INTRODUCTION
The genus Paragonimus contains over 50 nominal species of lung flukes of mammals distributed in many tropical and subtropical parts of the world. About 15 species are important zoonotic parasites causing paragonimiasis in man (Blair et al. Reference Blair, Xu and Agatsuma1999, Reference Blair, Agatsuma, Wang, Murrell and Fried2007; Sripa et al. Reference Sripa, Kaewkes, Intapan, Maleewong and Brindley2010). It is estimated that over 20 million people are infected in eastern and southern Asia, Central and South America, and parts of Africa (Keiser and Utzinger, Reference Keiser and Utzinger2009; Toscano et al. Reference Toscano, Yu, Nunn and Mott1995). The best known species is P. westermani (Kerbert, 1878), which is widely distributed in Asia from the Indian subcontinent to northern China, Korea, south to the Philippines, Vietnam, Thailand, Malaysia and Indonesia. Adult worms are morphologically very conservative across this extensive range. Despite this, the species is known to be infective to humans only in China, Japan, Korea, Taiwan and the Philippines. A number of molecular studies (Blair et al. Reference Blair, Agatsuma, Watanobe, Okamoto and Ito1997; Iwagami et al. Reference Iwagami, Ho, Su, Lai, Fukushima, Nakano, Blair, Kawashima and Agatsuma2000, Reference Iwagami, Rajapakse, Paranagama, Okada, Kano and Agatsuma2008; Binchai et al. Reference Binchai, Rangsiruji, Ketudat, Morishima and Sugiyama2007; Sugiyama et al. Reference Sugiyama, Morishima, Binchai, Rangsiruji and Ketudat2007; Tandon et al. Reference Tandon, Prasad, Chatterjee and Bhutia2007; Devi et al. Reference Devi, Narain, Agatsuma, Blair, Nagataki, Wickramasinghe, Yatawara and Mahanta2010; Doanh et al. Reference Doanh, Shinohara, Horii, Habe, Nawa and Le2007a,Reference Doanh, Shinohara, Horii, Habe, Nawa, The and Leb, Reference Doanh, Shinohara, Horii, Habe, Nawa and Le2008, Reference Doanh, Shinohara, Horii, Habe and Nawa2009) applied to P. westermani have discovered considerable genetic variation which, coupled with biological information such as differences in snail host and infectivity to different mammals, indicates that ‘P. westermani’ constitutes a species complex that also contains within it the morphologically distinct species P. siamensis (Miyazaki and Wykoff, Reference Miyazaki and Wykoff1965).
It had long been assumed that human cases of pulmonary paragonimiasis in India were due to P. westermani, but recent molecular studies demonstrated that a different species, P. heterotremus, is the culprit (Devi et al. Reference Devi, Narain, Bhattacharya, Negmu, Agatsuma, Blair, Wickramashinghe and Mahanta2007; Singh et al. Reference Singh, Sugiyama, Umehara, Hiese and Khalo2009). However, the presence of a member from the P. westermani complex has recently been confirmed with molecular and morphological evidence (Devi et al. Reference Devi, Narain, Agatsuma, Blair, Nagataki, Wickramasinghe, Yatawara and Mahanta2010; Tandon et al. Reference Tandon, Prasad, Chatterjee and Bhutia2007), but it remains unclear whether this might be infective to humans. Metacercariae of this form, found in freshwater crabs in northeastern (NE) India, are relatively thin-walled and ovoid and can mature in experimentally infected rats (Devi et al. Reference Devi, Narain, Agatsuma, Blair, Nagataki, Wickramasinghe, Yatawara and Mahanta2010). Here we refer to this as the type 1 metacercaria. In this paper 2 more types of metacercariae (types 2 and 3) are reported from NE India. Molecular data place both within the P. westermani complex, one of them is phylogenetically close to the P. westermani population that causes human disease in eastern Asia and the other is close to P. siamensis.
MATERIALS AND METHODS
Collection of crab specimens
From April 2009 to May 2011 edible freshwater crabs belonging to the species Maydelliathelphusa lugubris (Wood-Mason, 1871) (formerly, Barytelphusa lugubris) were collected from several hill streams of East Siang district of Arunachal Pradesh and small streams in the foothills of the northern region of Sonitpur district of Assam, India. The East Siang district of Arunachal Pradesh is a hilly region drained by the river Siang and its tributaries and lies between 27° 43′ N & 29° 21′ N and 94° 42′ E & 95° 35′ E. The northern part of the Sonitpur district of Assam is located on the north bank of the Brahmaputra River, close to hills of Arunachal Pradesh (between 26° 30′ N & 27° 01′ N and 92° 16′ E & 93° 43′ E). Freshwater crabs of a second species, Sartoriana spinigera (Wood-Mason, 1871), were collected from rice fields and wetlands of the Kamrup district of Assam. This district is situated between 25°46′N & 26°49′N and 90°48′E & 91°50′E. The 3 study regions, shown in Fig. 1, have a tropical monsoon rainforest climate and experience heavy seasonal rainfall and high humidity.

