Introduction
The herbicide 2,4-D has been formulated in many ways since its development in the 1940s (Peterson Reference Peterson1967). In the pure acid form, 2,4-D has low water solubility and does not readily go into solution (Peterson et al. Reference Peterson, McMaster, Riechers, Skelton and Stahlman2016). As such, 2,4-D acid is commonly reacted with bases to form salts or with alcohols to form esters (Peterson et al. Reference Peterson, McMaster, Riechers, Skelton and Stahlman2016). Several of the salts that were historically used shortly after 2,4-D was developed included sodium, potassium, ammonium, lithium, and several amine salts (Anonymous 2007; Ross and Lembi Reference Ross and Lembi2009). Currently, common 2,4-D formulations include water-soluble amine salts, a water-soluble choline salt, and emulsifiable concentrate esters (Jervais et al. Reference Jervais, Luukinen, Nuhl and Stone2008; Peterson et al. Reference Peterson, McMaster, Riechers, Skelton and Stahlman2016).
The most common 2,4-D amine formulation manufactured today is the dimethylamine salt (Jervais et al. Reference Jervais, Luukinen, Nuhl and Stone2008; Peterson et al. Reference Peterson, McMaster, Riechers, Skelton and Stahlman2016). Choline salts are a new, water-soluble, 2,4-D formulation that reduce volatilization and eye irritation (Eytcheson et al. Reference Eytcheson, Reynolds, Irby, Steckel, Walton, Haygood, Ellis and Richburg2012; Stagg et al. Reference Stagg, Blewett, Tank, Li and Liu2013). Water-soluble formulations prepared in water with pH equal to the pK a will result in half of the 2,4-D amine molecules being dissociated (i.e., 2,4-D acid no longer bonded to the amine salt; Tan and Crabtree Reference Tan and Crabtree1994). The pK a of 2,4-D is 2.64, which is its dissociation constant (Bekbölet et al. Reference Bekbölet, Yenigün and Yücel1999). Because spray water pH is higher than the pK a of 2,4-D, typically between 7 to 8 (Hem Reference Hem1985), a water-soluble formulation is dissociated (deprotonated) when in spray solution (Tan and Crabtree Reference Tan and Crabtree1994). When 2,4-D is dissociated, it interacts with divalent cations in the spray solution such as Ca, Fe, Mg, Mn, and Zn (Devkota and Johnson Reference Devkota and Johnson2016; Nalewaja et al. Reference Nalewaja, Wozinca and Manthey1990, Reference Nalewaja, Wozinca and Matysiak1991; Patton et al. Reference Patton, Weisenberger and Johnson2016; Roskamp et al. Reference Roskamp, Chahal and Johnson2013; Schortgen and Patton Reference Schortgen and Patton2020).
Recent papers describe the hard water antagonism that can occur from 2,4-D dimethylamine (Patton et al. Reference Patton, Weisenberger and Johnson2016; Roskamp et al. Reference Roskamp, Chahal and Johnson2013; Schortgen and Patton Reference Schortgen and Patton2020), but those researchers did not evaluate other amine formulations. Past research by Nalewaja et al. (Reference Nalewaja, Wozinca and Manthey1990) demonstrated that 2,4-D diethanolamine is also antagonized by hard water. Later, Nalewaja et al. (Reference Nalewaja, Wozinca and Matysiak1991) noted that 2,4-D antagonism of the diethanolamine formulation likely meant that other 2,4-D salt formulations and MCPA ([4-chloro-2-methylphenoxy]acetic acid) would also be antagonized. However, data on MCPA and other 2,4-D formulations was not published.
Commonly used low-volatile ester formulations with longer carbon chains include butoxyethyl (also called butoxyethanol) and 2-ethylhexyl (also called isooctyl; Thompson Reference Thompson1986). Esters, which are oil soluble, are more effective at entering the waxy plant cuticle, but have a high potential for drift due to a low vapor pressure (Grover et al. Reference Grover, Maybank and Yoshida1972; Jervais et al. Reference Jervais, Luukinen, Nuhl and Stone2008; Peterson et al. Reference Peterson, McMaster, Riechers, Skelton and Stahlman2016). Ester products of 2,4-D are formulated as emulsifiable concentrates and should not be influenced by hard water cations since emulsifiable concentrates do not go directly into solution, dissociate, or come into contact with the divalent cations present in hard water (Müller Reference Müller2000). However, little published data exists describing the efficacy of 2,4-D ester formulations applied in hard water (Müller Reference Müller2000; Nalewaja et al. Reference Nalewaja, Wozinca and Matysiak1991). Two reports described that “2,4-D ester” is not antagonized but no data on the ester formulation was presented and the ester formulation(s) tested were not named (Nalewaja et al. Reference Nalewaja, Wozinca and Manthey1990, Reference Nalewaja, Wozinca and Matysiak1991).
A report by Devkota and Johnson (Reference Devkota and Johnson2016) based on their research on 2,4-D choline efficacy was the first on hard water antagonism of the choline formulation. Their research was with a water-soluble 2,4-D choline salt formulation (GF-2654, U.S. Environmental Protection Agency [EPA] registration number [reg. no.] 62719-634; Montague Reference Montague2017) that was later improved for increased stability and performance in hard water based on Devkota and Johnson’s (Reference Devkota and Johnson2016) research. The GF-2654 formulation is different than Enlist™ One (2,4-D choline salt, GF-3335, EPA reg. no. 62719-695; Montague Reference Montague2017), which is formulated to form an emulsion upon dilution (Li et al. Reference Li, Tank, Kennedy, Zhang, Downer, Ouse and Liu2013; Shao and Tank Reference Shao and Tank2015). Additional research is needed on the influence of water hardness on commercially available formulations.
