Introduction
Microsentis wardae Martin & Multani, Reference Martin and Multani1966 is one of the smallest and most host and geographically restricted acanthocephalans known to date. It was well described with 12-line drawings from the long-jaw mudsucker Gillichthys mirabilis Cooper (Gobiidae) at Scammon's Lagoon in Baja California. It was reported another time since from the same host species and near the same Lagoon in Baja California but not in any of the geographically proximal locations at the Salton Sea, Seal Beach estuaries, Newport Bay and San Francisco Bay by Martin & Multani (Reference Martin and Multani1970). The only other report of M. wardae was by García-Prieto et al. (Reference García-Prieto, García-Varela, Mendoza-Garfias and Pérez-Ponce de León2010) from the same host species in Laguna Ojo de Liebre (also the same Scammon's Lagoon). This coastal lagoon is located in Mulegé Municipality near the town of Guerrero Negro in the northwestern Baja California Sur state of Mexico. It lies approximately halfway between the southern tip of the Baja California Peninsula and the United States–Mexico border, opening onto the Pacific Ocean (Anonymous, 2009; Phleger & Clifford, Reference Phleger and Clifford2009). The lagoon is within the Vizcaíno Biosphere Reserve United Nations Educational, Scientific and Cultural Organization World Heritage Site and is a Ramsar wetlands site. This restricted host and geographical distribution of this small acanthocephalan made it less readily accessible for further studies.
A small collection of fully developed adults of M. wardae, from the rectum of G. mirabilis collected in Anaheim Bay, California have just become available for our study. Anaheim Bay is a wetland salt marsh complex in Orange County about 759 km north of Scammon's Lagoon where M. wardae was originally described. These mudsuckers occur in estuaries, primarily in tidal sloughs with shallow mud-covered bottoms, where they often excavate burrows. Their range extends about 1828 km from Tomales Bay near San Francisco in the north to Bahia Magdalena, Gulf of California, in the south (Eschmeyer et al., Reference Eschmeyer, Herald and Hamann1983; García, Reference García2018). Our present study reports and describes acanthocephalans using scanning electron microscopy (SEM) for the first time and provides new information on its hook biochemistry and molecular biology. The study is the first report to generate molecular data for M. wardae and to assess the systematic position of the genus Microsentis within Neoechinorhynchidae using molecular data from the 18S nuclear ribosomal gene.
Materials and methods
Collections
Fourteen specimens were collected from the rectum of longjaw mudsuckers, G. mirabilis Cooper (Gobiidae) in the salt marshes of Anaheim Bay, California (33.735°N 118.094°W) in December 2017. Twelve specimens were made available. About 30 additional specimens were obtained from 30 examined hosts in Emory Cove Marsh, San Diego Bay (32.6717°N, 117.1441°W) in October 2021. Eight specimens from Anaheim Bay and nine specimens from San Diego Bay were processed for microscopical examination: three for SEM; and five for molecular analysis. The five specimens from Anaheim Bay used for molecular analysis were not properly fixed and were replaced by six specimens from the 2021 San Diego collection.
Deposited material
Specimens were deposited in the University of Nebraska's State Museum's Harold W. Manter Laboratory (HWML) collection, Lincoln, Nebraska.
Methods for microscopical studies
Worms were punctured with a fine needle and subsequently stained in Mayer's acid carmine, destained in 4% hydrochloric acid in 70% ethanol, dehydrated in ascending concentrations of ethanol (24 h each), cleared in 100% xylene and then in Canada balsam xylene in equal proportions (24 h each). Whole worms were then mounted in Canada balsam. Measurements are in micrometres, unless otherwise noted; the range is followed by the mean values in parentheses. Width measurements represent maximum width. Trunk length does not include proboscis, neck, or bursa.
SEM
Specimens that had been fixed and stored in 70% ethanol were processed for SEM following standard methods (Lee, Reference Lee1992). These included critical point drying and mounting on aluminium SEM sample mounts (stubs) using conductive double-sided carbon tape. The sample was sputter coated with an 80%–20% gold–palladium target for 3 min using a sputter coater (Quorum (Q150T ES) www.quorumtech.com) equipped with a planetary stage, depositing an approximate thickness of 20 nm. The sample was placed and observed in an FEI Helios Dual Beam NanoLab 600 (FEI, Hillsboro, Oregon) scanning electron microscope (FEI, Hillsboro, Oregon). Samples were imaged using an accelerating voltage of 5 kV, and a probe current of 86 pA, at high vacuum using a secondary electron detector.
Focused ion beam (FIB) sectioning of hooks
