Childhood maltreatment may affect between 10% and 40% of Western children (Gilbert et al., Reference Gilbert, Widom, Browne, Fergusson, Webb and Janson2009; Scher, Forde, McQuaid, & Stein, Reference Scher, Forde, McQuaid and Stein2004), while the incidence may be even higher in developing countries (Herbert et al., Reference Herbert, van As, Bachani, Mtambeka, Stevens, Millar and Hyder2012), and among certain vulnerable demographics (Benjet et al., Reference Benjet, Bromet, Karam, Kessler, McLaughlin, Ruscio and Koenen2016). Childhood maltreatment has been shown to increase the risk of developing mental disorders later in life, such as posttraumatic stress disorder (PTSD) and other anxiety as well as depressive disorders, suicidality, and substance use disorders (Neumann, Houskamp, Pollock, & Briere, Reference Neumann, Houskamp, Pollock and Briere1996; Ozer, Best, Lipsey, & Weiss, Reference Ozer, Best, Lipsey and Weiss2003). Given that psychiatric illness is a major contributor to disability and mortality on a global level (Murray & Lopez, Reference Murray and Lopez2013), and that childhood maltreatment is associated with a higher incidence of treatment resistance in psychiatric populations (Wegman & Stetler, Reference Wegman and Stetler2009), early maltreatment may foster a predisposition to serious long-term mental and physical ill-health. In this vein, animal models of early life stress demonstrate evidence of induced cellular and behavioral alterations throughout life, including changes in responses to acute stress, which may be modulated by behavioral interventions and administration of epigenetic-modifying drugs (Harrison & Baune, Reference Harrison and Baune2014; Sanchez, McCormack, & Howell, Reference Sanchez, McCormack and Howell2015). However, understanding the most efficacious interventions to adopt in humans will depend on better knowledge of which brain regions are persistently altered by childhood maltreatment.
There is growing evidence that childhood maltreatment is associated with structural and functional changes in the human brain, particularly in the anterior cingulate cortex (ACC), hippocampus, and amygdala (Hart & Rubia, Reference Hart and Rubia2012; Lupien, McEwen, Gunnar, & Heim, Reference Lupien, McEwen, Gunnar and Heim2009; Van Harmelen et al., Reference Van Harmelen, Hauber, Gunther Moor, Spinhoven, Boon, Crone and Elzinga2014), as well as the prefrontal cortex (PFC), a brain region that modulates emotional stimulation (Arnsten, Reference Arnsten2009). These effects might be long lasting, given that the brain is continuously molded during childhood, and specific brain areas might be particularly vulnerable to adversity during important developmental stages. Given that micro-alterations in neural structure may underlie differences in neural function (e.g., DeBello et al., Reference DeBello, McBride, Nichols, Pannoni, Sanculi and Totten2014), particularly in terms of hippocampal neurogenesis in those who have experienced childhood adversity (Hoeijmakers, Lucassen, & Korosi, Reference Hoeijmakers, Lucassen and Korosi2014), functional magnetic resonance imaging (fMRI) studies may also provide valuable information regarding neurobiological targets for psychiatric treatment.
From a psychological perspective, it has been proposed that experiences of maltreatment in childhood may enduringly facilitate threat-related processing, which may render individuals susceptible to develop mental disorders later in life (McCrory & Viding, Reference McCrory and Viding2015). Accordingly, hyperactivation of anxiety and stress reactivity circuitry (such as amygdala and ventral ACC; see Brooks & Stein, Reference Brooks and Stein2015) during socioaffective challenges may be key to understanding the mechanism of dysfunction in brain circuits following an early life experience of maltreatment (Barch, Reference Barch2014; Groenewold et al., Reference Groenewold, Roest, Renken, Opmeer, Veltman, van der Wee and Harmer2015). In this regard, experimental designs incorporating socioaffective cues (stimuli portraying social and/or emotional scenes that are designed to elicit negative and positive affective states, stimulate mentalizing, and potentially signal challenge, threat, or safety) are of particular interest. However, despite growing evidence from task-based fMRI studies showing effects of childhood maltreatment on brain activation patterns, there has not yet been an attempt to comprehensively map the consistency in results and increase statistical power of current findings in the field.
Against this background, we conducted a meta-analysis of fMRI studies examining the neural effects of early life adversity in adults. Given that we were concerned with the overall effects of childhood maltreatment and thus the full spectrum of resilience and vulnerability to stress, we incorporated results from both nonclinical and clinical populations. We examined only fMRI studies that utilized the standardized Childhood Trauma Questionnaire (CTQ; Bernstein & Fink, Reference Bernstein and Fink1998, Bernstein et al., Reference Bernstein, Stein, Newcomb, Walker, Pogge, Ahluvalia and Zule2003), which has been validated in clinical and nonclinical adult populations (Scher, Stein, Asmundson, McCreary, & Forde, Reference Scher, Stein, Asmundson, McCreary and Forde2001), to retain homogeneity across studies, although other measures of early life adversity are apparent in the fMRI literature. Moreover, considering indications in the literature that socioaffective cues may be perceived as more arousing and threatening by maltreatment-exposed individuals, we hypothesised that individuals with higher levels of childhood maltreatment would show increased amygdala and ACC activation during socioaffective stimulation across studies included in this meta-analysis.
