Introduction
The knowledge on fascioliasis has pronouncedly changed in recent years (Mas-Coma et al., Reference Mas-Coma, Bargues and Valero2014a).
From the veterinary point of view, epidemiological research focuses on the influences of climate change and global change, similarly as with other trematodiases (Boissier et al., Reference Boissier, Moné, Mitta, Bargues, Molyneux and Mas-Coma2015). Indeed, fascioliasis is a vector-borne disease of rural areas caused by the liver fluke species Fasciola hepatica of almost worldwide distribution and Fasciola gigantica restricted to parts of Africa and Asia. Their vectors are amphibious freshwater lymnaeid snails, which are markedly influenced by changing climatic factors (Mas-Coma et al., Reference Mas-Coma, Valero and Bargues2009a; Afshan et al., Reference Afshan, Fortes-Lima, Artigas, Valero, Qayyum and Mas-Coma2014). Among lymnaeid vector species, Galba truncatula is the most important transmitter of the disease to humans almost in all endemic areas where F. hepatica is the causal agent, as in Europe and throughout all human hyperendemic areas in Andean countries (Mas-Coma et al., Reference Mas-Coma, Funatsu and Bargues2001, Reference Mas-Coma, Valero and Bargues2009b; Bargues et al., Reference Bargues, Mera y Sierra, Gomez, Artigas and Mas-Coma2007a, Reference Bargues, Artigas, Khoubbane, Ortiz, Naquira and Mas-Coma2012, Reference Bargues, Artigas, Angles, Osca, Duran, Buchon, Gonzales-Pomar, Pinto-Mendieta and Mas-Coma2020; Artigas et al., Reference Artigas, Bargues, Mera y Sierra, Agramunt and Mas-Coma2011). In areas where this lymnaeid species is not present, human infection sustained by other species of the Galba/Fossaria group shows relatively low transmission levels (Zumaquero-Rios et al., Reference Zumaquero-Rios, Sarracent-Perez, Rojas-Garcia, Rojas-Rivero, Martinez-Tovilla, Valero and Mas-Coma2013; Bargues et al., Reference Bargues, Malandrini, Artigas, Soria, Velazquez, Carnevale, Mateo, Khoubbane and Mas-Coma2016, Reference Bargues, Gayo, Sanchis, Artigas, Khoubbane, Birriel and Mas-Coma2017).
Regarding global change, aspects related to liver fluke spread include anthropogenic modifications of the environment, such as irrigation systems (Esteban et al., Reference Esteban, Gonzalez, Bargues, Angles, Sanchez, Naquira and Mas-Coma2002; Afshan et al., Reference Afshan, Fortes-Lima, Artigas, Valero, Qayyum and Mas-Coma2014) and animal transport inside countries, as, for example, transhumance (Mas-Coma et al., Reference Mas-Coma, Valero and Bargues2009b; Chougar et al., Reference Chougar, Mas-Coma, Artigas, Harhoura, Aissi, Agramunt and Bargues2020), and livestock import/export between countries (Villavicencio et al., Reference Villavicencio, Bargues, Artigas, Guaman, Ulloa, Romero, Osca and Mas-Coma2019) or even different continents (Mas-Coma et al., Reference Mas-Coma, Valero and Bargues2009b).
From the public health point of view, human fascioliasis was considered a pathogenic disease mainly in the first short acute phase of 3–4 months. Consequently, it was considered of secondary public health importance until 1990 (Chen & Mott, Reference Chen and Mott1990). Complex clinical pictures with very severe neurological, meningeal, psychiatric and ophthalmological disorders (Mas-Coma et al., Reference Mas-Coma, Agramunt and Valero2014b) may be caused at distance by numerous migrating juveniles during the acute phase but also by a few adult flukes in the liver during the chronic phase (Gonzalez-Miguel et al., Reference Gonzalez-Miguel, Valero, Reguera-Gomez, Mas-Bargues, Bargues, Simon-Martin and Mas-Coma2019). Such situations may leave handicapped subjects behind (Mas-Coma et al., Reference Mas-Coma, Agramunt and Valero2014b). Patients not completely recovered have been reported many years after having been appropriately treated, despite their infections initially considered to be not problematic (Rondelaud et al., Reference Rondelaud, Dreyfuss and Vignoles2006).
The marked diversity of human infection sources adds to the complexity and public health importance of this zoonotic disease, which is characterized by a low specificity at animal host level. In domestic animal reservoirs, similarly as in lymnaeids, anthropophily stands out as a crucial factor because it assures a transmission closer to the human environment and, therefore, increases human infection probabilities (Mas-Coma et al., Reference Mas-Coma, Bargues and Valero2018).
Among the domestic animal species involved as reservoirs in the human endemic areas, species differ slightly from one area to another and mainly from one continent to another. In the Americas, cattle and sheep are the most usual, followed by pigs. In Africa and Asia, besides goats, the buffalo is an additional reservoir, mainly in areas where F. gigantica is present. There are other herbivorous mammal species that have been reported as secondary reservoirs at local level. Equines have been given attention only sporadically, despite their closeness to humans and their crucial role in human development in the past and still in rural areas worldwide in the present. However, the risk of climate and global changes to fascioliasis of equines has been recently highlighted (Williams & Hodgkinson, Reference Williams and Hodgkinson2017).
Donkeys appear to be the equines having received more focus. Indeed, recent palaeoparasitological studies have demonstrated that old donkeys, known as onagers, were already infected by F. hepatica during the Sassanid period, 224–651 AD, in the former Fertile Crescent area, in present Iran (Askari et al., Reference Askari, Mas-Coma, Bouwman, Boenke, Stôllner, Aali, Rezaiian and Mowlavi2018). At present, donkeys appear to be usually infected by fasciolids in countries of the Fertile Crescent–Ancient Egypt region, or neighbouring that region, such as in Iran, Iraq, Turkey, Egypt and Ethiopia, with prevalence from low to very high (see review in Askari et al., Reference Askari, Mas-Coma, Bouwman, Boenke, Stôllner, Aali, Rezaiian and Mowlavi2018). However, they have been reported to show liver fluke infection in many other countries, by F. hepatica in countries such as Morocco (Everaert et al., Reference Everaert, Jawhari and Gaufreteau1974; Pandey, Reference Pandey1983), Mexico (Collins, Reference Collins1961), Bolivia (Mas-Coma et al., Reference Mas-Coma, Rodriguez, Bargues, Valero, Coello and Angles1997; Valero et al., Reference Valero, Darce, Panova and Mas-Coma2001a) and Argentina (Mera y Sierra et al., Reference Mera y Sierra, Artigas, Cuervo, Deis, Sidoti, Mas-Coma and Bargues2009), as well as by F. gigantica in Chad (Guilhon & Graber, Reference Guilhon and Graber1963; Graber, Reference Graber1969). The reservoir role of donkeys in human endemic areas has been highlighted in Bolivia (Mas-Coma et al., Reference Bargues, Mangold, Muñoz-Antoli, Pointier and Mas-Coma1997; Valero et al., Reference Valero, Darce, Panova and Mas-Coma2001a) and Egypt (Haridy et al., Reference Haridy, Morsy, Gawish, Antonios and Abdel Gawad2002). The high pathogenicity and mortality induced by Fasciola in donkeys is an important factor to be considered (Askari et al., Reference Askari, Mas-Coma, Bouwman, Boenke, Stôllner, Aali, Rezaiian and Mowlavi2018).