A dual-beam SEM with gallium ion source (GIS) is used for the liquid ion metal source (LIMS) part of the process. The gallium beam (LIMS) is a gas injection magnetron sputtering technique whereby the rate of cutting can be regulated. The hooks were studied intact and sectioned at two positions (longitudinal sections and cross-sections) using the FEI Helios Dual Beam NanoLab 600 mentioned above. The dual-beam FIB/SEM is equipped with a gallium LIMS. The hooks of the acanthocephalans were centred on the SEM stage and sections were made using an ion accelerating voltage of 30 kV and a probe current of 2.7 nA following the initial cut. The time of cutting is based on the nature and sensitivity of the tissue. The sample also goes through a cleaning cross-section milling process to obtain a smoother surface. The cut was analysed using an X-ray for chemical ions with an electron beam (tungsten) to obtain an X-ray spectrum. The intensity of the GIS was variable according to the nature of the material being cut. Results were stored with the attached imaging software then transferred to a Universal Serial Bus for future use.
Energy dispersive X-ray analysis (EDXA)
The Helios NanoLab 600 is equipped with an EDAX (Mahwah, NJ) TEAM Pegasus system with an Octane Plus detector. The intact hook and the sectioned cuts were analysed by EDXA. Spectra of selected areas were collected from the centre and the edge of each cross-section or longitudinal section. EDXA spectra were collected using an accelerating voltage of 15 kV and a probe current of 1.4 nA. Data collected included images of the displayed spectra as well as the raw collected data. Relative elemental percentages were generated by the TEAM software.
Molecular methods
The DNA extraction from four specimens of M. wardae was done using DNeasy® Blood & Tissue Kit (QIAGEN, Hilden, Germany) following the manufacturer's instructions. The 18S region of nuclear ribosomal DNA was amplified by polymerase chain reaction (PCR) using the primer set 18SU467F (forward, 5′-ATCCAAGGAAGGCAGCAGGC-3′) and 18SL1310R (reverse, 5′-CTCCACCAACTAAGAACGGC-3′) (Suzuki et al., Reference Suzuki, Hoshino, Murakami, Takeyama and Chow2008). The PCR reactions (25 μl) comprised 3 μl DNA, 2.5 μl of 10× Taq buffer (Biotools, Madrid, Spain), 1 U of Taq polymerase (1 U,Biotools), 3 μl of deoxyribonucleoside triphosphates, 1 μl of each forward and reverse primer and 13.5 μl of deionized water. The amplification reaction was performed with the following cycling parameters: denaturation at 95°C for 3 min for 40 cycles of 94°C for 40 s; 55°C for 45 s; 72°C for 1-min; and termination at 72°C for 10 min. The PCR products were checked on 1% agarose gel and purified with the PureLink™ Quick Gel Extraction and PCR Purification Combo Kit (Invitrogen). Sequencing reactions were done using ABI Big Dye (Applied Biosystems, USA) Terminator vr. 3.1 cycle sequencing kit and detected using an ABI 3130 Genetic Analyzer.
Contigs generated in the present study were assembled using MEGA11 (Tamura et al., Reference Tamura, Stecher and Kumar2021). Obtained sequences for the 18S gene of M. wardae were aligned with other sequences downloaded from the GenBank database using the Clustal W software (Thompson et al., Reference Thompson, Gibson, Plewniak, Jeanmougin and Higgins1997). 18S sequences were aligned and a nucleotide substitution model was selected using jModelTest version 2.1.7 (Posada, Reference Posada2008) and the Akaike information criterion was applied. For the 18S dataset, the best nucleotide substitution model was GTR + G + I. The phylogenetic tree was reconstructed using the maximum likelihood (ML) method with the software MEGA11 (Tamura et al., Reference Tamura, Stecher and Kumar2021) and to assess nodal support 10,000 bootstrap replicates were run. We also estimated phylogenetic relationships using Bayesian inference (BI) in TOPALi 2.5 (Milne et al., Reference Milne, Lindner, Bayer, Husmeier, Mcguire, Marshall and Wright2009), with four independent runs of the Markov chain Monte Carlo chain and posterior probabilities were estimated over 1,000,000 generations with every 100th tree saved and ‘Burn-in’ was set to 25%. Genetic distances (uncorrected P-distance) were estimated with MEGA11.
Results
Morphological observations of our male and female specimens from Anaheim Beach and San Diego Bay, California, were found to be comparable to those described by Martin & Multani (Reference Martin and Multani1966) from Scammon's Lagoon over 700 km to the south, with a few variations. The original description included 12 largely accurate and well-presented line drawings. Our specimens, about half the size of those described by Martin & Multani (Reference Martin and Multani1966), were also fully developed sexually mature adults found in the rectal area of the same host species, G. mirabilis. Table 1 provides a morphometric comparison of the two populations. We opted not to create another set of line drawings that would be repetitious of the detailed ones in the original description. Instead, we have produced a new set of SEM images that depict many features not possible to observe with light microscopy, for the first time, and that elucidate features not described in the Martin & Multani's (Reference Martin and Multani1966) text or depicted in the original line drawings. Our treatment will also describe, for the first time, the micropores and the element composition of hooks using EDXA and a molecular profile of the examined specimens.
Table 1. Morphometric comparisons between two populations of Microsentis wardae from Gillichthys mirabilis in California.

