Introduction
Marine fisheries provide food, jobs and profit to a considerable portion of humanity. However, it is well documented that after attaining a maximum in the 1990s, marine fishery landings decreased (Pauly & Zeller, Reference Pauly and Zeller2016) or, at best, stabilized (FAO, 2020), stressing the necessity of assessment and management targeting the sustainability of fish stocks. In contrast with other fish resources, landings of cephalopods showed a growing trend in the last decades, caused by increasing demand (Caddy & Rodhouse, Reference Caddy and Rodhouse1998; Doubleday et al., Reference Doubleday, Prowse, Arkhipkin, Pierce, Semmens, Steer, Leporati, Lourenço, Quetglas, Sauer and Gillanders2016; Sauer et al., Reference Sauer, Gleadall, Downey-Breedt, Doubleday, Gillespie, Haimovici, Ibáñez, Katugin, Leporati, Lipinski, Markaida, Ramos, Rosa, Villanueva, Arguelles, Briceño, Carrasco, Che, Chen, Cisneros, Conners, Crespi-Abril, Kulik, Drobyazin, Emery, Fernández-Álvarez, Furuya, González, Gough, Krishnan, Kumar, Leite, Lu, Mohamed, Nabhitabhata, Noro, Petchkamnerd, Putra, Rocliffe, Sajikumar, Sakaguchi, Samuel, Sasikumar, Wada, Zheng, Tian, Pang, Yamrungrueng and Pecl2020).
A sustainable management of cephalopods is challenged by their unique life history, such as short lifespans and semelparous reproduction, high natural mortality rates, rapid and often non-asymptotic growth (Arkhipkin et al., Reference Arkhipkin, Hendrickson, Payá, Pierce, Roa-Ureta, Robin and Winter2020). Furthermore, for most octopods, fisheries statistics by species are scarce (Sauer et al., Reference Sauer, Gleadall, Downey-Breedt, Doubleday, Gillespie, Haimovici, Ibáñez, Katugin, Leporati, Lipinski, Markaida, Ramos, Rosa, Villanueva, Arguelles, Briceño, Carrasco, Che, Chen, Cisneros, Conners, Crespi-Abril, Kulik, Drobyazin, Emery, Fernández-Álvarez, Furuya, González, Gough, Krishnan, Kumar, Leite, Lu, Mohamed, Nabhitabhata, Noro, Petchkamnerd, Putra, Rocliffe, Sajikumar, Sakaguchi, Samuel, Sasikumar, Wada, Zheng, Tian, Pang, Yamrungrueng and Pecl2020), and the level of fishing effort that octopod stocks can withstand is difficult to assess due to our limited knowledge of their life cycle and population dynamics, including their growth patterns (Leporati et al., Reference Leporati, Semmens and Pecl2008).
Although many captivity studies have shown that coastal benthic octopods are short-living and fast-growing organisms, little is known about their population structure and growth in their natural environment (Semmens et al., Reference Semmens, Pecl, Villanueva, Jouffre, Sobrino, Wood and Rigby2004; Arkhipkin et al., Reference Arkhipkin, Bizikov, Doubleday, Laptikhovsky, Lishchenko, Perales-Raya and Hollyman2018). Environmental studies on population structure of benthic octopods are challenging because they spend most of the time in dens or bury themselves (Mather, Reference Mather1988; Leite et al., Reference Leite, Haimovici and Mather2009a); and many species perform ontogenetic migrations between depth ranges to ensure females find suitable habitat to brood their eggs (Ortiz et al., Reference Ortiz, Ré, Marquez and Glembocki2011; Lima et al., Reference Lima, Leite, Haimovici and Oliveira2014a; Rodhouse et al., Reference Rodhouse, Pierce, Nichols, Sauer, Arkhipkin, Laptikhovsky, Lipinski, Ramos, Gras, Kidokoro, Sadayasu, Pereira, Lefkaditou, Pita, Gasalla, Haimovici, Sakai and Downey2014). Regarding growth, although some attempts have been made to follow up marked octopods in their environment for short periods (Oosthuizen, Reference Oosthuizen2003), most studies in nature relied on age determination from hard structures such as stylets and beaks, for which the periodicity of bands, rings or microincrement formation was investigated and, in some cases, validated (Arkhipkin et al., Reference Arkhipkin, Bizikov, Doubleday, Laptikhovsky, Lishchenko, Perales-Raya and Hollyman2018). For example, age determinations from stylets were validated for Octopus pallidus, a holobenthic octopus that spawns eggs and has benthic hatchlings (Doubleday et al., Reference Doubleday, Semmens, Pecl and Jackson2006), and for meroplanktonic octopuses with a hatchling pelagic phase, such as Octopus aff. tetricus (Leporati et al., Reference Leporati, Hart, Larsen, Franken and De Graaf2015), and Octopus vulgaris (Hermosilla et al., Reference Hermosilla, Rocha, Fiorito, González and Guerra2010), but not for Octopus cyaena (Herwig et al., Reference Herwig, Depczynski, Roberts, Semmens, Gagliano and Heyward2012). Age determinations from beaks were initially proposed by Raya & Hernández-González (Reference Raya and Hernández-González1998) and later validated for O. vulgaris paralarvae in captivity (Hernández-López et al., Reference Hernández-López, Castro-Hernández and Hernández-García2001), and by marking (Canali et al., Reference Canali, Ponte, Belcari, Rocha and Fiorito2011; Perales-Raya et al., Reference Perales-Raya, Almansa, Bartolomé, Felipe, Iglesias, Sánchez and Rodríguez2014).
Studies on the population dynamics of tropical coastal octopuses are scarce because they are mostly targeted by small-scale fishers and often sold locally, for which reliable landing statistics are rarely available (Sauer et al., Reference Sauer, Gleadall, Downey-Breedt, Doubleday, Gillespie, Haimovici, Ibáñez, Katugin, Leporati, Lipinski, Markaida, Ramos, Rosa, Villanueva, Arguelles, Briceño, Carrasco, Che, Chen, Cisneros, Conners, Crespi-Abril, Kulik, Drobyazin, Emery, Fernández-Álvarez, Furuya, González, Gough, Krishnan, Kumar, Leite, Lu, Mohamed, Nabhitabhata, Noro, Petchkamnerd, Putra, Rocliffe, Sajikumar, Sakaguchi, Samuel, Sasikumar, Wada, Zheng, Tian, Pang, Yamrungrueng and Pecl2020). Octopus insularis (Leite et al., Reference Leite, Haimovici, Molina and Warnke2008), can be included in this category as it is captured with hand hooks in shallow waters of the Veracruz Reef System in the south-western Gulf of Mexico (González-Gómez et al., Reference González-Gómez, de los Angeles Barriga-Sosa, Pliego-Cárdenas, Jiménez-Badillo, Markaida, Meiners-Mandujano and Morillo-Velarde2018, Reference González-Gómez, Meiners-Mandujano, Morillo-Velarde, Jiménez-Badillo and Markaida2020); also in the Caribbean Sea, as bycatch in small-scale fishing along the coast and islands of Colombia (Díaz et al., Reference Díaz, Ardila and Gracia2000; Guerrero-Kommritz & Camelo-Guarin, Reference Guerrero-Kommritz and Camelo-Guarin2015). From north-eastern to central Brazil O. insularis is caught during low tide over flat coastal reefs in shallow waters with gaffs or handhold hooks, and by free-dive and small-scale lobster fishermen as bycatch or alternative target during the closures, using artisanal pots (Leite et al., Reference Leite, Haimovici, Molina and Warnke2008; Martins et al., Reference Martins, Souto and Schiavetti2012; Haimovici et al., Reference Haimovici, Leite, Marinho, Batista, Madrid, Oliveira, Lima, Candice, Haimovici, Andriguetto and Sunye2014; Lopes et al., Reference Lopes, Andrade, Pennino and Leite2021). Since 2005, O. insularis is also targeted by mid-scale fishing industrial boats geared with pot longlines based in Itarema, Ceará State (Braga et al., Reference Braga, Marinho, Batista and Rocha2007) (Figure 1). In the 2000s, recorded annual landings attained over 500 tonnes, not including the widespread gaff fishing at low tide. Although more recent landing statistics are lacking, fishing intensified along Rio Grande do Norte State in the last decade (Haimovici et al., Reference Haimovici, Leite, Marinho, Batista, Madrid, Oliveira, Lima, Candice, Haimovici, Andriguetto and Sunye2014).
