences were submitted to the GenBank database (NCBI).
Alignments and phylogenetic analysis
Ribosomal DNA sequences were assembled with SeqScape v.2.6 software, provided by Applied Biosystems. Alignments and estimations of the number of variable sites and sequence differences were performed using the MEGA 7.1 software (Kumar et al., Reference Kumar, Stecher and Tamura2016). Phylogenetic analysis was performed using the Bayesian algorithm with the MrBayes v. 3.1.2 software (Huelsenbeck et al., Reference Huelsenbeck, Ronquist, Nielsen and Bollback2001). The best nucleotide substitution model, TVM + I + G (Posada, Reference Posada2003) for 28S rDNA and TrN + I + G (Tamura and Nei, Reference Tamura and Nei1993) for ITS2 rDNA, were estimated with jModeltest v. 2.1.5 software (Darriba et al., Reference Darriba, Taboada, Doallo and Posada2012). Bayesian analysis was performed using 10 000 000 generations with two independent runs. Summary parameters and the phylogenetic tree were calculated with a burn-in of 25% of generations. The significance of the phylogenetic relationships was estimated using posterior probabilities (Huelsenbeck et al., Reference Huelsenbeck, Ronquist, Nielsen and Bollback2001). GenBank sequence data for representatives of Bucephallidae and outgroup taxa used in molecular analysis, including references and accession numbers are given in Table 1 and Supplementary Table 1.
Results
Rhipidocotyle husi n. sp.
Type-host: Huso dauricus (Georgi, 1775), Acipenseridae Bonaparte, 1831.
Type-locality: Amur River (53°7′N, 140°40′E).
Site: Intestine.
Prevalence: 3 of 4 fish.
Intensity of infection: 3–10 specimens.
Deposition: Holotype No. 160–Tr, paratypes No. 161–164–Tr.
Etymology: Species' name in concordance with generic name of definitive host species.
Adult worm (material examined: 5 specimens) (Fig. 1a; Table 2).
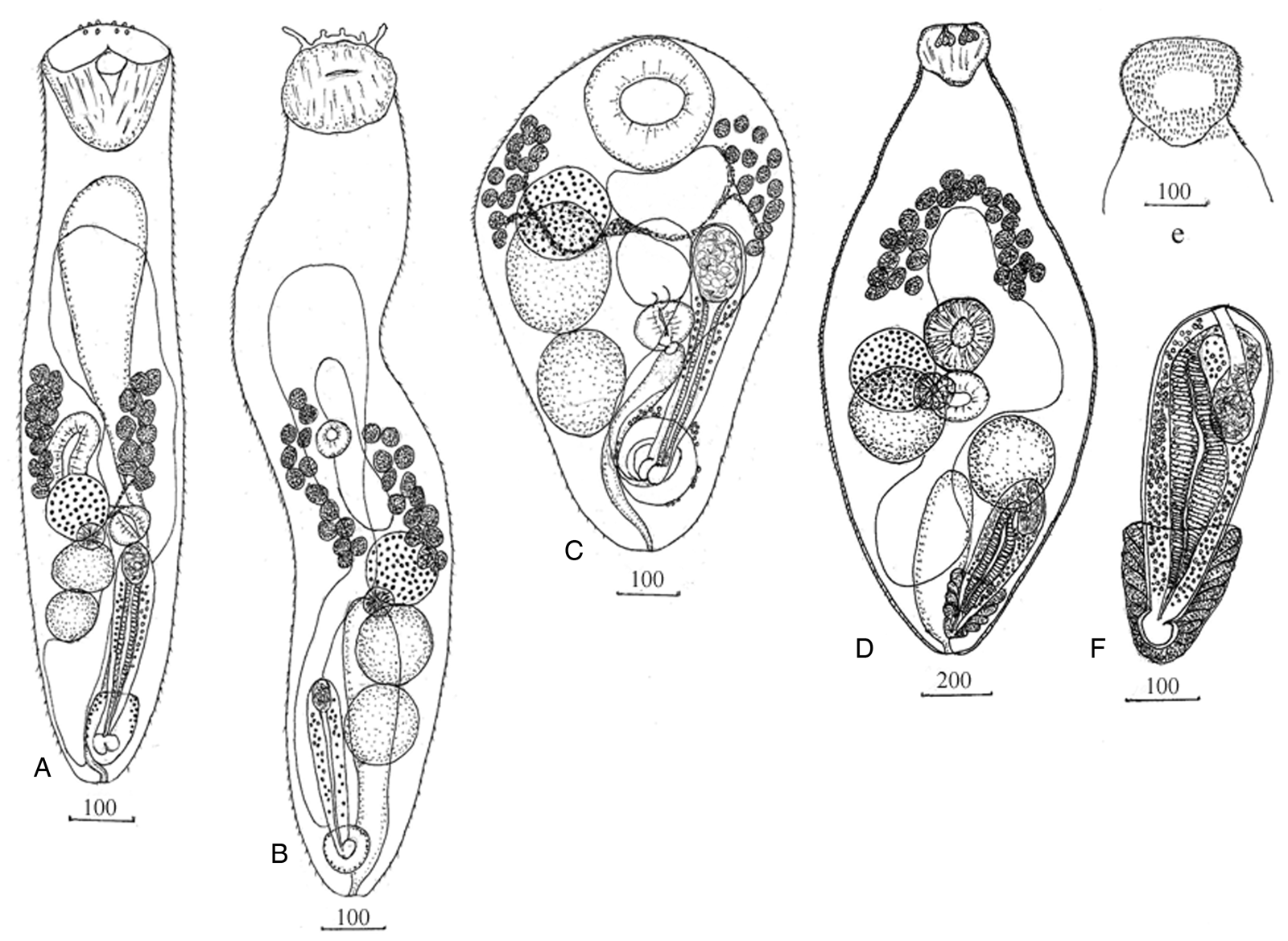
Fig. 1. Adult worms Bucephalidae. (a) Rhipidocotyle husi n. sp.; (b) Bucephalus skrjabini; (c) Prosorynchoides karvei; Prosorhynchus squamatus: (d) worm, (e) location of spines on the dorsal surface of Rhynchus, (f) cirrus sac.