a range (mean) in micrometres except when otherwise noted.
b figures shown in boldface type are of structures not measured in the original description.
Description
Microsentis wardae Martin & Multani, Reference Martin and Multani1966
General. Neoechinorhynchidae, with characters of the genus Microsentis. Small specimens, with no sexual dimorphism in size and with dorsal and ventral main longitudinal lacunar canals. See table 1 for measurements and counts. Trunk conical, aspinose, widest anteriorly. Neck prominent, widest posteriorly, often bent ventrally. Proboscis large, globular to pear-shaped, about 1/3 size of trunk (figs 1 and 2), with prominent apical organ (fig. 3), sensory pores (fig. 4), nucleated cells, and 16–20 longitudinal rows of 5–6 very small hooks, each. Hooks smallest posteriorly (figs 5–7). All hooks with rectangular roots slightly manubriated anteriorly. Body wall with few scattered electron-dense micropores (fig. 8) and 0–4 dorsal and 0–1 ventral giant hypodermal nuclei. Single-walled receptacle large, about half size of trunk, with cephalic ganglion near its base. Paired lemnisci shorter than receptacle.

Fig. 1. Scanning electron microscopy images of adult specimens of Microsentis wardae from the posterior gut of Gillichthys mirabilis collected in the salty marches of Anaheim Bay, California. A whole female specimen showing the relative size and shape of the spheroidal proboscis, neck and the conical trunk.

Fig. 2. Scanning electron microscopy images of adult specimens of Microsentis wardae from the posterior gut of Gillichthys mirabilis collected in the salty marches of Anaheim Bay, California. A pear-shaped proboscis and ventrally angulating neck.

Fig. 3. Scanning electron microscopy images of adult specimens of Microsentis wardae from the posterior gut of Gillichthys mirabilis collected in the salty marches of Anaheim Bay, California. A view of the anterior proboscis showing the apical disc of the apical organ.

Fig. 4. Scanning electron microscopy images of adult specimens of Microsentis wardae from the posterior gut of Gillichthys mirabilis collected in the salty marches of Anaheim Bay, California. A sensory pore at the anterior proboscis.

Fig. 5. Scanning electron microscopy images of adult specimens of Microsentis wardae from the posterior gut of Gillichthys mirabilis collected in the salty marches of Anaheim Bay, California. An apical hook. Note the epicuticular orifice at its point of insertion.

Fig. 6. Scanning electron microscopy images of adult specimens of Microsentis wardae from the posterior gut of Gillichthys mirabilis collected in the salty marches of Anaheim Bay, California. Image of a middle hook.

Fig. 7. Scanning electron microscopy images of adult specimens of Microsentis wardae from the posterior gut of Gillichthys mirabilis collected in the salty marches of Anaheim Bay, California. Image of a posterior hook.

