1. Introduction
Dolostones often form high-quality hydrocarbon reservoirs, containing more than half the hydrocarbons in carbonate rocks worldwide. In North America, up to 80% of carbonate-hosted hydrocarbons are hosted in dolostones (Zenger & Dunham, 1980; Sun, Reference Sun1995). In China, carbonate-hosted hydrocarbons are concentrated almost entirely in dolostones (Zheng et al. Reference Zheng, Wu, Wu, Zhang and Liu2007, Reference Zheng, Shi, Luo, Li and Li2008; Huang et al. Reference Huang, Chen, Ren and Bao2012 b). Studying the genesis of dolostones can therefore help predict the distribution of dolostone reservoirs and reduce the risk and cost of hydrocarbon exploration.
As early as 1779, the Italian scholar Arduinovon investigated the genesis of dolostones in the Tyrolean Alps in southern Italy. He reported that the dolostones were generated from the in situ reaction between limestone and magnesium-rich hydrothermal fluid (Morlot, Reference Morlot, Haidinger and Haidinger1847). In the middle of and in the late twentieth century, many research studies on dolostone were conducted worldwide and several dolostone formation mechanisms were proposed. These included the seepage reflux model (Adams & Rhodes, Reference Adams and Rhodes1960; Deffeyes et al. Reference Deffeyes, Lucia, Weyl, Pray and Murry1965, p. 71–88), sabkha model (Friedman & Sanders, Reference Friedman and Sanders1967; Hsu & Siegenthaler, Reference Hsu and Siegenthaler1969), burial model (Illing, Reference Illing1959; Kohout et al. Reference Kohout, Henry, Banks, Smith and Griffin1977, p. 1–34; Oliver, Reference Oliver1986; Tóth, Reference Tóth, Back, Rosenhein and Saeber1988; Wilson et al. Reference Wilson, Hardie and Phillips1990; Garven, Reference Garven2003), mixing zone model (Land, Reference Land1972; Badiozamani, Reference Badiozamani1973), hydrothermal dolomitization (Anderson & Garven, Reference Anderson and Garven1987; Cervato, Reference Cervato1990; Mountjoy & Halim-Dihardja, Reference Mountjoy and Halim-Dihardja1991; Davies & Smith, Reference Davies and Smith2011), and microbial/organogenic model (Vasconcelos & McKenzie, Reference Vasconcelos and McKenzie1997; Burns et al. Reference Burns, McKenzie and Vasconcelos2000; Mazzullo, Reference Mazzullo2000; Kenward et al. Reference Kenward, Goldstein, Brookfield, González and Roberts2012). Other scholars have comprehensively reviewed the genesis of dolostones (Zenger & Hardie, Reference Zenger and Hardie1987; Machel, Reference Machel2004; Zhang et al. Reference Zhang, Hu and Zhang2006; Wang et al. Reference Wang, Zhou, Chen, Hao, Peng, Wang, Jiang and Xie2013).
In the early 1990s, as the hydrocarbon exploration focus in China shifted from continental facies to marine facies, dolostones gradually received more attention from Chinese scholars. Among these dolostones, the middle Permian dolostones in the western region of the Yangtze platform are among the most important rock units for hydrocarbon exploration in Chinese marine carbonates. A series of studies were conducted on the genesis of these dolostones, and various dolostone formation mechanisms have been proposed including the mixing zone model (Zhang, Reference Zhang1982; Song, Reference Song1985; Chen, Reference Chen1989), burial model (He & Feng, Reference He and Feng1996; Wang & Jin, Reference Wang and Jin1997; Jiang et al. Reference Jiang, Hu, Wang, Wang, Li and Zhai2014), basalt leaching dolomitization model (Jin & Feng, Reference Jin and Feng1999), structurally controlled hydrothermal dolomitization model (Reference Huang, Li, Huang, Lan, Lv and WangHuang et al. 2012a ) and hydrothermal dolomitization model (Wang et al. Reference Wang, Shen, Huang, Shi, Li, Yuan and Yang2014). However, these previously proposed dolostone formation mechanisms cannot reasonably explain the distribution and geochemical characteristics of the middle Permian dolostones in the western region of the Yangtze platform.
We therefore propose a new dolomitization model: open thermal convection dolomitization. Unlike existing thermal convection models, the dolomitization model proposed here is related to the mantle plume and the scale of dolomitization is very large. In addition, the distribution of dolostones in this thermal convection model is controlled by sedimentary facies and the dolomitization is selective. The proposed model not only explains the characteristics and distribution of dolostones in the study area, but also serves as a reference to predict the distribution of dolostones in other areas subjected to thermal convection.
2. Geological setting
East Yunnan constitutes the western region of the Yangtze platform. With an area of 1.8 × 105 km2, East Yunnan is located in Yunnan Province on the southeastern side of the Longmenshan fault zone and the northeastern side of the Ailaoshan–Honghe fault zone (Ministry of Geology and Mineral Resources of PR China, 1982, p. 1–3) (Fig. 1). East Yunnan’s Permian strata are mainly exposed in its northeastern part (Fig. 1).

Fig. 1. Geological map of East Yunnan overlain by the upper Permian Emei basalt and middle Permian dolostones. Asterisk: data estimated by combining various types of data (as a result of complex geological conditions, including challenging access, it was difficult to precisely measure the thicknesses of dolomitized rocks (e.g. massive dolostone, patchy dolostone and patchy limestone)).
The Permian trisection scheme was used here as a stratigraphic scheme (Li et al. Reference Li, Li, Song, Song and Yang2005). Lower Permian strata are generally absent in East Yunnan. The middle Permian strata can be divided into the Liangshan Formation (oldest), Qixia Formation and Maokou Formation (youngest). The Liangshan Formation unconformably overlies Carboniferous strata, and conformably contacts the overlying Qixia Formation. The Maokou Formation conformably contacts the underlying Qixia Formation and unconformably contacts the overlying upper Permian strata (Fig. 2). Note that the middle Permian strata of the Yangtze platform corresponds to the Guadalupian and Kungurian of the international stratigraphic standard (Fig. 2) (Li et al. Reference Li, Li, Song, Song and Yang2005), which corresponds to the period 284–259 Ma (http://www.stratigraphy.org/index.php/ics-chart-timescale).