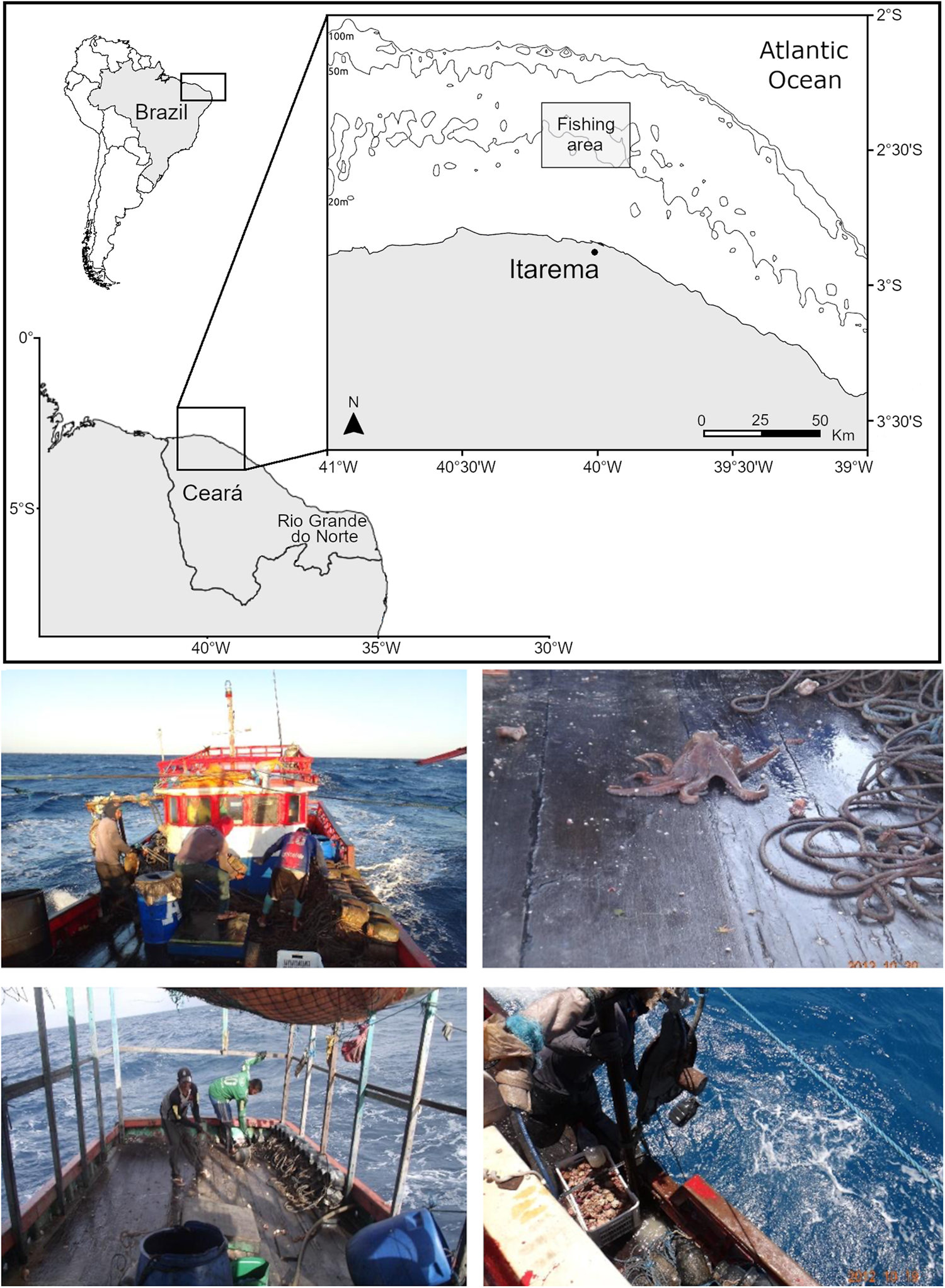
Fig. 1. Fishing grounds (above) of the longline pot fishery (below) targeting stout reef octopus Octopus insularis in Itarema, Ceará State in north-eastern Brazil.
Octopus insularis is a mid-sized octopus that usually attains less than 2 kg of total weight (Haimovici et al., Reference Haimovici, Leite, Marinho, Batista, Madrid, Oliveira, Lima, Candice, Haimovici, Andriguetto and Sunye2014) with a large distribution in the tropical and subtropical Atlantic Ocean (Lima et al., Reference Lima, Strugnell, Leite and Lima2020), mostly recorded at temperatures ranging from 24–30°C and at depths under 40 m. This species lays a large number of small eggs and has a meroplanktonic paralarval phase (Leite et al., Reference Leite, Haimovici, Molina and Warnke2008; Lenz et al., Reference Lenz, Elias, Leite and Vidal2015). Its population structure and reproductive dynamics in shallow reefs were studied in shallow coastal waters of the state of Rio Grande do Norte, north-eastern Brazil by Lima et al. (Reference Lima, Leite, Haimovici and Oliveira2014a, Reference Lima, Leite, Haimovici, Nóbrega and Oliveira2014b) and in the Veracruz Reef System in the Gulf of Mexico by Jiménez-Badillo et al. (Reference Jiménez-Badillo, del Río-Rodríguez, Gómez-Solano, Cu-Escamilla and Méndez-Aguilar2008) and González-Gómez et al. (Reference González-Gómez, Meiners-Mandujano, Morillo-Velarde, Jiménez-Badillo and Markaida2020).
In this study, based on a year-long intensive collection of data from a pot longline fishery based in Itarema, we studied the growth, lifespan and seasonal changes in size composition, sex ratios, sexual maturation and abundance of O. insularis in a tropical environment, as well as the sustainability of the fishery.
Materials and methods
The study area
The continental shelf along northern Ceará is ~60 km wide (Figure 1). From ~8–24 km from the coast, and down to a depth of 20 m, sediments are mostly quartz sand followed by rubble, rock and calcareous algae to the shelf-break at ~70 m depth (Summerhayes et al., Reference Summerhayes, Coutinho, Franca, Ellis, Milliman and Summerhayes1975; Marinho, Reference Marinho2011).
The regional climate of north-eastern Brazil is governed by the seasonally shifting intertropical convergence zone (ITCZ), for north-easterly and south-easterly Atlantic trade winds. The rainfall regime is strongly seasonal, with a rainy period in the austral summer, from January to July, in which almost all the yearly rainfall is concentrated. However, a large inter-annual variability associated with El Niño is responsible for droughts and floods (Maia et al., Reference Maia, Jiménez, Raventos and Morais1999; and references therein). In northern Ceará, the rainy season occurs in the first half of the year and is associated with higher sea surface water temperatures (SWT) and north-easterly weak trade winds. The dry season, the second half of the year is associated with lower SST and stronger south-easterly trade winds (Funceme, 2020). In the rainy season, river discharges may mix with coastal waters up to 6 miles from the coast and down to depths under 50 m, increasing turbidity and increasing productivity. During the dry season, there is a larger influence of the tropical waters of the North Brazil Current (Dias et al., Reference Dias, Castro and Lacerda2013).
Sea surface water temperature and chlorophyll-a concentration (Chl-a) were obtained with the Giovanni online data system, developed and maintained by the NASA GES DISC (2021) in the bounding box: 35.916°W 5.386°S, 34.810°W 4.7104°S; the total rainfall (TR) from Funceme (2020); and the wind intensity from Maia et al. (Reference Maia, Jiménez, Raventos and Morais1999) and Windguru (2020) measured in Jericoacoara (40°30′28.80″W 2°47′38″S).