Fig. 1. Map showing the study areas: East Siang district of Arunachal Pradesh, Kamrup and Sonitpur districts of Assam, India.
Crabs were examined for metacercarial infection as described by earlier workers (Sugiyama et al. Reference Sugiyama, Sonoda, Tomimura and Nishida1983). Metacercariae were recovered from the muscles, hepatopancreas and gills of the crabs and identified initially by microscopic observation. These metacercariae were used for molecular work and in experimental attempts to raise adult worms in Wistar rats. Prior to this, photomicrographs of the metacercariae were taken with an Olympus IX81 motorized system (Japan) fitted with an ‘Evolution VF cooled colour camera’ (Media Cybernetics, MD, USA) and sizes of metacercariae determined using Image-Pro® V 6.2 acquisition, image processing and analysis software (Media Cybernetics, MD, USA).
DNA extraction and PCR amplification
Two type 1 and 21 type 2 metacercariae recovered from M. lugubris, 3 type 3 metacercariae from S. spinigera, and 4 adult flukes recovered from experimental rats fed type 1 metacercariae were used for extraction of DNA. DNA from individual specimens was extracted using chelex (10% chelex in 1% Triton X-100). DNA sequences from several gene regions (nuclear ribosomal second internal transcribed spacer (ITS2), partial ribosomal 28S gene and partial mitochondrial cox1 gene) from individual metacercariae and adult worms were amplified by polymerase chain reaction (PCR). ITS2 was amplified using the primers 3S and A28 (Bowles et al. Reference Bowles, Blair and McManus1995a,Reference Bowles, Blair and McManusb) and a portion of the 28S gene was amplified using primers reported by Littlewood and Johnston (Reference Littlewood and Johnston1995). The partial cox1 mitochondrial gene was amplified using primers reported by (Bowles et al. Reference Bowles, Blair and McManus1995a,Reference Bowles, Blair and McManusb). Each PCR was carried out in a total volume of 25 μl containing 10 μl of genomic DNA, 12·5 μl of 2x HotStar Taq Plus Master mix (Qiagen, California, USA) and 2·5 μl of nuclease-free water. For ITS2 and 28S, PCR amplifications were performed with the following cycling conditions: initial 10 min. at 94 °C, followed by 31 cycles of 30 sec. at 94 °C, 30 sec at 50 °C and 1 min at 72 °C. Final extension was for 10 min at 72 °C. For cox1, the following cycling conditions were used: initial 10 min at 94 °C, followed by 41 cycles of 30 sec at 94 °C, 40 sec at 48 °C and 40 sec at 68 °C. Final extension was for 10 min at 68 °C. The quality of PCR product was determined by electrophoresis through a 1·5% agarose gel.
Purification and sequencing
PCR products were excised from the agarose gel and purified using Nucleospin® Extract II Kit (MACHEREY-NAGEL, GmbH & Co. KG, Germany). The purified products were cycle-sequenced using ABI Big-Dye v3.1 chemistry. Unincorporated dye terminators, salt ions, dNTPs & ddNTPs were removed from cycle sequencing reactions using the BigDye® XTerminator™ purification kit from Applied Biosystems. All samples were run on a 16-capillary 3130xl automated sequencer (Applied Biosystems, Hitachi, Japan) at the Regional Medical Research Centre, Dibrugarh, Assam. The cycle-sequencing reactions were run in both directions using the PCR primers as sequencing primers.