Several of the above-mentioned papers tested 2,4-D as a standalone ingredient but few experiments have tested potential hard water antagonism of commercially available premixes. Kelly (Reference Kelly1953) and Peterson et al. (Reference Peterson, McMaster, Riechers, Skelton and Stahlman2016) noted that proprietary ingredients such as sequestering agents are often added to improve the efficacy of 2,4-D formulations. Despite this, Devkota and Johnson (Reference Devkota and Johnson2016) reported that a commercially available premix formulation of 2,4-D choline + glyphosate is antagonized by hard water.
Many commercial formulations of 2,4-D (Table 1) are currently available, but past research has focused on hard water antagonism of the 2,4-D dimethylamine formulation. Furthermore, information is scant on potential antagonism of formulations with emulsifiers or formulations of synthetic auxin premixes. The objective of this research was to determine which formulations of 2,4-D or premixes of various formulations of synthetic auxin herbicides are subject to hard water antagonism.
Table 1. 2,4-D formulations or formulation combinations tested for efficacy in hard water in Experiment 1.
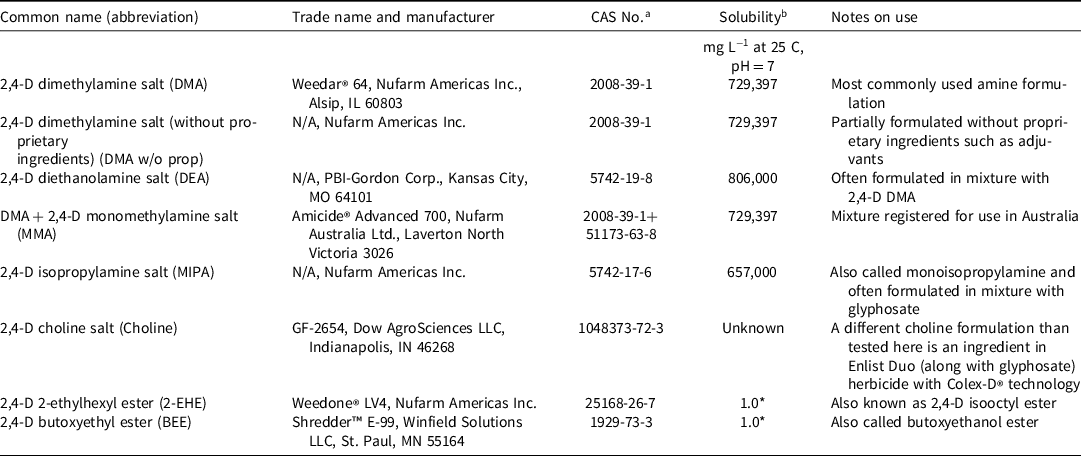
a Chemical Abstract Services number.
b Solubility data from Jervais et al. Reference Jervais, Luukinen, Nuhl and Stone2008. Those solubility values followed by asterisk (*) are formulated as emulsifiable concentrates.
Materials and Methods
Water Hardness and Adjuvant Inclusion Impact on 2,4-D Formulations
Two experiments were conducted at Purdue University to address the experimental objective. Experiment 1 was conducted in the Purdue University Horticulture greenhouses, West Lafayette, IN (40.421°N, 68.914°W) to determine the influence of water hardness on the efficacy of various 2,4-D formulations. Dandelion was used to evaluate the effects of hard water on 2,4-D formulation. Dandelion seed was collected locally in Lafayette, IN. Dandelions were germinated in 16 × 12 × 6 cm flats (Hummert International, Earth City, MO 63045) containing potting soil (Fafard growing mix, Sun-Gro Horticulture, Agawam, MA 01001) in the greenhouse. After germination and emergence, a single seedling was transplanted into 3.8 cm-diameter cone-tainers (Ray Leach SC-10 Super Cell Cone-tainers, Stuewe & Sons, Tangent, OR 97389) containing a 1:2:1 mixture of potting soil, sand, and a Whitaker silt loam soil (fine-loamy, mixed, active, mesic Aeric Endoaqualf). After transplanting, plants were irrigated with municipal tap water as needed and fertilized biweekly with two water-soluble fertilizers (3:1 mixture of 15 N–2.2 P–12.5 K and 21 N–2.2 P–16.6 K, respectively; The Scotts Co., Marysville, OH 43040) to provide the following (in mg L−1): 200 N, 26 P, 163 K, 50 Ca, 20 Mg, 1.0 Fe, 0.5 Mn and Zn, 0.24 Cu and B, and 0.1 Mo. Seventy-six percent of the nitrogen provided was in the nitrate form.
Two experimental runs were conducted in the greenhouse between April 2016 and June 2016, each arranged as a randomized complete block design on the greenhouse benches with four blocks in each run. Temperature and photosynthetically active radiation (PAR) from herbicide application to the conclusion of the experiment were collected with a mini-weather station (WatchDog 2475 Plant Growth Station, Spectrum Technologies, Plainfield, IL 60585). Temperatures for experimental run 1 averaged 24.4 C with an average daily light integral of 20 mol m−2 d−1. Temperatures for experimental run 2 averaged 25.3 C with an average daily light integral of 38 mol m−2 d−1.