Method
Ethics statement
This systematic review and meta-analysis meets all ethical guidelines as set out by the Declaration of Helsinki and the University of Cape Town's Faculty of Health Sciences Human Research Ethics Committee.
Childhood trauma measure: CTQ
The CTQ was used as a criterion for study selection as it is the most widely used scale of childhood maltreatment currently found in peer-reviewed journals. It is well validated across clinical and nonclinical populations and in different languages (Karos, Niederstrasser, Abidi, Bernstein, & Bader, Reference Karos, Niederstrasser, Abidi, Bernstein and Bader2014; Lochner et al., Reference Lochner, Seedat, Allgulander, Kidd, Stein and Gerdner2010). It has also been validated against therapist maltreatment ratings and objective measures such as data from child welfare agencies (Bernstein, Ahluvalia, Pogge, & Handelsman, Reference Bernstein, Ahluvalia, Pogge and Handelsman1997). The advantage of this approach is that the item contents are comparable across studies, thus limiting between-measure variance that might be a factor when including a range of other measures of childhood maltreatment. In addition, the CTQ is well suited to allow for secondary analyses based on severity of recollected maltreatment. The CTQ consists of five subscales assessing emotional abuse, physical abuse, sexual abuse, emotional neglect, and physical neglect. The CTQ scoring guidelines include cutoff total scores for classification of maltreatment as “none or minimal,” low to moderate,” “moderate to severe,” and “severe to extreme” in each subscale. The included studies that separated low and high maltreatment groups generally used the moderate to severe scoring thresholds to classify participants into a low or high maltreatment group, although others used the thresholds as recommended by Walker et al. (Reference Walker, Gelfand, Katon, Koss, Von Korff, Bernstein and Russo1999).
Search procedure
The online academic search engines: PubMed, Ovid MEDLINE, and Web of Science were searched. Additional hand searches were performed from reference lists of relevant articles. Records from all years, until August 2015, were evaluated. The following search parameters were used: (a) CTQ AND (fMRI OR activation OR brain OR MRI OR brain imaging OR neural correlates OR neural OR neuroimaging), (b) fMRI AND (“childhood trauma” OR child maltreatment OR childhood maltreatment OR child adversity OR child maltreated OR child deprivation OR child abuse OR child neglect OR childhood abuse), and (c) (“childhood trauma” AND (“brain imaging” OR “neuroimaging” OR brain imaging)) OR (“childhood adversity” AND (“brain imaging” OR brain imaging)). A total of 1,126 records were found by these means, 225 of which were duplicates, leaving 901 records.
Inclusion and exclusion criteria
Functional MRI studies were evaluated according to a set of inclusion and exclusion criteria; other brain scanning modalities, such as positron emission tomography or diffusion tensor imaging, were excluded. Included in the meta-analysis were original articles (a) published in English in a peer-reviewed journal, (b) with adult participants, (c) with a sample size of at least 10, (d) that used the CTQ as a measure of childhood maltreatment, (e) that used socioaffective task-based fMRI paradigms, (f) and that provided either Talairach or Montreal Neurological Institute (MNI) peak coordinates from a whole-brain (WB) and/or region of interest (ROI) activation analysis. For the meta-analyses, only WB coordinates were used. This was done in order to minimize bias introduced from ROI coordinates. The findings of our systematic review of relevant ROIs are however also referred to in the Results and Discussion sections. Excluded from the analysis were publications that were reviews or case studies, studies that did not conduct brain imaging, studies that reported on structural scans or used other imaging modalities, studies involving brain trauma rather than childhood trauma, and animal studies.
Screening procedure
After an initial screening of abstracts, 760 of the initially retrieved 901 records were excluded according to the criteria listed above. The 141 remaining records underwent full text screening for eligibility. During the full text screen, a further 124 studies were excluded due to their using a child sample (n = 8), or cognitive tasks (n = 6), not using the CTQ measure (n = 81), being connectivity or resting-state studies (n = 13), not using the CTQ scores directly (n = 12), or reporting null findings (n = 4; 2 WB and 2 ROI). Two WB studies presented null findings. Early life stress measured with the CTQ was found to have no effect on fMRI signal during the display of negative words in relatively small samples of 13 major depressive disorder patients, 15 other patients, and 13 controls (Hsu, Langenecker, Kennedy, Zubieta, & Heitzeg, Reference Hsu, Langenecker, Kennedy, Zubieta and Heitzeg2010; Hsu et al., Reference Hsu, Mickey, Langenecker, Heitzeg, Love, Wang and Enoch2012). Of note, an effect was detected with a different measure of early life stress. Two ROI studies presented null findings. In the first ROI study, no significant association was detected between CTQ scores and ventral striatum reactivity in a monetary reward task in 200 nonpatient young adults (Nikolova, Bogdan, Brigidi, & Hariri, Reference Nikolova, Bogdan, Brigidi and Hariri2012). In addition, the second ROI study reported no significant association with amygdala reactivity for negative emotional cues in a sample of 40 depressed patients and 43 controls (Tozzi et al., Reference Tozzi, Carballedo, Wetterling, McCarthy, O'Keane, Gill and Frodl2016). Of the 17 remaining studies, 5 studies reported only ROI findings, leaving 12 studies for inclusion in the WB meta-analysis. More details of the searching procedure are presented in the Preferred Reporting Items for Systematic reviews and Meta-Analyses diagram in the online-only supplementary material.