Horses are known to be infected by Fasciola since two centuries ago (Galli-Valerio, Reference Galli-Valerio1893). A review of the countries in which natural infection of horses has been reported was made in 1988 in a report from South Africa (Alves et al., Reference Alves, van Rensburg and van Wyk1988). Additional findings in horses include countries as different as Italy (Galli-Valerio, Reference Galli-Valerio1893), Ireland (Quigley et al., Reference Quigley, Sekiya, Egan, Wolfe, Negredo and Mulcahy2017), the UK (Howell et al., Reference Howell, Malalana and Beesley2020), Egypt (Haridy et al., Reference Haridy, Morsy, Gawish, Antonios and Abdel Gawad2002), Chile (Alcaino & Aguilar, Reference Alcaino and Aguilar1985; Alcaino et al., Reference Alcaino, Vega, Klein, Gorman and Apt1988, Reference Alcaino, Gorman, Gonzalez, Vega and Apt1990, Reference Alcaino, Apt, Vega, Gorman and Apt1992), Uruguay (Sanchis Polto et al., Reference Sanchis Polto, Madeira de Carvalho, Bonilla, Duque de Araujo, Arroyo, Suarez, Solari, Romero, Sanchez Andrade, Paz-Silva, Arias Vázquez and Sánchez-Andrade Fernandez2014; Bargues et al., Reference Bargues, Gayo, Sanchis, Artigas, Khoubbane, Birriel and Mas-Coma2017), Argentina (Mera y Sierra et al., Reference Mera y Sierra, Artigas, Cuervo, Deis, Sidoti, Mas-Coma and Bargues2009) and Australia (Bucknell et al., Reference Bucknell, Gasser and Beveridge1995). Infected racehorses have also been the focus of research, as in Europe (Krawiecki, Reference Krawiecki1986) and Chile (Alcaino et al., Reference Alcaino, Gorman, Guevara and Fernandez1983, Reference Alcaino, Parra and Gorman2005; Montes et al., Reference Montes, Zurita and Uhart1984; Muñoz et al., Reference Muñoz, Rubilar, Zamora, Sepulveda, Rehhof and Ortiz2008). Also in Chile, studies highlighted the infection of horses in human fascioliasis-endemic areas (Apt et al., Reference Apt, Aguilera and Vega1993). Compared to donkeys, horses appear to be more resistant to liver fluke infection (Alves et al., Reference Alves, van Rensburg and van Wyk1988; Soulé et al., Reference Soulé, Boulard, Levieux, Barnouin and Plateau1989; Dorchies, Reference Dorchies2010). Even ponies have been reported to be experimentally susceptible to liver fluke infection (Grelck et al., Reference Grelck, Horchner and Wohrl1977) and also infected in their natural environment (Owen, Reference Owen1977).
Infection of mules by the liver fluke has, however, been the focus of only very limited research. Fasciola infection was diagnosed by serology in five mules in Turkey (Acici et al., Reference Acici, Bolukbas, Gurler, Umur and Buyuktanir2013) and by coprology in another five mules in Catamarca, Argentina (Malandrini et al., Reference Malandrini, Soria, Carnevale, Velazquez and Pizarro2009). Their role in the transmission of the disease, epidemiological importance as reservoirs regarding human infection and pathogenicity caused by F. hepatica in them, are still aspects in need of investigation. The present study analyses these three aspects in mules for the first time.
This study was performed in high-altitude areas of the Andean region in Mendoza province, Argentina. Fascioliasis is endemic in this country, posing an important public health problem much more widespread than traditionally supposed, particularly in mountainous regions (Malandrini et al., Reference Malandrini, Soria, Foresi and Correa2002; Mera y Sierra et al., Reference Mera y Sierra, Agramunt, Cuervo and Mas-Coma2011, Bargues et al., Reference Bargues, Malandrini, Artigas, Soria, Velazquez, Carnevale, Mateo, Khoubbane and Mas-Coma2016), and an animal fascioliasis impact in most of its territories (Olaechea, Reference Olaechea, Suárez, Olaechea, Rossanigo and Romero2007; Mera y Sierra et al., Reference Mera y Sierra, Artigas, Cuervo, Deis, Sidoti, Mas-Coma and Bargues2009, Cuervo et al., Reference Cuervo, Sidoti, Fantozzi, Neira, Gerbeno and Mera y Sierra2013, Reference Cuervo, Cataldo and Fantozzi2015). These mules are currently used in diverse farming activities and mainly for the transport of people and goods from their stations, following different itineraries close to the Chile border, according to the weather conditions of the different seasons.
Materials and methods
Mule groups analysed
Mules studied were sampled in Mendoza province. These mules are moved to different Andean localities of Midwestern Argentina throughout the year, depending on the work that has to be done and the weather conditions in relation to the different seasons. Two groups of mules were studied.
A group of mules was stationed at Puente del Inca at 2790 m above sea level (asl) during the summer period (December to March), where they transport goods and persons through the tracks of the Aconcagua Provincial Park up to Plaza de Mulas at 4379 m asl (fig. 1). The site called Punta de Vacas is used as the base for these expeditions. Mules continuously arrive to and leave from Puente del Inca during the period between December and the beginning of March, in which all is closed due to the start of the great snowfalls. After activities in the Aconcagua Provincial Park, this group overwinters in Ansilta, located in the province of San Juan, or in San Carlos (Mendoza province). The second group of mules is permanently stationed in Uspallata (fig. 1).

Fig. 1. Maps showing the geographical area where the working mules are used. (A) Argentina and neighbouring countries in South America showing the area of working mules (yellow rectangle); (B) the Argentinian province of Mendoza showing mule displacements to overwintering sites; (C) Aconcagua Provincial Park in the Mendoza province close to the Chile border, showing tracks followed by the mules, the base camps of Puente del Inca and Punta de Vacas, and the base station of Uspallata.
In addition to the aforementioned usual paths, these mules sometimes follow another less transited route to Plaza Argentina, which involves a more dangerous climb to the Aconcagua peak through the Glaciar de los Polacos.
Interestingly, several of these mules are sometimes sent to the locality of Cochico, at only 468 m asl, in the province of La Pampa. It should be highlighted that Cochico is very far away from the fascioliasis-endemic areas of Mendoza.