Fig. 8. Scanning electron microscopy images of adult specimens of Microsentis wardae from the posterior gut of Gillichthys mirabilis collected in the salty marches of Anaheim Bay, California. A few scattered micropores in the body wall of a female specimen (arrows).
Males. Based on 12 sexually mature adults with sperm. See table 1 for measurements and counts. Testes contiguous, always overlapping, at mid-trunk, with anterior testis invariably larger than posterior testis. Sperm duct with prominent elongate sperm vesicles terminating at base of penis. Cement gland large, syncytial, with 5–8 giant nuclei each, connecting posteriorly with almost perfectly spherical cement reservoir opening into penis with 2 ducts. Penis prominent, conical. Saefftigen's pouch elongate, not bulbous anteriorly, invariably at the posterior-most end of trunk on dorsal side. Bursa muscular, without papillae externally (figs 9 and 10).
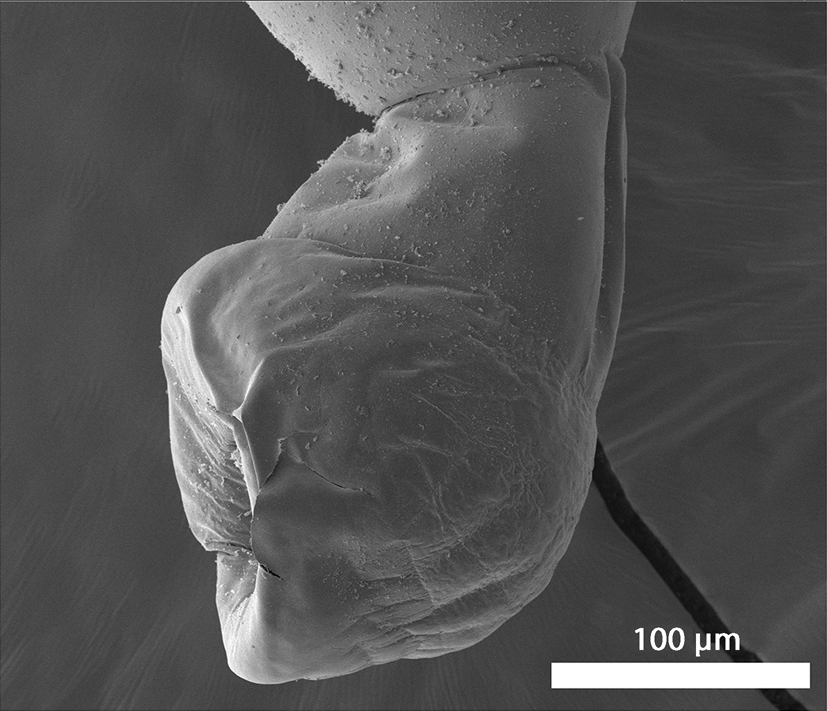
Fig. 9. Scanning electron microscopy images of adult specimens of Microsentis wardae from the posterior gut of Gillichthys mirabilis collected in the salty marches of Anaheim Bay, California. Lateral view of the bursa.

Fig. 10. Scanning electron microscopy images of adult specimens of Microsentis wardae from the posterior gut of Gillichthys mirabilis collected in the salty marches of Anaheim Bay, California. Ventral view of the bursa.
Females. Based on eight gravid females. Reproductive system somewhat short with relatively small elongate vagina, somewhat large muscular uterus, wide funnel-shaped uterine bell terminally attached to body wall at two different levels and very few basal uterine bell glands. Eggs small elongated ovoid (fig. 11) with slight prolongation of fertilization membrane.