Fig. 2. Permian stratigraphic subdivisions in East Yunnan. The unconformities are represented by dotted lines.
The Liangshan Formation is 2–20 m thick and contains marine and terrigenous facies, including carbonaceous shale mixed with mudstone, siltstone and limestone lenses (Ministry of Geology and Mineral Resources of PR China, 1982, p. 164; Jin & Feng, Reference Jin and Feng1999).
The Qixia Formation is 50–200 m thick and comprises medium- to thin-bedded carbonates: dark-grey to grey bioclastic packstone, bioclastic wackestone and dolostone. The palaeowater depth is interpreted to have been relatively deep (Ministry of Geology and Mineral Resources of PR China, 1982, p. 164–165; Jin & Feng, Reference Jin and Feng1999).
The Maokou Formation is 100–500 m thick and is dominated by thick-bedded to massive, grey to light-grey bioclastic grainstone, dolostone and bioclastic packstone. The palaeowater depth is interpreted to have been relatively shallow (Ministry of Geology and Mineral Resources of PR China, 1982, p. 165; Jin & Feng, Reference Jin and Feng1999). The Maokou Formation, which hosts most of the middle Permian dolostone in East Yunnan, has experienced substantial dolomitization during its geological history.
During the early middle Permian period, East Yunnan and the entire Upper Yangtze platform began to settle and transgression occurred. This transgression initiated the deposition of the Liangshan Formation, which extended over existing Carboniferous, Devonian and older strata. During the development of the Qixia and Maokou formations, the main environments of East Yunnan existed in those rocks as open-platform facies (Jin & Feng, Reference Jin and Feng1999; Chen et al. Reference Chen, Zhang, Huang and Hou2011).
During the late middle Permian period, the Dongwu movement, which is the rapid differential crustal uplift caused by the rising of the Emei mantle plume, affected southern China (Reference He, Xu, Wang and XiaoHe et al. 2005b ). As a result of the activity of the Emei mantle plume, the whole Yangtze platform was uplifted, resulting in a regional unconformity between middle Permian and upper Permian rocks (Reference He, Xu, Wang and XiaoHe et al. 2005b ). Simultaneously, a large-scale basic magmatic eruption occurred in the western Yangtze platform, forming thick Emei basalt (64–5386 m thick) (Ministry of Geology and Mineral Resources of PR China, 1982, p. 165). According to previous research results, the active area of the Emei mantle plume can be divided into an inner belt, a middle belt and an outer belt from west to east (He et al. Reference He, Xu, Xiao, Wang and Shao2003, Reference He, Xu, Wang, Luo and Wang2005a ; Luo et al. Reference Luo, Sun, Han and Wang2012). From the inner belt to the outer belt, the activity intensity of the Emei mantle plume gradually weakened. This weakening produced a corresponding and gradual reduction in the thicknesses of denudation of the Maokou Formation and the erupted Emei basalt (Fig. 1). During this stage, East Yunnan was dominated by extensional movement; basement faults were reactivated and tensional faults, strike-slip faults and fracture systems developed (Luo et al. Reference Luo, Jin, Zhu and Zhao1988; Liu & Zhu, Reference Liu and Zhu2009).
In the early late Permian period, transgression occurred again in East Yunnan, and upper Permian rock deposition commenced. The crustal uplift and basaltic eruption caused by Emei mantle plume activity significantly altered the depositional pattern of East Yunnan. From west to east, fluvial–alluvial plain facies, tidal flat-lagoon facies and carbonate platform facies were developed (Fig. 3) (Jin & Feng, Reference Jin and Feng1999; Chen et al. Reference Chen, Zhang, Huang and Hou2011).

Fig. 3. Palaeogeography of the upper Permian strata in East Yunnan (modified from Feng et al. Reference Feng, Yang, Jin, He, Ww, Xin, Bao and Tan1996; Chen et al. Reference Chen, Zhang, Huang and Hou2011).
Notably, the geological conditions in the study area are complex, with Permian strata being poorly exposed because of local limestone mining and small dip angles of the strata, mostly between 3 and 30° (Ministry of Geology and Mineral Resources of PR China, 1982, p. 175). In addition, the perennial rain of the region has caused significant erosion and weathering of the strata, adding difficulties to this research. By combining various petrological and geochemical methods, we attempt to make the best use of existing data to analyse the formation mechanism of these middle Permian dolostones and propose a reasonable and reliable dolomitization model.
3. Methods
The middle Permian samples studied here were collected from the Hunshuitang, Shuanglongcun, Xiaohuangpo, Fenglecun, Xishan, Shizong, Shilin, Shawan, Guanyinqiao, Xujiapo, Zhijin, Miaotian, Langdai and Panxian sections. Well Z11 and Well W22 also provided samples. The first seven sections are located in East Yunnan; the remaining seven sections and the wells are situated in Sichuan and Guizhou provinces, which are geographically close to East Yunnan. A total of 217 outcrop samples and 12 well samples were studied.
The strata thickness data of outcrop sections were obtained in two ways: (1) actual measurement by using measuring tape, geological compass, global positioning system (GPS) receiver and geological map for 10 outcrops (without an asterisk in Fig. 1); (2) estimated by combining GPS data, strata attitude data, geological map and photograph for four outcrops (with an asterisk in Fig. 1). The strata thickness data of well profiles were provided by Sinopec Group and China National Petroleum Corporation.
Petrographic studies on the 610 uncovered thin-sections (thickness, 30 μm) were performed using polarizing microscopy. A total of 562 thin-sections were stained with Alizarin red S (0.1 g Alizarin red S per 100 mL 0.2% hydrochloric acid) and 48 thin-sections were stained with Alizarin red S and potassium ferricyanide (0.12 g Alizarin red S and 0.8 g potassium ferricyanide per 100 mL 1.5% hydrochloric acid).
Cathodoluminescence (CL) observations were performed on 93 polished thin-sections at the State Key Laboratory of Geological Processes and Mineral Resources of the China University of Geosciences (Beijing) using a CITL CL8200 MK5 optical CL System (Cambridge Image Technology Limited, Welwyn Garden City, UK) operating at 12 kV and 300 μA, with an exposure time of 2 s. All samples were subjected to the same test conditions to facilitate the comparison of results.
Order degree values of the dolostones were measured for 30 powdered samples at the State Key Laboratory of Petroleum Resources and Prospecting of the China University of Petroleum (Beijing) using a Bruker AXS D2 Phaser (Bruker AXS GMBH, Karlsruhe, Germany). This procedure applied the computing method of Fuchtbauer (Reference Fuchtbauer1974) to data collected using Cu Kα radiation at a 2θ of 4.5–50°, with a step size of 0.02° and scan time of 0.5 s for each step.
Fluid inclusion analysis was conducted on 10 thin-sections (33 inclusions) of 80 μm thickness at the Analytical Laboratory of the Beijing Research Institute of Uranium Geology using a Linkam THMSG600 heating–freezing stage (Linkam Scientific Instruments, Tadworth, UK) coupled with an Olympus polarizing microscope (Olympus Corporation, Tokyo, Japan), using the standard method described by Goldstein & Reynolds (Reference Goldstein and Reynolds1994). The temperature error was ± 1°C.
Carbon and oxygen isotopic compositions of the dolostones were measured for 25 powdered samples (particle size, ≤ 74 μm) at the Nanjing Institute of Geology and Paleontology of the Chinese Academy of Sciences using a Finigan MAT 253 mass spectrometer with a Kiel IV Carbonate Device (Thermo Fisher Scientific, Waltham, Massachusetts, USA). Phosphoric acid of 100% purity was used in this study. The reaction temperature was 50.0 ± 0.1°C and the reaction time was 36 hours. Each sample was measured three times and the calibration standard was Chinese carbonate standard GBW-04405 (δ13CPDB value of 0.57 ± 0.03‰ and δ18OPDB value of −8.49 ± 0.14‰). The standard deviations of δ13CPDB and δ18OPDB were 0.04‰ and 0.08‰, respectively. The oxygen isotope composition of dolomite was calculated using the fractionation factor reported by Rosenbaum & Sheppard (Reference Rosenbaum and Sheppard1986). The isotopic ratios for carbon and oxygen were expressed as δ13C and δ18O per mil (‰) relative to the Vienna Pee Dee Belemnite (VPDB) standard.
Strontium isotopic compositions of the dolostones were measured for 10 powdered samples (particle size, ≤ 74 μm) at the Analytical Laboratory of the Beijing Research Institute of Uranium Geology, using a Phoenix thermal ionization mass spectrometer (Isotopx Ltd, Cheshire, UK). The acid used in this study is HCl (0.8 mol L–1), and the dissolution time was 2 hours. Isolation and purification of strontium were performed via ion exchange chromatography using AG 50 W-X8H+ resin (Bio-Rad, Hercules, California, USA). The standardized 86Sr/88Sr ratio was 0.1194, and the calibration standard was NBS-987 (87Sr/86Sr value of 0.71034 ± 0.00026). The experimental error on this spectrometer was 0.000011−0.000026.
Magnesium isotopic compositions of the dolostones were measured for seven powdered samples at the Radioisotope Laboratory of the University of Queensland using a Nu Plasma HR multi-collector inductively coupled plasma mass spectrometer (MC-ICP-MS) (Nu Instruments, Wrexham, UK). This procedure employed a cooling gas flow rate of 16 L min–1, an auxiliary gas flow rate of 0.8 L min–1, a sample carrier gas flow rate of 1.1 L min–1, an extraction voltage of −2000 V, a background 24Mg value of 7 mV and a 24Mg signal intensity of 32 V (μg/g)–1. Mg isotopic results are reported in standard δ-notation in per mil relative to DSM3 (Galy et al. Reference Galy, Yoffe, Janney, Williams and Carignan2003).
4. Results
4.a. Distribution characteristics of dolostones
Stratigraphically, the middle Permian dolostones of East Yunnan are mainly found in the Maokou Formation. In Xishan and Shuanglongcun outcrops, the Qixia Formation also contains some dolostones (Fig. 4). The dolomitized rocks of the Maokou and Qixia formations account for an average of 92.58% and 7.42% of the middle Permian dolomitized rocks, respectively. The average dolomitized proportions of the rocks of the Maokou and Qixia formations are 54.14% and 19.66%, respectively. The non-dolomitized strata comprise mainly dark-grey to grey bioclastic packstone (Fig. 4).