Even though both male and female mules are used, females are preferred due to their greater docility, and included 75% of the animals. The breeds of donkeys and mares used in their production varied; thus, they are not genetically uniform and the donkeys and mares used are frequently crossbreeds. The army uses male Catalan donkeys and the mares are either of Argentine Creole breed or Thoroughbred crossbreeds. The mules analysed included 4–19-year-old specimens, with a mean value of 11 ± 3.8 years old.
Mule coprology and fasciolid egg morphometry
Faecal samples from 208 mules were obtained at the beginning of the mule routes in Puente del Inca and taken directly from the rectum. A total of 81 mules studied were from Aconcagua and another 127 mules were from Uspallata. The samples were stored at 4°C in plastic bags until they arrived at the laboratory. They were then analysed using the Lumbreras sedimentation technique (Lumbreras et al., Reference Lumbreras, Cantella and Burga1962), by initially weighing 10 g of the sample to quantify the eggs per gram (epg). The Wisconsin sugar flotation technique was performed to investigate and quantify the presence of other parasites (Cox & Todd, Reference Cox and Todd1962) in 36 mules that were positive for F. hepatica in the coprological analysis. Larvae culture (Roberts & O'Sullivan, Reference Roberts and O'Sullivan1950) was performed to obtain third-stage larvae in animals with higher strongyle epg output.
To estimate the amount of fasciolid eggs that each mule could eliminate, the amount of faeces produced by an adult equine (USDA-NRCS, 2000) was multiplied by the mean of epg produced.
Fasciola hepatica eggs were obtained from mule faeces. A total of 179 eggs were measured, of which 110 eggs were from mules of Aconcagua and 69 eggs were from mules of Uspallata. Egg measurements were carried out under a calibrated microscope and obtained according to standardized methods (Valero et al., Reference Valero, Panova and Mas-Coma2005), including egg length (EL), egg width (EW), egg shape (EL/EW ratio) and egg size (EL multiplied by EW). For each measure, minimum and maximum values, mean and standard deviation were determined.
For statistical analyses, the proportion of positive animals from both sites was compared by means of the Fisher's test. The comparison of egg measurement data was done by means of the Mann–Whitney U-test, and considered significant when P < 0.05.
DNA marker sequencing of lymnaeid snails
Markers used
Lymnaeids used for the experimental infection to assess transmission capacity of mule fasciolids were from a snail population in Uspallata. They were molecularly characterized to allow for their systematic classification. DNA sequencing of the complete nuclear ribosomal DNA (rDNA) internal transcribed spacer (ITS) markers ITS-2 and ITS-1 was thus performed. The usefulness of these markers has been already proved for the classification of lymnaeid vector species and the comparative assessment of their intraspecific variability in many countries of Latin America (Bargues et al., Reference Bargues, Gonzalez, Artigas and Mas-Coma2011a, Reference Bargues, Artigas, Khoubbane, Ortiz, Naquira and Mas-Coma2012), including Argentina (Bargues et al., Reference Bargues, Mera y Sierra, Gomez, Artigas and Mas-Coma2006, Reference Bargues, Mera y Sierra, Gomez, Artigas and Mas-Coma2007a, Reference Bargues, Artigas, Mera y Sierra, Pointier and Mas-Comab, Reference Bargues, Malandrini, Artigas, Soria, Velazquez, Carnevale, Mateo, Khoubbane and Mas-Coma2016; Mera y Sierra et al., Reference Mera y Sierra, Artigas, Cuervo, Deis, Sidoti, Mas-Coma and Bargues2009) and neighbouring countries such as Bolivia (Bargues et al., Reference Bargues, Artigas, Angles, Osca, Duran, Buchon, Gonzales-Pomar, Pinto-Mendieta and Mas-Coma2020), Chile (Artigas et al., Reference Artigas, Bargues, Mera y Sierra, Agramunt and Mas-Coma2011) and Uruguay (Bargues et al., Reference Bargues, Gayo, Sanchis, Artigas, Khoubbane, Birriel and Mas-Coma2017). It should be considered that, in the Americas, F. hepatica is transmitted by lymnaeid species belonging to the Galba/Fossaria group, which are undifferentiable morphologically (Bargues et al., Reference Bargues, Artigas, Mera y Sierra, Pointier and Mas-Coma2007b, Reference Bargues, Gonzalez, Artigas and Mas-Coma2011a, Reference Bargues, Artigas, Khoubbane, Flores, Glöer, Rojas-Garcia, Ashrafi, Falkner and Mas-Comab).
DNA extraction
The head-foot tissue of each snail, preserved in 70% ethanol at room temperature after collection, was used for DNA extraction with phenol–chloroform and DNA was precipitated with ethanol. Ten specimens randomly selected from the Uspallata population were suspended in 400 μl of lysis buffer (10 mM Tris Hydrochloride, pH 8.0, 100 mM ethylenediamine tetra-acetic acid (EDTA), 100 mM sodium chloride, 1% sodium dodecyl sulphate) containing 500 μg/ml Proteinase K (Promega, Madison, Wisconsin, USA) and digested for 2 h at 55°C with alternate shaking each 15 min. The procedure steps were performed according to methods outlined previously (Bargues et al., Reference Bargues, Mangold, Muñoz-Antoli, Pointier and Mas-Coma1997, Reference Bargues, Artigas, Mera y Sierra, Pointier and Mas-Coma2007b). The pellet was dried and resuspended in 30 μl sterile TE buffer (pH 8.0) and this suspension was finally stored at −20°C until use.
Sequencing of rDNA markers
Each one of the two markers were polymerase chain reaction (PCR) amplified independently for each specimen and each PCR product was sequenced for a bona fide haplotype characterization. The complete rDNA spacers ITS-2 and ITS-1 were amplified using primers previously described (Bargues et al., Reference Bargues, Horak, Patzner, Pointier, Jackiewicz, Meier-Brook and Mas-Coma2003, Reference Bargues, Artigas, Mera y Sierra, Pointier and Mas-Coma2007b). Amplification procedures and thermal cycler conditions for each one of the DNA markers were carried out in a Mastercycle ep Gradient (Eppendorf, Hamburg, Germany), as previously described (Bargues et al., Reference Bargues, Artigas, Mera y Sierra, Pointier and Mas-Coma2007b).
PCR products were purified using the Ultra Clean™ PCR Clean-up DNA Purification System (MoBio, Solana Beach, California, USA) according to the manufacturer's protocol, and resuspended in 50 μl of 10 mM TE buffer (pH 7.6). The final DNA concentration was determined by measuring the absorbance at 260 and 280 nm on an Eppendorf BioPhotometer (Hamburg, Germany).
The sequencing of each molecular marker was performed on both strands by the dideoxy chain-termination method. It was carried out with the Taq dye-terminator chemistry kit on an Applied Biosystems 3730xl DNA Analyzer (Applied Biosystems, Foster City, California, USA) using PCR primers.