Table 2. Adult worms Rhipidocotyle Diesing, 1858
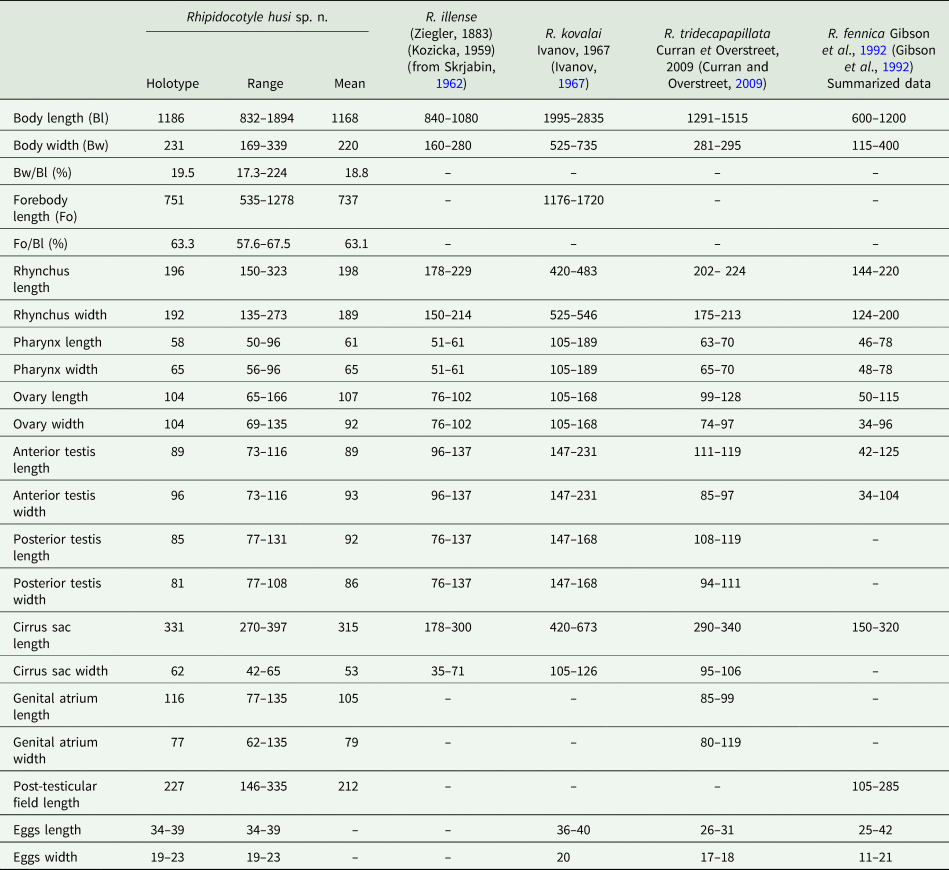
Body elongate with a narrowed posterior end. Tegument covered with needle-shaped spines. Rhynchus cup-shaped, simple muscular sucker surmounted by a muscular disc with a ventral notch and 10 papillae. Prepharynx not identified. Pharynx round or transversely oval in the middle of posterior half body. Caecum sac-like, thick-walled, located dextral and anterior to the pharynx. Testes tandem, contiguous, entire, oval or round, dextral to the median line of the body posterior to the pharynx. Cirrus sac elongate, club-shaped, sinistral, proximal end at the level of the testis. Seminal vesicle oval. Pars prostatica long, thick-walled with a large number of prostatic cells. Ejaculatory duct short. Genital pore opening ventro-subterminally into the genital atrium. Genital atrium near posterior end body, oval, surrounded by few glandular cells, contains bipartite genital lobe. Ovary spherical, dextral, pretesticular, contiguous with anterior testis. Mehlis' gland between the ovary and anterior testis. Uterus occupies most of the anterior half of the body, region at the level of sinistral vitelline field and posttesticular region. Eggs numerous, oval. Vitelline fields, two, each of 12–13 follicles, lateral, in the middle third of the body, posteriorly reach to anterior margin of the ovary. Excretory vesicle elongate, I-shaped anteriorly, at short distance posterior to rhynchus; pore terminal.
Molecular data
A ribosomal 28S rDNA fragment 1041 bp in length for 15 specimens of Rhipidocotyle husi n. sp. ex Huso dauricus was generated. All sequences were identical to each other. Nucleotide sequences were submitted to the NCBI database under the following numbers: (will be available after acceptance).