Fig. 11. Scanning electron microscopy images of adult specimens of Microsentis wardae from the posterior gut of Gillichthys mirabilis collected in the salty marches of Anaheim Bay, California. A mature egg. Note the absence of surface corrugations or fibrillar topography.
Taxonomic summary
Host. Longjaw mudsuckers, G. mirabilis Cooper (Gobiidae).
Localities. The salt marshes of Anaheim Bay, California (33.735°N 118.094°W) and at Emory Cove Marsh, San Diego Bay (32.6717° N, 117.1441° W).
Site of infection. Posterior gut.
Specimens. HWML Helminthological Coll. No. 216779 (voucher specimens on four slides).
Representative DNA sequence. The 18S rDNA sequence of M. wardae was deposited in GenBank under the accession numbers OM831948–OM831951.
Remarks
Despite the apparent similarities between our specimens and those described by Martin & Multani (Reference Martin and Multani1966) from the same host species in the same intestinal site in California, new and different features became apparent as especially revealed by SEM (figs 1–14) used for the first time. Both populations have included sexually mature adults with sperm and eggs. Table 1 demonstrates that in our specimens, the trunk of both males and females was about half as large as that measured by Martin & Multani (Reference Martin and Multani1966) and that the ratio of the size of the proboscis to the size of trunk was considerably larger in our specimens (31–33%) compared to 21–25% in the Martin & Multani (Reference Martin and Multani1966) specimens. The same relationship was also observed for the receptacle to trunk ratio (49–44% compared to 30–31%). The extended lemnisci in our specimens were shorter than the receptacle. We added new measurements of the apical organ, the seminal vesicle, the bursa and the diameter of proboscis hooks at base. Our SEM images revealed new features not reported by Martin & Multani (Reference Martin and Multani1966) including the ribbed neck, different shaped proboscis, the surface appearance of the apical organ, sensory pores on the proboscis, the elevated proboscis rims at the base of hooks, the widely spaced micropores and the shape of the bursa and the eggs. The line drawings in the original description were detailed, informative and equally applicable to our specimens, the reason for not being repetitious since our SEM images revealed more features not presented by Martin & Multani (Reference Martin and Multani1966).

Fig. 12. X-ray panels of elemental scans of Microsentis wardae hooks. See table 2 for percentage weight of depicted elements. In all figures 12–14, the tallest peak to the far left is carbon and the 2 adjacent unlabelled peaks to the far right are palladium and gold. Scan of whole middle hook. Note the high level of sodium and sulphur compared to the lower levels of phosphorous, magnesium and calcium. Inset: whole middle hook.

Fig. 13. X-ray panels of elemental scans of Microsentis wardae hooks. See table 2 for percentage weight of depicted elements. In all figures 12–14, the tallest peak to the far left is carbon and the 2 adjacent unlabelled peaks to the far right are palladium and gold. Scan of a longitudinal section of middle hook. Note the highest levels of sulphur and phosphorus in multiple specimens and the moderate level of calcium. Insert: longitudinal section of a middle hook.

Fig. 14. X-ray panels of elemental scans of Microsentis wardae hooks. See table 2 for percentage weight of depicted elements. In all figures 12–14, the tallest peak to the far left is carbon and the 2 adjacent unlabelled peaks to the far right are palladium and gold. Scan of a cross-section of middle hook. Note the highest level of sulphur and phosphorous and the low level of all other elements. Insert: cross-section of a middle hook.
Micropores. The trunk had apparent osmiophilic micropores in most areas – the micropores were more widely spaced compared to the usual less widely distributed micropores more often observed in other acanthocephalan species.
EDXA
The EDXA results of whole hooks and hook sections (table 2) of M. wardae show highest level of sulphur and sodium. The EDXA spectra of the mid-hook longitudinal section again show the high-sulphur relative concentration characteristic of the centre core of the mid-hook cross-section as well as the marked concentration of phosphorus. The presence of sulphur, calcium and phosphorus in the EDXA spectra obtained from the hook longitudinal section close to edge of the hook base is attributed to the proximity of the exterior shell to the centre core. EDXA of the whole hook showed unusually highest level of sodium (table 2). It is worth noting that the reported WT% numbers should not be interpreted as compositional. They are, however, indicative of relative differences observed between the selected areas.
Table 2. The chemical composition of middle hooks of Microsentis wardae from Gillichthys mirabilis in California.

Molecular results
The 18S data set of M. wardae included sequences from four isolates with 880–887 base pairs that show no intraspecific sequence divergence. Table 3 provides data for the sequences used from GenBank for the reconstruction of phylogenetic analyses. For the 18S gene, both ML and BI algorithms produced trees with identical topology (fig. 15). The 18S phylogenetic tree inferred from ML and BI analyses shows that the sequences of isolates of M. warade obtained is closely associated with a clade formed by species of the family Neoechinorhynchidae, that is, Neoechinorhynchus spp., Hebesoma violentum and Floridosentis mugilis (fig. 15). The sister relationships with the above species of acanthocephalans with M. wardae received strong bootstrap support (99%) and Bayesian posterior probabilities (1.0). Four sequences of M. wardae were strongly supported (100% ML and 1.00 BI) and remained clusters into a distinct clade (fig. 15). In both analyses, nevertheless, species of Neoechinorhynchidae are nested together which removes any inconsistencies and confirmed the status of this monotypic genus Microsentis with species M. wardae under Neoechinorhynchidae (fig. 15), which is also in accordance with the previous studies (Amin, Reference Amin2013). Genetic divergence among the newly generated M. wardae sequences with Neoechinorhynchus spp. ranged 0.058–0.082% (36–50 nt difference), 0.122% (79 nt difference) with H. violentum, and 0.075 (45 nt difference) with F. mugilis, respectively.

