Introduction
With more than 700 species, Eucalyptus is a wide plant genus originating almost exclusively from Australia where these trees are key component for many natural ecosystems (Ladiges et al., Reference Ladiges, Udovicic and Nelson2003). Following James Cook expeditions, several species of Eucalyptus were described for the first time in the late 18th century (Doughty, Reference Doughty2000). As a consequence of their interest for timber production or ornamental purposes, eucalypts were then introduced in more than 90 countries worldwide and, at present, almost 20 million hectares of Eucalyptus are cultivated in the world, mainly in Brazil, India and China (Doughty, Reference Doughty2000; Iglesias Trabado & Wilstermann, Reference Iglesias Trabado and Wilstermann2008). Current plantations are dominated by nine species among which some (e.g. E. globulus) spread beyond cultivated areas and became invasive (Gordon et al., Reference Gordon, Flory, Cooper and Morris2012).
In Australia, up to 20,000 species of phytophagous insects are associated with Eucalyptus among which the most abundant guilds are leaf feeders (Coleoptera and Lepidoptera) and sapsuckers (Hemiptera, Psyllidae) (Majer et al., Reference Majer, Recher, Wellington, Woinarski, Yen, Williams and Woinarski1997). Several species of these guilds are now worldwide invaders such as the psyllids Ctenarytaina spp., Glycaspis brimblecombei Moore and Blastopsylla occidentalis Taylor, as well as the eucalyptus snout beetles Gonipterus spp. (Curculionidae). Gall-inducers are also main pests for Eucalyptus species (Paine et al., Reference Paine, Steinbauer and Lawson2011; Hurley et al., Reference Hurley, Garnas, Wingfield, Branco, Richardson and Slippers2016). Contrary to the Holarctic region where most of gall-inducers are gall midges (Diptera, Cecidomyiidae) and cynipid gall wasps (Hymenoptera, Cynipidae) (Csoka et al., Reference Csoka, Stone, Melika, Raman, Schaefer and Withers2005; Yukawa & Rohfritsch, Reference Yukawa, Rohfritsch, Raman, Schaefer and Withers2005), gall-inducers associated with Eucalyptus trees in Australia are chalcid wasps (Chalcidoidea), and especially species from the family Eulophidae, the largest family among chalcidoid wasps (Noyes, Reference Noyes2002). Since Eulophid gall wasps represent a major radiation in Australia (Austin et al., Reference Austin, Yeates, Cassis, Fletcher, La Salle, Lawrence, McQuillan, Mound, Bickel, Gullan, Hales and Taylor2004), it is not surprising that some of those developing on eucalypts became invasive recently such as Epichrysocharis burwelli Schauff, Leptocybe invasa Fisher & La Salle, Moona spermophaga Kim & La Salle, Leprosa milga Kim & La Salle and Selitrichodes globulus La Salle & Gates (Schauff & Garrison, Reference Schauff and Garrison2000; Mendel et al., Reference Mendel, Protasov, Fisher and La Salle2004; Kim et al., Reference Kim, McDonald and La Salle2005; Kim & La Salle, Reference Kim and La Salle2008; La Salle et al., Reference La Salle, Arakelian, Garrison and Gates2009). Other Eulophid pests belong to the genus Ophelimus.
Firstly described by Haliday (Reference Haliday1844), this genus belongs to the subfamily Opheliminae and contains almost 50 known species, mainly native from Australia (Bouček, Reference Bouček1988; Burks et al., Reference Burks, Heraty, Gebiola and Hansson2011). The precise biology of most species is unknown but all may be associated with galls, and probably as gall-inducers (La Salle, Reference La Salle, Raman, Schaefer and Withers2005). Ophelimus eucalypti (Gahan) was the first invasive species reported outside its native area. In New Zealand, it was reported to induce economic damage on Eucalyptus species of both the Transversaria and Maidenaria sections and this pest may have contributed to the decline of some Eucalyptus species (Withers et al., Reference Withers, Raman and Berry2000; Withers, Reference Withers2001). Currently, the most largely distributed invader is however Ophelimus maskelli (Ashmead). Originally described by Ashmead in 1900 as Pteroptrix maskelli, it was transferred to the genus Ophelimus by Bouček in 1988. Outside its native area, O. maskelli was firstly reported in Italy (Arzone & Alma, Reference Arzone and Alma2000) and in Spain (Pujade-Villar & Riba-Flinch, Reference Pujade-Villar and Riba-Flinch2004), where it has been misidentified as O. eucalypti (Protasov et al., Reference Protasov, La Salle, Blumberg, Brand, Saphir, Assael, Fisher and Mendel2007b). From then, it rapidly disseminated throughout the Mediterranean region: Israel (Mendel et al., Reference Mendel, Protasov, Saphir, Brand, Assale, Blumberg, La Salle, Bento, Miranda-Arabolaza and Pereira2005), Greece (Kavallieratos et al., Reference Kavallieratos, Kontomidas, Anagnou-Veroniki and Emmanuel2006), France (EPPO, 2006), Turkey (Doğanlar & Mendel, Reference Doğanlar and Mendel2007), Portugal (Branco et al., Reference Branco, Boavida, Durand, Franco and Mendel2009), Tunisia (Dhahri et al., Reference Dhahri, Ben Jamaa and Lo Verde2010), Algeria (Caleca, Reference Caleca2010), Malta (Mifsud, Reference Mifsud2012) and probably in the UK (Tilbury & Jukes, Reference Tilbury and Jukes2006). It has also been recently reported from Indonesia, South Africa, Vietnam, Mauritius and, for the first time in the Nearctic Region, in California (Lawson et al., Reference Lawson, Griffiths, Nahrung, Noack, Wingfield, Wilcken, Slippers, Lo, Thu, Lee, Lelana, Ketphanh, Zhou and Eungwijarnpanya2012; Burks et al., Reference Burks, Mottern, Waterworth and Paine2015a). Males of O. maskelli have only been mentioned by Ashmead (Reference Ashmead1900) in its original description. However, in all the invaded areas, this species seems to be represented only by females that thus reproduce by thelytoky. The pervasiveness of asexuality in invasive strains has been repeatedly observed for other Hymenoptera for which both sexual and asexual reproductions coexist in the native areas (see Fusu (Reference Fusu2017) for details). This pattern may be explained by the increased probability of establishment of asexual individuals in new environments (absence of Allee effects linked to the necessity of meeting and mating). The biology of O. maskelli was studied by Protasov et al. (Reference Protasov, La Salle, Blumberg, Brand, Saphir, Assael, Fisher and Mendel2007b). Females are able to oviposit around 100 eggs on midribs of leaves of several Eucalyptus species among which E. camaldulensis Dehnh. seems to be the most common host. In the case of high population levels, the entire leaf surface can be covered with galls. O. maskelli develops on a single-cell gall and its entire development (from oviposition to adult emergence) takes around 90 days in ventilated greenhouses (temperature: 18–25°C) and, according to the local climatic conditions, several generations can be completed within a year (e.g. three generations in Israel, Protasov et al., Reference Protasov, La Salle, Blumberg, Brand, Saphir, Assael, Fisher and Mendel2007b).