Remarks
Rhynchus structure, organs morphology and relative arrangement of worms extracted from kaluga (H. dauricus), correspond to diagnostic criteria of the genus Rhipidocotyle. Among representatives of Rhipidocotyle, two species have been reported from acipenserids, namely R. illense (Ziegler, 1883) and R. kovalai (Ivanov, Reference Ivanov1967), in the Danube River, the Amudarya River and the Volga-Caspian basin (Skrjabina, Reference Skrjabina1974). In East Asia, trematodes of this genus have not been found in acipenserid fish species until now. In comparison to the species mentioned above, the worm R. husi n. sp. was most similar to R. illense by metric and morphological parameters (Table 2). However, these worms differed from R. illense by the maximum values of all metric parameters (Table 2) and by the rhynchus structure and arrangement of vitellaria. The rhynchus disc of R. husi n. sp., in contrast to that of R. Illense, not lobed. Vitelline fields in the new species reached the ovary level, while in R. illense, they are distributed well anterior to the ovary. Among other Rhipidocotyle spp., R. husi n. sp. shows considerable morphometric similarity to R. tridecapapillata Curran and Overstreet, Reference Curran and Overstreet2009, which parasitizes Morone chrysops (Rafinesque, 1820), sMoronidae Jordan & Evermann, 1896, from the Luxapalila River, United States (Curran and Overstreet, Reference Curran and Overstreet2009). They are similar in body and organ sizes, except egg sizes (Table 2), and also both possess a rhynchus with a simple muscular sucker surmounted by a muscular disc, ventrally notched with papillae on the surface. Despite the morphometric similarities, they were identified from very different waterways with no common biogeographical history and belonged to different species based on host specificity and differences in egg sizes and vitellarium arrangement relative to the ovary. In R. tridecapapillata, in contrast to East Asian worms, the vitellarium was arranged at a distance anterior to the ovary. Another species, R. fennica Gibson, Taskinen, Valtonen, 1992, is also similar to worms obtained from H. dauricus. However, specimens of R. fennica have a smaller body and organs (Table 2), do not possess a rhynchus with a muscular disc with papillae on the surface, and infect percid and esocid fish species, which are taxonomically distant from acipenserids (Gibson et al., Reference Gibson, Taskinen and Valtonen1992). Therefore, we infer that the worms obtained from Huso dauricus caught in the Amur River represented a new species of the genus Rhipidocotyle, named R. husi n. sp.
The 28S ribosomal DNA (rDNA)-based phylogenetic tree topology showed that all specimens of R. husi n. sp. from our study were within the same highly supported clade as R. fennica (Fig. 2). However, these two species demonstrated polytomy rather than a common phylogenetic dichotomy, where R. fennica appeared as a well-supported group with a single branch included in the polytomy. Both species were distant from the five other Rhipidocotyle spp., including the type species R. galeata (Rudolphi, 1819), demonstrating the paraphyly of this genus. Rhipidocotyle husi n. sp. differed from R. fennica by 1.36 ± 0.35%. Substitution analysis indicated that the 28S rDNA fragment of R. husi n. sp. differed from that of R. fennica by 14 variable sites, which contained 11 transitions and 3 transversions that, along with morphological differences, support the validity of R. husi n. sp.

Fig. 2. Bayesian phylogenetic tree of Bucephalidae reconstructed on the basis of 28S rDNA sequences. Original sequences are unbolded. Nodal numbers – a posterior probability values (only significant values presented).
Bucephalus skrjabini Akhmerov, 1963
Host: Siniperca chuatsi (Basilewsky, 1855), Percichthyidae Jordan & Eigenmann, 1890.
Locality: Amur river (53°7′N, 140°40′E).
Site: Intestine.
Prevalence: 3 of 5 fish.
Intensity of infection: 1–12 specimens.
Voucher deposition: No. 165–169–Tr.
Adult worm (material examined: 5 specimens) (Fig. 1b; Table 3).
Table 3. Adult worms Bucephalus Baer, 1827 and Prosorynchoides Dollfus, 1929

Body elongate with posterior end tapered. Tegument covered with needle-shaped spines. Rhynchus spherical with irregular surface and tentacles contains groups of longitudinal muscles. Prepharynx not identified. Pharynx round or transversely oval immediately pre-equatorial, post-equatorial or equatorial. Caecum sac-like, in the midline, anterior or posterior to the pharynx. Testes tandem, contiguous, entire, oval or round, in the posterior third of the body. Cirrus sac elongated, club-shaped, dextral to testes, extends the level of the posterior end of the anterior testis or anterior end posterior testis. Seminal vesicle oval. Pars prostatica long, thin-walled with a large number of prostatic cells. Genital pore opening ventro-subterminally into genital atrium. Genital atrium near of posterior end body, irregular containing genital lobe. Ovary spherical, pretesticular, contiguous or separated with anterior testis. Mehlis' gland between the ovary and anterior testis. Uterus occupies most of the anterior half of the body, region at the level of dextral vitelline field and partly posttesticular region. Eggs numerous, oval. Vitelline fields, two, each of 13–14 follicles, lateral, in the middle third of the body. The anterior end of the dextral vitelline field is slightly anterior or at the level of the pharynx, sinistral vitelline field is anterior at the level of the pharynx. Posterior end of vitelline fields at level of the ovary. Excretory vesicle elongated, I-shaped extend to ovary, pore terminal.
Molecular data
A ribosomal 28S rDNA fragment 1040 bp in length for 5 specimens of Bucephalus skrjabini ex S. chuatsi was generated. One of five sequences contained a single T/C transition at nucleotide position #260. Nucleotide sequences were submitted into the NCBI database under the following numbers: (will be available after acceptance).