The experiment was conducted to evaluate the effect of solution hardness on the performance of 2,4-D formulations. Formulations included a 2,4-D dimethylamine (DMA) with and without proprietary ingredients (DMA w/o prop). Other water-soluble formulations (amines) included 2,4-D diethanolamine (DEA), 2,4-D isoproplyamine (MIPA), and 2,4-D dimethylamine salt plus monomethylamine (MMA) salt, and a choline salt formulation (Table 1). Water-insoluble or emulsifiable concentrate formulations of 2,4-D included 2,4-D 2-ethylhexyl ester (2-EHE) and 2,4-D butoxyethyl ester (BEE; Table 1). The same acid equivalent of each formulation was added to mixtures to achieve an herbicide rate of 1.60 kg ae ha−1 (1.42 lb ae ha−1, the maximum single application rate to ornamental turf) in both soft and hard water. Soft water with a hardness levels of 0 mg L−1 was achieved by using distilled water and hard water was created with CaCl2 2[H2O] L−1 to achieve a hardness level of 600 mg CaCO3 L−1 following the methods described by Schortgen and Patton (Reference Schortgen and Patton2020). Calcium chloride was used to mimic a naturally occurring hard water from calcium carbonate based on a report by Nalewaja et al. (Reference Nalewaja, Wozinca and Matysiak1991) since 1) carbonates and chloride salts are similarly antagonistic, 2) calcium chloride is antagonistic to 2,4-D, and 3) artificially created hard water from calcium chloride is similarly antagonistic to naturally occurring hard water from wells. Water hardness is reported here in milligrams per liter of equivalent calcium carbonate per standard convention (Boyd Reference Boyd2015). “The total hardness of water results from divalent cations—mainly from calcium and magnesium—expressed as equivalent calcium carbonate. The total hardness equivalence of 1 mg/L calcium is 2.5 mg/L, while 1 mg/L magnesium equates to 4.12 mg/L” (Boyd Reference Boyd2015, p.179).
Treatments were applied to 5- to 12-cm-diameter dandelion rosettes. Applications were made on April 4, 2016 in experimental run 1 and May 11, 2016 in experimental run 2. Calcium solutions were prepared prior to adding the herbicide. After the herbicide was added, treatments were mixed thoroughly and applied immediately afterward. The pH of each treatment was determined using a meter (VWR B40PCID sympHony benchtop multi parameter meter, VWR International, Inc., Radnor, PA 19087) at each step of mixing to ascertain the influence of formulation on spray carrier pH. Treatments were applied using compressed air in a track spray chamber (Generation III Research Sprayer, DeVries Manufacturing, Hollandale, MN 56045) calibrated to deliver 815 L ha−1, a common spray volume used in the lawn care industry (Christians et al. Reference Christians, Patton and Law2017), using a TeeJet® 8004EVS nozzle (TeeJet Technologies, Spraying Systems Co., Wheaton, IL 60187) at 275 kPa. Plants were not irrigated for 24 h after application.
Weed epinasty was assessed 1 and 2 wk after application (WAA) on a 0% to 100% scale, where 0% was no epinasty and 100% represented complete epinasty with all leaves exhibiting symptoms including twisting or bending of stems and curling of leaves. Visual estimates of percent weed control were recorded at 3 and 4 WAA on a scale of 0 (no control) to 100 (complete plant death). Digital images were taken of the weeds 4 WAA using a camera and light box and analyzed for percent green coverage using ImageJ (v. 1.48v, National Institutes of Health, Bethesda, MD 20892; Schneider et al. Reference Schneider, Rasband and Eliceiri2012) as described by Patton et al. (Reference Patton, Weisenberger and Schortgen2018). At 4 WAA, plants were destructively harvested by washing the roots free of soil and dissecting into an aboveground shoot tissue and belowground root tissue fraction. Next, leaf fresh weight was measured and then tissues were placed into a forced-air dryer at 60 C for 3 d before dry weights were measured. All measurements (fresh and dry weight, green leaf area) collected during destructive harvest were compared to the nontreated control within block of each respective run to calculate the percent reduction. Data were converted to percent reduction in each block as {[1 − (treatment mass or coverage/mass or coverage in nontreated cone-tainer)] × 100} prior to analysis.
Analysis of variance was performed using the PROC GLIMMIX procedure in SAS 9.4 (SAS Institute Inc., Cary NC 27513). When F-tests were significant at P ≤ 0.05, Tukey’s HSD test (α = 0.05) was used for mean separation. Correlation coefficients between measurements were determined using the PROC CORR procedure. Additionally, heat maps of the data were created using Prism software (GraphPad Software, Inc., La Jolla, CA 92037) to assist with the visualization of hard water antagonism and comparison of treatments.
Synthetic Auxin Premix Efficacy Is Dependent on Formulation and Water Hardness
A second experiment was conducted in the Purdue University horticulture greenhouses to determine the influence of water hardness on the efficacy of different commercially available premixes of synthetic auxin herbicides with 2,4-D or MCPA (Table 2). Herbicides were selected to include three groups: water-soluble formulations (i.e., soluble liquids), water insoluble emulsifiable formulations (i.e., emulsifiable concentrates, EC), and emulsion formulations (i.e., emulsion-in-water, EW; microemulsion, ME; Mulqueen Reference Mulqueen2003; Tu et al. Reference Tu, Hurd and Randall2001). Two commercially available herbicides in each group with a different set of ingredients were selected (Table 2). The efficacy of each of the six herbicides was tested in two water hardness levels: distilled water or water with 600 mg CaCO3 L−1 equivalents for a total of 12 treatment combinations. Spray solutions were prepared as described previously and weed propagation, transplanting, and plant culture were also similar to the first experiment.
Table 2. Variable formulations of commercially available premixtures containing 2,4-D tested for their efficacy in soft and hard water in Experiment 2.