Fig. 15. Phylogenetic reconstruction using 18S rDNA sequences of Microsentis wardae (shown in boldface type) and sequences of Acanthocephala deposited in the GenBank. The numbers indicate values of bootstrap >75%. Numbers above branches indicate nodal support as maximum likelihood and posterior probabilities from Bayesian inference. Species of Arachiacanthocephala and Palaeacanthocephala are used as an outgroup. The scale-bar indicates the expected number of substitutions per site.
Table 3. Acanthocephalan species information used for phylogenetic analysis based on the 18S gene sequences. NA = not available.

*, shows the unpublished status of a species on GenBank database.
Discussion
Morphology
The original assignment of the genus Microsentis was made to the neoechinorhynchid subfamily Tenuisentinae, now Family Tenuisentidae Van Cleave, 1936, but was later reassigned to Neoechinorhynchinae (Ward, 1917) Travassos, 1926. The morphometric findings of M. wardae from the same host species and locality quantified in table 1 show an extreme case of intraspecific morphological variations especially in trunk size and proportion of the size of proboscis and the receptacle to trunk size. We observed one worm with a calcareous collar similar to that shown by Martin & Multani (Reference Martin and Multani1966, fig. 3). Our specimens were sexually mature and females as small as 1.57 mm had fully ripe eggs. Martin & Multani (Reference Martin and Multani1966; p. 537) reported females reaching 5.00 mm long but a female as small as 0.98 mm long contained ovarian balls and another 1.3 mm long female contained eggs. Martin & Multani's (Reference Martin and Multani1966) ‘measurements were taken mainly of living material (p. 536) and hence are greater than would be obtained solely of fixed material.’ They also reported that ‘both sexes continue to grow after reaching sexual maturity.’ Size differences are related to different rates of growth and development. And since, in acanthocephalans, growth does not occur at the same rate in different body parts, one ends up with different ratios. In M. wardae, the trunk grows at a slower rate than the proboscis and the receptacle later in development. Our worms were younger than those described by Martin & Multani (Reference Martin and Multani1966) and somatic development priorities appear to be devoted to attachment structures first, to ensure efficient attachment to host gut after recruitment. A similar initial surge of proboscis and receptacle growth was observed in juveniles of other acanthocephalan species including Neoechinorhynchus cylindratus (Van Cleave, 1913) Van Cleave (1919) from Micropterus salmoides (Lacépède) in Wisconsin (Amin, Reference Amin1986). Differential growth rates have been observed in other species of acanthocephalans especially dorsally vs. ventrally causing the translocation of female gonopore from terminal in immatures to sub-ventral in more mature adults. Examples: see Amin & Heckmann (Reference Amin and Heckmann1992) on Neoechinorhynchus idahoensis Amin & Heckmann, Reference Amin and Heckmann1992; and Amin & Bullock (Reference Amin and Bullock1998) on N. cylindratus.
We noted usually six giant nuclei in the cement glands but eight were occasionally observed. Martin & Multani (Reference Martin and Multani1966) reported eight giant nuclei and papillae on each side of the penis that were barely visible in our microscopical observations. Our SEM images of the bursa (figs 9 and 10) show only external features.
Micropores
The micropores of M. wardae are associated with internal crypts and vary in diameter and distribution in different trunk regions corresponding with differential absorption of nutrients. We have reported micropores in a large number of acanthocephalan species (Heckmann et al., Reference Heckmann, Amin and El Naggar2013) and in a few more since, and demonstrated the tunnelling from the cuticular surface into the internal crypts by transmission electron microscopy in Corynosoma strumosum (Rudolphi, 1802) Lühe, 1904 from the Caspian seal Pusa caspica (Gmelin) in the Caspian Sea (figs 19 and 20 of Amin et al., Reference Amin, Heckmann, Halajian and El-Naggar2011) and in Neoechinorhynchus personatus Tkach, Sarabeev, Shvetsova, 2014 from Mugil cephalus Linn. in Tunisia (figs 26, 29 and 30 in Amin et al., Reference Amin, Sharifdini, Heckmann, Rubtsova and Chine2020); Amin et al. (Reference Amin, Heckmann, Radwan, Mantuano and Alcivar2009) gave a summary of the structural–functional relationship of the micropores in various acanthocephalan species. Wright & Lumsden (Reference Wright and Lumsden1969) and Byram & Fisher (Reference Byram and Fisher1973) reported that the peripheral canals of the micropores are continuous with canalicular crypts constituting a huge increase in external surface area … implicated in nutrient up take. Whitfield (Reference Whitfield1979) estimated a 44-fold increase at a surface density of 15 invaginations per 1 μm² of Moniliformis moniliformis tegumental surface.