Monthly means for the available data from 1980 to 2020 and between September 2009 and August 2010 are shown in Figure 2. Along the sampling period, the mean monthly SST ranged from 26.3°C in August 2010 and 29.6°C in March 2010 (Figure 2A). The rainfall was over 1 mm day−1 between January and July 2010, with a 4.9 mm day−1 peak in April, and it was lower than the long-term mean (Figure 2B). Chl-a was less than 0.30 mg m−3 between October 2009 and March 2010 and increased to a peak at 0.60 in July 2010, at the end of the rainy season (Figure 2C). The lowest monthly mean wind intensity was in October 2009 and the highest in July 2010 (Figure 2D).

Fig. 2. Monthly changes in the seawater surface temperature (A), rainfall (B), chlorophyll-a intensity concentration (C) and wind (D) along northern Ceará State, Brazil. Punctuated lines represent long-term trends (1980–2020), full circles refer to data taken between September 2009 to August 2010 (sources in the text).
The pot longline fishery in Itarema
The fishery of octopuses with pot longlines along Ceará State was described by Braga et al. (Reference Braga, Marinho, Batista and Rocha2007). It was introduced in 2005 in an area of around 2000 km2, at a depth ranging from 19–40 m between 39°30′W and 40°30′W, distant from the coast 27–55 km (Figure 1). The fishing boats that land their catch in Itarema are 10–15 m long with a small cabin at the stern, powered by motors of over 60 HP, and equipped with an echo sounder, GPS, VHF radios and hydraulic winches (Figure 1). Longlines are 2000–4000 m long, of twisted polyester and polypropylene of 10 mm of diameter, each with 200–400 pots attached with snaps to 0.5 m secondary lines of 3 mm twisted polyester. The pots, 30 cm long, 17 cm in diameter, and 11 cm in the mouth are made of polyethylene and have a large base ballasted with up to 3 kg of concrete and short secondary lines to avoid wearing due to rolling over the stone and gravel bottoms over which the species is captured. Longlines are moored with anchors, no buoys are used for localization to avoid theft, and pots are examined at 2-week intervals.
Data collection
From September 2009 to August 2010, the pot longline fishery for Octopus insularis in Itarema was studied, combining dockside interviews, onboard samplings and collection of specimens. Skippers provided logbooks of 119 fishing trips in which the number of pots, the number and total weight of octopuses captured, the fuel cost, and other expenses, were reported.
A total of 1438 specimens were collected onboard, randomly from the catch of 12 monthly fishing trips. Each specimen was weighed in grams with a digital dynamometer, the dorsal mantle length (ML) measured in mm and the sex determined visually, as the males have a large white membrane along the hectocotylus arm. Also onboard, the total number of hauled pots and the number of pots with attached egg masses were recorded.
In the laboratory, 312 specimens collected along the year, weighed with 0.1 g and measured with 1 mm precision, were examined for morphometrics and sexual maturity. Maturity stages were recorded in three categories: maturing, mature and spawned, based on a macroscopic four stage scale (Guerra, Reference Guerra1975). Furthermore, 164 beaks of specimens from the catches representing all size ranges were collected for morphometrics and age determinations.
Age and growth
The periodicity of the formation of growth increments in the beaks of six specimens collected in Itarema was studied in captivity for up to 21 days. The specimens were kept in circular tanks of 320 litres with filtered seawater flowing at a rate of 87.5 l h−1 with a complete renewal of seven times a day and fed twice a day with live portunid crabs Callinectes sp. Environmental conditions along the experiment were fairly stable, photoperiod was 12/12 h typical at equatorial latitudes, and water temperature and salinity were 25 ± 1°C and 32 respectively. Each tank was shared by two specimens with individual PVC shelters (Batista, Reference Batista2011).
After two days of acclimatization, each octopus was injected with 1 ml of a 120 mg ml−1 solution of oxytetracycline and received a second dose 7 days later. Oxytetracycline chelates with chitin, one of the major components of the beaks (Miserez et al., Reference Miserez, Rubin and Waite2010), resulting in marks visible by fluorescence, permitting correlation of the number of growth increments (GI) with a known number of days between the date of treatment and the date of an animal's sacrifice (Oosthuizen, Reference Oosthuizen2003). The doses used in the experiments were the same as those used by Oosthuizen (Reference Oosthuizen2003) to mark Octopus vulgaris of similar sizes.
Before sacrifice, marked octopuses were placed in 20 l jars in which seawater temperature was decreased (1°C min−1) and anaesthetized with magnesium chloride (Messenger et al., Reference Messenger, Nixon and Ryan1985). All six specimens showed a fluorescent band in the distal portion of the lateral wall of the longitudinally sectioned beaks when examined under an epifluorescence microscope with ultraviolet illumination of 400 nm wavelength (Figure 3A). The number of growth increments marked edges of the lateral walls was counted three times with a binocular microscope with a 2-week interval between readings (Figure 3B). The consistency between the counts of newly formed rings along the border and the number of days was considered a criterion to validate the age determinations.
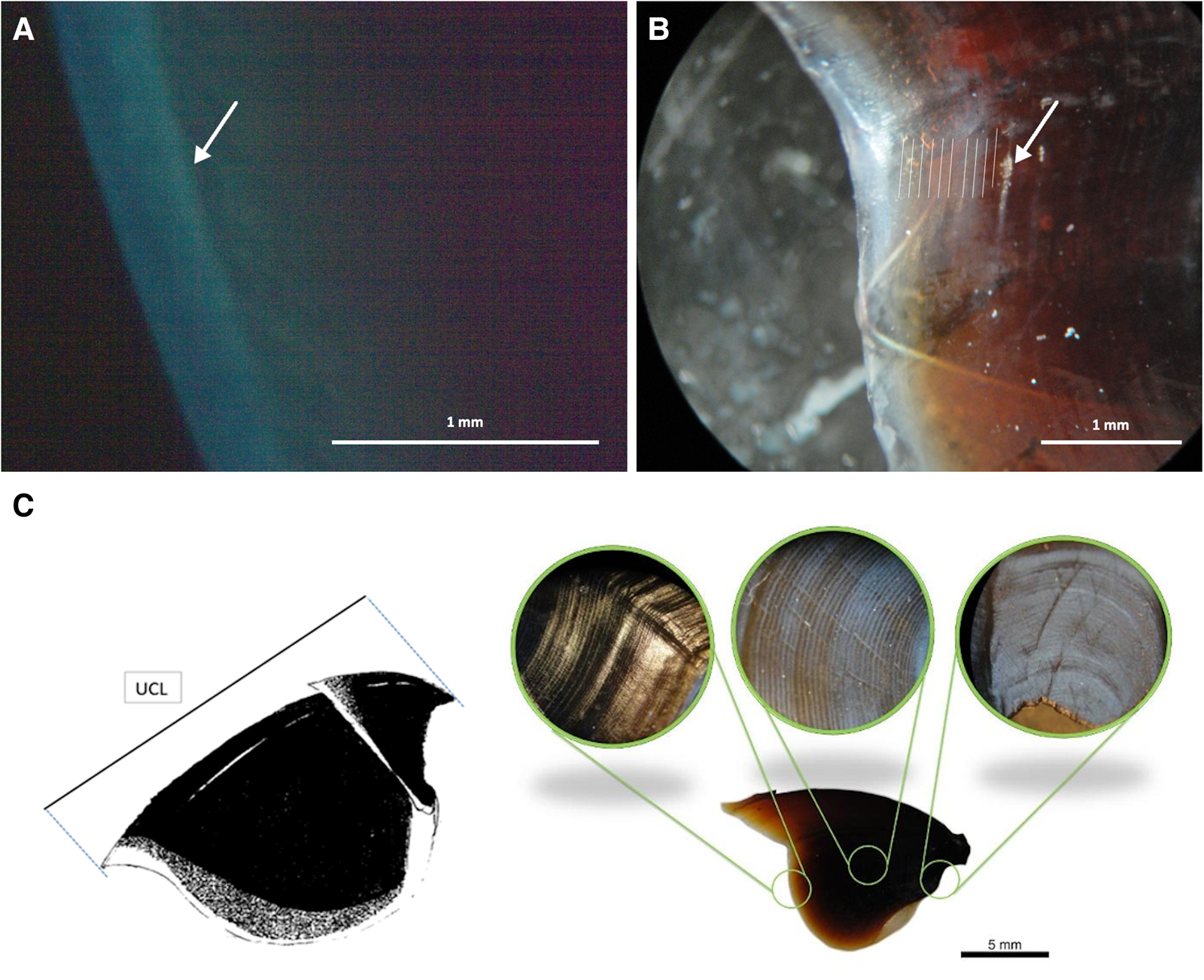
Fig. 3. The stained distal portion of the lateral wall of the upper beak of a specimen of Octopus insularis previously injected with oxytetracycline examined with ultraviolet illumination (A); position of the growth increments counted along the stained portion of the lateral wall (B); the crest length (UCL) and the growth increments along the lateral wall of the upper beak (C).