Sequence alignment and phylogenetic analysis
Forty-nine new sequences of 28S (12), ITS2 (30) and cox1 (7) obtained by us are deposited in GenBank™ with the Accession numbers 28S (JN656173 – JN656181 & JQ322628 – JQ322630), ITS2 (JN656182 – JN656208 & JQ322634 – JQ322636) and cox1 (JN656169 – JN656172 & JQ322631 – JQ322633). Sequences were aligned with previously published corresponding sequences of lung flukes in the sequence editor program Geneious Pro 5.4.6 (Drummond et al. Reference Drummond, Ashton, Buxton, Cheung, Cooper, Duran, Field, Heled, Kearse, Markowitz, Moir, Stones-Havas, Sturrock, Thierer and Wilson2011) or DAMBE 5.2.34 (Xia and Xie, Reference Xia and Xie2001). Bayesian and Maximum Likelihood phylogenetic analyses of sequence data were performed using MrBayes as implemented in Geneious Pro 5.4.6 and MEGA v5.0 (Tamura et al. Reference Tamura, Peterson, Peterson, Stecher, Nei and Kumar2011), respectively. The appropriate model of nucleotide substitution was determined using jModeltest (Posada and Crandall, Reference Posada and Crandall2001) and model selection criteria were based on decision theoretic framework (DT) (Minin et al. Reference Minin, Abdo, Joyce and Sullivan2003). The General Time Reversible (GTR) model was the best-fit substitution model for ITS2 and 28S data sets and the Hasegawa-Kishino-Yano (HKY) model was the best substitution model for the cox1 gene data set. These models included rate variation among sites either both a proportion of invariant sites (+I) and gamma distributed rates (+G) in cases of cox1, ITS2 and 28S data sets or only gamma distributed rate (+G) for the concatenated data set. For construction of Bayesian trees, up to 5 million iterations were employed for each alignment (2 independent runs; 4 chains per run) and states sampled every 500 or 1000 cycles. Convergence of the runs was considered complete if the average standard deviation of split frequencies was <0·01. Burn-in was 10% of iterations. Maximum likelihood analysis was performed using the close-neighbour-interchange (CNI) heuristic strategy. To quantify the stability of the clades bootstrap analysis of 500 replicates was used.
In addition to the phylogenetic analyses of individual genes, phylogenetic trees based on combined genes (28S, cox1 and ITS2) were also constructed using maximum likelihood and Bayesian analysis to see what the topology of a combined analysis would look like compared to the separate genes. For the concatenated dataset both non-partitioned and partitioned analyses were conducted. For non-partitioned analysis the most suitable model of substitution was GTR + G and for partitioned analysis each partition was assigned its own substitution model. However, there was no difference in topology between partitioned and non-partitioned data sets.
Development of adult worms in Wistar rats
Type 1 metacercariae from Assam were fed to 3 rats (18–30 metacercariae each) for development of adult flukes. Metacercariae of type 2 were fed to Wistar rats (15 metacercariae to each of 2 rats in a first experiment, and 35 metacercariae to each of 5 rats in a second). Experimentally infected rats were sacrificed 2–3 months post-infection. Type 3 metacercariae were not used for experimental infection because too few were available. Adult worms recovered from infected animals were either kept for sequencing or stained with Aceto-Carmine.
Ethics statement
The Ethics Committee of the Regional Medical Research Centre, Northeastern Region of Indian Council of Medical Research, Dibrugarh, Assam reviewed and approved the protocol of this study prior to conducting the animal experiments. Control and Supervision of Experiments on Animals (CPCSEA) guidelines were adhered to.
RESULTS
Morphology of the metacercariae
Three distinct types of metacercariae (mc) belonging to the P. westermani complex (Fig. 2a–f) were found to exist in NE India: types 1 and 2 co-existed in the same crab host, M. lugubris, and in the same localities in Arunachal Pradesh and Assam. The dimensions of the three types of metacercariae are given in Table 1.