Sequence analyses
Sequences were edited and assembled using Sequencher v5.4.6. (Gene Codes Co., Ann Arbor, Michigan, USA)) and aligned using CLUSTALW2 (Larkin et al., Reference Larkin, Blackshields and Brown2007) in MEGA 6.0.6 (Tamura et al., Reference Tamura, Stecher, Peterson, Filipski and Kumar2013), using default settings. Minor corrections for a better fit of nucleotide or indel correspondences were made. Homologies were performed using the BLASTN programme from the National Center for Biotechnology information website (http://www.ncbi.nlm.nih.gov/BLAST). Comparative sequence analyses and haplotype identification of lymnaeids were made using available ribosomal sequence data downloaded from GenBank.
DNA haplotype nomenclature
The haplotype (H) terminology used for the sequences obtained follows the standard nomenclature proposed for lymnaeid snails previously described (Bargues & Mas-Coma, Reference Bargues and Mas-Coma2005; Mas-Coma et al., Reference Mas-Coma, Valero and Bargues2009b). It shall be noted that haplotype codes are only definitive in the case of complete sequences. When dealing with fragments or incomplete sequences, haplotype codes are provisional.
Experimental infection of lymnaeids
Fasciolid eggs were washed in water and preserved in darkness at 4–6°C until used. For embryonation, they were kept in darkness in Petri dishes at 24°C for 14 days. Afterwards, they were exposed to light to stimulate hatching of the miracidia, which was observed under the stereomicroscope.
Specimens of a snail population from Uspallata (32°35′25.25″S, 69°21′04.95″W; 1881 m asl) and systematically classified by the aforementioned molecular methods were used. The lymnaeids were maintained in Petri dishes in climatic chambers with a temperature of 16–18°C, according to previous experiments having demonstrated that higher temperatures were associated with a high mortality. A 12 h/12 h light/darkness photoperiod was applied (Mas-Coma et al., Reference Mas-Coma, Funatsu and Bargues2001). They were fed ad libitum with fresh lettuce and commercial fish flakes (Schulet S.A., Provincia de Buenos Aires, Argentina) following indications of previous studies (Rondelaud et al., Reference Rondelaud, Abrous and Dreyfuss2002; Belfaiza et al., Reference Belfaiza, Abrous, Rondelaud, Moncef and Dreyfuss2004). The water was changed weekly, always using water that was brought from the original location.
Snails that reached at least 4 mm in length were put in individual Petri dishes (one snail per dish) with natural freshwater from the original site. The miracidia were collected by a micropipette. Snails were placed individually in Petri dishes at 22°C with natural water and exposed to one miracidium. Meanwhile, they were observed under a stereomicroscope until confirmation that the miracidium had penetrated the snail. The snails were then returned to the climatic chamber. From day 30 onwards, the snails were observed under the stereomicroscope for the presence of metacercariae in the Petri dishes and quantification of their metacercarial production.
Haematological parameters and biochemical markers
From 13 mules that were positive for F. hepatica in the coprological analysis, blood samples were collected from the jugular vein. Due to the lack of adequate installations in the remote regions where the samples were taken, difficulties were encountered in the handling of some of the animals. Thus, collection of blood samples or the necessary blood volume was not always possible. The sample for haemogram (tube with EDTA) was prioritized and biochemical parameters were determined according to the amount of sample (tube without anticoagulant) available. Haemograms were performed in 13 mules and different biochemical parameters determined in 12 mules. The whole blood sample was stored at 4°C right after collection for subsequent haematological analysis. The non-anticoagulant tube sample was allowed to clot (for serum) and then the sample was centrifuged at 1000g for 5 min to separate the blood components. The serum samples were then stored in 1.5 ml microcentrifuge tubes at 4°C until they were analysed at the laboratory.
The following haematological parameters were determined in an Abacus Junior Vet® (Diatron, Budapest, Hungary) automated haematology analyser: red blood cell count (RBC), haemoglobin concentration (HGB), packed cell volume (PCV), platelets (Plt) and total leucocyte count (WBC). A blood smear was fixed with methanol and stained with Giemsa to perform manual relative and differential cell counts to obtain absolute counts for neutrophils, eosinophils, lymphocytes, monocytes and basophils.
Biochemical markers were determined using an InCCA® (Diconex S.A., Buenos Aires, Argentina) auto analyser. The following markers were obtained according to their value for fascioliasis diagnosis (Valero et al., Reference Valero, Bargues and Khoubbane2016):
(1) liver damage: aspartate aminotransferase (AST), gamma glutamyl transferase (or γ-glutamyl transpeptidase (GGT)), total bilirubin, alkaline phosphatase (ALP);
(2) protein metabolism: creatinine, urea;
(3) plasma proteins: albumin, globulin (Glb), albumin/globulin ratio (A/G), total protein (T-Pro);
(4) hepatic lipid metabolism: cholesterol; and
(5) muscular enzymes: creatine phosphokinase (CPK).
For the interpretation of haematological parameters and biochemical markers, there are very few published reports furnishing data useful as reference values for mules. Data available are from reports in which values were noted only when different from in horses (Greene et al., Reference Greene, Hurson and Wickler2006; McLean & Wang, Reference McLean and Wang2013; McLean et al., Reference McLean, Wang, Navas Gonzalez and Rodriguez2016). When no mule reference values were available, the results were compared to the reference values of horses.
Post-mortem liver examination and histopathology
Additionally, in two mules that died of natural cause, the necropsy was performed. Their livers were extracted, sent refrigerated to the laboratory, inspected and dissected for the classification of macroscopic lesions and the presence of flukes (the entire organ was dissected in 1-cm slices). Samples of liver tissue were taken, fixed in 10% formalin and anatomo-pathologically studied by means of histological sections stained with haematoxylin–eosin.
Results
Prevalence, intensities and egg measurements
Of the 208 mules analysed, 63 (30.3%) were positive for F. hepatica eggs. The egg quantification showed a mean value of 4.5 epg ± 8.0, with a range of 0.1–35. Of the 81 mules from Aconcagua, 32 (39.5%) were positive for F. hepatica, with a mean epg value of 3.6 ± 2.5, and a range of 0.5–8. Of the 127 mules from Uspallata, 31 (24.4%) were positive for F. hepatica, with a mean epg value of 4.7 ± 8.7, and a range of <1–35.
The difference of prevalence in both sites proved to be statistically significant (P = 0.0298) (Fisher's test). However, the difference between the epg of the two sites was not statistically significant (P = 0.5839) (Student's t-test).
An adult equine produces approximately 22.7 kg of faeces per day. The resulting estimate is that, on average, a mule may be releasing 2270–794,500 (mean 101,242) fasciolid eggs to the environment on a daily basis.
The presence of other parasites could be assessed in 36 faecal samples of F. hepatica-positive mules. A total of 32 (86.5%) were positive for strongyle eggs, with a mean value of 30.5 epg ± 37.9 and a range of 1–120. Due to the low egg counts, successful larvae culture could be performed in only two animals, in which larvae of Strongylus vulgaris and cyathostomins (small strongyles) were identified. No eggs of paramphistomids were found.