Overall, six specimens weighing 100–320 g, were kept in the tanks after being marked, one died in the 10th day, three sacrificed after 12 days and two after 21 days (Table 1). The mean increase in grams/day and the specific growth rate (SGR) in %/day was calculated for each specimen as (SGR = 100 (eg – 1) in which g is the instantaneous growth rate (g = ln (W2 /W1)/(t2–t1)) as defined in Ricker (Reference Ricker1975) and Crane et al. (Reference Crane, Ogle and Shoup2020).
Table 1. Growth rates of six specimens of Octopus insularis marked with oxytetracycline in captivity (CV coefficient of variation)

A total of 164 specimens collected in the fishing trips had their beaks examined for age determinations (Figure 3C). After several preliminary readings, 14 specimens (9.3%) were discarded because growth increments could not be counted along the upper beaks' lateral walls. Two final counts of the growth increments with a one-month time interval by the first author provided a counting error measure. The coefficient of variation (CV) between the two readings was calculated as:

where R1 and R2 were the first and second counts, and R the average of both counts (Campana, Reference Campana2001). Fourteen specimens for which CV was over 10% were excluded from the former analysis. The mean CV for the remaining 136 age determinations was 2.303.
Perales-Raya et al. (Reference Perales-Raya, Almansa, Bartolomé, Felipe, Iglesias, Sánchez and Rodríguez2014) showed that for three adults of O. vulgaris of known-age the GI counts in the lateral wall of the beaks were 21 increments lower than the true ages. Therefore, although we validated the daily deposition of the increments to describe the growth and the relation with maturity, we refer to the number of daily growth increments (GI) or rings counted and not in terms of days of life.
Statistical analysis
Statistical analysis included regression models in calculating the relationships between the dorsal mantle length (ML), the body weight (TW), the upper beak crest length (UCL) and the number of growth increments (GI). Covariance analyses were used to compare the linear regressions and the log-transformed potential regressions between sexes. χ2 tests for heterogeneity were used to compare sex ratios between months and size ranges (Zar, Reference Zar1999).
A Redundancy Analysis (RDA) (Legendre & Legendre, Reference Legendre and Legendre1998) was applied to analyse the influence of environmental variables on the abundance and proportion of large octopus in the monthly catches. The response variables included in the RDA were the log transformed mean monthly CPUE (kg day−1) and the monthly percentage of specimens over 800 g (TW > 800 g). The environmental explanatory variables were: the sea surface temperature (SST), rainfall (RAIN), wind stress (WIND), chlorophyll-a of the corresponding month (CHL) and chlorophyll-a 6 months earlier (CHL- 6), a proxy of the productivity at the early stages of the octopus if we assume that most specimens had between 100 and 160 GI and were born 4–8 months earlier. A permutational multivariate analysis of variance (PERMANOVA) was used to confirm the validity of the groups formed in the RDA. All calculations were performed in electronic spreadsheets and the free access PAST Statistical Package 4.03 (Hammer et al., Reference Hammer, Harper and Ryan2001).
Results
Morphometric relationships
Octopus insularis mantle length and body weight were recorded for a set of 162 males ranging 45–160 mm ML and 81–1245 g, and 150 females 50–160 mm ML and 60–1280 g. Males were heavier than females of the same mantle length (F = 9.44; P = 0.0023). The corresponding equations were BWmales = 0.0046 ML2.4613. R 2 = 0.8476; BWfemales = 0.0048 ML2.4342. R 2 = 0.8312. For both sexes pooled, the relationship was BW = 0.004 ML2.4862. R 2 = 0.8308.
The upper crest length (UCL) of the upper beaks was measured in the beaks of 67 males and 69 females. The linear regressions between of the upper crest length and the mantle length did not differ between sexes (F = 0.0043; P95% = 0.947) and was ML (mm) = 0.9817 + UCL 6.767 (R 2 = 0.7719) (Figure 4A). The power regression of the upper crest length and the body weight was BW(g) = 1.284 TL 2.9305 (R 2 = 0.8981) (Figure 4B) and did not differ between sexes (F = 0.553; P95% = 0.458).

Fig. 4. Upper beak crest length relationships with the dorsal mantle length (A) and the body weight (B); and number of daily growth increments relationships with the dorsal mantle length (C) and the body weight (D) of Octopus insularis fished in Itarema, north-eastern Brazil.
Age, growth and maturity
Validation of the daily formation of growth increments in the beaks
The specimen dead on the 10th day after being marked had 10 GI in the dyed distal border of the lateral walls in all the counts. Those sacrificed after 12 days, had in average 10.9 GI with a CV% of 0.22, and two specimens sacrificed after 21 days, had 21 GI and a CV% of 0.22 (Table 1). It was concluded that each ring or growth increment in the posterior border of the lateral wall of the beaks corresponds to one day of life. Otherwise, one specimen did not accept food during the experiment, the other five increased their weight by 40–113 g, on average 90 g. Among those that accepted food, the average daily weight increase was 4.24 g and the specific growth ratio in weight ranged from 0.7% day−1 and 4.6% day−1, on average 2.6% day−1 (Table 1).
Growth in the wild
The number of rings in the beaks of the 67 aged males measuring from 47–168 mm ML ranged from 43–172 GI, and in 69 females measuring 43–145 mm ML from 50–162 GI (Table 2). The linear regressions between the mantle length and the number of GI of males and females did not differ (F = 2.85; P = 0.105), and the common linear regression was ML (mm) = 0.9123 GI + 2.2425, R 2 = 0.9178 (Figure 4C). The linear regression between the log converted body weight and the GI of males and females did not differ between sexes (F = 0.67; P = 0.415), and the common potential regression was TW(g) = 0.0044 GI2,428, R 2 = 0.8294 (Figure 4D).
Table 2. Absolute (ΔBW), relative (ΔBW%) growth and instantaneous growth rates in 20 daily growth increments (GI) intervals of Octopus insularis fished in Itarema, NE Brazil

The mean absolute body weight increase (ΔBW), and the specific growth rate were compared among seven bins of 20 GI classes (Table 2). The mean ΔBW was 1.3 g day−1, among octopus with >60 GI, was between 3.7 and 7.8 in the five following bins and decreased sharply among those with >140 GI to 0.3 g day−1. The specific growth rate decreased from 8.8% in the 40–59 GI to 3.7% in the 140–160 GI bins and 0.4% for those with over 160 GI. The sharp slow-down among those with 160 GI is evidence of the proximity of the end of their life cycle.
Maturity and reproduction
Maturing and mature males and females were recorded year-round. In addition, spent females were observed in all months except July, and egg masses attached to pots were observed in all months except October. Therefore, it was concluded that O. insularis reproduces year-round in the study area.
Among the 150 females sampled, 36.7% were in early maturation (stage 2), weighed 420 g mean (range 57–854 g) and had 62–166 GI; 46.0% were mature (stage 3), weighed 562 g mean (144–1251 g) and had 50–162 GI; the remaining 17.3% were spent females (stage 4), weighed 697 g mean (389–1280 g) and had 120–158 GI. All 162 males sampled were mature (stage 3), with a mean body weight of 592 g (range 45–1245 g), and with 43–172 GI in their beaks (Table 3).