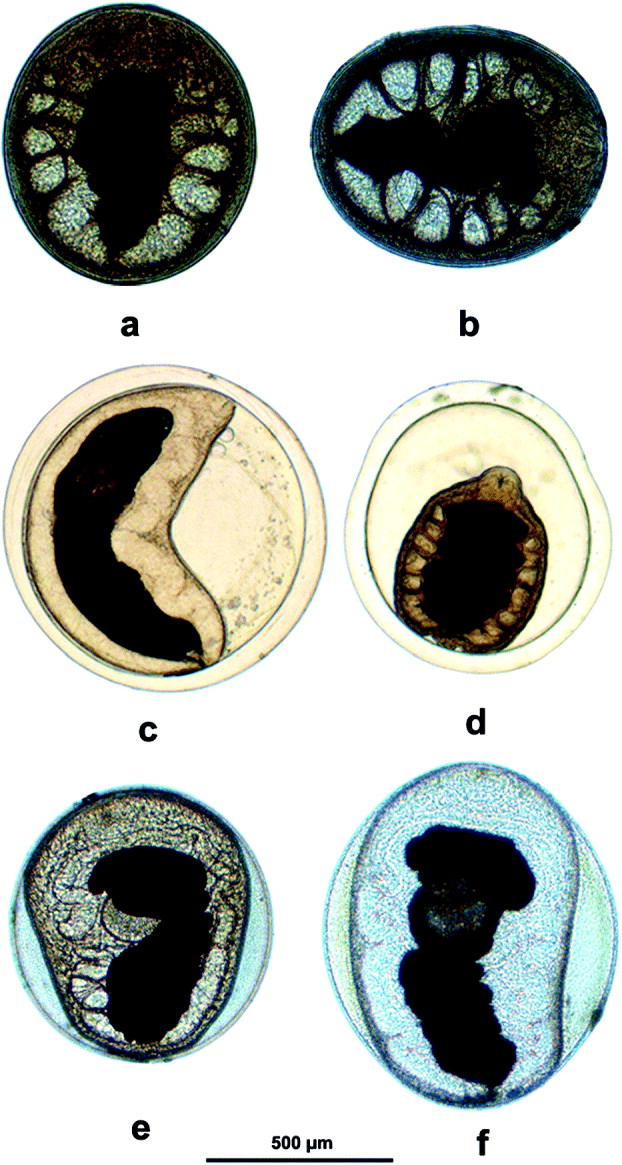
Fig. 2. (a–f) Photomicrographs of metacercariae of Paragonimus westermani complex. (a and b) thin-walled ovoid metacercariae (type 1); (c and d) thick-walled and spherical metacercariae (type 2) from the fresh water crab, Maydelliathelphusa lugubris; (e and f) thin-walled ovoid metacercariae (type 3) from the fresh water crab, Sartoriana spinigera.
Table 1. Dimensions of three types of metacercariae belonging to the Paragonimus westermani complex recovered from the freshwater crab species Maydelliathelphusa lugubris and Sartoriana spinigera from Arunachal Pradesh and Assam, India

Type 1 (Fig. 2a and b) (previously described by Devi et al. (Reference Devi, Narain, Agatsuma, Blair, Nagataki, Wickramasinghe, Yatawara and Mahanta2010)). Ovoid, measuring 724·2 × 598·0 μM: inner cyst wall thickness 9·9 ± 3·5 μM (4·6–16·3 μM): worm occupies entire space within cyst.
Type 2 (Fig. 2c and d): spherical, measuring about 801 μM in diameter: cyst wall very thick, 53·6 ± 12·5 μM (28·4–89·0 μM): worm does not occupy entire space within cyst.
Type 3 (Fig. 2e and f) found in S. spinigera from Assam: Ovoid, about 916 × 771 μM and thin-walled, but, unlike type 1, the worm does not occupy the entire space within the cyst.
Prevalence and intensity of metacercariae in crabs
A total of 2548 M. lugubris and 131 S. spinigera crabs were collected from April 2009 to May 2011 from various localities in Arunachal Pradesh and Assam. Table 2 gives the prevalence and intensity of infection by the types of metacercariae in the crab hosts. The prevalence of type 1 metacercariae in Arunachal Pradesh varied from 3·6 to 7·1% and in Assam it was 0·3 to 4%: the mean intensity of infection was 7·25 and 48·6 per infected crab for Arunachal Pradesh and Assam, respectively. The prevalence of type 2 varied from 0·8 to 24·2% and 0·2 to 5·3% in Arunachal Pradesh and Assam, respectively, and the mean intensity of infection was 10·1 in Arunachal Pradesh and 1·0 in Assam. The prevalence of type 3 was 2·3% (n = 131 crabs): the mean intensity of infection was 1·7 per infected crab.
Table 2. Prevalence and intensity of three types of metacercariae belonging to the Paragonimus westermani complex collected from the freshwater crab species Maydelliathelphusa lugubris and Sartoriana spinigera from Arunachal Pradesh and Assam, Indian

* Metacercariae recovered from hepatopancreas and muscles.
† Metacercariae recovered from gills.
Development of adult worms
Experimental infections of rats using type 2 metacercariae were unsuccessful. However, adult worms did develop from type 1 metacercariae – in total, 41 adults were recovered from 4 rats. The overall worm recovery rate was 48·8% (range 33·3 to 72·2%). These worms were similar in morphology to those reported by Devi et al. (Reference Devi, Narain, Agatsuma, Blair, Nagataki, Wickramasinghe, Yatawara and Mahanta2010) (Fig. 3). Adult worms varied from 5·9 to 7·4 mm in length from 3·7 to 4·7 mm in width. The ovary was typically 6 lobed and testes had 4 to 5 irregular lobes. The worm body was covered by single spines.