a Three formulation types tested included: water-soluble, emulsifiable, and emulsion.
b Application rate for each ingredient in the commercial premix. The application rate selected for each herbicide was the high label rate.
c The emulsifiable concentrate (EC) formulation was used in this experiment. The formulation of SpeedZone was changed in 2020 from EC to emulsion-in-water (EW).
The experiment was initiated in the greenhouse in autumn 2017 and repeated in winter 2018 with each arranged as a randomized complete block design with four blocks. Treatments were applied to 5- to 12-cm-diameter dandelion rosettes. Applications were made on October 31, 2017 in experimental run 1 and February 20, 2018 in experimental run 2 using the same equipment and application parameters described in Experiment 1. Temperatures for experimental run 1 averaged 23.5 C with an average daily light integral of 14 m−2 d−1. Temperatures for experimental run 2 averaged 23.8 C with an average daily light integral of 23 mol m−2 d−1.
Weed epinasty, control, digital images, fresh weights, and dry weights were collected in the same manner as the first experiment. A nontreated control was included in this experiment and prior to analysis all measurement data were converted to percent reduction in the same manner described in the first experiment. ANOVA was conducted in two different ways with the PROC GLM procedure in SAS 9.4. Both the ANOVA of formulation type by water hardness and ANOVA of commercially available herbicide premixes by water hardness were conducted. Main effects were formulation type (three levels: water-soluble formulations, emulsifiable formulations, and emulsions) and hardness (two levels) in the first ANOVA and commercially available herbicide premixes (six herbicides) by hardness (two levels) in the second ANOVA. For data sets where main effects or interactions were significant at P ≤ 0.05, Fisher’s protected LSD test (α = 0.05) was used for mean separation. No significant treatment by experimental run interactions were present, so data were analyzed across experimental runs. Additionally, heat maps of the data were created similar to Experiment 1 to assist with the visualization of hard water antagonism by formulation type.
Results and Discussion
Water Hardness and Adjuvant Inclusion Impact on 2,4-D Formulations
To ensure that mixture pH was not a confounding factor, measurements were taken at each step of preparation for all 2,4-D formulation mixtures at both 0 mg CaCO3 L−1 and 600 mg CaCO3 L−1 (data not shown). The pH readings for all mixtures were above the pK a (2.64) of the 2,4-D in its acid form and ranged from 3.52 (for 2-EHE) to 9.39 (for MMA). The lowest pH of a water-soluble formulation was 4.61, which is 1.97 pH units above the pK a (2.64) of 2,4-D. At a pH of 4.64, 99% of the 2,4-D molecules are predicted to become dissociated (Tan and Crabtree Reference Tan and Crabtree1994). Thus, we can infer that all water-soluble formulations (amines and choline) were dissociated and able to interact with Ca2+ in the hard water solutions (Tan and Crabtree Reference Tan and Crabtree1994). Herbicides formulated as emulsifiable concentrates do not have a pK a value because they do not dissociate in solution, or come into direct contact with cations that cause hard water antagonism. This applies to all water-insoluble ester formulations of 2,4-D (Peterson et al. Reference Peterson, McMaster, Riechers, Skelton and Stahlman2016).
No significant treatment by experimental run interactions were present, so data were analyzed across experimental runs. The main effect of 2,4-D herbicide formulation and the main effect of water hardness were both significant for all observed ratings and calculated percent reductions (P < 0.001, data not shown). ANOVA also revealed 2,4-D formulation-by-water hardness interactions for all ratings and measurements (P < 0.025, data not shown) and discussion of our results were based on these interactions.
Dandelions treated with 2,4-D BEE exhibited more epinasty than amine and choline formulations at 1 WAA but there were no differences in epinasty between 2,4-D applied in soft water to those applied in hard water (Table 3). The increased efficacy of ester formulations continued throughout the duration of the experiment (Tables 3 and 4), and was consistent with faster uptake of this formulation (Peterson et al. Reference Peterson, McMaster, Riechers, Skelton and Stahlman2016). Treatments with the lowest epinasty rating 2 WAA were amines and a choline formulation of 2,4-D mixed in hard water. There was a separation between soft and hard water treatments with a reduction in epinasty for several of the water-soluble formulations (DMA w/o prop, MMA, and choline) of 2,4-D mixed with hard water (Table 3). One observation from this study was that hard water antagonism is difficult to diagnose the first week after 2,4-D applications, as there is sufficient herbicide uptake to cause epinasty, but insufficient time to evaluate weed control.
Table 3. Percent epinasty 1 and 2 WAA and percent control 3 and 4 WAA following applications of each respective 2,4-D formulation at 1.6 kg ae ha−1 in Experiment 1 as influenced by 2,4-D formulation and water hardness. c.d

a Distilled water with 0 mg CaCO3 L−1 was used as the soft water source. Hard water was prepared by adding calcium chloride (CaCl2 2[H2O] L−1) at 887.1 mg L−1 to achieve a water hardness level of 600 mg CaCO3 L−1.
b Means followed by the same letter within columns type are similar according to Tukey’s HSD test (α = 0.05). Each mean value is representative of eight observations. Means followed by an asterisk (*) within a column and within a formulation type indicate statistical separation from the distilled (soft) water carrier and the occurrence of hard water antagonism.
c Abbreviation: WAA, weeks after application. See Table 1 for additional abbreviations.
d Data were combined across experimental runs.