Table 3. Body weight and the number of daily growth increments in different stages of maturity of Octopus insularis fished along Itarema, NE Brazil between September 2009 and August 2010

Size and sex composition
When all 1438 specimens measured on board were grouped in bins of 100 g of body weight, the most frequent weight ranges were 400–499 g and for females 500–599 (Figure 5A).
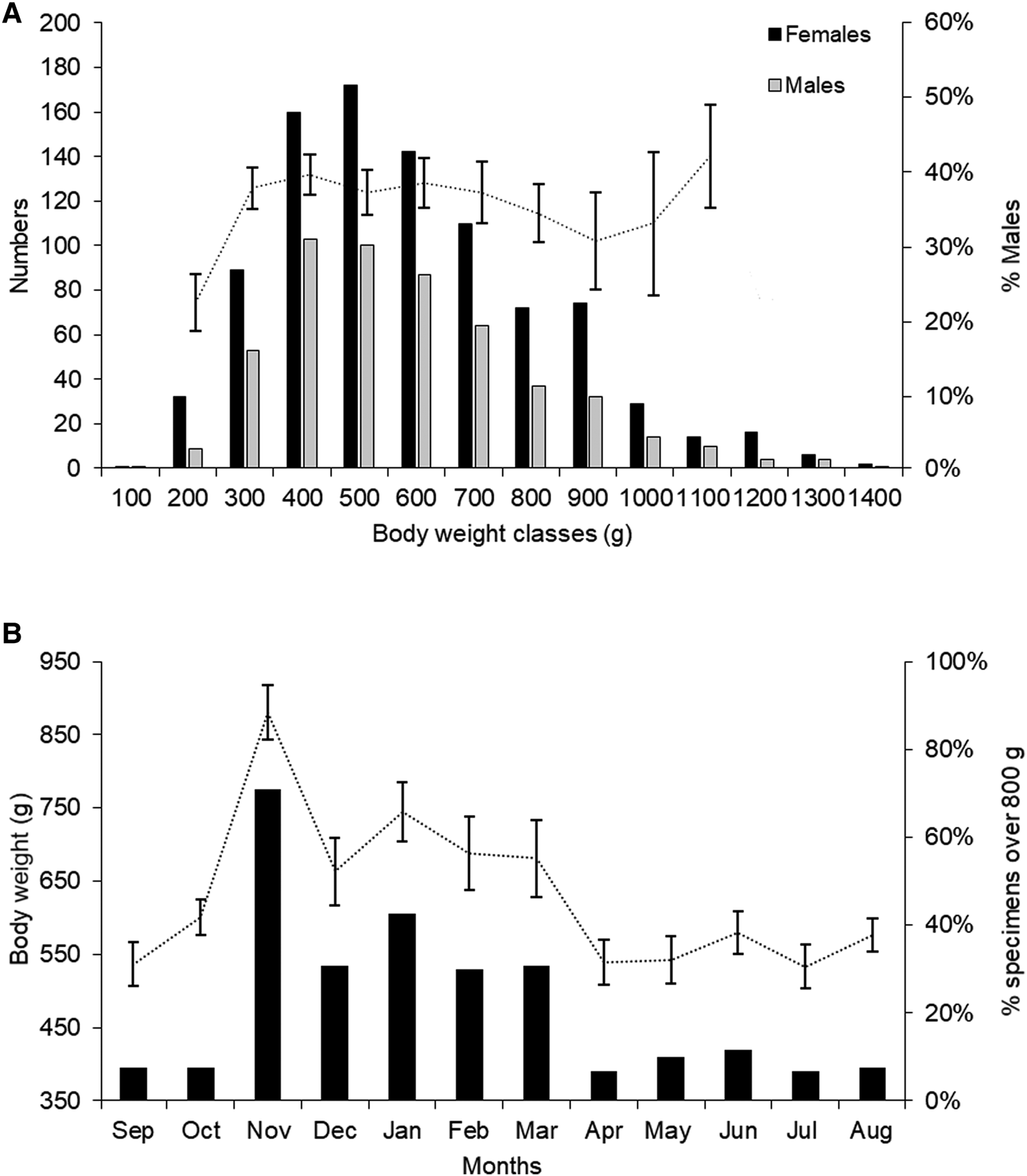
Fig. 5. Per cent frequency and distribution of the body weight by sex (A) and monthly average body weight and percentage of specimens over 800 g (B) of Octopus insularis fished with pot longlines along Itarema, north-eastern Brazil between September 2009 and August 2010 (N = 1438, vertical bars represent the 95% confidence intervals).
Mean monthly weights show an annual cycle in which the higher values were between October and March and the lower between April to September. A remarkable difference in the proportion of large specimens (over 800 g) was observed between these periods: 7–12% from April to October and 31–71% from November to March (Figure 5B).
Overall, the sex ratio in the onboard sampling was 63.8% females and 36.1% males (1.77 F: 1). Sex ratios did not differ among the months sampled (χ2(11.0.05) = 1.402; P = 0.410). No differences were observed among body weight classes either (χ2(5.0.05) = 1.3907; P = 0.994).
Catch and effort
During the sampled year, the average monthly number of fishing trips landed in Itarema was 9.92, ranging from 7–17. The average monthly trip duration was 5.8 days, ranging from 4.9–6.6 days, and the average monthly catch was 8370 kg, ranging from 3119–22,179 kg. As a result, the mean monthly catch-per-unit-effort (CPUE) ranged from 58.8–256.9 kg per day at sea, 0.04–0.16 kg per 1000 hauled pots, and the fuel expenses ranged from 0.60–2.91 R$ per kg of octopus (Table 4). A strong correlation was observed among the three CPUE units and a clear annual cycle with the higher values in October 2009, decreasing sharply to the lower values in January 2010, with a slow increase from February onwards (Figure 6).
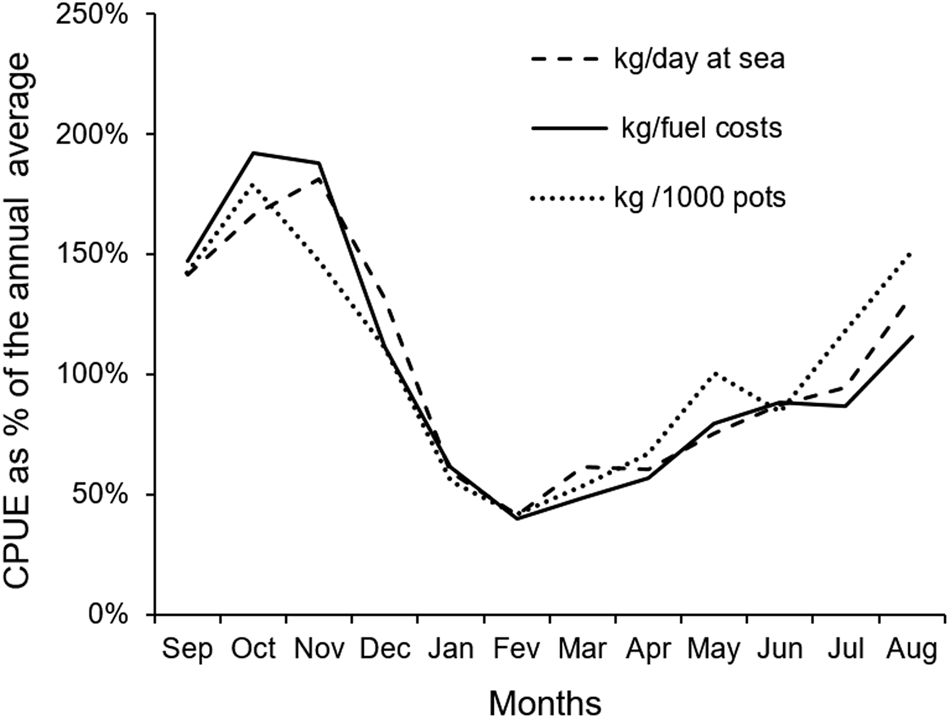
Fig. 6. Monthly catch-per-unit-effort (CPUE) recorded in 112 longline pot fishing trips for Octopus insularis landed in Itarema, north-eastern Brazil, between September 2009 and August 2010. CPUE expressed as percentages of the year-round mean total weight of octopus fished in kg per day at sea (dotted line), per 1000 examined pots (full line) and kg per fuel expenses (dashed line).