EDXA
Our studies of acanthocephalan worms have usually involved X-ray scans (EDXA) of FIB-sectioned hooks and spines (Heckmann et al., Reference Heckmann, Amin and Standing2007, Reference Heckmann, Amin, Radwan, Standing, Eggett and El Naggar2012b; Standing & Heckmann, Reference Standing and Heckmann2014). Hooks and spines are evaluated for chemical ions with sulphur, calcium and phosphorus being the prominent elements. Sulphur is usually seen at the outer edge of large hooks and calcium and phosphorus are major ions in the base and middle of hooks where tension and strength are paramount for hook function. Sodium, a rarely prominent metal, was most prominent in scans of whole hooks of M. wardae (table 2, fig. 12). It was also prominent in whole hooks of Pallisentis nandai Sarkar, 1953 (see Amin et al., Reference Amin, Heckmann, Chaudhary, Rubtsova and Singh2021) as well as in egg shells of Neoechinorhynchus qatarensis Amin, Saoud, Alkuwari, 2002 (see Heckmann et al., Reference Heckmann, Amin and Standing2007). ‘The absence of sodium at the hook tip and its presence at the hook base and the opposite pattern of sulfur are characteristic of Neoechinorhynchus dimorphospinus Amin and Sey, 1996 hooks’ (Amin et al., Reference Amin, Sharifdini, Heckmann and Ha2019a, p. 616).
Results of the X-ray analysis of the FIB-sectioned hooks (dual beam SEM) of M. wardae show differential composition and distribution of metals in different hook parts characteristic of that species. Whole hooks and sections showed the highest level of sulphur and lowest levels of calcium and phosphorus (table 2). Cavisoma magnum (Southwell, 1927) Van Cleave, 1931 from Mugil cephalus in the Arabian Sea, has a similar pattern but considerably higher levels of sulphur in hook tips (43.51 WT%) and edges (27.46 WT%) (Amin et al., Reference Amin, Heckmann and Bannai2018). This element (sulphur) is part of the prominent outer layer of most acanthocephalan hooks and is a major contributor of the hardening process of hooks. Our results are comparable to those of mammalian teeth enamel. The EDXA appears to be species-specific, as in fingerprints. For example, Moniliformis cryptosaudi Amin, Heckmann, Sharifdini, Albayati, 2019 from Iraq is morphologically identical to Moniliformis saudi Amin, Heckmann, Mohammed, Evans, 2016 from Saudi Arabia, and it was erected based primarily on its distinctly different EDXA pattern (Amin et al., Reference Amin, Heckmann, Sharifdini and Albayati2019b) as a cryptic species. Our methodology for the detection of the chemical profile of hooks in the Acanthocephala has also been used in other parasitic groups including the Monogenea (Rubtsova et al., Reference Rubtsova, Heckmann, Smit, Luus-Powell, Halajian and Roux2018; Rubtsova & Heckmann, Reference Rubtsova and Heckmann2019) and Cestoda (Rubtsova & Heckmann, Reference Rubtsova and Heckmann2020).
The biological significance of EDXA as a diagnostic tool is exemplified by the observation that populations of an acanthocephalan species will consistently have similar EDXA spectra irrespective of host species or geography, even though comparative morphometrics of different populations of the same species usually vary with host species and geography; see, for example, Amin & Redlin (Reference Amin and Redlin1980) and Amin & Dailey (Reference Amin and Dailey1998). The taxonomic identity of species is deep-seated at the genetic level manifesting the organism's morphology and biochemistry as revealed, in part, by its elemental spectra. In discussing the EDXA of N. personatus Tkach, Sarabeev, Shvetsova, 2014 from M. cephalus Linn., Amin et al. (Reference Amin, Sharifdini, Heckmann, Rubtsova and Chine2020) noted that ‘The anterior and