A total of 179 eggs from 63 infected mules of the two sites were measured (table 1). There were significant differences between both sites in egg length and size, but not in width and shape.
Table 1. Measurements of fasciolid eggs found in mule faeces.

Mean ± standard deviation, with range of extreme values in parenthesis; P-value from Mann–Whitney U-test.
Molecular classification and experimental infection of lymnaeid snails
The ITS-1 sequences from the ten lymnaeid specimens from the Uspallata population analysed presented the same length of 504 base pairs (bp) and a scarcely biased guanine-cytosine (GC) content of 57.5%. The nucleotide sequence was in all cases the same. An initial BLASTN search showed marked sequence similitude with G. truncatula. Consequently, the following ITS-1 sequences present in GenBank were used for comparisons: G. truncatula haplotype A (accession number AJ243018), haplotype B (AJ296270) and haplotype C (AJ272052). In the comparison alignment, the ITS-1 sequence of the Uspallata specimens proved to be identical with that of G. truncatula haplotype C from Bolivia and Chile.
The ITS-2 sequences from the same lymnaeid specimens presented a length of 401 bp and a scarcely biased GC content of 58.6%. A BLASTN analysis indicated that the sequences belonged to the species G. truncatula. Consequently, the following ITS-2 sequences present in GenBank were used for comparisons: G. truncatula haplotype 1 (AJ296271), haplotype 2 (AJ243017) and haplotype 3 (AJ272051). No one nucleotide difference appeared between the Uspallata sequence and that of haplotype 3 from the Northern Bolivian Altiplano and Chile when analysing the corresponding alignment.
Of 22 monomiracidially infected snails, eight (36.4%) became infected and shed cercariae. The total cercarial shedding capacity per snail ranged from four to 237 cercariae/snail, with a mean of 54.3 cercariae/snail and a standard deviation of 84.8. Detailed results are presented in table 2.
Table 2. Cercarial shedding by Galba truncatula specimens from Uspallata experimentally infected with one miracidium from Fasciola hepatica eggs collected in mule faeces.

Haematological parameters and biochemical markers in liver-fluke-infected mules
The values of the haematological parameters determined in 13 Fasciola-infected mules are presented in table 3. Altered values regarding reference values were shown by PCV, RBC, haemoglobin, leukocytes and lymphocytes in a high percentage of the mules. No parameter proved to be modified in every mule studied.
Table 3. Haematological parameters in 13 mules infected with Fasciola hepatica.

RR, reference ranges; SD, standard deviation; VGM, mean globular volume.
a RR according to McLean et al. (Reference McLean, Wang, Navas Gonzalez and Rodriguez2016).
b RR according to reference ranges for horses. Parameters out of the reference range in at least half of the studied mules are highlighted in bold.
c RR according to McLean & Wang (Reference McLean and Wang2013).
The values of the biochemical markers determined in the serum of 12 Fasciola-infected mules are presented in table 4. When compared to the reference ranges, pronouncedly increased values were found in mainly GGT and AST in all the mules analysed, secondarily ALP and CPK in half of them, and also creatinine in several specimens.
Table 4. Biochemical markers in mules infected with Fasciola hepatica.

RR, reference ranges; SD, standard deviation; AST, aspartate aminotransferase; IU/L, international units per litre.
a RR according to McLean et al. (Reference McLean, Wang, Navas Gonzalez and Rodriguez2016).
b RR according to reference ranges for horses. Markers out of the reference range in at least half of the studied mules are highlighted in bold.
Liver examination and histopathology
The livers of two infected mules were analysed after autopsy. A total of 20 and 97 fasciolid flukes were found. The mule infected with the larger amount of 97 flukes showed an evident out-of-condition and emaciated aspect (fig. 2). In both livers, the flukes obtained showed the typical F. hepatica form with cephalic cone, pronounced shoulders and non-parallel lateral body walls.
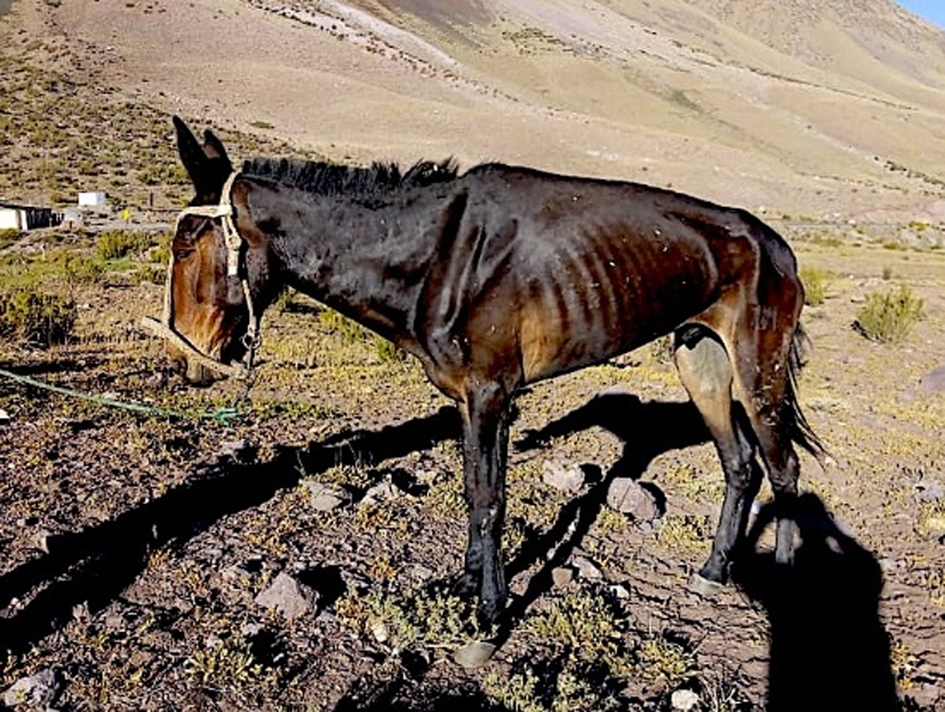
Fig. 2. Out of condition and pronouncedly emaciated mule, which proved to be massively infected by 98 fasciolid adult flukes in the post-mortem liver dissection.
The liver of the mule infected by the lower burden of 20 flukes showed no lesions or pathological changes upon macroscopic exam of the Glisson capsule or the underlying parenchyma. Resistance was perceived upon the parenchyma incisions. There was mild thickening of the bile ducts, as well as an increased bile viscosity. Thickening of the bile ducts and increased bile viscosity were observed.
The liver of the mule infected by 96 flukes in the biliary ducts showed blunt lobe borders, Glisson capsule with moderate opacity, parenchyma with multifocal subcapsular haemorrhages and firm and stringy organ consistency. A protuberance was found in the right lobe. At the dissection of this protuberance, an opaque brownish fluid was observed, and an additional immature fluke of F. hepatica immersed in the fluid was found. There was mild thickening of the bile ducts, as well as an increased bile viscosity. When performing the incisions of the parenchyma, a resistance was perceived, probably due to fibrosis.