Data extraction procedure
The 12 included studies were divided into those that reported positive associations (e.g., increased activation) and those that reported negative associations (e.g., decreased activation) between childhood maltreatment and brain activation in response to socioaffective stimuli. Furthermore, analysis type was recorded, separating studies that conducted regression analyses (with CTQ scores as predictor of brain activation) from studies that did contrast analyses (comparing brain activation in high maltreatment and low maltreatment groups). Five studies included both patient and healthy control participants. In 4 of those 5 studies (Croy et al., Reference Croy, Schellong, Gerber, Joraschky, Iannilli and Hummel2010; Noll-Hussong et al., Reference Noll-Hussong, Otti, Laeer, Wohlschlaeger, Zimmer, Lahmann and Guendel2010; Skokauskas, Carballedo, Fagan, & Frodl, Reference Skokauskas, Carballedo, Fagan and Frodl2015; Steuwe et al., Reference Steuwe, Daniels, Frewen, Densmore, Pannasch, Beblo and Lanius2014), trauma-exposed patient samples were contrasted with nonexposed control samples, while in the remaining study (Van Harmelen et al., Reference Van Harmelen, Hauber, Gunther Moor, Spinhoven, Boon, Crone and Elzinga2014) both group contrasts and regression analyses were performed, and these included combined patient and control samples. Such comparisons and correlations may have been partially confounded by psychiatric status. Peak coordinates (Talairach or MNI) and field of view were extracted, as well as sample and study characteristics. Talairach coordinates were converted to MNI space using WFUpickatlas (Maldjian, Laurienti, Burdette, & Kraft, Reference Maldjian, Laurienti, Burdette and Kraft2003). Of the 12 included studies reporting WB coordinates, 6 exclusively reported positive associations, 3 reported both positive and negative associations, and 3 exclusively reported negative associations. The types of stimuli used in the included studies are detailed in Table 1. Any duplicate coordinates from regression and group comparison analyses were omitted from combined analyses.
Table 1. Design characteristics extracted from the 13 positive and 8 negative association functional MRI studies included in the meta-analyses and systematic review
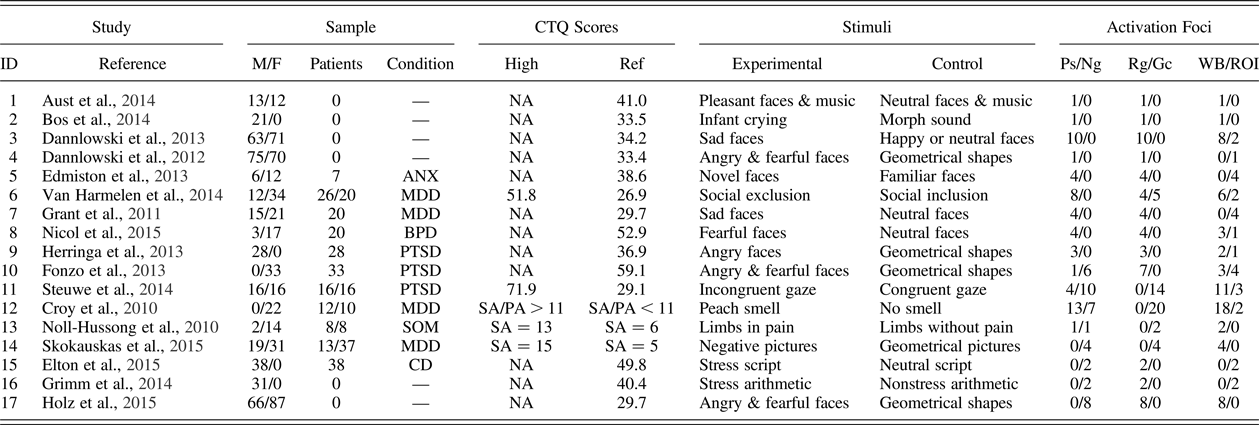
Note: CTQ, Childhood Trauma Questionnaire; M, male; F, female; Ps, postive; Ng, negative; Rg, regression; Gc, group comparison; WB, whole brain; ROI, region of interest; ANX, anxiety disorder; MDD, major depressive disorder; BPD, borderline personality disorder; PTSD, posttraumatic stress disorder; SA, sexual abuse; PA, physical abuse; SOM, somatoform disorder; CD, cocaine dependence. For group comparisons, the patients and CTQ scores columns list high and low maltreatment groups. The dominant psychiatric condition is listed in the condition column.