Table 4. Monthly catch and effort data recorded by the skippers in 112 pot longline fishing trips targeting Octopus insularis landed in Itarema, NE Brazil, along an annual cycle from September 2009 to August 2010

Relationship between abundance and size composition with seasonal environmental changes
The RDA of the log-transformed variables explained 90.99% of the variability along the first two axes (Figure 7), in which the first axis explained 70.84% and showed a strong association between the percentage of specimens over 800 g (LARGE OCTOPUS) and the chlorophyll-a 6 months earlier (CHL-6). The second axis explained 20.15% and showed a strong positive association between CPUE (kg day−1) and stronger winds (WIND), lower sea surface temperatures (SST), and lower rainfall (RAIN). Positive values along axis 2 were associated with the dry and colder months (July to December) of the second semester and the negative values to the warmer and rainy months (January to June) of the first semester. Linear r-Pearson correlations were found between TW > 800 and CHL-6 (0.7061; P = 0.0102), kg day−1 and WIND (0.8264; P = 0.0009) and negative correlation between kg day−1 and RAIN (−0.6361; P = 0.0261) and between kg day−1 and SST (−0.7909; P = 0.0021). A PERMANOVA comparing the two 6-month periods discriminated by axis 2 showed significant differences (F = 5.602; P = 0.0092).
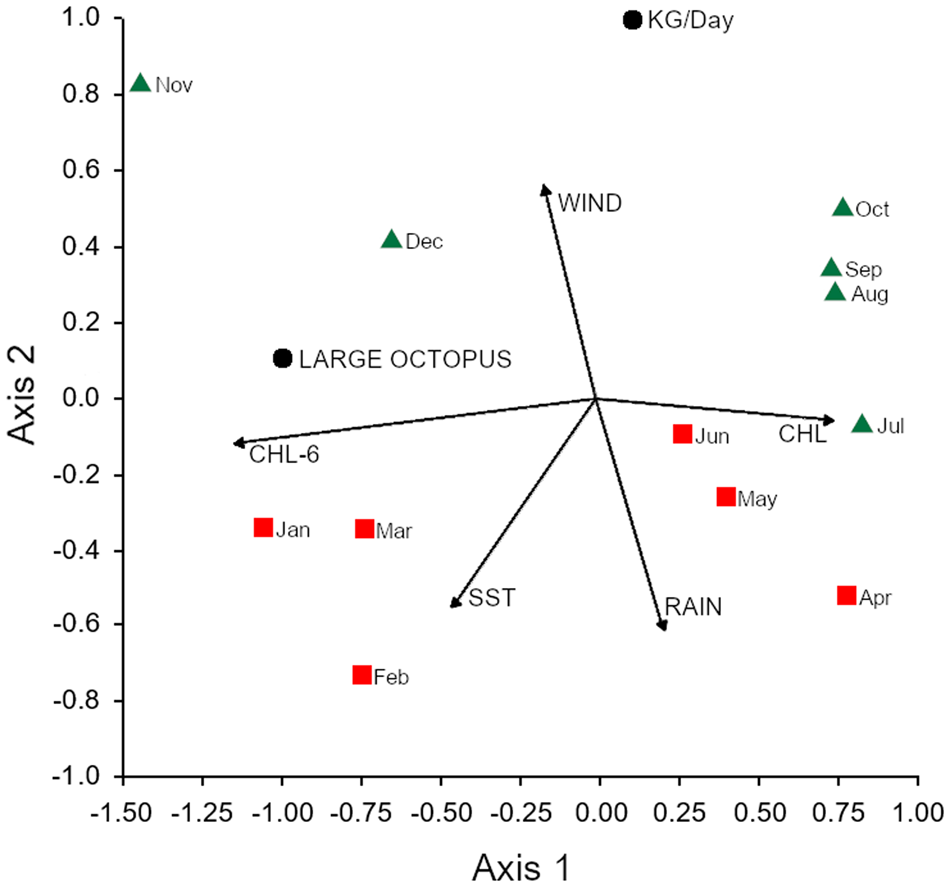
Fig. 7. Redundancy analysis plot of the monthly catch-per-day at sea (KG/Day) and the percentage of stout reef octopus over 800 g (LARGE OCTOPUS) with the monthly mean of the sea surface temperature (SST), rainfall (RAIN), wind stress (WIND) and chlorophyll-a of the corresponding month (CHL) and 6 months earlier (CHL-6) along Itarema, north-eastern Brazil. Green triangles represent the months of the dry season in the second semester and red squares the months of the first semester season (rainy season).
Discussion
Age, growth and lifespan
This study provides the first experimental evidence of a daily deposition of growth increments in the upper beak's lateral walls of Octopus insularis. Our results agree with the one-day-one-increment hypothesis raised by Raya & Hernández-González (Reference Raya and Hernández-González1998) and validation studies for Octopus vulgaris on reared paralarvae (Hernandez-Lopez et al., Reference Hernández-López, Castro-Hernández and Hernández-García2001), specimens marked with thermal shocks (Canali et al., Reference Canali, Ponte, Belcari, Rocha and Fiorito2011), marked with calcofluor and other markers (Perales-Raya et al., Reference Perales-Raya, Almansa, Bartolomé, Felipe, Iglesias, Sánchez and Rodríguez2014). Longer experiments with other marking treatments are desirable to further explore the one-ring-one-day hypothesis for O. insularis, however, our results provide some insight into the growth and lifespan of this species.
We compared the lifespan and growth of O. insularis in the natural environment with other congeneric species for which the daily periodicity of the growth increments was validated, either on beak or stylets. The species included were: O. vulgaris (Hernández-López et al., Reference Hernández-López, Castro-Hernández and Hernández-García2001; Oosthuizen, Reference Oosthuizen2003; Perales-Raya et al., Reference Perales-Raya, Bartolomé, García-Santamaría, Pascual-Alayón and Almansa2010; Canali et al., Reference Canali, Ponte, Belcari, Rocha and Fiorito2011); Octopus cyaena (Herwig et al., Reference Herwig, Depczynski, Roberts, Semmens, Gagliano and Heyward2012); Octopus aff. tetricus (Leporati et al., Reference Leporati, Hart, Larsen, Franken and De Graaf2015); and Octopus americanus (formerly O. vulgaris type II) (Castanhari & Tomas, Reference Castanhari and Tomás2012), for which no validation is still available. For each species, we recorded the latitude, the highest and lowest monthly temperatures of sea-surface water, body weight, number of growth increments range, and the specific growth rate between the younger and older ages reported assuming an exponential growth (Table 5). Although some bias was inevitable, we observed that O. insularis had a smaller maximum number of growth increments (172 GI) than all the other species (314–601 GI), and a higher specific growth rate (2.62% day−1) than the others (0.94–1.62% day−1) (Figure 8). We concluded that O. insularis in north-eastern Brazil's tropical environment has a faster growth and a shorter lifespan than those species of higher latitudes (Figure 8). It is tempting to associate the faster growth and shorter lifespan of O. insularis in north-eastern Brazil solely with seawater temperature. However, numerous biotic and abiotic factors influence the growth of octopuses, among which food and temperature are considered to play a major role (Forsythe & Van Heukelem, Reference Forsythe, Van Heukelem and Boyke1987; Semmens et al., Reference Semmens, Pecl, Villanueva, Jouffre, Sobrino, Wood and Rigby2004). Therefore, as no variables other than temperature were considered in our comparisons and no other tropical octopuses were included in the comparison, further studies are necessary to test the hypothesis that faster growth, a short lifespan, and a smaller maximum size are part of a suitable strategy for octopuses living in a tropical oligotrophic environment.