Within the anatomo-pathological study of the liver carrying 20 adult flukes, the examination of the histological sections led to a diagnosis of mild lymphocytic hepatitis. The hepatic parenchyma showed modifications of the trabecular pattern with polyhedral hepatocytes of irregular distribution. Several of these hepatocytes showed granular cytoplasm, thickening of the nuclear membrane and increased size indicating reactive hepatocytes. The portal triads presented a scarce lymphocytic inflammatory infiltrate and oedema of the epithelium of the biliary duct. Several sections appeared covered by a thick fibro-collagenous capsule. Arterial vessels of medium size showed marked dilatation and vascular congestion.
The anatomo-pathological study of the liver with 97 flukes led to a diagnosis of chronic hepatitis, with areas of acute inflammation and pronounced interstitial fibrosis. The interlobular connective tissue was markedly thickened and forming thick tracts that delimitated smaller-sized lobules in several sectors. Hepatocytes showed an acidophilic cytoplasm and intracellular cholestasis was frequent. Portal triads presented a slight lymphocytic inflammatory infiltrate, marked fibrosis and hyperplasia of the bile ducts, some of which were dilated and with bile emboli. The sinusoids were permeable and occupied by a moderate amount of erythrocytes. Thickening of the space of Disse was frequent. There were sectors of hyperplasic fibrous tissue, with a marked mixed inflammatory infiltrate consisting of numerous neutrophils with a scarce amount of lymphocytes and eosinophils with areas that penetrated adjacent plates. There were haemorrhagic areas with irregular and poorly defined limits with aggregation of neutrophils in which karyorrhexis was frequent. There was presence of pseudocysts lined partially by a fine fibrinous leucocitary exudate with a lymphoplasmacytic inflammatory infiltrate and subjacent fibrosis. The wall was thick and formed by fibrocollagenous tissue, with some bile ducts and wide brown pigment-laden macrophage aggregates.
Discussion
Liver fluke infection in mules
This is the first fascioliasis study in which the mule is the target host. The mule shows a liver fluke infection susceptibility intermediate between donkey and horse, although closer to the latter.
Anatomo-pathological differences between the mules with low and high burden were reflected macro- and microscopically. The low-burden animal showed no macroscopic abnormalities and only mild histological liver alterations, similar to reports in horses (Nansen et al., Reference Nansen, Andersen and Hesselholt1975). The high-burden animal presented severe chronic and acute lesions. Reinfection may occur and, thus, the mules suffer seasonal exacerbations of the disease. A decrease in red blood cells and haemoglobin indicates anaemia – an effect well known in other liver-fluke-infected livestock species (Valero et al., Reference Valero, Girones, Garcia-Bodelon, Periago, Chico-Calero, Khoubbane, Fresno and Mas-Coma2008, Reference Valero, Perez-Crespo, Chillon-Marinas, Khoubbane, Quesada, Reguera-Gomez, Mas-Coma, Fresno and Girones2017) and also long since highlighted in horses (Krajl et al., Reference Krajl, Srebocvan, Marzan, Turner and Wikerhauser1960).
The decrease in leucocyte number but mainly the pronounced lymphocyte decrease indicates a strong immunosuppression. This Fasciola infection consequence has been emphasized in other animals in the chronic phase (Girones et al., Reference Girones, Valero, Garcia-Bodelon, Chico-Calero, Punzon, Fresno and Mas-Coma2007) and reinfections (Valero et al., Reference Valero, Perez-Crespo, Chillon-Marinas, Khoubbane, Quesada, Reguera-Gomez, Mas-Coma, Fresno and Girones2017). Indeed, reinfection probabilities are likely to be high in mules repeatedly using the same routes, base camps and stations.
The strongly increased values of GGT, AST and ALP indicate pronounced liver function alterations, as reported in other animal species (Valero et al., Reference Valero, Bargues and Khoubbane2016), including horses (Montes et al., Reference Montes, Zurita and Uhart1984; Soule et al., Reference Soulé, Boulard, Levieux, Barnouin and Plateau1989; Howell et al., Reference Howell, Malalana and Beesley2020). Furthermore, an increase in creatinine suggests alterations in protein metabolism, and the increase of the CPK enzyme may underlie potential effects as endocrine disorders, heart damage and skeletal muscle disease.
Such disorders, together with the anaemia and other typical chronicity effects such as lithiasis and bacteriobilia (Valero et al., Reference Valero, Santana, Morales, Hernandez and Mas-Coma2003, Reference Valero, Navarro, Garcia-Bodelon, Marcilla, Morales, Garcia, Hernandez and Mas-Coma2006), may be of severe consequences for working mules daily brought under strong efforts for weight transport in rugged mountain climbing routes and very high-altitude hypoxia. High-altitude hypoxia conditions were noted as the potential origin of a reduced egg production by the flukes (Valero et al., Reference Valero, Panova and Mas-Coma2001b) when considering that oxygen is required for egg production in F. hepatica (McGonigle & Dalton, Reference McGonigle and Dalton1995).
Haematological, biochemical and pathological findings indicate that F. hepatica may severely affect mule health. Particularly striking was the death of the high-burden mule (fig. 2). Evidence suggests reinfection, which could lead to a cumulative fluke effect, causing its death. Moreover, F. hepatica-infected animals are at a higher risk of suffering the effects of other insults to the liver, which would ultimately impact on their performance and welfare (Quigley et al., Reference Quigley, Sekiya, Egan, Wolfe, Negredo and Mulcahy2017).
Altered biochemical markers may be useful for Fasciola infection diagnosis. An indirect enzyme-linked immunosorbent assay (ELISA) test may also be used for the diagnosis in mules, similarly as in other hosts (Mas-Coma et al., Reference Mas-Coma, Bargues and Valero2014a), and also donkeys (Haridy et al., Reference Haridy, Morsy, Abdou and Morsy2007; Awad et al., Reference Awad, Ibrahim and Salib2009; Acici et al., Reference Acici, Bolukbas, Gurler, Umur and Buyuktanir2013; Quigley et al., Reference Quigley, Sekiya, Egan, Wolfe, Negredo and Mulcahy2017).