Table 4. Percent dandelion tissue weight reduction 4 WAA and percent green leaf area reduction following applications of each 2,4-D formulation at 1.6 kg ae ha−1, as influenced by 2,4-D formulation and water hardness. d,e

a Distilled water with 0 mg CaCO3 L−1 was used as the soft water source. Hard water was prepared by adding calcium chloride (CaCl2 2[H2O] L−1) at 887.1 mg L−1 to achieve a water hardness level of 600 mg CaCO3 L−1.
b Means followed by the same letter within columns type are similar according to Tukey’s HSD test (α = 0.05). Each mean value is representative of eight measurements. Means followed by an asterisk (*) within a column and within a formulation type indicate statistical separation from the distilled (soft) water carrier and the occurrence of hard water antagonism.
c Means with a negative value indicate the plants were larger (higher mass or leaf area) than the nontreated control.
d Abbreviation: WAA, weeks after application. See Table 1 for additional abbreviations.
e Data were combined across experimental runs.
Formulation by water hardness interactions for control ratings 3 and 4 WAA revealed similar results to earlier 2 WAA epinasty ratings. Ester formulations continued to provide the highest dandelion control of all the 2,4-D formulations tested. Furthermore, the water-insoluble ester formulations were not influenced by water hardness at any rating (Table 3). The improved control from the ester formulations is largely attributed to increased leaf absorption of the ester compared to amine formulations (Richardson Reference Richardson1977). Differences in control 3 and 4 WAA between water hardness treatments revealed that hard water reduced the efficacy of nearly all water-soluble amine formulations when mixed with hard water. The only water-soluble formulation that did not have a significant reduction in control 3 and 4 WAA was 2,4-D DEA, which was attributed to the poor dandelion control observed in this experiment in both soft and hard water (Table 3).
The destructive harvesting and calculation of percent fresh shoot weight reduction, dry shoot weight reduction, dry root weight reduction, and green leaf area reduction revealed a similar story about the 2,4-D formulation by water hardness interaction. Measurements collected 4 WAA were highly correlated with one another (r = >0.96, P < 0.0001). The highest reduction in dandelion tissue weight or green leaf area occurred when dandelions were treated with either the 2-EHE or BEE water-insoluble ester formulations of 2,4-D (Table 4). Furthermore, the water insoluble ester formulations performed in similar ways when used in both soft and hard water mixes (Table 4; Figure 1). This observation supports statements on the indifference of using hard or soft water when mixing 2,4-D esters for applications (Müller Reference Müller2000; Nalewaja et al. Reference Nalewaja, Wozinca and Manthey1990, Reference Nalewaja, Wozinca and Matysiak1991). The 2,4-D ester formulation is protected against hard water antagonism since the 2,4-D remains dissolved in the oil, whereas the emulsifying agent disperses the droplets (Peterson et al. Reference Peterson, McMaster, Riechers, Skelton and Stahlman2016).
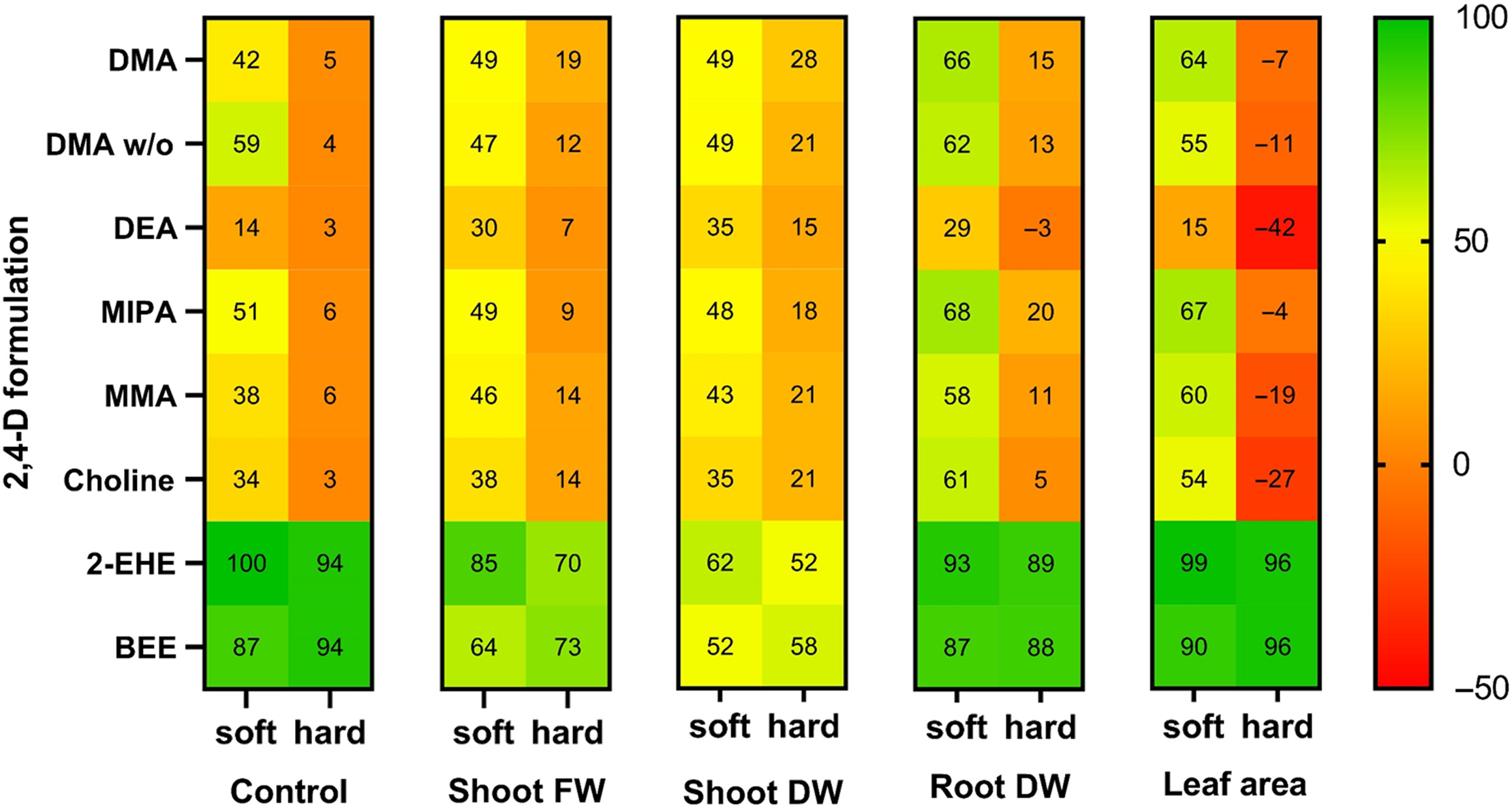
Figure 1. Visualization of hard water antagonism using a heat map with the relative performance of each 2,4-D formulation at 1.6 kg ae ha−1 4 wk after application, as influenced by 2,4-D formulation and water hardness. Mean values appear in Tables 3 and 4. A four-color scale with green indicating highest weed control, yellow indicating intermediate control, and orange or red indicating poor control. A shift in heat color within a formulation from soft (0 mg CaCO3 L−1) to hard water (600 mg CaCO3 L−1) is indicative of hard water antagonism. A change in color by formulation is indicative of efficacy differences. See statistical comparisons provided in Tables 3 and 4. Abbreviations: DW, dry weight; FW, fresh weight.