Activation likelihood estimation (ALE)
We used a well-established statistical modeling technique for performing neuroimaging meta-analyses, following the GingerALE protocol (http://www.brainmap.org/ale/) as determined by Eickhoff, Bzdok, Laird, Kurth, and Fox (Reference Eickhoff, Bzdok, Laird, Kurth and Fox2012), and using GingerALE version 2.3.6. ALE employs a three-dimensional Gaussian probability distribution around the foci coordinates to create a model activation map for each included study. The probability distributions were weighted by sample size, to account for between-study differences in estimation accuracy. The individual study maps were combined to create an experimental ALE map covering the whole brain. The spatial distribution of activation likelihood values across studies was tested against the null hypothesis that there is random variation in the spatial distribution of foci, regarding the within-study variance fixed. In all, both the primary and secondary analyses we used included a cluster-level inference thresholding method with 1,000 permutations and a thresholding value of p < .05 to correct for multiple comparisons (Eickhoff et al., Reference Eickhoff, Bzdok, Laird, Kurth and Fox2012). An initial threshold of p < .01 uncorrected was applied in the cluster-forming stage. To aid the interpretation of significant results, contributing studies were recorded. GingerALE considers a study to be “contributing” if its coordinates are located within the boundaries of an ALE cluster, but it does not discount other studies, which might be located near these boundaries but outside of the cluster and may have also contributed to it.
Primary and secondary analyses
All analyses were performed separately for positive and negative associations between brain activation and CTQ scores, as the analysis software requires that negative and positive associations are separately analyzed. For the primary analysis, in line with the general linear modeling statistical framework and to maximize power as done previously (van der Velde et al., Reference van der Velde, Servaas, Goerlich, Bruggeman, Horton, Costafreda and Aleman2013), regression-based and contrast-based peak coordinates were entered into a combined meta-analysis. To reduce heterogeneity, since regression-based analyses may be more sensitive to detect linear effects (e.g., Grimm et al., Reference Grimm, Pestke, Feeser, Aust, Weigand, Wang and Bajbouj2014) whereas contrast-based analyses are designed to detect differences at distribution extremes, secondary analyses were performed on those analysis types separately. In further analyses, the studies were separated based on the social nature and valence of the task stimuli (see, e.g., Miller, Hamilton, Sacchet, & Gotlib, Reference Miller, Hamilton, Sacchet and Gotlib2015, for a discussion of task-general and task-specific meta-analyses). Even though we had selected exclusively socioaffective tasks in our initial search parameters, the task using an odor stimulus was judged to be nonsocial, and a minority of the social tasks were either positive or neutral. Hence, we separately analyzed social stimuli findings and negative-valence stimuli findings.
In order to address the potential confounding of psychiatric conditions in samples that include a combination of clinical and nonclinical groups, we conducted an additional stratified analysis looking at positive associations between CTQ scores and neural activations separately in clinical and in nonclinical samples. Van Harmelen et al. (Reference Van Harmelen, Hauber, Gunther Moor, Spinhoven, Boon, Crone and Elzinga2014) reported within-group regression findings for clinical and nonclinical groups as supplementary information, and these results were included in the respective stratified analyses.
Other methods notes
In order to guarantee reliable inclusion of studies, the search criteria were predefined, and two authors (S.J.H. and N.A.G.) conducted the systematic screening of eligible papers and data extraction. Inconsistencies at any step were resolved by consensus meetings with the last author (S.J.B.). Two authors (S.J.H. and N.A.G.) were responsible for conducting the ALE analyses. We used the anatomical image overlay program Mango (http://ric.uthscsa.edu/mango) to illustrate the results of our meta-analyses in MNI space. Finally, post hoc uncorrected ALE meta-analyses (p < .001) were conducted on extracted WB peaks in order to detect any nonsignificant trends that might correspond to replicated ROI findings.
Results
Positive associations between CTQ scores and brain activation for socioaffective cues
For the primary analysis, with maximum power, the consistency in results from all nine studies reporting positive associations between CTQ scores and brain activation was examined (combining results from regression and group comparison approaches). The studies, with a total of 344 participants, contributed 42 foci to this analysis. Activation in one cluster in the left superior frontal gyrus extending into the medial frontal gyrus (centered at x = –12, y = 41, z = 43; Brodmann Area 8 [BA8]) was found to have a positive association with CTQ scores (see Table 2). Within the bounds of this cluster, there were four contributing foci from two different studies, namely, Studies 6 and 13 in Table 1. The clusters from this and the secondary analyses are depicted in Figure 1.

Figure 1. (Color online) Clusters that showed positive associations between Childhood Trauma Questionnaire scores and brain activation to socioaffective cues in (a,b) the left superior frontal gyrus and (b,c) and left middle temporal gyrus. The legend (color online) indicates activation likelihood estimation values as found in Table 2: (a) y = 46, (b) z = 39, and (c) x = −42.
Table 2. Areas showing associations between Childhood Trauma Questionnaire scores and brain activation in response to socioaffective cues in combined meta-analyses of regression and group comparison studies

Note: All ps < .05 at cluster-level inference. BA, Brodmann Area; MNI, Montreal Neurological Institute; ALE, activation likelihood estimation.
Positive associations between CTQ and activation for selectively social cues
There was only a single study reporting positive associations that did not employ a specifically social task paradigm (i.e., one that includes either human forms or sounds, or virtual interactions with other people). Hence, for the social cues only analysis, Study 12 was excluded. Combining results from regression and group comparison approaches left eight studies, with a total of 322 participants, contributing 29 foci to this analysis. The left superior/medial frontal gyrus cluster from the primary analysis was replicated, and an additional cluster emerged in the left posterior middle temporal gyrus, extending toward the parietal lobe and into the precuneus. The left middle temporal gyrus cluster (centered at x = –42, y = –72, z = 35; BA39/19), and had three contributing foci from one study (Study 6).