Ultimately, the easiest method of diagnosis is the consideration that fasciolids produce eggs in this host. It should be taken into account that fasciolids present different egg sizes, depending on the host species, even inside the same endemic area (Valero et al., Reference Valero, Panova and Mas-Coma2005; Mas-Coma et al., Reference Mas-Coma, Bargues and Valero2014a). Thus, in the Bolivian Altiplano, where only F. hepatica is present, fasciolid eggs shed by donkeys proved to be shorter and wider than eggs shed by sheep and cattle – donkey: 96.4–140.8/63.3–84.7 μm (mean: 125.4 ± 8.3/75.0 ± 3.7 μm); sheep: 114.8–151.2/65.5–81.4 μm (mean: 130.8 ± 7.1/72.6 ± 3.9 μm); cattle: 105.3–155.9/61.7–82.5 μm (mean: 132.0 ± 10.5/71.1 ± 4.4 μm) (Valero et al., Reference Valero, Darce, Panova and Mas-Coma2001a). The EL/EW of 82.6–155.0/49.5 106.0 μm (mean 136.0/78.2 μm) found in the Argentinian mules fits well with the aforementioned egg size in donkeys of Bolivia (Valero et al., Reference Valero, Darce, Panova and Mas-Coma2001a) and also the egg measurements of 105–147/72–90 μm (mean: 126/81 μm) from donkeys in Egypt (Badawy et al., Reference Badawy, Abouzaid and Merwad2014). Interestingly, egg size in mules also fits with the size of fasciolid eggs found in the archaeological remains of Persian onagers, old donkeys which were of a similar body size as mules (Askari et al., Reference Askari, Mas-Coma, Bouwman, Boenke, Stôllner, Aali, Rezaiian and Mowlavi2018). However, limits of egg-finding coproscopy in fascioliasis diagnosis have been emphasized in horses (Soule et al., Reference Soulé, Boulard, Levieux, Barnouin and Plateau1989), in which a copro-antigen ELISA has been seen to be useless (Palmer et al., Reference Palmer, Lyon, Palmer and Forshaw2014).
Only very low burdens of strongyles were found, as previously described in horses of the same region (Neira et al., Reference Neira, Godoy, Logarzo and Mera y Sierra2019). The extremely cold winter temperatures and very low precipitation do not favour their larval development. Concerning trematodes, F. hepatica is the sole species described so far in the province.
For the treatment of fascioliasis in mules, drugs successfully used in horses may be applied. Triclabendazole continues to be the drug of choice because it is the only one efficient against both migrating fluke juveniles and fluke adults in the bile ducts, for both animals and humans (Gandhi et al., Reference Gandhi, Schmitt, Chen, Samantray, Venishetty and Hughes2019). Triclabendazole is widely used for equines nowadays (Dorchies, Reference Dorchies2010). Other drugs used in the past were closantel (Alcaino & Aguilar, Reference Alcaino and Aguilar1985), oxyclozanide (Owen, Reference Owen1977), rafoxanide (Moisant et al., Reference Moisant, Jolivet and Pitre1972) and piperazine phloroglucinate (Guilhon & Graber, Reference Guilhon and Graber1963).
Epidemiological role of mules
The high prevalence rates of 24.4% and 39.5% found in the two mule groups and the estimated amounts of 2270–794,500 eggs/mule/day (mean 101,242 eggs/mule/day) fit well in the ranges reported from the Northern Bolivian Altiplano: 9000–808,000 eggs/day in donkeys; 800–721,800 eggs/day in sheep; 3750–840,000 eggs/day in cattle; and 2000–195,000 eggs/day in pigs (Mas-Coma et al., Reference Bargues, Mangold, Muñoz-Antoli, Pointier and Mas-Coma1997). In this Bolivian hyperendemic area, donkeys are considered one of the four important reservoir species. Interestingly, horses from the UK do not appear to be ‘high’ egg excretors (Howell et al., Reference Howell, Malalana and Beesley2020).
The very high transmission capacity of F. hepatica through G. truncatula in this very high-altitude hyperendemic area of Bolivia allows us to highlight the findings in the mules. Indeed, the routes followed by the working mules are also at very high altitude. It should be considered that the limit between high altitude and very high altitude is usually put at 3200 m asl. The life cycle of F. hepatica with G. truncatula as vector species has been proven to be enhanced under the very high-altitude conditions, with a longer cercarial shedding period and a higher metacercarial production per snail individual than in the lowlands (Mas-Coma et al., Reference Mas-Coma, Funatsu and Bargues2001; Bargues et al., Reference Bargues, Gayo, Sanchis, Artigas, Khoubbane, Birriel and Mas-Coma2017). Additionally, it should be taken into account that G. truncatula does not only play this transmitter role under Altiplanic flatland conditions, but also in other high-altitude Andean areas, such as Cajamarca (Bargues et al., Reference Bargues, Artigas, Khoubbane, Ortiz, Naquira and Mas-Coma2012), where the highest human prevalence rates in a valley endemiotope have been reported (Gonzalez et al., Reference Gonzalez, Esteban, Bargues, Valero, Ortiz, Naquira and Mas-Coma2011).
Therefore, four results obtained in the present study deserve special epidemiological importance:
(1) the field finding of Galba/Fossaria lymnaeids in the mule overwintering station of Uspallata;
(2) the molecular conclusion that the lymnaeids found in Uspallata belong to the species G. truncatula;
(3) the complete DNA sequences of rDNA ITS-1 and ITS-2 proving that the Uspallata G. truncatula population belongs to the same rDNA ITS combined haplotype 3C, which ensures the high disease transmission at the very high altitude in the Bolivian Altiplano; and
(4) the experimental demonstration that miracidia obtained from fasciolid eggs found in mule faecal samples are infective to the Uspallata G. truncatula combined haplotype 3C.
The infectivity of miracidia from mule origin, the number of cercariae/snail and the cercarial shedding period of longer than 70 days fit the enhanced transmission pattern in F. hepatica/G. truncatula at very high altitude (Mas-Coma et al., Reference Mas-Coma, Funatsu and Bargues2001). This pattern differs from that known in the lowlands (Bargues et al., Reference Bargues, Gayo, Sanchis, Artigas, Khoubbane, Birriel and Mas-Coma2017). These features, together with the prevalence rates and intensities detected, indicate that the mule is able to maintain the F. hepatica cycle independently.
Throughout human history, mule size, power and endurance have led to their increased use. The added value of mules includes their usefulness as pack animals in facilitating trade and communication over short and mainly long distances along difficult rugged mountainous routes and arid or semi-arid flatlands, as well as their capacity to replace oxen in ploughing (Mitchell, Reference Mitchell2018). Indeed, besides mainly Old World camelids and donkeys, mules participated as pack animals (Mitchell, Reference Mitchell2018) and were also included in the tribute paid to the Chinese overlords (Kim, Reference Kim2011) along the long routes of the Silk Road. This many centuries-long trade event has contributed to the spread of both F. hepatica and F. gigantica throughout Asia (Mas-Coma et al., Reference Mas-Coma, Valero and Bargues2009b).
The question is posed as to whether mules may also play a role in the transmission of F. gigantica. Indeed, there are very important mule populations in countries where this fasciolid is present, such as the People's Republic of China, which has over three million mules (ChartsBin, 2015). However, the pronounced higher physiopathogenicity of F. gigantica should be considered (Valero et al., Reference Valero, Bargues and Khoubbane2016), and indicates that appropriate studies are needed to assess such a potential role.