The only amine (water-soluble) treatments that achieved similar results to those of the ester formulations were those applied with soft water (Table 4; Figure 1). All water-soluble 2,4-D formulations had reduced efficacy when applied with hard water at 600 mg CaCO3 L−1 in this experiment (Figure 1). This is consistent with earlier reports on older standalone 2,4-D amine formulations such as 2,4-D diethanolamine, 2,4-D triethanolamine, and the previously used alkali salt formulations (Kelly Reference Kelly1953; Müller Reference Müller2000; Nalewaja et al. Reference Nalewaja, Wozinca and Manthey1990, Reference Nalewaja, Wozinca and Matysiak1991; Ross and Lembi Reference Ross and Lembi2009; Szabo and Buchholtz Reference Szabo and Buchholtz1961). This is the first report of hard water antagonism of 2,4-D MMA and MIPA, although antagonism of DEA and DMA was previously reported (Nalewaja et al. Reference Nalewaja, Wozinca and Manthey1990, Reference Nalewaja, Wozinca and Matysiak1991; Patton et al. Reference Patton, Weisenberger and Johnson2016; Roskamp et al. Reference Roskamp, Chahal and Johnson2013). Our results are consistent with unpublished results reported by Nalewaja et al. (Reference Nalewaja, Wozinca and Matysiak1991) who indicated that other 2,4-D salt formulations were subject to hard water antagonism similar to the 2,4-D DEA formulation. Kelly (Reference Kelly1953) noted that 2,4-D formulations were designed to perform well in 1,000 mg CaCO3 L−1 through the addition of sequestering agents in the formulation to protect against hard-water antagonism. However, this experiment demonstrated that current water-soluble 2,4-D formulations contain little protection against hard water (600 mg CaCO3 L−1) antagonism.
Based on a report by Devkota and Johnson (Reference Devkota and Johnson2016) and preliminary research by Schortgen (Reference Schortgen2017) that demonstrated hard water antagonism of the 2,4-D choline formulation, Dow AgroSciences improved the 2,4-D choline formulation to have greater stability and performance in hard water. The GF-2654 formulation of 2,4-D choline salt used in this experiment is different from that of Enlist™ One (2,4-D choline salt, GF-3335, EPA reg. no. 62719-695; Montague Reference Montague2017), which is formulated to form an emulsion upon dilution (Li et al. Reference Li, Tank, Kennedy, Zhang, Downer, Ouse and Liu2013; Shao and Tank Reference Shao and Tank2015). (Note: The GF-2654 formulation of 2,4-D choline salt is available as Freelexx® (EPA reg. no. 62719-634) and Embed® (EPA reg. no. 62719-634.)
Synthetic Auxin Premix Efficacy Is Dependent on Formulation and Water Hardness
Data were analyzed across experimental runs since there were no significant treatment by experimental run interactions. ANOVA for 2,4-D treated dandelions revealed a hardness-by-formulation interaction for control, shoot fresh weight, dry weight, green leaf coverage, and regrowth (Table 5). Epinasty 1 WAA was less than 16% for all treatments with less than 5% difference between formulations with no difference in epinasty between treatments 2 WAA (data not shown). Subtle differences occurred between premix formulation type applied in distilled water. Most notable was that treatments of water-soluble premix formulations in hard water (600 mg CaCO3 L−1) were less effective at controlling dandelions compared to other premix formulations, revealing reduced efficacy due to hard water antagonism (Table 6; Figure 2). Dandelions treated with water-soluble formulations of synthetic auxin herbicides in hard water (600 mg CaCO3 L−1) had higher fresh and dry shoot weights, green leaf area, and regrowth following a harvest 4WAA compared with water-soluble formulations applied in distilled water (Table 6; Figure 2).