posterior hooks of our N. personatus in the Mediterranean and Black Sea had comparable biochemical profiles’. Metal analysis of hooks has become a diagnostic standard since hooks have the highest level of elements compared to the mid- and posterior trunk regions of the acanthocephalan body (Heckmann et al. Reference Heckmann, Amin, Radwan, Standing and Eggett2012a). Specifically, the sulphur content in the proboscis is paramount in the composition of disulphide bonds in the thiol groups for cysteine and cystine of the polymerized protein molecules (Stegman, Reference Stegman2005). Protein synthesis occurs in two stages, transcription and translation, by transferring of genetic instructions in the nuclear DNA to mRNA in the ribosomes followed by post-translational events such as protein folding and proteolysis (Stegman, 2005). The formed disulphide bonds are direct by-products of the DNA-based process of protein synthesis which makes up the identity of a biological species. Accordingly, the level of sulphur, in our EDXA profiles, will indicate the number of sulphur bonds that along with the levels of calcium phosphates, will characterize the identity of a species based on its nuclear DNA personality. Differences in chemical compositions probably indicate differences in allele expression. The DNA generated sulphide bonds evident in our EDXA profiles have an important role in the stability and rigid nature of the protein accounting for the high sulphur content of the proboscis (Heckmann et al., 2012a). The above processes explain the observed species-specific nature of EDXA profiles noted in our many findings.
Molecular analysis
To date, no genetic data have been provided for this monotypic genus Microsentis Martin & Multani, Reference Martin and Multani1966 with species M. wardae. Lack of molecular data creates difficulties for determining relationships among members of other genera and their taxonomic position. We add new molecular data for M. wardae inferred from the 18S ribosomal gene for the first time. The 18S analysis reliably showed that the species M. wardae forms a strongly supported clade with members of the family Neochinorhynchidae. This relationship was morphologically established previously and M. wardae was assigned to the Neochinorhynchidae (Amin, Reference Amin2013), which is also supported by the present study. Here in the tree, the presence of a single clade for four isolates of M. wardae from the United States was confirmed but the distribution pattern based on the genetic data will be confirmed after including a number of isolates of M. wardae from different regions and hosts to better understand the evolutionary relationships among the order Neoechinorhynchida. Regarding the phyletic assemblage of the genus Neoechinorhynchus species present in the phylogenetic tree (fig. 15), we found that the sequences were not construed as a monophyletic assemblage in the18S analysis. The genus Neoechinorhynchus requires a taxonomic revision.
To summarize, we suggest that the morphological description of acanthocephalan diversity necessitates the use of molecular data. Sequences from species of other genera of the Neoechinorhynchidae emphasize the importance of revealing relationships within the Eoacanthocephala in future studies.
Acknowledgements
The energy dispersive X-ray analysis and scanning electron microscopy methodologies used in this project have been pioneered by Dr Richard A. Heckmann (deceased) (Brigham Young University (BYU)) to whom we shall always dedicate our highest gratitude. We thank Madison Laurence, Bean Museum (BYU) for expert help in the preparation and organization of plates and figures and Michael Standing, Electron Optics Laboratory (BYU), for his technical help and expertise. We acknowledge the laboratory facilities provided by the Department of Zoology, Chaudhary Charan Singh University, Meerut, India. We are also very grateful to Dr. Ralph Appy, Cabrillo Marine Aquarium, San Pedro, California for providing the specimens of M. wardae used in this study.
Financial support
This project was supported by the Department of Biology, Brigham Young University, Provo, Utah, and by an Institutional Grant from the Parasitology Center, Inc, Scottsdale, Arizona.
Conflict of interest
None.
Ethical approval
The authors declare that they have observed all applicable ethical standards.