In the New World, horses and donkeys were introduced by the Spanish ‘conquistadores’ during the early colonial period around 500 years ago. In South America, like the donkeys that fathered them, mules were raised at lower altitudes because of the lack of suitable pastures in very high-altitude areas and the challenges that an oxygen-deficient environment posed to equine reproduction. By the 1770s, as many as 500,000 mules and donkeys were estimated to be present in Peru and Bolivia, with two million across Latin America as a whole (Mitchell, Reference Mitchell2018). By the early 1800s, donkeys and mules had largely supplanted llamas and alpacas everywhere below 3000 m asl (Cade, Reference Cade1992). On the one hand, the American camelids could not carry more than 30 kg, whereas a mule could carry three to four times this weight. On the other hand, llamas and alpacas could not be ridden and were harder to drive (Mitchell, Reference Mitchell2018). Huge numbers of mules were imported from as far as northern Argentina, totalling up to 60,000 per year, to work in the Bolivian mines in around 1610 (Flynn & Giraldez, Reference Flynn and Giraldez2002).
In Argentina, there are currently 44,000 mules (SENASA, 2016). In its Andean regions, mules have been used since colonial times (Martinez, Reference Martinez1961). The army has traditionally employed them for transport in mountainous regions and still has a mule production centre in Mendoza province (Junin & Queirolo, Reference Junin and Queirolo2018). Mules are also used by mountain-climbing expeditions to carry equipment and food to base camps.
The Aconcagua Provincial Park and Uspallata are located in Mendoza province. Mendoza city, founded in 1561, traded northward with Alto Peru (what is now Bolivia and southern Peru) and westward with Chile during the colonial period. Until commerce through the port of Buenos Aires was liberated in 1778, goods imported from Spain to Mendoza usually came either by land from Lima, or crossing the Andes from Valparaiso (Encina, Reference Encina1952). All goods travelling northward had to pass through Cordoba (Giberti, Reference Giberti1954). All commerce from the Virreynato del Río de la Plata, created in 1776, to Chile was done through Mendoza (Lacoste, Reference Lacoste2008). All land transport of goods and persons was done with pack animals, mainly mules or ox-driven carts, depending on the type of terrain – mules in the mountains and ox carts almost exclusively in the plains.
Mules were important because of their carrying capacity of up to 150 kg (Lacoste, Reference Lacoste2008) and transport capacity of up to 60 km/day (Farro, Reference Farro2008). Mules were bred in the lowlands in Buenos Aires and Santa Fe provinces, fattened in Cordoba and Salta and sold in Peru (Lacoste, Reference Lacoste2008). From Salta, more than 30,000 mules were exported to Peru around 1778 and 1779 (Serrera, Reference Serrera1992). The crossing of the Andes was done exclusively with mules, with up to 25,000 making the journey between Mendoza and Chile, as was also the case for most of the commerce between Argentina and Bolivia.
In summary, Mendoza province was a crucial trade crossroad for more than two centuries, and the mule played an important role as a pack animal in exchanges with neighbouring countries. Thus, mules could contribute to the spread of both F. hepatica and G. truncatula combined haplotype 3C to areas where human fascioliasis-endemic areas have been described in Chile (Apt et al., Reference Apt, Aguilera and Vega1993; Artigas et al., Reference Artigas, Bargues, Mera y Sierra, Agramunt and Mas-Coma2011) and Bolivia (Mas-Coma et al., Reference Mas-Coma, Angles, Esteban, Bargues, Buchon, Franken and Strauss1999, Reference Mas-Coma, Funatsu and Bargues2001; Bargues et al., Reference Bargues, Artigas, Angles, Osca, Duran, Buchon, Gonzales-Pomar, Pinto-Mendieta and Mas-Coma2020). All this may also help in historically understanding why Cordoba is by far the province in which more human fascioliasis cases have been reported (Mera y Sierra et al., Reference Mera y Sierra, Agramunt, Cuervo and Mas-Coma2011).
Acknowledgements
Technical support was provided by the Servicio Central de Secuenciación para la Investigación Experimental (SCSIE) of the Universidad de Valencia (Dr A. Martínez). Studies of this article have been performed within the framework of the Worldwide Initiative of WHO against Human Fascioliasis (WHO Headquarters, Geneva, Switzerland).
Financial support
Studies in Argentina were funded by the Fondo para la Investigación Científica y Tecnológica (FONCYT) of the Agencia Nacional de Promoción Científica y Tecnológica (ANPCyT), Argentina (project number PICT-2017-1361); by the Secretaría de Ciencia y Técnica, Universidad Juan Agustín Maza, Mendoza, Argentina (project numbers 1163/18 and 576/18); and by Beca Interna Doctoral, Consejo Nacional de Investigaciones Científicas y Técnicas, República Argentina. Spanish collaboration was funded by the Program Innovación para el Desarrollo of the Agencia Española de Cooperación Internacional para el Desarrollo (AECID), Ministry of Foreign Affairs and Cooperation, Madrid, Spain (project number 2017/ACDE/001583); by the International Atomic Energy Agency (Animal Production and Health Section, Joint FAO/IAEA Division of Nuclear Techniques in Food and Agriculture, Department of Nuclear Sciences and Applications, IAEA Headquarters Vienna, Austria) (project number RLA5049); by Subprograma Estatal de Generación de Conocimiento de la Acción Estratégica en Salud (AES) y Fondos FEDER, Plan Estatal de Investigación Científica y Técnica y de Innovación, ISCIII-MINECO, Madrid, Spain (Health Research project number PI16/00520); by the Red de Investigación de Centros de Enfermedades Tropicales – RICET (project number RD16/0027/0023 of the National Program on I + D + I, ISCIII-Subdirección General de Redes y Centros de Investigación Cooperativa RETICS), Ministry of Health and Consumption, Madrid; by the PROMETEO Program, Programa of Ayudas para Grupos de Investigación de Excelencia, Generalitat Valenciana, Valencia, Spain (project number 2016/099); and by the V Convocatoria de Proyectos de Cooperación al Desarrollo of the Universidad de Valencia de 2016, Valencia, Spain (project number 2017/01).
Conflicts of interest
None.
Ethical standards
Ethics guidelines regarding animal care were strictly adhered. The study was performed in accordance with the ethical standards as laid down in the Declaration of Helsinki of 1975 and revised in 2008. Ethics approval was granted by the Institutional Animal Care and Use Committee (Comité Institucional de Cuidado y Uso de Animales en Investigación y Docencia) of Universidad Juan Agustín Maza, Mendoza (protocol number 154). Permission for animal research in the Valencia centre was obtained from the Servicio de Sanidad y Bienestar Animal, Dirección General de Producción Agraria y Ganadería, Consellería de Presidencia y Agricultura, Pesca, Alimentación y Agua, Generalitat Valenciana, Valencia, Spain (number 2015/VSC/PEA/00001).