Table 5. Formulation solubility type ANOVA for epinasty, visual control, shoot fresh weight reduction, shoot dry weight reduction, and percent green leaf area reduction, and regrowth dry shoot weight reduction with P-values for the main effects of formulation solubility type, water hardness, and the interaction between hardness and formulation solubility type in Experiment 2. a

a Abbreviation: WAA, weeks after application.
b Three formulation types tested included: water-soluble, emulsifiable, and emulsion.
Table 6. Influence of herbicide formulation type and water hardness level on dandelion control efficacy in experiment two. a,b,c

a Abbreviation: WAA, weeks after application.
b Data were combined across experimental runs.
c Means followed by the same letter within columns type are similar according to Tukey’s HSD test (α = 0.05). Each mean value is representative of eight measurements. Means followed by an asterisk (*) within a column and within a formulation type indicate statistical separation from the distilled (soft) water carrier and the occurrence of hard water antagonism.
d Three formulation types tested included: water-soluble, emulsifiable, and emulsion. Products described as emulsion-in-water and microemulsion are included in the emulsion group (Table 2).
e Distilled water with 0 mg CaCO3 L−1 was used as the soft water source. Hard water was prepared by adding calcium chloride (CaCl2 2[H2O] L−1) at 887.1 mg L−1 to achieve water hardness levels of 600 mg CaCO3 L−1.

Figure 2. Visualization of hard water antagonism using a heat map with the relative performance of each formulation type 4 wk after application (and 8 wk after application for regrowth), as influenced by formulation type and water hardness. Mean values appear in Table 6. A three-color scale with green indicating highest weed control, yellow indicating intermediate control, and red indicating poor control. A shift in heat color within a formulation from soft (0 mg CaCO3 L−1) to hard water (600 mg CaCO3 L−1) is indicative of hard water antagonism. A change in color by formulation is indicative of efficacy differences. See statistical comparisons provided in Table 6. Abbreviations: DW, dry weight; FW, fresh weight.
ANOVA with herbicide premix as the main effect (Table 7) also demonstrated hard water antagonism of water-soluble herbicides regardless of their synthetic auxin ingredients (Table 8). Herbicides formulated as esters (emulsifiable concentrates) and newer emulsion formulations (emulsion-in-water or microemulsion) were not antagonized by hard water when evaluated 4 WAA (Table 8). Although the formulations were not tested as single ingredients, results from these soluble premixes (2,4-D + MCPP + dicamba; MCPA + triclopyr + dicamba) are consistent with other reports that water-soluble formulations of MCPA are subject to hard water antagonism similar to that of 2,4-D (de Villiers et al Reference de Villiers, Smit, Lindeque and Smit2000; Nalewaja et al. Reference Nalewaja, Wozinca and Matysiak1991; Nalewaja and Matysiak Reference Nalewaja and Matysiak1993). We were unable to find any previously published information on the potential for hard water antagonism of MCPP or triclopyr. Previously, Roskamp et al. (Reference Roskamp, Chahal and Johnson2013) reported that dicamba diglycolamine salt was not subject to hard water antagonism when tested as a standalone ingredient at 280 g ae ha−1 and applied at 140 L ha−1. Our results show that premixes with dicamba dimethylamine salt (applied here at 108–133 g ae ha−1) are subject to hard water antagonism. Possible explanations are that 1) instead of dicamba being antagonized by hard water, the phenoxycarboxylic acids 2,4-D, MCPA, and MCPP in these premixes (Table 2) are being antagonized, thereby leading to a reduced efficacy; or 2) lower rates of dicamba dimethylamine tested here at higher application volumes (i.e., more calcium cations in solution) are subject to hard water antagonism. The potential for dicamba to be antagonized at lower rates by hard water is supported by Nalewaja and Matysiak (Reference Nalewaja and Matysiak1993) who reported dicamba dimethylamine salt antagonism when applied at 70 g ae ha−1 in a spray volume of calcium chloride solution at 160 L ha−1.
Table 7. Commercially available herbicide ANOVA for epinasty, visual control, shoot fresh weight reduction, shoot dry weight reduction, and percent green leaf area reduction, and regrowth dry shoot weight reduction with P-values for the main effects of commercially available herbicide premixes, water hardness, and the interaction between hardness and commercially available herbicide premixes in Experiment 2. a

a Abbreviation: WAA, weeks after application.
b Three commercially available herbicide premixes tested are listed in Table 2.
Table 8. Efficacy of herbicide premixes and water hardness level on dandelion control efficacy in Experiment 2. a,b
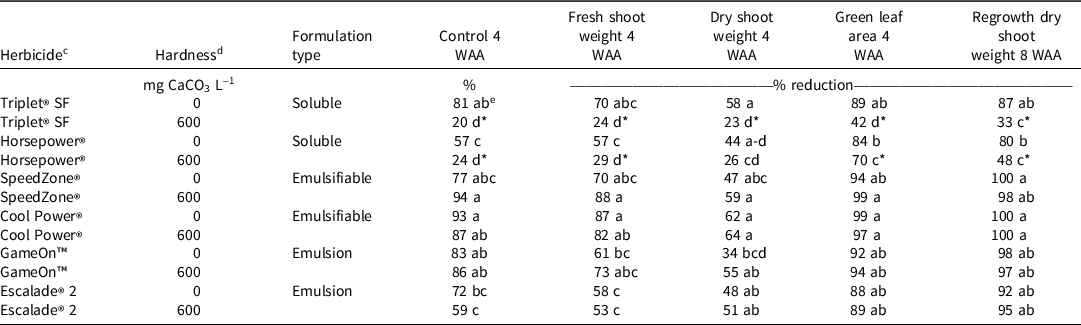
a Abbreviation: WAA, weeks after application.
b Data were combined across experimental runs.
c Herbicide ingredients and rates are listed in Table 2.
d Distilled water with 0 mg CaCO3 L−1 was used as the soft water source. Hard water was prepared by adding calcium chloride (CaCl2 2[H2O] L−1) at 887.1 mg L−1 to achieve water hardness levels of 600 mg CaCO3 L−1.
e Means followed by the same letter within columns type are similar according to Tukey’s HSD test (α = 0.05). Each mean value is representative of eight measurements. Means followed by an asterisk (*) within a column and within a formulation type indicate statistical separation from the distilled (soft) water carrier and the occurrence of hard water antagonism.