Fig. 4. Stratigraphic correlation of middle Permian sections in East Yunnan. The top surface of the middle Permian strata is flattened, and the section line is shown in Figure 5. 1, bioclastic grainstone; 2, bioclastic packstone; 3, patchy limestone with vertically elongated dolomite patches; 4, patchy limestone with parallel-elongated dolomite patches; 5, patchy limestone, containing grid-shaped dolomite patches; 6, patchy limestone, containing irregularly shaped dolomite patches; 7, patchy dolostone with vertically elongated dolomite patches; 8, patchy dolostone, containing grid-shaped dolomite patches; 9, dolostone (pure); 10, basalt; and 11, mudstone/shale.
Note that the thickness of dolomitized rocks gradually decreases from the inner belt to the outer belt of the active area of the Emei mantle plume, and the dolomitization was not strictly controlled by faults (Fig. 5).

Fig. 5. Thickness map of the middle Permian dolomitized rocks in East Yunnan overlain by the upper Permian fault patterns (Luo et al. Reference Luo, Jin, Zhu and Zhao1988, Reference Luo, Sun, Han and Wang2012). Asterisk: as in Figure 1.
4.b. Petrographic characteristics of dolostones
The middle Permian dolostones in East Yunnan can be divided into two types based on their occurrence in single beds: massive dolostones (complete dolomitization, with dolomite content of 90–100%); and patchy dolostones (incomplete dolomitization, with dolomite content of 50–90%).
Massive dolostones are dark grey with no residual limestone in single bed, and some have slash marks. Vertically, the beds of massive dolostone often alternate with the beds of limestone (mainly bioclastic packstone) (Figs 4, 6).

Fig. 6. Macroscopic characteristics of massive dolostones. (a) Dark-grey massive dolostone (indicated by red arrows) interbedded with grey limestone (indicated by yellow arrows) (Maokou Formation, Panxian). (b) Dark-grey massive dolostone (indicated by red arrow) interbedded with grey limestone (indicated by yellow arrow) (Maokou Formation, Shilin). (c) Dark-grey massive dolostone interbedded with grey limestone (Maokou Formation, Xiaohuangpo). (d) Dark-grey massive dolostone with slash marks resulting from differential weathering (Machel, Reference Machel2004) (Maokou Formation, Fenglecun). (e) Dark-grey massive dolostone interbedded with grey limestone (Maokou Formation, Shizong). (f) Dark-grey massive dolostone interbedded with grey limestone (Maokou Formation, Shuanglongcun).
Patchy dolostones are grey or dark grey and contain dolomite and limestone patches (metasomatic remnants); dolomite patches constitute more than 50% of the rock volume, and the limestone patches are mainly bioclastic packstones. When the dolomite patch content falls below 50%, the patchy dolostones turn into patchy limestones (Fig. 7).

Fig. 7. Macroscopic characteristics of patchy dolostones. (a) Grey patchy dolostone, in which dolomite patches exhibit various shapes. The stratigraphic dip is 27° (Maokou Formation, Xishan). (b) Grey patchy dolostone, in which dolomite patches show darker colour (indicated by red arrow). The stratigraphic dip is 12° (Maokou Formation, Shuanglongcun). (c) Grey patchy limestone with parallel-elongated dolomite patches. The stratigraphic dip is 12° (Maokou Formation, Shuanglongcun). (d) Grey patchy limestone, in which dolomite patches show darker colour. The stratigraphic dip is 18° (Maokou Formation, Xiaohuangpo). (e) The inner structure of patchy dolostone, in which dolomite patches are grid-shaped. The stratigraphic dip is 12° (Maokou Formation, Shuanglongcun). (f) Dark-grey patchy dolostones (top) transitioning to grey patchy limestones (bottom). Dolomite patches are darker in colour. The stratigraphic dip is 12° (Maokou Formation, Shuanglongcun). (g) Grey patchy dolostones with vertically elongated dolomite patches. The stratigraphic dip is 12° (Maokou Formation, Shuanglongcun). (h) Grey patchy dolostones with vertically elongated dolomite patches (indicated by red arrow). The stratigraphic dip is 12° (Maokou Formation, Shuanglongcun). (i) The top surface of a patchy dolostone with vertically elongated dolomite patches (indicated by red arrow). The stratigraphic dip is 10° (Maokou Formation, Shilin).
Dolomite patches vary in size from 0.5 cm to more than 1.5 m and exhibit various shapes (Fig. 7). Patchy dolostones/limestones can be divided into four types according to the patch shape: vertically elongated dolomite patches (i.e. dolomite patches perpendicular to the bedding planes); parallel-elongated dolomite patches (i.e. dolomite patches parallel to the bedding planes); grid-shaped dolomite patches; and irregularly shaped dolomite patches (Fig. 8).
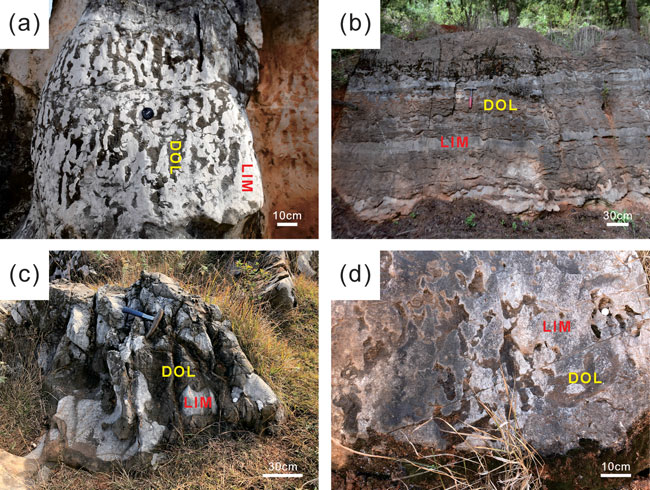
Fig. 8. Types of patchy dolostones/limestones. (a) Grey patchy limestones with vertically elongated dolomite patches. The stratigraphic dip is 10° (Maokou Formation, Shilin). (b) Grey patchy dolostones with parallel-elongated dolomite patches. The stratigraphic dip is 10° (Maokou Formation, Shilin). (c) Grey patchy dolostones, containing grid-shaped dolomite patches. The stratigraphic dip is 12° (Maokou Formation, Shuanglongcun). (d) Grey patchy limestones, containing irregularly shaped dolomite patches. The stratigraphic dip is 11° (Maokou Formation, Fenglecun).
Notably, the massive dolostones and patchy dolostones show similar microstructural characteristics (i.e. crystal size distribution, crystal size, crystal shape and CL) and geochemical characteristics (Table 1), with the only difference being the degree of dolomitization.
Table 1. Geochemical characteristics (average values) of massive and patchy dolostones

The crystal size distribution of the dolostones in the study area is mainly unimodal, and the crystals mainly comprise fine-grained (100–250 μm), medium-grained (250–500 μm) and coarse-grained (500–1000 μm) dolomite. The dolomite crystal shapes are mainly planar-subhedral and nonplanar-anhedral (Fig. 9a). Saddle dolomites with a ladder-like curved crystal face can be observed in a small number of samples (Fig. 9b).