Remarks
Bucephalus skrjabini was first detected by Akhmerov (Reference Akhmerov and Schikhobalova1963) in Chinese perch (S. chuatsi Basilewsky, 1855) in the Amur River. Mature specimens of B. skrjabini were collected from the same fish host in this study. Morphologically, these worms agreed well with the original description. There were differences between these worms in body length and ovary and cirrus sac sizes (Table 3). However, in Akhmerov (Reference Akhmerov and Schikhobalova1963) publication, one of the specimens presented in the figures does not exceed 1.375 μm (on the basis of the provided scale), a distinct discrepancy in the metric parameters of body length. At the same time, discrepancies in the sizes of the ovary and cirrus sac probably reflect the intraspecific variation. Based on host species, locations, and similarities in morphology, we considered the worms from our study to be B. skrjabini.
Prosorynchoides karvei Bhalerao, Reference Bhalerao1937
Host: Strongylura strongylura (van Hasselt, 1823), Belonidae Bonaparte, 1835.
Locality: Halong Bay East; 20°84ʹN, 106°59ʹE.
Site: Intestine.
Prevalence: 3 of 5 fish.
Intensity of infection: 1–10 specimens.
Voucher deposition: No. 170–176–Tr.
Adult worm (material examined: 7 specimens) (Fig. 1c; Table 3).
Body small, inversely pear-shaped. Tegument covered with spines. Rhynchus simple muscular sucker, large, spherical. Mouth opening median, postequatorial. Prepharynx short. Pharynx round or transversely oval at level posterior testis. Oesophagus short. Caecum short, sac-like, extends to anteriorly from the pharynx at the level of the anterior testis. Testes tandem, contiguous or partly overlapping, entire, oval or round, dextral to the median line of the body. The anterior testis is mostly located in the anterior half of the body. Posterior testis located in the posterior half body. Cirrus sac elongated extends to the level of the anterior testis. Seminal vesicle oval. Pars prostatica long with a large number of prostatic cells. Ejaculatory duct opening into the genital atrium. Genital atrium near of posterior end body surrounded by few glandular cells, contains bipartite genital lobe. Ovary spherical, pretesticular, or overlapping anterior testis and can be partially covered by rhynchus. Uterus filling most of the anterior body space. Eggs numerous, oval. Vitelline fields, in 2 lateral groups of 10–15 rounded follicles, usually extent from the level middle of rhynchus to level anterior half anterior testis, rare locate at level ovary and anterior of testis. Excretory vesicle elongated, I-shaped extending to pharynx or caecum. Excretory pore terminal.
Molecular data
Ribosomal 1034 bp 28S and 556 bp ITS2 rDNA fragments were generated for 4 and 5 specimens of Prosorhynchoides karvei ex S. strongylura, respectively. All 28S rDNA sequences were identical to each other and for ITS 2 three variable singleton substitutions were revealed. Nucleotide sequences were submitted into the NCBI database under the following numbers: (will be available after acceptance).
Remarks
The genus Prosorynchoides is cosmopolitan and comprises numerous morphologically similar species that are intestinal parasites of a wide range of definitive hosts, including marine and freshwater fish species. The considerable morphological similarity is typical, for example, for Prosorynchoides ozakii (Nagaty, Reference Nagaty1937); P. karvei, with a number of synonymous species; P. fijiensis Manter, Reference Manter1963; P. galaktionovi Hammond, Cribb, Nolan, Bott, 2020; and P. kohnae Hammond, Cribb, Nolan, Bott, 2020 (Bhalerao, Reference Bhalerao1937; Manter, Reference Manter1963; Urabe et al., Reference Urabe, Ogawa, Nakatsugawa, Nakai, Tanaka and Wang2007; Maurya et al., Reference Maurya, Gupta and Saxena2018; Hammond et al., Reference Hammond, Cribb, Nolan and Bott2020). The geographic distribution of these worms ranges from coastal waters of Japan to India, as well as coastal waters of Fiji, Australia and French Polynesia. Species differentiation and synonymization of most known representatives of Prosorynchoides based only on morphological data create difficulties in the validation or invalidation of a great number of representatives in the genus Prosorynchoides. This challenges the estimation of both interrelationships within Prosorynchoides and intergeneric relationships within the subfamily.
The morphological characteristics of worms from Vietnamese S. strongylura unambiguously indicated membership of these worms in Prosorynchoides; they are morphologically similar to P. karvei, P. fijiensis, P. galaktionovi and P. kohnae. Specimens of P. karvei have been detected in fish species of the families Schilbeidae Bleeker, 1858 and Belonidae Bonaparte 1832, in particular in Indian S. strongylura. Specimens of P. fijiensis have been detected in Strongylura gigantea (Teraminck and Schlegel, 1846) from the coastal waters of Fiji. In that case, P. fijiensis was described on the basis of a single mature specimen (Manter, Reference Manter1963), and the species was delimitated from P. karvei by the presence of a relatively long prepharynx and extension of the excretory bladder. Comparative analysis of morphometric data (Table 3) showed no difference for most of the parameters between worms from Vietnam and specimens of P. karvei from the studies of Bhalerao (Reference Bhalerao1937), Gupta (Reference Gupta1956) and Maurya et al. (Reference Maurya, Gupta and Saxena2018) and specimens of P. fijiensis from Manter (Reference Manter1963). We think that the criteria used by Manter (Reference Manter1963) for differentiation of P. fijiensis and P. karvei, namely differences in prepharynx length and excretory bladder extension, are not sufficient to delimit these species. In our material, some specimens had a prepharynx and some did not. For P. karvei, Maurya et al. (Reference Maurya, Gupta and Saxena2018) denote considerable variation in relative organ reciprocal arrangement and extension of the excretory bladder. Given this variability, and given the shared infection of belonid fish species as definitive hosts in the Indo-Malaysian region and Oceania it cannot be excluded that P. fijiensis represents a junior synonym of P. karvei. The final conclusion about the membership of these worms to the same or different species can only be made by generating additional molecular data for specimens from India and Fiji. As we mentioned above, the worms from our study are morphologically similar to P. galaktionovi and P. kohnae, parasites of belonid fish species from Australia and French Polynesia. However, there were differences in most of the metric parameters (Table 3). Moreover, P. galaktionovi and P. kohnae are more similar to each other than to Vietnamese Prosorynchoides species based on body and organ sizes. On the basis of the aforementioned information, we identify the new specimens from Vietnamese S. strongylura as P. karvei.