The 2,4-D-containing emulsion formulation types (emulsion-in-water or microemulsion) tested in this experiment were not antagonized by hard water (Tables 6 and 8; Figure 2). In Experiment 1 presented here and a previous report by Devkota and Johnson (Reference Devkota and Johnson2016), the water-soluble 2,4-D choline salt was antagonized by hard water. However, in Experiment 2, the premix of 2,4-D choline + fluroxypyr + halauxifen-methyl (GameOn™) was not antagonized due to a formulation difference. Li (Reference Li2015) detailed a new method of combining the water-soluble 2,4-D choline salt with water-insoluble formulations of other synthetic auxin herbicides, which provides a stable, transparent, aqueous solution formulation that forms an oil-in-water emulsion upon dilution. The GameOn™ formulation tested here combines the 2,4-D choline salt with fluroxypyr meptyl 1-methylheptyl ester and the methyl ester of halauxifen-methyl (Anonymous 2019). The Escalade® 2 formulation, which also was not subject to antagonism, is a microemulsion formulation (Anonymous, 2020), and combines the 2,4-D dimethylamine salt with fluroxypyr meptyl 1-methylheptyl ester and dicamba acid (Anonymous 2016). As such, formulating water-soluble 2,4-D using water-based dispersion technology such as oil-in-water emulsions appears to be an effective method to prevent hard water antagonism. Water-based dispersion technology has advantages over conventional emulsifiable concentrates in that less solvent is needed, lower skin and eye toxicity is achieved, formulations are more compatible with containers, and particle drift can be reduced (Li et al. Reference Li, Tank, Kennedy, Zhang, Downer, Ouse and Liu2013; Mulqueen Reference Mulqueen2003).
Previous research demonstrated that 2,4-D dimethylamine was subject to hard water antagonism (Patton et al. Reference Patton, Weisenberger and Johnson2016; Roskamp et al. Reference Roskamp, Chahal and Johnson2013; Schortgen and Patton Reference Schortgen and Patton2020). This research demonstrates that all 2,4-D water-soluble formulations and water-soluble premixes with phenoxycarboxylic acid herbicides are subject to hard water antagonism. Formulations of 2,4-D containing emulsifying agents can protect ingredients against antagonism by the water-insoluble nature of the ingredients in their formulation.
Applicators can prevent hard water antagonism of water-soluble formulations by using high quality water low in calcium, magnesium, and iron cations or by mixing with a water conditioning agent such as ammonium sulfate or urea ammonium nitrate (Schortgen Reference Schortgen2017; Schortgen and Patton Reference Schortgen and Patton2020). A common communication problem is that manufacturer labels of water-soluble formulations of 2,4-D do not include instructions on how to mix it with hard water or precautions against applying it using hard water (Schortgen and Patton Reference Schortgen and Patton2020). Schortgen and Patton (Reference Schortgen and Patton2020) suggest that manufacturers of 2,4-D dimethylamine should consider adding statements to the label about the use of ammonium sulfate similar to the statement on the glyphosate label. Furthermore, manufacturers may need to modify sequestering agents in the formulation to better protect against hard-water antagonism (Kelly Reference Kelly1953; Peterson et al. Reference Peterson, McMaster, Riechers, Skelton and Stahlman2016).
Another solution to preventing hard water antagonism is to use formulations with emulsifying agents. Applications of esters when environmental conditions permit or the use of advanced formulations, such as emulsion-in-water formulations, will prevent hard water antagonism. While these emulsion-in-water, microemulsion, or other advanced emulsion formulations are shown here to protect against hard water antagonism, practitioners often struggle to know the formulation type they are using because the information is often difficult to find. For example, neither the Escalade® 2 or GameOn™ labels (Anonymous 2016, 2019) indicate their formulation type or that they form an emulsion-in-water. Conversely, both the Cool Power® and SpeedZone® labels note that the material forms an emulsion-in-water (Anonymous 2003, 2009). None of these four labels contain a statement that inform the applicator that the products are formulated for effective weed control when mixed with hard water. Manufacturers should amend labels of all 2,4-D-containing herbicides to assist applicators in the prevention of hard water antagonism.
Acknowledgments
We thank the Midwest Regional Turf Foundation for funding this project and Dow AgroSciences LLC, Nufarm Americas Inc., PBI-Gordon Corp., and Winfield Solutions LLC for providing 2,4-D formulation samples or commercially available herbicides for testing. This work was supported by Hatch project 1004762 from the U.S. Department of Agriculture–National Institute of Food and Agriculture. No conflicts of interest have been declared. We also thank Drs. Steve Weller, Fred Whitford, and Bryan Young for their helpful review of this manuscript; and express our appreciation to Katherine Wierzbowski, Joe Ikely, Dan Weisenberger, and Julie Young for their assistance with this experiment.