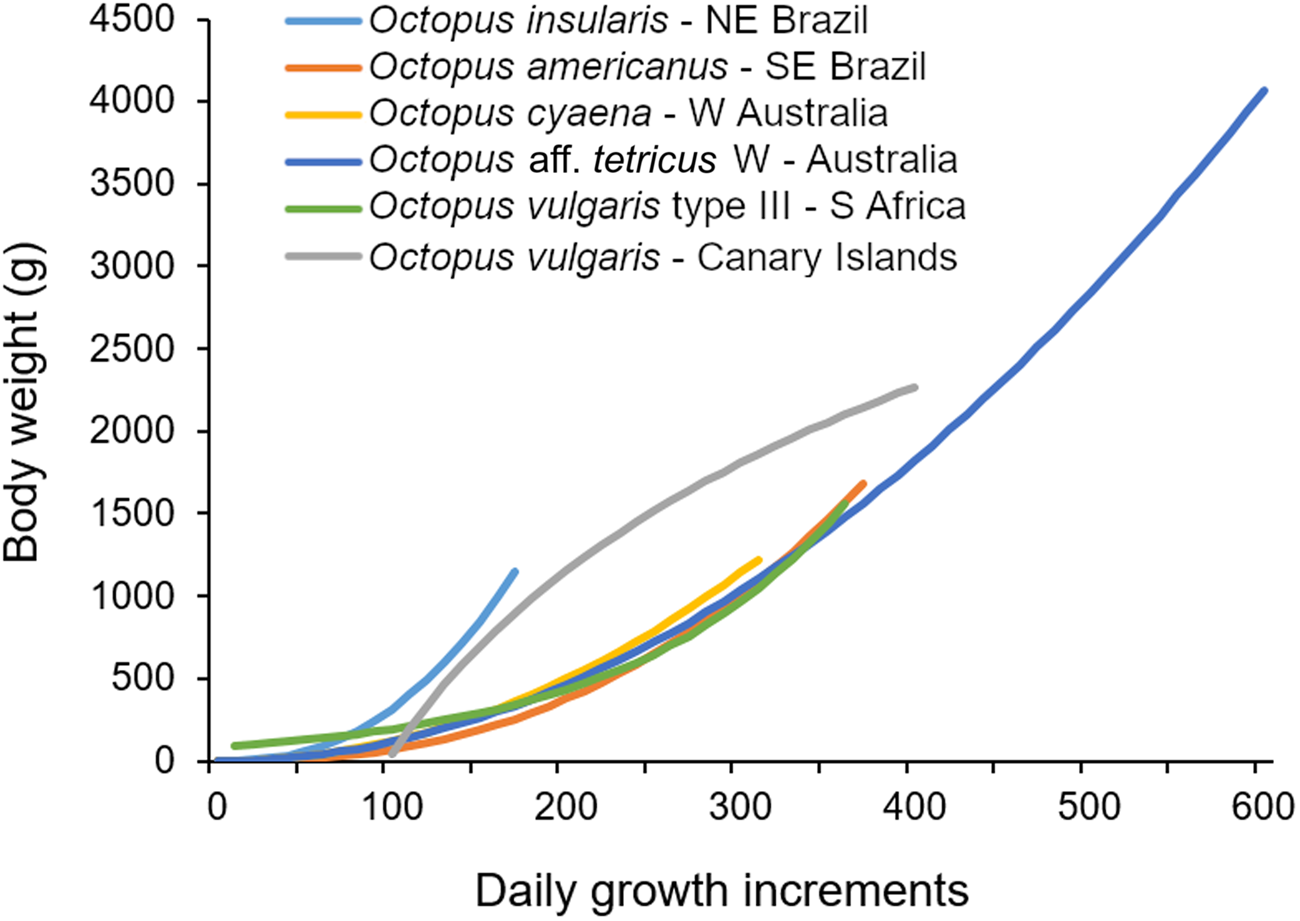
Fig. 8. Growth curves of seven coastal benthic Octopus species in their natural environment (references in the text).
Table 5. Comparisons between growth and longevity of mid and large-sized Octopus species for which ages were determined in their natural environment
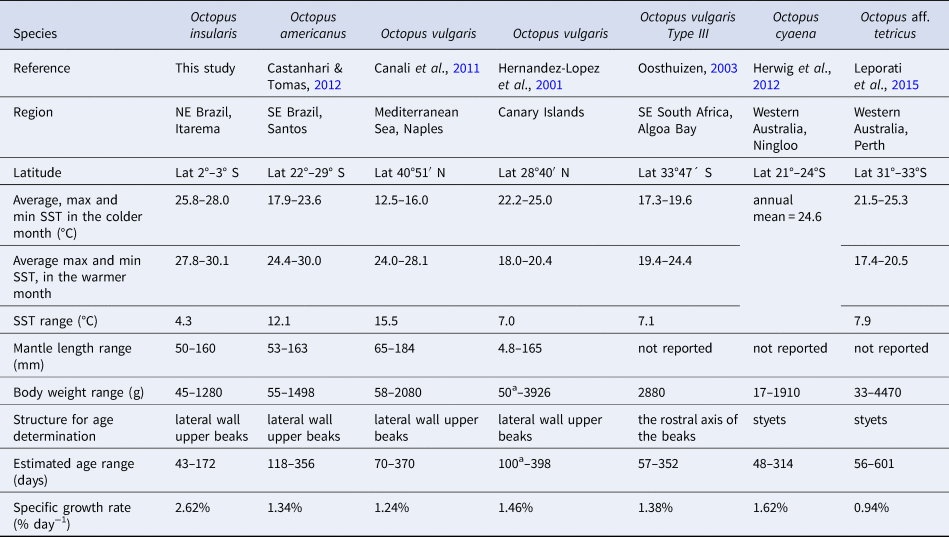
Specific growth rates calculated between the minimum and maximum reported ages and body weights. Maximum, minimum and the annual range of the surface water temperatures (SST) were obtained from https://www.seatemperature.org/.
a Assumed for juveniles to adults.
Reproductive dynamics
Ontogenetic migrations of benthic octopus were inferred from fishery data for several species, such as O. sinensis, O. vulgaris and Enteroctopus dofelini (Sauer et al., Reference Sauer, Gleadall, Downey-Breedt, Doubleday, Gillespie, Haimovici, Ibáñez, Katugin, Leporati, Lipinski, Markaida, Ramos, Rosa, Villanueva, Arguelles, Briceño, Carrasco, Che, Chen, Cisneros, Conners, Crespi-Abril, Kulik, Drobyazin, Emery, Fernández-Álvarez, Furuya, González, Gough, Krishnan, Kumar, Leite, Lu, Mohamed, Nabhitabhata, Noro, Petchkamnerd, Putra, Rocliffe, Sajikumar, Sakaguchi, Samuel, Sasikumar, Wada, Zheng, Tian, Pang, Yamrungrueng and Pecl2020). Ontogenetic migrations between depth ranges, usually towards deeper waters to ensure females have a more suitable habitat to brood their eggs, were suggested by Smale & Buchan (Reference Smale and Buchan1981) for O. vulgaris, Ortiz et al. (Reference Ortiz, Ré, Marquez and Glembocki2011) for E. megalocyathus and Leporati et al. (Reference Leporati, Hart, Larsen, Franken and De Graaf2015) for O. aff. tetricus. However, these migrations are difficult to confirm because Octopus females use rocky shelters and stop feeding while brooding their eggs, becoming less vulnerable to fishing activities. Lima et al. (Reference Lima, Leite, Haimovici, Nóbrega and Oliveira2014b) and González-Gómez et al. (Reference González-Gómez, Meiners-Mandujano, Morillo-Velarde, Jiménez-Badillo and Markaida2020) suggest that O. insularis migrate towards deeper waters to reproduce based on the scarcity of mature females and the absence of spawned females and egg masses in their samplings of shallow coastal waters in Rio do Fogo (depths <15 m) and in Veracruz (depth <5 m). In contrast, our samplings in a depth range of 19–40 m, contained mature specimens of both sexes, spawned females, and egg masses attached to the pots supporting the hypothesis of an ontogenetic migration of O. insularis towards deeper waters for spawning. However, Leite et al. (Reference Leite, Andrade, Haimovici, Aguiar, Lins-Oliveira and Hazin2009b, Reference Leite, Haimovici, Mather and Oliveira2009c) observed females guarding egg masses in a shallow inlet between two islands in St Peter and St Paul Archipelago in the mid-Atlantic ridge, where no shelf habitats are available, suggesting flexibility in the choice of the depth range for brooding.