Phylogenetic reconstructions showed that P. karvei is closely related to P. kohnae and P. galaktionovi within a highly supported polytomic clade (Fig. 2). The genetic p-distance values between P. karvei and two closely related species, P. kohnae and P. galaktionovi, were 0.34 ± 0.18% and 0.66 ± 0.25%, respectively. Additionally, P. karvei differed from P. kohnae by four transitions, namely three A/G and one T/C substitution. Because P. kohnae was represented by a single 28S rDNA sequence, all four substitutions were classified as singletons until additional data on this species become available. Prosorhynchoides karvei and P. galaktionovi differed by seven fixed transitions, namely four A/G and three T/C substitutions. Of these, five substitutions were similar in P. karvei and P. kohnae, and two in P. galaktionovi and P. kohnae. These results clearly demonstrated that P. karvei and P. galaktionovi differ from each other at the interspecific level. However, a statement cannot be made regarding P. karvei and P. kohnae; in other words, there were no significant differences between P. karvei and P. kohnae to be interpreted as interspecific based on the 28S rDNA sequence data. According to the available data, the only differences between these species were metric parameters of the body and organs (Table 3). Prosorhynchoides kohnae, P. karvei and P. galaktionovi were located within the same monophyletic clade with P. cutmorei, P. waeschenbachae and P. moretonensis (Fig. 2). The genetic p-distance within this clade ranged from 0.34 ± 0.18% to 1.87 ± 0.41%. Another well-supported sister clade consisted of four species of the genus Bucephalus, namely B. cynoscion, B. varicus, B. margaritae and B. gorgon, and three Prosorhynchoides spp., P. gracilescens, P. ovatus and P. paralichthydis. Within this clade, there were two lineages, each including representatives of both genera with internal p-distance values from 1.94 ± 0.41% (B. varicus/P. gracilescens) to 5.42 ± 0.47% (B. varicus/B. cynoscion) and from 3.63 ± 0.52% (B. gorgon/P. paralichthydis) to 4.32 ± 0.52% (P. ovatus/P. paralichthydis). Differentiation between these lineages ranged from 3.53 ± 0.56% (P. paralichthydis/P. gracilescens) to 5.52 ± 0.68% (B. cynoscion/B. gorgon). Rhipidocotyle transversalis was a sister to these two clades, with p-distance values from 4.26 ± 0.59% to 5.82 ± 0.69% and from 4.31 ± 0.63% to 5.52 ± 0.68%, respectively. These values corresponded with p-distances both within and between two observed clades. These results indicated that the first clade could be recognized as the monophyletic genus Prosorhynchoides, whereas the second clade may have united representatives of one or several genera that belong to neither Bucephalus nor Prosorhynchoides, as well as worms identified as R. transversalis that do not belong to the genus Rhipidocotyle.
Additionally, we performed an analysis of ITS2 sequence data of P. karvei and closely related species. The obtained results showed a genetic differentiation of 0.6 ± 0.34% to 0.8 ± 0.39% within P. karvei (including GenBank data), 0.81 ± 0.39% to 1.44 ± 0.54% between P. karvei and P. galaktionovi, and 1.43 ± 0.52% to 2.07 ± 0.67% between P. karvei and P. kohnae. Additionally, 1.01 ± 0.45% to 1.21 ± 0.47% of Differentiation from 1.01 ± 0.45% to 1.21 ± 0.47% was revealed between P. karvei and P. cutmorei. Phylogenetic analysis based on ITS2 rDNA sequences showed that P. karvei and P. galaktionovi were within a polytomic clade, which provides no basis for the differentiation of these two species (Fig. 3, Supplementary Fig. 1). Prosorhynchoides cutmorei was sister to the P. karvei + P. galaktionovi clade, and P. kohnae was a basal relative to these three species. These data confirmed the species validity of P. cutmorei and P. kohnae. Despite some metric differences between P. karvei and P. galaktionovi, the conspecificity of these worms cannot be excluded on the basis of available molecular data and accepting the morphological similarity of these trematodes.

Fig. 3. The fragment of Bayesian phylogenetic tree of Prosorhynchoides species reconstructed on the basis of ITS2 rDNA sequences. Original sequences are unbolded. Nodal numbers – a posterior probability values (only significant values presented).
Prosorhynchus cf. squamatus Odhner, 1905
Host: Myoxocephalus polyacanthocephalus (Pallas, 1814), M. ochotensis Schmidt, 1929, M. tuberculatus Soldatov et Pavlenko, 1922, Cottidae Bonaparte, 1831.
Locality: Okhotsk Sea (54°14′N, 142°11′E).
Site: Intestine.
Prevalence: 1 of 1 in three species of fish.
Intensity of infection: 2–12 specimens.