Fig. 9. Microscopic features of dolostones. (a) Dolostone with medium- to coarse-sized crystals. The crystal shapes are mainly planar-subhedral and nonplanar-anhedral; plane-polarized light (PPL) (Maokou Formation, Zhijin). (b) Saddle dolomite with medium-sized crystals. The crystal face is indicated by the arrow; the red colour indicates calcite. PPL (Maokou Formation, Shizong). (c) Dolostone in which original microstructural features from the limestone precursor are preserved. The crystals mainly contain fine-grained dolomites; PPL (Maokou Formation, Langdai). (d) Dolostone in which original microstructural (echinoderm) features from the limestone precursor are preserved. The crystals mainly contain fine- to medium-grained dolomites; PPL (Maokou Formation, Fenglecun). (e) Dolomites were scattered relatively evenly among the calcites, but were enriched near the stylolites; PPL (Maokou Formation, Miaotian). (f) Enlarged image delineated by the yellow box in (e), in which the dolomites are cut by stylolites (indicated by red arrows); PPL. (g) Dolomites developed preferentially in bioclastic grainstone areas of the rock. The crystals mainly contain fine-grained dolomites; PPL (Maokou Formation, Xishan). (h) Dolomites developed preferentially in areas with relatively low micrite matrix content. The crystals mainly contain fine-grained dolomites; PPL (Maokou Formation, Shilin). (i) Dolomites developed preferentially in areas with relatively low micrite matrix content. The crystals mainly contain medium- to coarse-grained dolomites; PPL (Maokou Formation, Hunshuitang). (j) Dolomites developed preferentially in intergranular areas. The crystals mainly contain medium-grained dolomites; PPL (Maokou Formation, Shizong). (k) Dolomites developed preferentially in intergranular pores and intragranular pores (indicated by yellow arrow). Crescent-shaped calcite cements centred in the grain contact area (indicated by red line). The crystals mainly contain medium- to coarse-grained dolomites; PPL (Maokou Formation, Zhijin). (l) Dolomites developed preferentially in intergranular and intragranular pores (indicated by yellow arrow). The crystals mainly contain medium- to coarse-grained dolomites; PPL (Maokou Formation, Langdai).
In the study area, the ferroan dolomite is rare. In some samples, the dolomite preserves its original microstructural features from the limestone precursor (Fig. 9c, d). In some samples, scattered dolomites are relatively evenly distributed among calcites, but more enrichment is observed near stylolites (Fig. 9e), and the dolomites are cut by stylolites (Fig. 9f). For patchy dolostones, the contact between dolomite patches and limestone patches is sharp; within the dolomite patches, the dolomite crystal size and shape showed no significant change (Fig. 9g, h).
Notably, the dolomites of some samples are mainly concentrated in two types of sites: (1) areas of relatively low micrite matrix content (Fig. 9g–i); and (2) intergranular and intragranular pores (Fig. 9j–l). In some samples, crescent-shaped calcite cements were centred in grain contact areas and other calcite cements were found between dolomites and calcite grains (Fig. 9k, l).
4.c. Cathodoluminescence analysis
The general CL characteristics of the different dolomites in the study area are consistent, including weak CL intensity and relatively uniform CL colour, with the only subtle difference in specific CL colour such as dark red and deep purple. In contrast, the calcites did not exhibit luminescence (Fig. 10).

Fig. 10. CL of dolomite. (a) Dolostone of fine crystal size. The crystal shapes are mainly planar-subhedral and nonplanar-anhedral; PPL (Maokou Formation, Hunshuitang). (b) CL image corresponding to (a), in which the dolomites show weak CL. (c) Dolostone of fine to medium crystal size. The crystal shapes are mainly planar-subhedral. Saddle dolomites can be observed with warped crystal faces (indicated by arrow); PPL (Maokou Formation, Xiaohuangpo). (d) CL image corresponding to (c), in which the dolomite shows weak CL and the calcite does not show CL. (e) Dolostone of fine to medium crystal size. The crystal shapes are mainly planar-subhedral; PPL (Maokou Formation, Xiaohuangpo). (f) CL image corresponding to (e). (g) Dolostone of fine to medium crystal size. The crystal shapes are mainly planar-subhedral; PPL (Maokou Formation, Xiaohuangpo). (h) CL image corresponding to (g). (i) Dolostone of fine to medium crystal size. The crystal shapes are mainly planar-subhedral; PPL (Maokou Formation, Hunshuitang). (j) CL image corresponding to (i). (k) Dolostone of fine crystal size. The crystal shapes are mainly planar-subhedral and nonplanar-anhedral; PPL (Maokou Formation, Shuanglongcun). (l) CL image corresponding to (k). (m) Dolostone of powder to fine crystal size. The crystal shapes are mainly planar-euhedral and planar-subhedral; PPL (Maokou Formation, Shuanglongcun). (n) CL image corresponding to (m). (o) Dolostone of fine to medium crystal size. The crystal shapes are mainly planar-subhedral; PPL (Maokou Formation, Shuanglongcun). (p) CL image corresponding to (o). The dolomite crystal boundaries suffering from dissolution show brighter luminescence in CL (indicated by arrow). (q) Dolostone of fine to medium crystal size. The crystal shapes are mainly planar-subhedral and nonplanar-anhedral; PPL (Maokou Formation, Fenglecun). (r) CL image corresponding to (q). (s) Dolostone of fine to medium crystals. The crystal shapes are mainly planar-subhedral; PPL (Maokou Formation, Fenglecun). (t) CL image corresponding to (s).
The dolomite crystals exhibit zonal structure, in which the crystal cores are mainly characterized by uniformly dark red (Fig. 10b, d, f, h, j, r, t) and deep purple (Fig. 10l, n, p) in CL, and the crystal edges (growth zones) show a stronger luminescence with brighter colour and less thickness (Fig. 10b, d, j, l, n, p, t).
In some samples, such as a sample of the Maokou Formation in Xiaohuangpo, dolomite crystals of different sizes show similar luminescence in CL (Fig. 10f). In other samples, such as those from the Maokou Formation in Xiaohuangpo and in Fenglecun, the CL intensities of the dolomites were generally weak but differed slightly in dolomites of different sizes; dolomites in which the original structure was preserved (echinoderms) exhibited relatively strong CL (Fig. 10h, t). Generally, brighter luminescence occurs in cleavage slits (Fig. 10t) and at crystal boundaries suffering from dissolution (Fig. 10p).
4.d. Order degree of dolomite
The order degree values for the dolomite crystals in the study area range from 0.7240 to 0.9976, with an average of 0.8892. Maokou Formation dolomite crystals and Qixia Formation dolomite crystals exhibit similar characteristics (Table 2).
Table 2. Order degree of middle Permian dolomite crystals in East Yunnan

4.e. Fluid inclusion analysis
Primary fluid inclusions of useful size for microthermometry (i.e. > 2 μm in diameter; Goldstein & Reynolds, Reference Goldstein and Reynolds1994) were scattered throughout the dolomite crystals, and neither stretching nor directionality was observed. These inclusions were two-phase inclusions, liquid-rich with a vapour bubble, colourless and transparent with clear boundaries and regular shapes. The inclusions ranged in size from 2.5 to 9 μm with an average of 4.7 μm (Fig. 11).

Fig. 11. Fluid inclusion features and histograms of homogenization temperatures and fluid inclusion salinities for middle Permian dolostones of East Yunnan. (b) Enlarged image delineated by the yellow box in (a). Fluid inclusions are indicated by red circles.
Homogenization temperatures of the fluid inclusions within dolostones range from 101 to 192°C (average, 169.52°C). The homogenization temperatures are mainly concentrated in the range of 164–185°C. Salinities of fluid inclusions within dolostones range from 4.49 to 8.41 wt% NaCl eq (average, 5.50 wt% NaCl eq). The salinities of fluid inclusions are mainly concentrated in the range of 4.49–5.59 wt% NaCl eq (Fig. 11).
Note that compared with patchy dolostones, massive dolostones have higher homogenization temperatures and salinities of fluid inclusions (Table 1; Fig. 12). Additionally, there is a slight linear relation between the salinity and homogenization temperature of fluid inclusions, such that the salinity increases with the temperature (Fig. 12).