Positive associations between CTQ and activation for selectively negative affective cues
For a negative-affect cues only analysis, Studies 1 and 11 were excluded. Combining results from regression and group comparison approaches left six studies, with a total of 265 participants, contributing 24 foci to this analysis. The same two clusters emerged as significant; however, the middle temporal gyrus cluster now extended to include two additional contributing foci from Study 3. These results indicate that the main findings in the specifically social cues analysis were driven by negative social stimuli.
Increased activation for socioaffective cues: Regression studies and group comparison studies
To examine whether childhood maltreatment severity was associated with brain activation in a continuous fashion, a secondary analysis was performed on regression-based studies. Six studies with a total of 274 participants contributed 20 foci to the analysis. The left superior/medial frontal gyrus cluster detected in the primary analysis of stronger activation in relation to CTQ scores was replicated in this analysis, but was more medially located. The cluster (centered at x = –3, y = 52, z = 35; BA8/9/6). Three foci from one study (Study 6) contributed within the boundaries of the significant cluster. An additional secondary analysis was performed on four group comparison studies (which each directly compared groups of people with high CTQ scores to groups of people scoring low on the CTQ), allowing for nonlinear effects, with a total of 116 participants, contributing 23 foci. The cluster previously detected in the left posterior middle temporal gyrus (centered at x = –42, y = –72, z = 35; BA39/19) saw more activation in high-scoring CTQ groups versus low-scoring CTQ groups. Three foci from one study (Study 6) contributed.
Higher activation for socioaffective cues: Stratified analysis on clinical versus nonclinical groups
To address potential confounding effects of psychiatric comorbidity, we performed additional analyses stratified for clinical and nonclinical groups. The clinical group included patients with depression, anxiety, PTSD, borderline personality disorder, somatoform disorder, and cocaine dependence (see Table 1 for details). The clinical group analysis included five experiments and 132 participants, and the nonclinical group analysis included four experiments and 226 participants. No clusters emerged as significantly activated in either of these two analyses.
Negative associations between CTQ scores and brain activation for socioaffective cues
For the primary analysis on negative associations, the consistency in results from all six studies reporting negative associations between childhood maltreatment scores and brain activation was examined (combining results from regression and group comparison approaches). The studies with a total of 306 participants contributed 31 foci to this analysis. One cluster with peaks in the left superior parietal lobule, incorporating the precuneus (centered at x = –31, y = –51, z = 50; BA7/40) showed a negative association with childhood maltreatment (see Table 2). Three foci from one study (Study 14) contributed to this cluster. The clusters from this and the secondary analyses are depicted in Figure 2.

Figure 2. (Color online) Clusters that showed negative associations between Childhood Trauma Questionnaire scores and brain activation to socioaffective cues in (a,c) the left superior parietal lobule and (b,c) the left hippocampus. The legend (color online) indicates activation likelihood estimation values as found in Table 2: (a) y = −51, (b) z = −17, and (c) x = −27.
Negative associations between CTQ and activation for selectively social cues
There was only a single study reporting negative associations that did not employ a social task paradigm. For the social cues only analysis, Study 12 was excluded. Combining results from regression and group comparison approaches left five studies, with a total of 284 participants, contributing 25 foci to this analysis. The superior parietal lobule cluster from the primary analysis was replicated.
Negative associations between CTQ and activation for selectively negative affective cues
For a negative affective cues only analysis, Study 11 was additionally excluded. This left four studies, with a total of 252 participants, contributing 15 foci to this analysis. Again, the cluster from the primary analysis was replicated.
Lower activation for socioaffective cues in high CTQ groups compared to low CTQ groups
The next secondary analysis was performed on five group comparison studies, allowing for nonlinear effects, with a total of 153 participants, contributing 23 foci. Two clusters emerged as being significantly less activated in high-CTQ groups. The left superior parietal lobule cluster from the primary analysis emerged as well as a new cluster in the left hippocampus (centered at x = –26, y = –29, z = –18; BA28). Due to the low number of regression-based studies to be included, no further secondary analyses were performed for lower activation in relation to CTQ scores.
Systematic review of ROI investigations of the association between brain activation and CTQ scores
From the identified studies, 12 studies used a ROI approach. The extracted peaks could not be incorporated into the meta-analyses without introducing bias, and were therefore reviewed. The majority (7/12) of ROI studies performed a small-volume correction using a more liberal threshold for the ROI compared to the rest of the brain. The remaining studies fully reduced the field of view to an anatomical or functional ROI. All but 2 studies examined the amygdala (8 studies) and/or hippocampus (4 studies). Multiple studies focused on the ACC, medial PFC (mPFC), and insula.