Voucher deposition: No. 177–181–Tr.
Adult worm (material examined: 5 specimens from M. polyacanthocephalus) (Fig. 1d–f; Table 4).
Table 4. Adult worms Prosorhynchus Odhner, 1905 and Dollfusitrema Eckmann, 1934

a Summarized data from Odhner, 1905, Isaiitschikow, 1928, Layman, 1930, Miller, 1941.
b Summarized data from Odhner, 1905, Ozaki, 1928, Yamaguti, 1938, Layman, 1930, Linton, 1940 (from Skrjabin, Reference Skrjabin1962).
Body fusiform is widest at the level of the middle of the body. Tegument covered with spines. Rhynchus apex, cup-shaped, with longitudinal muscles and six drop-shaped glandular cells, a ventral surface without spines, dorsal surface spines absent from the central region. Prepharynx not identified. Pharynx spherical or subspherical immediately pre-equatorial, post-equatorial or equatorial. Caecum sac-like, thiсk-walled, located in midline anteriorly to the pharynx. Testes two, spherical, opposite, diagonally, dextral testis at the level of pharynx, sinistral testis posterior to the pharynx. Cirrus sac elongated, club-shaped, sinistrally anteriorly reaches at a level of the left testis. Seminal vesicle elongated, curved. Pars prostatica long, thiсk-walled, glandular with a large number of prostatic cells. The ejaculatory duct is short, narrow. Genital pore opening ventral – subterminally into of genital atrium. Genital atrium near the posterior end of the body, elongate with genital lobe, surrounded by numerous large glandular cells. Ovary spherical, dextral to the pharynx and overlapping with the right testis. Mehlis' gland at a level to region overlap of the ovary and testis. Uterus extend anterior to caecum, posteriorly reaches of the level genital atrium. Eggs numerous, oval. Vitelline fields, two, each of 13–14 follicles in a confluent arc in the middle of the anterior half of body, posteriorly of vitelline fields reaches of level end caecum. Excretory vesicle elongated, I-shaped reaches level sinistral of testis, pore terminal.
Molecular data
A ribosomal 28S rDNA fragment 1040 bp in length was generated for 5 specimens of Prosorhynchus squamatus from three fish species. One specimen of P. squamatus ex Myoxocephalus tuberculatus differs from trematodes from the other two host species by three variable sites, contained two T/C transitions at sites #536 and #665 and one T/A transversion at site #944. Nucleotide sequences were submitted into the NCBI database under the following numbers: (will be available after acceptance).
Remarks
From our study, worms collected from Myoxocephalus Tilesius, 1811 from coastal waters of Kamchatka, were morphologically similar to the cosmopolitan Prosorhynchus crucibulum (Rudolphi, 1819) Odhner, 1905 and P. squamatus Odhner, 1905, which were first reported in the intestines of marine eels (Conger (Linnaeus, 1758)) and gobies (Cottus Linnaeus, 1758; = Myoxocephalus), respectively. Subsequent studies reported P. squamatus from fish in the families of Rajidae Bonaparte, 1831, Cottidae Bonaparte, 1831 and Salmonidae Cuvier, 1816 (Pratt and McCauley, Reference Pratt and McCauley1961; Cheung, Reference Cheung and Stoskopf1993; McDonald and Margolis, Reference McDonald and Margolis1995; Benz and Bullard, Reference Benz, Bullard, Smith, Warmolts, Thoney and Hueter2004; Kuklin et al., Reference Kuklin, Kuklina and Kisova2012). Fish species from these three families, denoted as definitive hosts for P. crucibulum, and Hemitripteridae Cuvier, 1829 and Hexagrammidae Gill, 1889 have been identified as definitive hosts of P. squamatus (Shvetsova and Pozdnyakov, Reference Shvetsova, Pozdnyakov and Pozdnyakov1999). Assessments about the validity of these worms are controversial because of morphological similarities. Some authors believed that P. squamatus is a junior synonym of P. crucibulum (Eckmann, Reference Eckmann1932; Nagaty, Reference Nagaty1937; Dawes, Reference Dawes1947; Zhukov, Reference Zhukov1960), while others considered these worms a separate valid species (Polyansky, Reference Polyansky1955; Brinkmann, Reference Brinkmann1957). Moreover, the type species of the genus Prosorhynchus was recognized as either P. crucibulum by Skrjabin (Reference Skrjabin1962) or P. squamatus by Overstreet and Curran (2002). As mentioned above, worms from our study had no significant morphological or pronounced metric differences (Table 4) relative to P. crucibulum and P. squamatus. Morphological similarity of the worms can be evidence of its belonging to the same species. Nevertheless, the results of some studies indicate that morphological similarity of mature worms is not always sufficient for regarding those worms as the same species. For this reason, the question of the taxonomic status of S. squamatus and P. crucibulum could be resolved using molecular data for these worms from type locations from representatives of Myxocephalus и Conger, respectively. We identify the worms from our material as P. squamatus on the basis of its occurrence in Myxocephalus (Cottidae) – the type host for this trematode, whereas for P. crucibulum the type host is species of the genus Conger (Congridae Kaup, 1856).
It is important to note that, based on morphological characteristics, worms of the discussed species were similar to Prosorhynchus brayi Cutmore, Nolan and Cribb, Reference Cutmore, Nolan and Cribb2018, obtained from Epinephelus Bloch, 1973 in Moreton Bay, Queensland, Australia (Cutmore et al., Reference Cutmore, Nolan and Cribb2018). However, P. brayi differed from both P. crucibulum and P. squamatus by most metric parameters (Table 4).