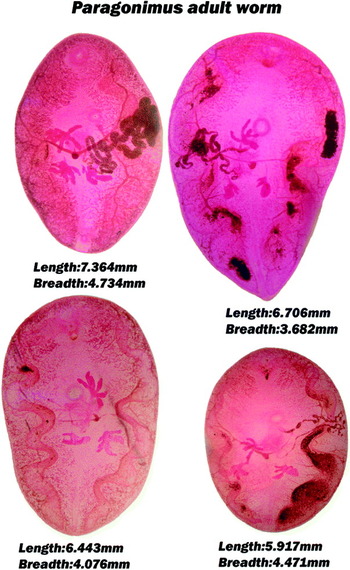
Fig. 3. Paragonimus adult worms developed in experimental Wistar rats from thin-walled ovoid metacercariae (type 1).
Genetic differences and phylogenetic analysis
The final length of the aligned sequences was 300, 291, 1205 & 1212 for cox1, ITS2, 28S and concatenate data sets, respectively. Table 3 gives an indication of genetic differences between the different genotypes and species of genus Paragonimus based on p-distances. Phylogenetic trees based on maximum likelihood and Bayesian analyses (Figs 4–7) differ somewhat in the arrangement of forms within the P. westermani complex. However, they demonstrate very clearly that the lung flukes having type 2 metacercariae are very close to populations of P. westermani from E Asia (Japan, Korea, China and Vietnam), populations that are often relatively derived in phylogenetic trees. In contrast, forms which have type 1 metacercariae represent a more basal lineage of lung flukes in most trees. Type 3 metacercariae from S. spinigera formed a strongly supported separate clade along with P. siamensis from Sri Lanka and Thailand. These relationships are apparent in all the trees inferred from cox1, ITS2 and 28S sequences, and the concatenated sequences.

Figs. 4–7. Maximum likelihood (ML) (left side of each figure) and Bayesian (right) phylogenetic trees inferred from each alignment (cox1, ITS2 and 28S) and from concatenated sequences of all these genes. Figure 4 is based on partial cox1 sequences, Fig. 5 on ITS2 sequences, Fig. 6 on partial 28S sequences and Fig. 7 on alignments containing concatenations of all three regions for the 7 newly sequenced individuals for which these were available. Numbers at nodes represent bootstrap support values (ML) or posterior probabilities (Bayesian). Three-letter codes are used for country names: CHN, China; ECU, Ecuador; IND, India; JPN, Japan; LKA, Sri Lanka; MYS, Malaysia; PHL, Philippines; THA, Thailand; VNM, Vietnam. Bold Accession numbers represent new sequences obtained during the present study.
Table 3. p-distances among concatenated (28S+ cox1 + ITS2) gene sequences of Paragonimus species

DISCUSSION
Despite the conservative nature of adult morphology within P. westermani sensu lato, there is considerable variation at the molecular level (Table 3). P-distance values between some members of the P. westermani group are as great as between very distinct species. For example, P. westermani from China (HM172631) and Indian type 1 sequences are as distant from one another as are P. miyazakii and P. heterotremus (Table 3). The morphology of metacercarial cysts also varies considerably within the complex. Cysts of populations from eastern Asia (Japan, China, Korea), are spherical, <300–500 μM (depending on ploidy and locality) with a relatively thick inner cyst wall (∼20 μM) and a juvenile worm that fully occupies the cyst (Miyazaki, Reference Miyazaki, Sasa, Tanaka and Kurihara1974). Metacercariae from Sri Lanka and NE India yielding adult worms morphologically identical with P. westermani are generally ovoid, around 724 μM in length and with an inner cyst wall about 9 μM thick (Devi et al. Reference Devi, Narain, Agatsuma, Blair, Nagataki, Wickramasinghe, Yatawara and Mahanta2010). In these, the metacercaria occupies the entire cyst cavity. We now have 2 more types of metacercariae belonging to the P. westermani complex from India. The thick-walled spherical type 2 metacercaria described in the present study is very close to P. westermani from the Far East based on molecular sequences and phylogenetic analysis. This was reconfirmed by sequencing 21 metacercariae individually. It is pertinent to mention that morphologically similar metacercariae from Thailand have been reported by Yaemput et al. (Reference Yaemput, Dekumyoy and Visiassuk1994) as belonging to P. siamensis. However, the morphology of the metacercariae of P. siamensis from Thailand as described by Miyazaki and Wykoff (Reference Miyazaki and Wykoff1965) and from Sri Lanka as described by Kannangara and Karunaratne (Reference Kannangara and Karunaratne1969) are different from the morphology of P. siamensis metacercaria as given by Yaemput et al. (Reference Yaemput, Dekumyoy and Visiassuk1994). On the other hand the morphology of metacercariae of P. siamensis from India (type 3 in the present study) resemble metacercariae of P. siamensis from Thailand and Sri Lanka as described by Miyazaki and Wykoff (Reference Miyazaki and Wykoff1965) and Kannangara and Karunaratne (Reference Kannangara and Karunaratne1969), respectively. That the type 3 metacercaria belongs to P. siamensis is clearly confirmed by phylogenetic analysis using ITS2, 28S and cox1 gene sequences.