Fig. 12. Scatter diagram of homogenization temperatures and fluid inclusion salinities for middle Permian dolostones of East Yunnan.
Fluid inclusions within Maokou Formation dolostones and Qixia Formation dolostones exhibit similar characteristics (Table 3).
Table 3. Homogenization temperatures and fluid inclusion salinities for middle Permian dolostones of East Yunnan

4.f. Carbon and oxygen isotope analysis
δ13CPDB values for dolomites in the study area range from 2.24 to 5.27‰ with an average of 3.82‰. The δ18OPDB values range from −6.31 to −13.75‰, with an average of −9.43‰ (Fig. 13). Note that, compared with patchy dolostones, massive dolostones have lower δ18OPDB values (Table 1; Fig. 13). The Maokou Formation dolostones and Qixia Formation dolostones show similar δ13CPDB and δ18OPDB characteristics (Table 4).

Fig. 13. Carbon and oxygen isotope data for middle Permian dolostones in East Yunnan. The dashed-line box represents the δ13CPDB and δ18OPDB ranges for the middle Permian low-Mg calcitic shells (Veizer et al. Reference Veizer, Ala, Azmy, Bruckschen, Buhl, Bruhn, Garden, Diener, Ebneth, Godderis, Jasper, Korte, Pawellek, Podlaha and Strauss1999).
Table 4. Carbon and oxygen isotope data for middle Permian dolostones in East Yunnan

4.g. Strontium isotope analysis
87Sr/86Sr values for dolomites in the study area range from 0.707354 to 0.708522 with an average of 0.707841. A total of five datasets of 87Sr/86Sr values for Permian seawater were collected to compare with those of the dolostones in East Yunnan. The first three datasets were obtained from the Yangtze platform (Lu et al. Reference Lu, Cui, Yang and Zhang1992; Huang, Reference Huang1997; Huang et al. Reference Huang, Shi, Zhang, Shen, Liu and Wu2001), and the remaining data were obtained from elsewhere in the world (McArthur et al. Reference Mc Arthur, Howarth and Bailey2001; Korte et al. Reference Korte, Jasper, Kozur and Veizer2006). Notably, these five datasets show similar 87Sr/86Sr ranges for the Permian seawater. However, the 87Sr/86Sr values for middle Permian seawater from the first three datasets (Yangtze platform) are higher than those from the latter two datasets (Table 5).
Table 5. Comparison of strontium isotope data between middle Permian dolostones and Permian seawater
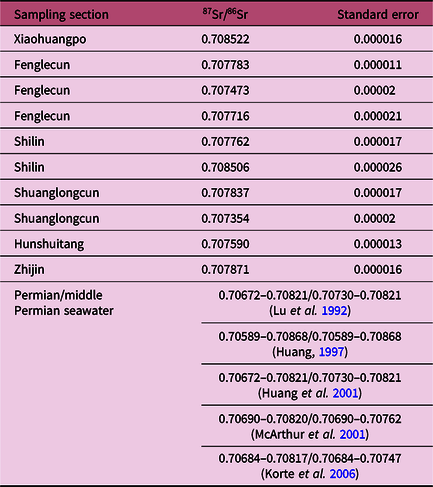
4.h. Magnesium isotope analysis
δ26MgDSM3 values for dolomites in the study area range from −0.91 to −2.12‰, with an average of −1.74‰. Notably, the δ26MgDSM3 values for dolomites in the study area gradually decrease from the inner belt to the outer belt of the active area of the Emei mantle plume (Fig. 14; Table 6).

Fig. 14. Magnesium isotopic composition (δ26MgDSM3) of different Mg sources (Geske et al. Reference Geske, Goldstein, Mavromatis, Richter, Buhl, Kluge, John and Immenhauser2015). 1, Magnesium isotope data for middle Permian dolostones in East Yunnan. 2, δ26MgDSM3 values calculated from the fractionation formula for dolomite precipitation (Li et al. Reference Li, Beard, Li, Xu and Johnson2015). 3, Average value of different Mg sources (Geske et al. Reference Geske, Goldstein, Mavromatis, Richter, Buhl, Kluge, John and Immenhauser2015).
Table 6. Magnesium isotope data for middle Permian dolostones in East Yunnan