One study reported higher activation in the left amygdala, one in the right amygdala, and one in the bilateral amygdala in association with CTQ scores. All of the studies presented negative faces and showed an association with severity of maltreatment exposure. One finding was potentially confounded by comorbid psychopathology. Five studies reported null findings for an amygdala ROI, two for neutral social stimuli, and three for negative faces. The majority of negative findings were in PTSD patients. Post hoc uncorrected ALE analyses (p < .001) of WB studies confirmed that right amygdala activation was positively related to CTQ scores. Findings were most prominent for negative faces (x = 26, y = –2, z = –22; k = 152 mm3) and regression-based studies (x = 26, y = –2, z = –22; k = 152 mm3).
Two studies reported lower activation in the left hippocampus in association with CTQ scores: one for olfactory and one for stress stimuli. One study reported higher activation in the left hippocampus for novel faces and a null finding was reported for angry faces. Two of the findings might have been confounded by comorbid psychopathology. Nonetheless, regional activation correlated with the severity of maltreatment exposure in two studies. These ROI findings are partly consistent with the lower activation observed in the left hippocampus in the fully corrected ALE analyses.
For the ACC, mPFC, and insula ROIs, the findings were mixed. Four studies reported higher activation, two studies reported lower activation, and one study reported null findings in the ACC or mPFC. Higher activation was mostly reported in the ventral ACC, in response to negative faces and social exclusion, and in clinical and nonclinical samples without evident confounding by comorbid psychiatric conditions. Lower activation was reported in the dorsal ACC, in response to odors and negative faces; however, findings were possibly confounded by comorbid affective disorders. Many of the ROI studies demonstrated an association between maltreatment severity and ACC activation. Post hoc uncorrected ALE analyses (p < .001) of WB studies confirmed that left ventral ACC activation (BA32/10) was positively related to CTQ scores. Findings were most prominent for negative faces (x = –12, y = 44, z = 2; k = 200 mm3) and regression-based studies (x = –12, y = 44, z = 2; k = 152 mm3). In uncorrected ALE analyses, dorsal ACC activation was more inconsistently replicated with smaller cluster sizes. For the insula, there were no replicated findings.
Discussion
Overview of main findings
This meta-analysis of fMRI studies examining brain activation in response to socioaffective cues suggested that the superior frontal gyrus (location corresponding to the dorsomedial PFC) and left temporoparietal cortex may be hyperresponsive in adults exposed to childhood maltreatment. Secondary analyses indicated that these activation abnormalities were largely driven by studies presenting negative socioaffective cues. Hypoactivation was observed in the left superior parietal cortex and hippocampus, although these results were somewhat less robust. Further, our review of ROI studies revealed a hyporesponsivity in the hippocampus, and hyperresponsivity in the amygdala and ventral ACC, coupled with childhood maltreatment severity. These findings may indicate that childhood maltreatment affects brain functioning in a dose-dependent way (i.e., the more frequent, disruptive, and encompassing the maltreatment experience, the stronger the hyperresponsivity to future threatening stimuli). Combined, these findings tentatively suggest that childhood maltreatment may have a profound, long-lasting impact on the functioning of social and emotional brain circuitry in adults.
Interpretation of observed activation differences in the context of typical socioemotional processing
Elevated activation in the dorsomedial PFC and in the left temporoparietal junction was associated with childhood maltreatment exposure. These two regions have also been associated with maltreatment in a ROI study (Steuwe et al., Reference Steuwe, Daniels, Frewen, Densmore, Pannasch, Beblo and Lanius2014) comparing direct versus averted gaze pictures. Dorsomedial PFC (over)activation has been interpreted as a sign of social uncertainty and distress when faced with the prospect of social rejection (Van Harmelen et al., Reference Van Harmelen, Hauber, Gunther Moor, Spinhoven, Boon, Crone and Elzinga2014). Similarly, the link between child maltreatment and dorsomedial PFC reactivity to pain-related cues might index a general increased tendency to perceive danger (Noll-Hussong et al., Reference Noll-Hussong, Otti, Laeer, Wohlschlaeger, Zimmer, Lahmann and Guendel2010). The dorsomedial PFC activation might reflect a monitoring process focused on evaluating whether negative information from the immediate social environment is relevant to oneself or not (van der Meer, Costafreda, Aleman, & David, Reference van der Meer, Costafreda, Aleman and David2010). Furthermore, it could be indicative of an increased sensitivity to social hierarchy and social stress, in line with previous work (Muscatell et al., Reference Muscatell, Dedovic, Slavich, Jarcho, Breen, Bower and Eisenberger2016). The left temporoparietal junction finding was fully driven by group comparison studies, which suggest that more extreme traumatic experiences might be particularly influential. This region is typically activated by a broad array of social cognitive tasks requiring abstract reasoning and other-perspective taking (Schurz, Radua, Aichhorn, Richlan, & Perner, Reference Schurz, Radua, Aichhorn, Richlan and Perner2014), which is in line with an excessive focus on cues for negative judgments by others. The findings here therefore suggest that social monitoring, social uncertainty, and social pain concepts deserve further neuroscientific investigation in adults with a history of childhood maltreatment.