Two sympatric forms referable to P. westermani on adult morphology but differing in metacercarial morphology and infectivity to cats have been reported from Thailand (Sugiyama et al. Reference Sugiyama, Morishima, Binchai, Rangsiruji and Ketudat2007). In this case, differences between the metacercariae were primarily in size: both were spherical cysts with thick walls and a juvenile worm that filled the inner space. Molecular data from these two types placed them far apart within the P. westermani complex (Binchai et al. Reference Binchai, Rangsiruji, Ketudat, Morishima and Sugiyama2007), a situation comparable with the one reported here. It is intriguing that Miyazaki (Reference Miyazaki1981) noted at least 2 morphological types of Paragonimus metacercariae in the Philippines that yielded identical adult worms (P. westermani) in experimental hosts. No molecular study comparing the Philippine metacercarial variants has been done.
The thick-walled spherical metacercariae (type 2) could not develop in experimental Wistar rats in contrast to thin-walled ovoid metacercariae (type 1) which could develop into adult flukes. Paragonimus westermani in eastern Asia does not easily develop to adulthood in rats, however, populations from elsewhere (e.g. Philippines) can develop in rats (Blair et al. Reference Blair, Xu and Agatsuma1999).
Until recently, a relatively simple biogeographical scenario seemed sufficient to explain the distribution of the P. westermani complex: the complex arose in southern Asia, perhaps in what is now Sri Lanka and, radiated as populations, were established further north and east in Asia, utilizing different snail hosts within the Cerithioidea. This was articulated in part in Blair et al. (Reference Blair, Davis and Wu2001). However, the presence of pairs of distantly related members of the complex in the same place, as in Thailand and NE India, is not easily explained by such a scenario. A particular difficulty is the presence in both NE India and E Asia of forms that are phylogenetically very similar. It is now clear that the complex offers more taxonomic and nomenclatural problems than previously thought. Ad hoc theories invoking particular routes of dispersal are probably best avoided until more is known. The identities of snail hosts of members of the complex across the range are incompletely known and may reflect and illuminate the evolution of this group of lung flukes.
Human infections due to any member of the P. westermani complex are not known from India. The presence of a member of the complex in NE India that is phylogenetically close to the pathogenic forms from eastern Asia implies that we should be alert for human cases in India. However, they are closest to the Vietnamese form of P. westermani which is not known to infect humans (Doanh et al. Reference Doanh, Dung do, Thach, Horii, Shinohara and Nawa2011). At present, only P. heterotremus is known to cause human disease in India (Devi et al. Reference Devi, Narain, Bhattacharya, Negmu, Agatsuma, Blair, Wickramashinghe and Mahanta2007; Singh et al. Reference Singh, Sugiyama, Umehara, Hiese and Khalo2009).
ACKNOWLEDGEMENTS
The award of a JSPS (RONPAKU) Ph.D. Fellowship to K.R.D. (ID Number: IND-10802) is greatly appreciated. The authors thank Mr S. K. Rajguru and Mr Mrinmoy Batshya for their assistance during this work. The Indian Council of Medical Research supported this study vide letter No. 49/7/RMRC/07-NCD-II dated 14th November, 2007. Authors’ contributions: K.R.D., K.N., J.M., D.B. and T.A. conceptualised and designed the study and drafted the manuscript; K.R.D., K.N., S.P.S. and T.N. carried parasitological investigation, DNA sequencing and field work; K.R.D., K.N., T.N. and D.B. carried out phylogenetic analysis. All authors read and approved the final manuscript. T.A. and J.M. are guarantors of the paper. Competing interests: The authors declare that they have no competing interests.