Phylogenetic analysis showed that, for the general phylogenetic tree (Fig. 2), the genus Prosorhynchus was paraphyletic. Prosorhynchus cf. squamatus is closely related to species of the genus Dollfustrema Eckmann, 1934 within a highly supported polytomic clade, with p-distance values from 4.06 ± 0.58% to 5.03 ± 0.68%. Five Prosorhynchus spp. from the GenBank database formed a distinct, highly supported clade, sister to Prosorhynchus cf. squamatus/Dollfustrema (Fig. 1). Within this clade, the p-distances ranged from 1.55 ± 0.4% (P. maternus Bray, Justine, Reference Bray and Justine2006/P. luzonicus Velasquez, 1959) to 4.95 ± 0.63% (P. longisaccatus Durio and Manter, Reference Durio and Manter1968/P. brayi). Genetic differentiation between representatives of these two clades ranged from 5.05 ± 0.66% (P. cf. squamatus/P. luzonicus Velasquez, 1959) to 7.18 ± 0.77% (D. hefeiensis Liu, 1999/P. longisaccatus). At the same time, P. cf. squamatus and D. gibsoni Nolan, Cribb, Reference Nolan and Cribb2010 from one clade and P. brayi from another clade were characterized by close metric values (Table 2), and morphological similarity based on the body form, relative arrangement of organs, and the structure of vitellaria. By the last character, Prosorhynchus cf. squamatus is similar to both D. durum and P. longisaccatus as well. Alongside this, Prosorhynchus cf. squamatus differs from D. durum by elongate body and ovary arrangement. Nevertheless, these two species are within the same subclade. Prosorhynchus longisaccatus from the sister subclade most similar to D. durum by body form and ovary arrangement relative to the anterior testis (from the right side or partially behind anterior testis) (Durio and Manter, Reference Durio and Manter1968; Nolan et al., Reference Nolan, Curran, Miller, Cutmore, Cantacessi and Cribb2015). Prosorhynchus pacificus Manter, Reference Manter1940, P. maternus, and P. luzonicus from corresponding subclades have an elongate body and vitellaria fields do not unite anteriorly (confluent arc) (Manter, Reference Manter1940; Bray and Justine, Reference Bray and Justine2006; Cutmore et al., Reference Cutmore, Nolan and Cribb2018). Thus, within this clade there are species that are both similar to each other morphologically and some of them differ from each other in body form, vitellaria structure and ovary arrangement relative to the anterior testis. Unfortunately, we were unable to trace the original description of D. hefeiensis as well as D. vaneyi, which was distinguished from D. hefeiensis, based on molecular data (Chen et al., Reference Chen, Wang, Yao and Nie2007).
The problem of the taxonomy of the species studied here is not resolved by analysis of morphological characteristics or by the definitive host specificity of these trematodes. Prosorhynchus cf. squamatus and Dollfustrema spp. each forms a clade that infects fish of the Scorpaeniformes Greenwood et al., 1966 and Anguilliformes, respectively (Nolan and Cribb, , Reference Nolan and Cribb2010; Cutmore et al., Reference Cutmore, Nolan and Cribb2018). However, if the worms identified as P. squamatus and P. crucibulum (type host fish species of Anguilliformes) are recognized as a single species, then fish of Anguilliformes will be considered definitive hosts alongside others for these trematodes, as well as for Dollfustrema spp. Within sister clade, only Prosorhynchus spp. with definitive hosts from Perciformes are presented. Accepting the above-mentioned morphological characteristics of representatives of the considered clades and similar values of molecular differentiation within and between clades, the hypothetical congeneric nature of all representatives from these clades cannot be excluded. However, final conclusions in this respect require additional data on definitive host specificity and molecular data both nuclear and mitochondrial markers, Prosorhynchus cf. squamatus was subdivided into two lineages, including specimens obtained from Myoxocephalus polyacanthocephalus (Pallas, 1814) and Myoxocephalus ochotensis Schmidt (voucher numbers 1811 and 1884) and single specimens obtained from M. tuberculatus (voucher number 1883; Figure 2, Table 1). Variation in the 28S rDNA of these trematodes can presently be characterized as intraspecific. However, additional material from M. tuberculatus is needed to explore the depth of this variation.
Discussion
Corner et al. (Reference Corner, Cribb and Cutmore2020) reported that complex data, including the simultaneous generation of morphological and molecular characteristics, are needed to resolve systematic and phylogenetic relationships within Bucephalidae. The identification of worm species using molecular data but without general morphological description does not resolve the issue when species from different genera cluster in one clade. The complexity of the problem can be seen from the phylogenetic structure of Bucephalidae (Fig. 2). In several cases, worms identified as members of different genera clustered together, suggesting that they belong to the same genus. On the basis of general reconstruction using overall molecular data (Fig. 2), the family Bucephalidae is monophyletic, but the genera Rhipidocotyle, Bucephalus, Prosorhynchoides and Prosorhynchus are poly- or paraphyletic, an outcome that has also been shown in previous studies (Nolan et al., Reference Nolan, Curran, Miller, Cutmore, Cantacessi and Cribb2015; Hammond et al., Reference Hammond, Cribb and Bott2018, Reference Hammond, Cribb, Nolan and Bott2020; Corner et al., Reference Corner, Cribb and Cutmore2020). For this reason, we also performed phylogenetic analysis of Bucephalidae using our material and GeneBank molecular data on bucephalid species supported by morphological descriptions (Fig. 4). This restricted analysis includes representatives of seven genera (Bucephalus with only one representative). Of these, Aenigmatrema and Prosorhynchus are not monophyletic. Rhipidocotyle represents a monophyletic subclade with a single specimen of R. fennica and specimens of R. husi n. sp. The genus Prosorhynchoides is also monophyletic, P. karvei is closely related to P. galaktionovi and P. kohnae, forming a shared terminal subclade with highly supported polytomy. Another three species from Australia, P. cutmorei, P. waeschenbachae and P. moretonensis (Genbank data), appeared as distinct branches within a monophyletic clade, including the P. karvei + P. galaktionovi + P. kohnae group.