The larger proportion of females (1.7:1) in the pots hauled at Itarema differed from the 1:1 sex ratio observed in the shallow waters of Rio Grande do Norte (Lima et al., Reference Lima, Leite, Haimovici, Nóbrega and Oliveira2014b) and Veracruz (González-Gómez et al., Reference González-Gómez, Meiners-Mandujano, Morillo-Velarde, Jiménez-Badillo and Markaida2020). The difference could be a consequence of higher mortality of the early maturation of the smaller males (Lima et al., Reference Lima, Leite, Haimovici, Nóbrega and Oliveira2014b) or to a lower rate of migration of males towards deeper waters. However, it can also be explained by the vulnerability of males to pots. Leite et al. (Reference Leite, Haimovici and Mather2009a) observed in O. insularis the ‘adjacent guardian courtship behaviour’ as defined by Huffard et al. (Reference Huffard, Caldwell and Boneka2008), in which one or several males remain near the den of a mature female, and therefore, not seeking for pots as dens. On the other hand, no mated males were captured in the pots, being evidence that senescent males do not look for shelters. Photoperiod, seawater temperature and food intake have been attributed to influence the onset of maturation and the duration of lifespan in cephalopods (Boyle & Rodhouse, Reference Boyle and Rodhouse2005). Species such as O. insularis, with a wide geographic range, may present different seasonal reproductive patterns at different latitudes: year-round in north-eastern Brazil (see above and Lima et al., Reference Lima, Leite, Haimovici, Nóbrega and Oliveira2014b) and from autumn to late spring in the Veracruz Reef System's higher latitudes in the Gulf of Mexico (Jiménez-Badillo et al., Reference Jiménez-Badillo, del Río-Rodríguez, Gómez-Solano, Cu-Escamilla and Méndez-Aguilar2008; González-Gómez et al., Reference González-Gómez, Meiners-Mandujano, Morillo-Velarde, Jiménez-Badillo and Markaida2020). Our data do not support a seasonality in the intensity of reproduction in Itarema (Figure 6); however, Lima et al. (Reference Lima, Leite, Haimovici and Oliveira2014a) observed that in Rio do Fogo, the peaks of maturation were strongly correlated with the cycles of the sea surface temperatures and wind intensity that lasted for ~3 months and occurred with 7–10 months intervals.
Seasonal changes in population structure and abundance
The abundance of O. insularis in the fishing grounds of Itarema follows an annual cycle, as shown by the larger CPUE during the dry season in the second semester of the year (Figure 7). Larger landings in the dry season in former years were reported by Madrid (Reference Madrid2009). Therefore, this seasonality can be considered a recurrent pattern in Itarema.
In north-eastern Brazil, the presence of mature and spawned females and egg masses shows that O. insularis spawns year-round, but the success in recruiting to the bottom and subsequent growth also depends on food availability. Thus, it can be hypothesized that larger cohorts of pelagic hatchlings may recruit during the season in which planktonic food availability is higher, as observed in other cephalopods of higher latitudes, e.g. lllex argentinus (Crespi-Abril & Baron, Reference Crespi-Abril and Barón2012).
Filter feeders of benthic detritus such as small crabs and molluscs are the main prey of O. insularis (Leite et al., Reference Leite, Haimovici and Mather2009a). Seasonal changes in chlorophyll-a levels are a proxy of planktonic productivity which, several months later, increase the benthic biomass. At Itarema, chlorophyll-a increases from April to August (Figure 3). Octopuses spawning shortly earlier should be 4–6 months old by August to December, approximately when the higher CPUE and catches were observed (Figure 7). This argument is also consistent with the positive correlation between larger specimens from November to March and the chlorophyll-a 6 months earlier (Figure 7). Therefore the seasonal cycle in the abundance of adults in Itarema may be a consequence of seasonal variations in the recruitment and subsequent growth success; however, this and alternative hypotheses require further studies. For example, a longer sampling period to check if the annual cycle described in 2009/2010 is typical for the region, as the rainfall along the sampled year was much lower than the mean (Figure 3). The rains regime along north-eastern Brazil is highly variable, and also there are interannual changes in the abundance of O. insularis, as observed in a decadal study for the small-scale fisheries along Rio Grande do Norte and recorded by Lopes et al. (Reference Lopes, Andrade, Pennino and Leite2021).
The state of the octopus fishery in Itarema
In north-eastern Brazil, the pot longline fishery was introduced in 2005 and shortly after regulated by establishing a maximum number of 25 licensed fishing boats, each fishing with a maximum of 5000 pots (Braga et al., Reference Braga, Marinho, Batista and Rocha2007). This rule was never enforced; therefore, this fishery can be considered free access.
We know very little about the fishery during the last decade as the landing statistics of the pot longline fishery along Itarema were collected only along a short period between 2005 and 2010. In 2010, 17 fishing boats landed 225 tonnes of octopuses (Marinho, Reference Marinho2011). In a recent interview with the owner of several fishing boats (Pedro Cotinha, pers. comm.), he informed us that fleet fishing along Itarema increased to around 25 boats and each boat now fishes with 10,000 to 15,000 pots compared with 9000 in 2010 (Table 4). He also pointed out that the fishery is still profitable.
Unmanaged open-access fisheries are seldom sustainable because of fishers' natural trend to reinvest their gains in more or bigger boats or more efficient gears and new fishers to join the fishery (Pitcher & Hart, Reference Pitcher and Hart1982). However, this is not the case in Itarema pot longline fishery in which the fleet did not increase or decrease sharply in the last decade. The fact that the fishery did not collapse can be attributed to two factors. The first is the life strategy of O. insularis: semelparity, short lifespan, fast growth and high fecundity. These biological characteristics favour the resilience of cephalopod fisheries and their quick recovery after intense fishing (Rodhouse et al., Reference Rodhouse, Pierce, Nichols, Sauer, Arkhipkin, Laptikhovsky, Lipinski, Ramos, Gras, Kidokoro, Sadayasu, Pereira, Lefkaditou, Pita, Gasalla, Haimovici, Sakai and Downey2014; Doubleday et al., Reference Doubleday, Prowse, Arkhipkin, Pierce, Semmens, Steer, Leporati, Lourenço, Quetglas, Sauer and Gillanders2016). The second factor is the lack of strong pressure of customer demand. Because of its relatively small size, O. insularis fished in Itarema and Rio Grande do Norte are consumed regionally in Brazil's north-eastern region (Pedro Cotinha pers. comm.; Andrade, Reference Andrade2015). Therefore, it was concluded that unless demand and prices increase to stimulate an increase of fishing effort, the Itarema fishery is not likely to collapse.
Acknowledgements
We thank Mr Pedro Cotinha and the skippers of the fishing boats from Itarema for their support and valuable information on the fishery, to Francisco Getúlio Silva Guimaraes, Jorge Lima, Vladimir Holanda and Paulo Roberto Brasil, for their participation in the collection and processing of the data students and the Federal University of Ceará for the logistical support. We thank Tatiana S. Leite and Ricardo Palma for useful comments to the final draft, and the anonymous reviewers for their thorough analysis and comments on the submitted manuscript.
Financial support
This work was supported by Fisheries and Aquaculture Ministry (R.M. grant number 00350.001296/2008-71); the Coordination for the Improvement of Higher Education Personnel (B.B. and E.K. scholarships); and the Brazilian National Council for Scientific and Technological Development (CNPq) (M.H. research grant 3007994/2020-1).
Conflict of interest
No potential conflict of interest was reported by the author(s). The procedures followed to anaesthetize the specimens before sacrifice followed the Brazilian and international standards.