Consistent with the findings from the positive association analysis, the negative association between child maltreatment and activation in the superior parietal cortex was also most prominently present for negative social cues. It has been suggested that lower parietal activation is associated with impairments in attending to and navigation of the surrounding environment (Skokauskas et al., Reference Skokauskas, Carballedo, Fagan and Frodl2015). This is particularly relevant for negative social cues, as attentional control subserved by the superior parietal cortex plays a critical role in downregulating the impact of negative emotional information (Buhle et al., Reference Buhle, Silvers, Wager, Lopez, Onyemekwu, Kober and Ochsner2014). Of note, this particular association was observed in depressed patients who were exposed to sexual abuse in childhood. It needs to be verified whether the association holds for nonclinical groups. The left hippocampus was found to be hypoactive in group comparisons of high maltreatment versus low maltreatment groups, and this was replicated in ROI studies. Microstructural defects after severe traumatic experiences might play a role in altered functioning of the hippocampal complex later in life (Hoeijmakers et al., Reference Hoeijmakers, Lucassen and Korosi2014). One of the ROI studies demonstrated hyporesponsivity of the left hippocampus in a stress experiment (Grimm et al., Reference Grimm, Pestke, Feeser, Aust, Weigand, Wang and Bajbouj2014). It could be speculated that the observed hypoactivation attests to blunted or exhausted stress reactivity in adults after exposure to childhood trauma.
The most important finding from the ROI review was that negative facial expressions activate amplified responsivity in the amygdala and ventral ACC in adults exposed to childhood maltreatment. The amygdala is seen as a critical node for detecting salient information in the environment, and functions as a gateway for prioritizing downstream information processing (Sander, Grafman, & Zalla, Reference Sander, Grafman and Zalla2003). Amygdala activation also covaries with the subconscious perception of untrustworthiness in faces (Freeman, Stolier, Ingbretsen, & Hehman, Reference Freeman, Stolier, Ingbretsen and Hehman2014). After exposure to childhood maltreatment, negative facial expressions such as anger, fear, and sadness may elicit heightened vigilance and tension (McCrory & Viding, Reference McCrory and Viding2015). The ventral ACC plays a major role in the pathophysiology of depression (Hamani et al., Reference Hamani, Mayberg, Stone, Laxton, Haber and Lozano2011), and activation in this area has been linearly associated with a negatively biased cognitions and vulnerability for depression (Groenewold et al., Reference Groenewold, Roest, Renken, Opmeer, Veltman, van der Wee and Harmer2015). Of note, the amygdala and ACC ROI findings were consistent with uncorrected but not with corrected meta-analytic findings. It is important to consider therefore that ROI replications may be subject to experimenter bias. Nonetheless, the observed discrepancies in amygdala findings are likely to be at least partly explained by methodological characteristics of the input studies. Studies that have implemented optimized sequences and tasks (e.g., negative faces) for obtaining signal in amygdala ROIs might preferentially present ROI rather than WB analyses. Further, the small size of the amygdala might preclude obtaining significance in WB analyses that apply stringent cluster-extent thresholding without small-volume correction.
Implications of findings for research into vulnerability for psychopathology
The brain areas found to be associated with childhood maltreatment are assumed to play a key role in the pathophysiology of a diversity of trauma-related psychiatric disorders, most notably PTSD (Brooks & Stein, Reference Brooks and Stein2015; Etkin & Wager, Reference Etkin and Wager2007). The convergence in findings for childhood maltreatment and anxiety disorders is in line with the viewpoint of latent vulnerability (McCrory & Viding, Reference McCrory and Viding2015), suggesting that hypervigilance to social and affective threat is a neurocognitive process that is present prior to the development of psychopathology, exacerbating susceptibility to the development of psychopathology under challenging circumstances. Of note, similar functional abnormalities in frontolimbic brain structures have been reported in at-risk child and adolescent samples (Barch, Reference Barch2014; Clauss, Avery, & Blackford, Reference Clauss, Avery and Blackford2015), correlate with vulnerability measures for psychopathology in healthy adult cohorts (Groenewold et al., Reference Groenewold, Roest, Renken, Opmeer, Veltman, van der Wee and Harmer2015; Williams et al., Reference Williams, Gatt, Schofield, Olivieri, Peduto and Gordon2009; Zhong et al., Reference Zhong, Wang, Xiao, Yi, Zhu, Liao and Yao2011), and have shown predictive value for disrupted negative affectivity and stress reactivity in prospective studies (Admon et al., Reference Admon, Lubin, Stern, Rosenberg, Sela, Ben-Ami and Hendler2009; Swartz, Knodt, Radtke, & Hariri, Reference Swartz, Knodt, Radtke and Hariri2015). Our meta-analysis adds to these findings by suggesting that recollected childhood maltreatment is associated with altered brain activity many years after maltreatment exposure. It is possible that the nature of alterations in brain activation associated with CTQ scores is dependent on the type of clinical outcome. In our secondary analysis selectively including clinical samples, the inclusion of diverse clinical samples may contribute to the observed inconsistency in findings. It should also be noted that the large majority of patient studies included in the meta-analysis investigated the effects of childhood maltreatment within their patient sample, eliminating the confounding effects of psychiatric diagnosis, and that both clinical and nonclinical samples contributed to the amplified activation findings in our meta-analysis. This suggests some commonality between clinical and nonclinical groups in brain activation outcomes following childhood maltreatment exposure.