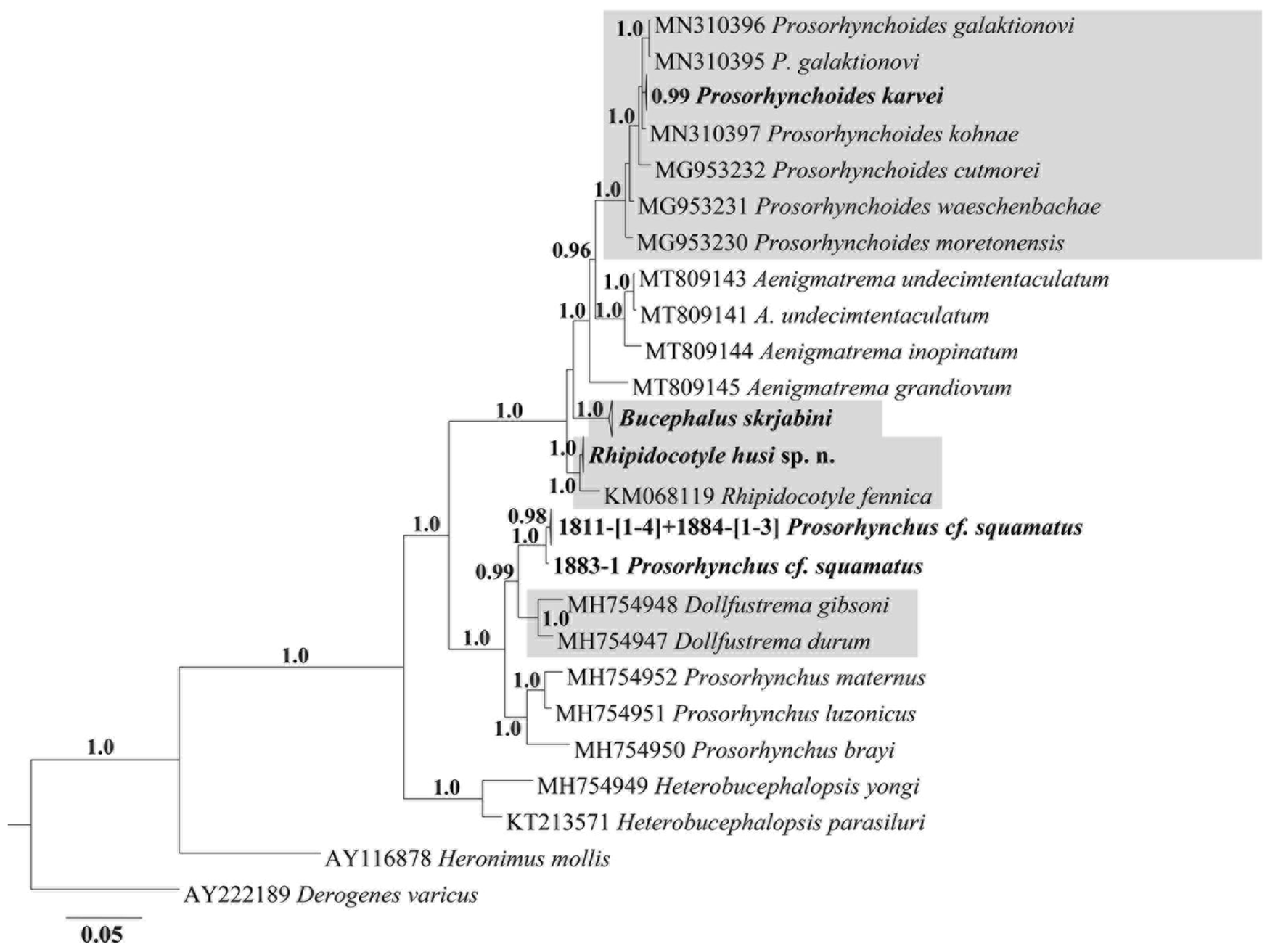
Fig. 4. Bayesian phylogenetic tree of morphologically validated species of Bucephalidae reconstructed on the basis of 28S rDNA sequences. Original sequences are unbolded. Monophyletic genera are framed. Nodal numbers – a posterior probability values (only significant values presented).
Similar to the full tree, in the reduced phylogenetic tree, the genus Prosorhynchus maintained paraphyly, and Prosorhynchus cf. squamatus was closely related to Dollfustrema species, supporting our suggestion relative to the taxonomic status of Prosorhynchus and Dollfustrema provided above.
Supplementary material
The supplementary material for this article can be found at https://doi.org/10.1017/S0031182022000208.
Financial support
This work is a part of the state-supported studies in the Federal Scientific Center of the East Asia Terrestrial Biodiversity of FEB RAS (project no. 0267-2019-0018).
Conflict of interest
The authors declare there are no conflicts of interest.