5. Discussion
5.a. Characteristics of dolomitizing fluid
The massive dolostones and patchy dolostones are of the same origin, with the only difference being the degree of dolomitization (Table 1). The homogenization temperatures and salinities of fluid inclusions within the dolostones, the petrographic characteristics of the dolostones, and the carbon and oxygen isotopic characteristics of the dolostones all indicate that the dolomitization fluid was of high temperature and moderate salinity.
The homogenization temperature of the fluid inclusions within the dolostones is generally greater than 101°C (Fig. 11), indicating that the dolomitization fluid was a high-temperature fluid that broke through the dynamic barrier to form the dolomite (Warthmann et al. Reference Warthmann, Van lith, Vasconcelos, McKenzie and Karpoff2000; Wright & Wacey, Reference Wright, Wacey, Braithwaite, Rizzi and Darke2004).
The salinities of the fluid inclusions within the dolostones range from 4.49 to 8.41 wt% NaCl eq, with an average of 5.50 wt% NaCl eq (Fig. 11), indicating that the dolomitization fluid was of moderate salinity.
Petrography reveals the dolomite crystals to be fine to coarse sized. The crystal shapes of the dolostones are mainly planar-subhedral, nonplanar-anhedral and nonplanar-saddle (Fig. 9); these shapes also indicate that the dolomites were formed by relatively high-temperature fluids (Shukla et al. Reference Shukla, Gregg and Sibley1984; Machel, Reference Machel2004).
δ18OPDB values of the dolomites are negative (Fig. 13), which is mainly attributed to the high-temperature effect (Vasconcelos et al. Reference Vasconcelos, McKenzie, Warthmann and Bernasconi2005; Sharp, Reference Sharp2007), consistent with the analysis of the fluid inclusions.
In addition, δ13CPDB values of the dolomites are high, mostly above 3‰. These values are similar to the carbon isotope signatures of other marine carbonates deposited during the same period (Fig. 13) (Huang, Reference Huang1997; Veizer et al. Reference Veizer, Ala, Azmy, Bruckschen, Buhl, Bruhn, Garden, Diener, Ebneth, Godderis, Jasper, Korte, Pawellek, Podlaha and Strauss1999; Huang & Zhou, 2010). This similarity indicates that the carbon in the dolomites mainly originates from the dissolution of local calcite and/or aragonite. The high δ13CPDB values are attributed to the high-speed burial of organic matter during this period (Huang, Reference Huang1997).
Calcite in the study area is non-luminescent in CL, indicating that it is the primary marine carbonate mineral with low manganese, with the diagenetic fluid possibly being seawater. The ferroan dolomite in the study area is rare and the dolomite shows weak CL, indicating that the dolostone was less affected by non-marine fluids and formed in reducing environments (Machel, Reference Machel1985; Huang, Reference Huang1992; Gillhaus et al. Reference Gillhaus, Richter, Meijer, Neuser and Stephan2001) (Fig. 10). The dolomite crystals are characterized by zonal structures, indicating that they have undergone multi-stage diagenesis of at least two stages (Fig. 10). The initial dolomites (crystal cores) formed by replacement are characterized by a relatively uniform CL colour, indicating that the diagenetic environment in the early stage was relatively stable; the latter dolomite cements (crystal edges) demonstrate a stronger luminescence with a brighter CL colour and a lesser thickness, indicating that the dolomitization fluid composition and dolomite crystallization rate varied in the latter stage, while the action time was relatively short (Fig. 10).
In some samples (Fig. 10f) dolomite crystals of different sizes exhibit the same luminescence in CL, indicating that their dolomitization fluids were the same. In contrast, in other samples (Fig. 10h, t) dolomite crystals of different sizes exhibit slightly different luminescence intensity in CL, with dolomites that preserved the original structure (echinoderm) showing relatively strong CL. This is not due to different dolomitizing fluids, but because the original mineral phases of dolomites are different (Huang, Reference Huang1990). The brighter luminescence in cleavage slits and crystal boundaries suffering from dissolution suggests that late-stage fluids interacted with the dolomite and that their properties were different from the early dolomitization fluids (Fig. 10p, t).
5.b. Origin of dolomitizing fluid
On the basis of the above, the dolomitizing fluid in the study area should have the following characteristics: (1) high temperature; (2) moderate salinity; (3) abundant in Mg2+; and (4) a long-term, sustainable and active hydrological driving mechanism. This last expectation is based on the region’s large geographic and volumetric scale of dolostone, requiring a substantial quantity of dolomitizing fluid.
Currently, the main formation mechanisms of dolostone include the microbial/organogenic model, sabkha model, seepage reflux model, mixing zone model, hydrothermal dolomitization, compaction model, tectonic (squeegee) model, topography-driven model and thermal convection model (Machel, Reference Machel2004).
The crystals of the dolostones in the study area ranged from fine to coarse (Fig. 9), which is much larger than that predicted by the microbial/organogenic model (typically < 10 μm) (Vasconcelos & McKenzie, Reference Vasconcelos and McKenzie1997; Warthmann et al. Reference Warthmann, Van lith, Vasconcelos, McKenzie and Karpoff2000; Hu et al. Reference Hu, Zhu, Wang, You and He2014). The origin of the dolomitizing fluid therefore cannot be explained by the microbial/organogenic model.
The order degree of dolomite is relatively high in the study area (Table 2). Compared with previous research (Huang, Reference Huang1985; Zeng & Wan, Reference Zeng and Wan2004, Zhang et al. Reference Zhang, Shou, Zhang, Pan and Zhou2014), the order degree of dolomite in the study area is significantly higher than that predicted by the sabkha model, seepage reflux model or mixing zone model (mean value, 0.59–0.67). In addition, the dolomitizing fluid in the study area had a high temperature; the origin of the dolomitizing fluid therefore cannot be explained by the sabkha, seepage reflux or mixing zone models (Zenger & Dunham, 1980; Machel, Reference Machel2004).
A hydrothermal model would seem to better fit these order degrees and temperature expectations. However, the dolostones of the study area rarely exhibit typical characteristics of hydrothermal dolostone, such as: zebra texture (in which dark matrix dolomite bands alternate with bands of white saddle dolomite) (Machel, Reference Machel2004); milky white colour with a pearly luster in hand specimens; intercrystalline holes that are filled with hydrothermal minerals; or being strictly controlled by basement faults (Machel, Reference Machel2004). Additionally, the salinities of fluid inclusions within dolostones in the study area are significantly lower than those of the hydrothermal fluid in the study area (15–24 wt% NaCl) (Li et al. Reference Li, Mao and Zhang2011; Hou et al. Reference Hou, Wang, Zhang, Wang, Liu, Deng, Pei and Yang2013). The dolomitizing fluid was therefore not a deep hydrothermal fluid, and the dolostone in the study area does not belong to this class of structurally controlled hydrothermal dolostone.
Burial compaction (compaction model) and tectonically induced flow (tectonic (squeegee) model) can generate only limited amounts of dolostone; the former involves a limited volume of compaction water and the latter involves a short-lived squeegee-type flow system with low flux (Machel & Anderson, Reference Machel and Anderson1989; Deming et al. Reference Deming, Nunn and Evans1990; Amthor et al. Reference Amthor, Mountjoy and Machel1993; Machel et al. Reference Machel, Cavell, Buschkuehle and Michael2000; Buschkuehle & Machel, Reference Buschkuehle and Machel2002; Machel, Reference Machel2004). The origin of the dolomitizing fluid therefore cannot be explained by these two models.
The dolomitizing fluid of the topography-driven model is meteoric water, which dissolves enough Mg2+ en route before encountering limestone (Tóth, Reference Tóth, Back, Rosenhein and Saeber1988; Machel, Reference Machel2004). Although the Emei basalt covering the Maokou Formation is rich in Mg2+ (4.5–6.51%) (Meade, Reference Meade2010), causing some researchers to hypothesize that the Mg2+ of the dolomitizing fluid originated from this basalt (Jin & Feng, Reference Jin and Feng1999), the 87Sr/86Sr values for the dolomites in the study area are significantly higher than those of the Emei basalt (87Sr/86Sr value, 0.7045–0.7065) (Mao, Reference Mao1991; Jiang et al. Reference Jiang, Hu, Wang, Wang, Li and Zhai2014). The topography-driven model therefore cannot explain the origin of the dolomitizing fluid.
We believe that the dolomitization in the study area must be modelled as an open thermal convection mechanism (Raffensperger & Vlassopoulos, Reference Raffensperger and Vlassopoulos1999). Thermal convection would be driven by spatial variations in temperature caused by elevated heat flux in the vicinity of the Emei mantle plume. This convection would result in changes in pore-water density and thus produce an effective hydraulic head (Machel, Reference Machel2004). Open convection cells would form in carbonate platforms that were open to seawater recharge and discharge at the top. The dolomitizing fluid would be mostly seawater, with a minor contribution from deep hydrothermal fluid driven by the Emei mantle plume. The evidence for this hypothesis is discussed in the following.