It is an interesting observation that most of the studies reporting lower activation in areas associated with attentional control and stress regulation were conducted in clinical groups selected for severe abuse histories, or involved stress elicitation. This is in line with the prevailing hypothesis that dysfunction of neurocircuitry governing such regulatory processes may possibly play a causal role in disorder pathogenesis (Arnsten, Reference Arnsten2009; Gilbertson et al., Reference Gilbertson, Shenton, Ciszewski, Kasai, Lasko, Orr and Pitman2002). Still, it remains to be determined whether brain functional abnormalities are evident in all individuals exposed to maltreatment or merely in a subgroup, whether newly encountered stressors in adulthood modulate functional alterations, and whether there are meaningful differences between resilient and vulnerable individuals prior to exposure to stressors.
Strengths and limitations
The present study is the first meta-analysis to summarize the results from fMRI studies on the effects of childhood maltreatment on socioaffective processing. A rigorous systematic literature search was conducted following Preferred Reporting Items for Systematic reviews and Meta-Analyses guidelines. Analyses were performed with a well-established statistical technique (ALE), conducting random-effects analysis to accurately weigh the contributions of separate experiments, with appropriate multiple comparisons corrections. Since the adopted analytical approach evaluates the spatial proximity of included peak coordinates against a random pattern, it did not allow for inclusion of null findings (due to the absence of peak coordinates), generation of pooled effect size estimates across included studies, or assessment of publication bias with a funnel plot. It also did not allow for capturing subanalyses in a multilevel data structure; thus, the analysis here is of the CTQ total score. Regrettably, the paucity of eligible studies forced us to collapse studies across sample characteristics (not only psychiatric conditions but also other conditions such as alexithymia), and thus conclusions regarding generalization of the results still remain preliminary. The inclusion of mixed-sex samples adds to this, as response to maltreatment may differ by sex for a number of reasons (e.g., Bale & Epperson, Reference Bale and Epperson2015). Moreover, the results from the primary meta-analyses were not replicated in analyses stratified for nonclinical and clinical samples, and therefore some of the findings may be confounded by an active psychiatric diagnosis, although findings in nonclinical groups (Dannlowski et al., Reference Dannlowski, Kugel, Huber, Stuhrmann, Redlich, Grotegerd and Suslow2013; Van Harmelen et al., Reference Van Harmelen, Hauber, Gunther Moor, Spinhoven, Boon, Crone and Elzinga2014) did contribute to the findings and power in stratified analyses was relatively low. It is worth noting that traumatic experiences in adulthood and current stress levels would not be captured by the CTQ measure, and therefore it remains uncertain to what extent such stressors may contribute to the observed alterations in neural responsivity. Unfortunately, very few of the included studies reported results that were adjusted for these potentially informative covariates. Finally, although well validated, the CTQ is a self-report measure rendering assessments vulnerable to recall bias over time. In particular, a negative memory bias may to some extent inflate CTQ scores, and partly confound the reported associations. Longitudinal studies are thus warranted to replicate the results from this meta-analysis.
Directions for future research
Although most studies included in this meta-analysis defined maltreatment as an umbrella concept, several studies investigated physical and sexual abuse as a separate construct to neglect (see Croy et al., Reference Croy, Schellong, Gerber, Joraschky, Iannilli and Hummel2010; Nicol, Pope, Romaniuk, & Hall, Reference Nicol, Pope, Romaniuk and Hall2015; Noll-Hussong et al., Reference Noll-Hussong, Otti, Laeer, Wohlschlaeger, Zimmer, Lahmann and Guendel2010; Skokauskas et al., Reference Skokauskas, Carballedo, Fagan and Frodl2015). This distinction may be relevant for neuroimaging research, since it has recently been proposed that abuse predominantly impacts threat-related processing, whereas neglect or deprivation may have a broader impact on neurocognitive development (McLaughlin, Sheridan, & Lambert, Reference McLaughlin, Sheridan and Lambert2014). This interesting hypothesis deserves further investigation, which may be realized through data-sharing initiatives allowing for mega-analyses on subject-level data points. Given that the scale at which fMRI studies are performed is continually increasing, there are opportunities to longitudinally investigate risk groups from adolescence to early adulthood, the age at which psychopathology generally starts to manifest. Such a design is required to test the hypothesis that neural reactivity to threat cues as a function of childhood maltreatment exposure is maintained across neurodevelopment, and predicts onset of affective psychopathology.
Conclusions
This study provides preliminary evidence that exposure to childhood maltreatment is associated with hyperactivation and hypoactivation of frontolimbic and temporoparietal brain areas during socioaffective processing in adulthood, which appears to be most prominent for cues that indicate a potential threat. The mPFC, temporoparietal junction, hippocampus, and superior parietal lobule were identified as key regions. There was marked heterogeneity in the reviewed ROI findings for the hippocampus, amygdala, and ACC, and potential effect modifiers deserve further exploration. The results suggest that childhood maltreatment has long-lasting effects on brain function, and brain functional abnormalities should continue to be investigated as a potential mechanism conveying vulnerability for the development of psychopathology after early life adversity.
Supplementary Material
To view the supplementary material for this article, please visit https://doi.org/10.1017/S0954579417001717.