First, the difference between the 87Sr/86Sr values for middle Permian seawater and the Yangtze platform and elsewhere in the world (Table 5) can be explained using the influence of the Yunnan movement that occurred at the end of the Carboniferous Period (Zhou et al. Reference Zhou, Chen, Wang, Jin, Tang, Wang and Liang2005; Su et al. Reference Su, Jiang, Chen, Wang, Jiang, Bian, Feng and Wu2015). As a result of the Yunnan movement that occurred in southern China during the latter part of the Carboniferous Period, the Yangtze platform was uplifted and suffered strong denudation, resulting in a regional unconformity between middle Permian and Carboniferous rocks and the absence of lower Permian strata in the Yangtze platform. At this time, the amount of exposed land area drastically expanded, and more crustal-source Sr (rich in 87Sr) accumulated in strata (Zhou et al. Reference Zhou, Chen, Wang, Jin, Tang, Wang and Liang2005; Chen et al. Reference Chen, Zhang, Huang and Hou2011; Su et al. Reference Su, Jiang, Chen, Wang, Jiang, Bian, Feng and Wu2015). During the early part of the middle Permian, the transgression occurred, and this crustal-source Sr was released from rocks and introduced into the ocean, causing the middle Permian seawater of the Yangtze platform and the carbonate rocks formed during this period to have higher 87Sr/86Sr values.
Most of the 87Sr/86Sr values for the dolomites in the study area were therefore within the ranges for Permian or middle Permian seawater in the Yangtze platform (Table 5). This similarity suggests that the Permian seawater of the Yangtze platform (rich in Mg2+) is the primary candidate for the dolomitizing fluid in the study area.
Only two dolomite data points exceed the 87Sr/86Sr range for Permian seawater (Table 5), which is assumed to be influenced by some external factors such as deep hydrothermal fluids passing through the aluminosilicate basement rocks.
Second, the carbonate platform in the study area was underlain by a mantle plume, which can result in particularly fast and pervasive dolomitization and would have provided a lasting and active hydrologic driving mechanism.
Third, the high temperature of the dolomitizing fluid can be explained by the activity of the Emei mantle plume. Based on their burial history and geothermal features (Wang et al. Reference Wang, Huang, Huang and Wang1986; Yuan et al. Reference Yuan, Ma, Hu, Guo and Fu2006; Wo et al. Reference Wo, Zhou and Xiao2007; Huang et al. Reference Huang, Lan, Huang and Lv2014; Jiang et al. Reference Jiang, Hu, Wang, Wang, Li and Zhai2014; Tian et al. Reference Tian, Lin, Zhang, Peng, Yang, Luo and Xu2014; Hao et al. Reference Hao, Liu and Xu2015), the dolostones in the study area were clearly affected during dolomitization by high-temperature thermal events, likely related to the Emei mantle plume.
Fourth, the moderate salinity of the dolomitizing fluid was likely to the result of the mixing of seawater and deep hydrothermal fluid driven by the Emei mantle plume during thermal convection. The Permian seawater of the Yangtze platform, which has a low salinity of 4.4 wt% NaCl (Chen et al. Reference Chen, Zhao, Gao, Liu and Zhou2013), is the primary candidate for the dolomitizing fluid; the hydrothermal fluid, which has a high salinity of 15–24 wt% NaCl (Li et al. Reference Li, Mao and Zhang2011; Hou et al. Reference Hou, Wang, Zhang, Wang, Liu, Deng, Pei and Yang2013) is the secondary candidate. In some areas, the proportion of hydrothermal fluid could be higher, so that the saddle dolomites can be formed (but the overall number is very low). The salinity slightly increased linearly with the homogenization temperature for these fluid inclusions (Fig. 12). This is probably because the temperature and hydrothermal component of the dolomitizing fluids is higher in regions showing more intense plume activity, such that the salinity of the fluids is also higher.
Fifth, the dolomitization of the study area was selective. From a macroscopic perspective, the dolostones are mainly distributed in the Maokou Formation, which is dominated by bioclastic grainstone. Further, the Qixia Formation, which is dominated by bioclastic packstone, does not exhibit obvious dolomitization (Fig. 4). From a microcosmic perspective, dolomitization appears to have been initiated at sites with relatively low micrite matrix contents (i.e. high primary porosity and permeability), as shown in Figure 9g–i, and at sites of intergranular pores and intragranular pores, which are mainly primary pores as shown in Figure 9j–l. In some samples, crescent-shaped calcite cements were centred in the grain contact areas (Fig. 9k); these were likely formed when the limestone experienced a freshwater vadose environment (James & Choquette, Reference James and Choquette1984). Furthermore, in some samples, such as that shown in Figure 9k, calcite cements were found between dolomites and calcite grains. All of these characteristics indicate that the limestone was buried (though not very deep) and underwent diagenesis before dolomitization (see details in Section 5.c). If the limestone was not buried before dolomitization, the bioclastic packstone would have a relatively high primary porosity and permeability similar to that in bioclastic grainstone (i.e. the difference is not obvious). If the limestone was buried deeply before dolomitization, the porosity and permeability of the bioclastic grainstone and bioclastic packstone would be very low as a result of diagenesis (Longman, Reference Longman1980). In both cases, the dolomitization of the study area would not be selective. Before dolomitization, the porosity and permeability of the bioclastic packstone were therefore low, whereas those of the bioclastic grainstone were high enough to allow thermal convection.
From the inner belt to the outer belt of the active area of the Emei mantle plume, the temperature gradient would have gradually decreased, causing thermal convection to gradually weaken such that the thickness of dolostone (i.e. dolomitized rocks in that carbonate sequence) would gradually have decreased (Fig. 5). In addition, compared with patchy dolostones, massive dolostones (which indicate relatively strong thermal convection) have higher average homogenization temperatures and fluid inclusions with greater salinities and lower δ18OPDB average values (Table 1; Figs 12, 13); these observations can also be explained by the open thermal convection model.
Sixth, the distribution and morphological characteristics of East Yunnan dolostones can be explained by the open thermal convection model (see details in Section 5.d). The predictions of this model can also be used to effectively interpret the CL characteristics of East Yunnan dolostones.
Seventh, during the active stage of the Emei mantle plume during the late middle Permian – early late Permian period, the entire Yangtze platform was uplifted; we therefore believe that the palaeogeography of the upper Permian strata reflects the differences in the degree of crustal uplift (i.e. the differences in the intensity of the Emei mantle plume activity). For instance, the terrestrial sedimentary settings indicate relatively strong activity of the Emei mantle plume, whereas the marine sedimentary settings indicate relatively weak Emei mantle plume activity (Fig. 3).
The thickness of dolomitized rocks closely correlates with the activity intensity of the Emei mantle plume (Fig. 5). It is therefore also likely that the thickness of dolomitized rocks has an indirect relation with the palaeogeography of the upper Permian strata. The palaeogeography of the upper Permian strata may therefore indicate that the dolomitization in this area is related to the mantle plume.
Eighth, a previous study concluded that dolomites lack typical δ26Mg signatures characterizing their specific environment of dolomitization precipitation (Geske et al. Reference Geske, Goldstein, Mavromatis, Richter, Buhl, Kluge, John and Immenhauser2015). In addition, δ26Mg values for Permian seawater are lacking and no related literature exists for reference. Our δ26Mg data are therefore difficult to utilize when interpreting the origin of dolomitizing fluid. The δ26MgDSM3 values of the dolomites gradually decrease from the inner belt to the outer belt of the active area of the Emei mantle plume (Fig. 14; Table 6), presumably because the influence of upper mantle fluids decreases away from the plume. If anything, this δ26MgDSM3 pattern also indicates that the dolomitization in this area is likely to be related to the mantle plume and mantle-driven fluids.
5.c. Age of dolomitization
The dolostones in the study area were clearly affected during dolomitization by high-temperature thermal events related to the Emei mantle plume (Wang et al. Reference Wang, Huang, Huang and Wang1986; Yuan et al. Reference Yuan, Ma, Hu, Guo and Fu2006; Wo et al. Reference Wo, Zhou and Xiao2007; Huang et al. Reference Huang, Lan, Huang and Lv2014; Jiang et al. Reference Jiang, Hu, Wang, Wang, Li and Zhai2014; Tian et al. Reference Tian, Lin, Zhang, Peng, Yang, Luo and Xu2014; Hao et al. Reference Hao, Liu and Xu2015). The age of dolomitization therefore cannot be estimated from the homogenization temperature of the fluid inclusions. The high-temperature thermal events related to the Emei mantle plume began during the late middle Permian period. The dolomitization should therefore be of late middle Permian age.
The dolomitization model in the study area is a thermal convection model, with selective dolomitization. During dolomitization, the porosity and permeability of strata (bioclastic grainstone) were still high enough to sustain viable thermal convection cells. Previous studies have found that open thermal convection is active to a burial depth of about 2–3 km, provided that the sequence does not contain effective aquitards. Below 2–3 km of burial depth, compaction has reduced porosity and permeability to levels that are too low to sustain viable convection cells (Machel, Reference Machel2004). This mechanism therefore indicates an estimated dolostone burial depth of no more than 2–3 km.
In some microscopic samples, scattered dolomites were relatively evenly distributed among the calcites but enriched near the stylolites (Fig. 9e), and the dolomites were cut by stylolites (Fig. 9f). It could be that the pressure solution caused the calcites to dissolve, resulting in the enrichment of dolomites (which are not easy to dissolve) near the stylolites (Wang et al. Reference Wang, Gao, Li, Ge and Zhang2017). These phenomena indicate that the dolomitization appeared before the pressure solution; the burial depth of dolostones is therefore no more than 600–1000 m (Lind, Reference Lind, Berger, Kroenke and Mayer1993; Fabricius, Reference Fabricius, Leckie, Sigurdson, Acton and Draper2000, p. 177–190; Machel, Reference Machel2004).
The middle Permian strata of East Yunnan is 150–700 m thick (Ministry of Geology and Mineral Resources of PR China, 1982, p. 164–165), meeting the burial depth requirements described above. In conclusion, the dolomitization in the study area should have occurred between the end of the middle Permian period at 259 Ma, and the initial basalt eruption period at 257 Ma (after the eruption, basalt covered the middle Permian rocks such that the carbonate platforms could not open to seawater) (Guo et al. Reference Guo, Fan, Wang and Li2004; He et al. Reference He, Xu, Huang, Luo, Shi, Yang and Yu2007; Liu & Zhu, Reference Liu and Zhu2009; Li et al. Reference Li, Gu, Wen, Han, Sheng, Xx, Cao, Wu and Zou2012; http://www.stratigraphy.org/index.php/ics-chart-timescale).
5.d. Migration characteristics of dolomitizing fluid
5.d.1. Migration pathway
Previous studies have concluded that the magnitude and distribution of permeability are the most important parameters governing flow and dolomitization in an open thermal convection model (Kohout et al. Reference Kohout, Henry, Banks, Smith and Griffin1977, p. 1–34; Simms, Reference Simms1984; Kaufman, Reference Kaufman1994; Sanford et al. Reference Sanford, Whitaker, Smart and Jones1998; Wilson et al. Reference Wilson, Sanford, Whitaker and Smart2001; Whitaker et al. Reference Whitaker, Wilson, Sanford, Smart, Rizzi, Darke and Braithwaite2002, Reference Whitaker, Jones and Smart2003, Reference Whitaker, Smart, Jones, Braithwaite, Rizzi and Darke2004, p. 99–139). As stated above, open thermal convection (Kohout convection) is active to a depth of about 2–3 km, providing that the sequence does not contain effective aquitards.
In the middle Permian limestone strata of East Yunnan, pores in bioclastic grainstone are the most important pathways for fluid migration. The distribution and microscopic characteristics of these dolostones (Figs 4, 9) indicate that dolostone development was closely related to the sedimentary setting: the host rocks of the dolostones are mainly bioclastic grainstone (shoal facies). Bioclastic grainstone has high porosity and permeability, which can provide pathways for fluid migration. The Maokou Formation contains the strongest development of middle Permian shoal facies; dolostones are therefore mainly distributed in the Maokou Formation.
In addition, the faults and fractures that formed during the Dongwu movement are also important migration pathways. They connect different shoal bodies such that the dolomitizing fluid can travel longer distances not only horizontally, but also vertically from one shoal body to another.
5.d.2. Driving force for migration
The open thermal convection of the study area was driven by spatial variations in temperature. These variations, caused by elevated heat flux in the vicinity of the Emei mantle plume, resulted in changes in pore-water density and thus produced an effective hydraulic head (Machel, Reference Machel2004).
As a result of the influence of abnormally high temperatures resulting from Emei mantle plume activity, convective fluxes substantially increased, resulting in particularly fast and pervasive dolomitization (Combarnous & Bories, Reference Combarnous and Bories1975; Wood & Hewett, Reference Wood and Hewett1982; Phillips, Reference Phillips1991).
5.d.3. Migration direction
By integrating all observed characteristics of the dolostone in the study area, particularly macroscopic characteristics, this study interprets the following circulation flow pathways for the dolomitizing fluid (Fig. 15).
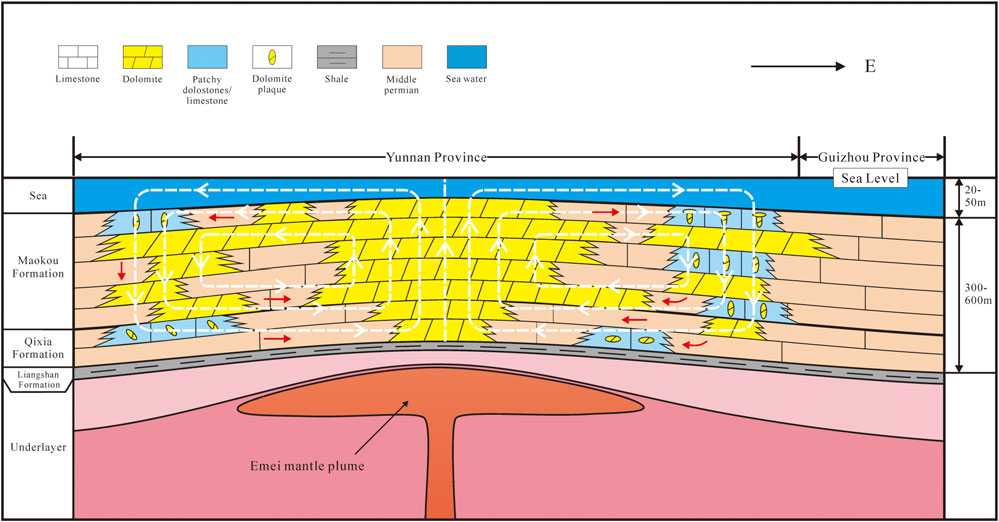
Fig. 15. Dolomitization model during the middle Permian period in East Yunnan. White dashed lines represent thermal convection flow pathways. Red solid lines represent flow pathways controlled by faults and fracture systems formed during the Dongwu movement.
In the horizontal plane, the driving force for thermal convection decreased away from the Emei mantle plume. The thickness of dolomitized rocks therefore gradually decreases from the inner belt to the outer belt of the active area of the Emei mantle plume (Fig. 5).
Vertically, the shape characteristics of dolomite patches suggest the direction of fluid migration (Figs 7, 8). Patchy dolostone/limestone with vertically elongated dolomite patches mainly exists in the vertical-flow region of thermal convection (i.e. when the direction of fluid was perpendicular to the bed plane). Patchy dolostone/limestone with parallel-elongated dolomite patches mainly occurs in the horizontal-flow region of thermal convection (i.e. when the direction of fluid was parallel to the bed plane). Patchy dolostone/limestone with grid-shaped dolomite patches or irregularly shaped dolomite patches mostly exists in the curved-flow region of thermal convection (i.e. when the direction of fluid was oblique to the bed plane). The mudstone of the Liangshan Formation would have prevented the fluid from migrating downwards (Fig. 15).
5.e. Establishment of dolomitization model
We propose a mantle-plume-induced dolomitization model: open thermal convection dolomitization (Fig. 15). In this model, the Mg2+ of dolomitizing fluids mostly originates from seawater, with a minor component from deep hydrothermal fluid. The fluid moves along circulation flow pathways driven by spatial variations in temperature, resulting in large-scale dolomitization.
The dolomitizing fluid mainly migrates through the pores in bioclastic grainstone; the depositional setting of the dolostone is therefore as expected, that is, mainly shoal facies. The Maokou Formation in the study area is dominated by bioclastic grainstone and the water is relatively shallow; it is therefore the main locus for dolomitization in the middle Permian strata of East Yunnan.
This model explains the characteristics and distribution of dolostones in the study area; it can also be used to predict dolostone distributions in other areas subjected to thermal convection.
6. Conclusions
The main conclusions of this study are as follows.
-
1. The formation mechanism for the middle Permian dolostones in East Yunnan is open thermal convection dolomitization. Mg2+ in dolomitizing fluids predominantly originated from seawater, with a minor contribution from deep hydrothermal fluid.
-
2. The dolomitizing fluid mainly migrates through pores in bioclastic grainstone (shoal facies) and faults and fracture systems. Driven by spatial variations in temperature (due to elevated heat flux in the vicinity of the Emei mantle plume), the fluid migrates along the circulation flow pathways, resulting in fast and pervasive dolomitization of Middle Permian limestone.
-
3. The dolostones that have undergone open thermal convection dolomitization are mainly distributed in the Maokou Formation, which primarily contains shoal facies.
Finally, it is necessary to note that the dolomitization model proposed in this study is a concise and idealized model with a single hotspot (Emei mantle plume). However, the actual geological conditions of East Yunnan (700 km in diameter) must be much more complex than those of the proposed model. There could be some small secondary hotspots in the active area of the Emei mantle plume, which can also affect the composition and migration of the dolomitizing fluid. The elucidation of the specific impacts of these small secondary hotspots on dolomitization requires more experimental data and further studies in the future.
Acknowledgements
This research project was funded by the China National Petroleum Corporation (grant no. XNS14KS2016-040), the Sinopec Group (grant no. 34450000-15-ZC0607-0015) and the National Natural Science Foundation of China (grant no. 41872108). We thank the reviewer Theodore Present (Caltech) and an anonymous reviewer, whose useful suggestions and constructive criticisms helped to improve the manuscript. We thank Shuo Yi for her help with sample analysis. We also thank Enago for assistance with language.
Conflict of interest
None.