As for other invasive species (Simberloff, Reference Simberloff2009), the eradication of O. maskelli in particular is almost impossible once established. Since part of its success as invader may be due to the absence of natural enemies (enemy release hypothesis: Torchin et al., Reference Torchin, Lafferty, Dobson, McKenzie and Kuris2003; Colautti et al., Reference Colautti, Ricciardi, Grigorovich and Maclsaac2004), its regulation may nevertheless be achieved through classical biological control, i.e. the deliberate introduction of an adapted natural enemy for its permanent establishment and the long-term control of the target pest (Eilenberg et al., Reference Eilenberg, Hajek and Lomer2001). Closterocerus chamaeleon (Girault), a chalcid wasp belonging to the family Eulophidae and the subfamily Entedoninae, was hence identified as relevant candidate for biological control. In its native range, it is indeed widely distributed and mostly reported to parasitize O. maskelli on Eucalyptus species although it was found associated with other gall-inducing Eulophids on the same host-plants (Protasov et al., Reference Protasov, Blumberg, Brand, La Salle and Mendel2007a). Biological control programmes using C. chamaeleon were thus implemented in Israel and in Italy where it successfully established (Mendel et al., Reference Mendel, Protasov, Blumberg, Brand, Saphir, Madar and La Salle2007; Caleca et al., Reference Caleca, Lo Verde, Rizzo and Rizzo2011). The high dispersal ability of this parasitoid observed in these countries was confirmed by its quick spread in other neighbouring countries without reported intentional releases: Turkey (Doğanlar & Mendel, Reference Doğanlar and Mendel2007), Spain (Borrajo et al., Reference Borrajo, Lopez, Ocete, Lopez and Ruiz2008), Portugal (Branco et al., Reference Branco, Boavida, Durand, Franco and Mendel2009), Algeria (Caleca, Reference Caleca2010), Tunisia (Lo Verde et al., Reference Lo Verde, Dhahri and Ben Jamaa2010) and France (Borowiec et al., Reference Borowiec, Thaon, Brancaccio, Warot, Ris and Malausa2012). In only a few years, C. chameleon extremely reduced the populations of O. maskelli in Israel (Protasov et al., Reference Protasov, Blumberg, Brand, La Salle and Mendel2007a), Italy (Caleca et al., Reference Caleca, Lo Verde, Rizzo and Rizzo2011), Tunisia and Portugal (Branco et al., Reference Branco, Dhahri, Santos and Ben Jamaa2014).
Within this context of an overall control of O. maskelli by C. chamaeleon, severe damages on foliage were nevertheless reported on Eucalyptus plantations located in South-eastern France. First observations indicated that the gall-inducer should belong to the genus Ophelimus but both the galls’ shape as well as the intensity of damages were intriguing.
From these observations, we thus decided to more precisely investigate the identity of Ophelimus species present in different Mediterranean countries using simultaneously molecular, morphological and bio-ecological information.
Materials and methods
Wasp samplings
All the specimens used in this study were collected on Eucalyptus species.
Field sampling were conducted between 2010 and 2012 in South-eastern France (Alpes-Maritimes, Bouches-du-Rhône and Var) and Western Italy (Liguria) and, in 2013, in Portugal (in a Eucalyptus arboretum in Tapada da Ajuda, Lisbon). To collect living specimens of Ophelimus, small branches with mature galls were collected and placed in small plastic bags at INRA Sophia Antipolis (for samples collected in France and Italy), and at Laboratory of Forest Entomology of Instituto Superior de Agronomia, Lisbon (for samples collected in Portugal). Emergences were checked daily and all emerged adults were killed in 95% ethanol to allow molecular and morphological characterizations. The presence of parasitoids in the samples was checked to provide information on natural parasitism.
We also used additional Ophelimus specimens kept in 95% ethanol and collected in the native area of this genus: Australia (dry specimens from the Australian National Insect Collection, Canberra) and New Zealand (from North and South Islands). Detailed information about specimens used in this study is given in table 1.
Table 1. Information about specimens used in this study.
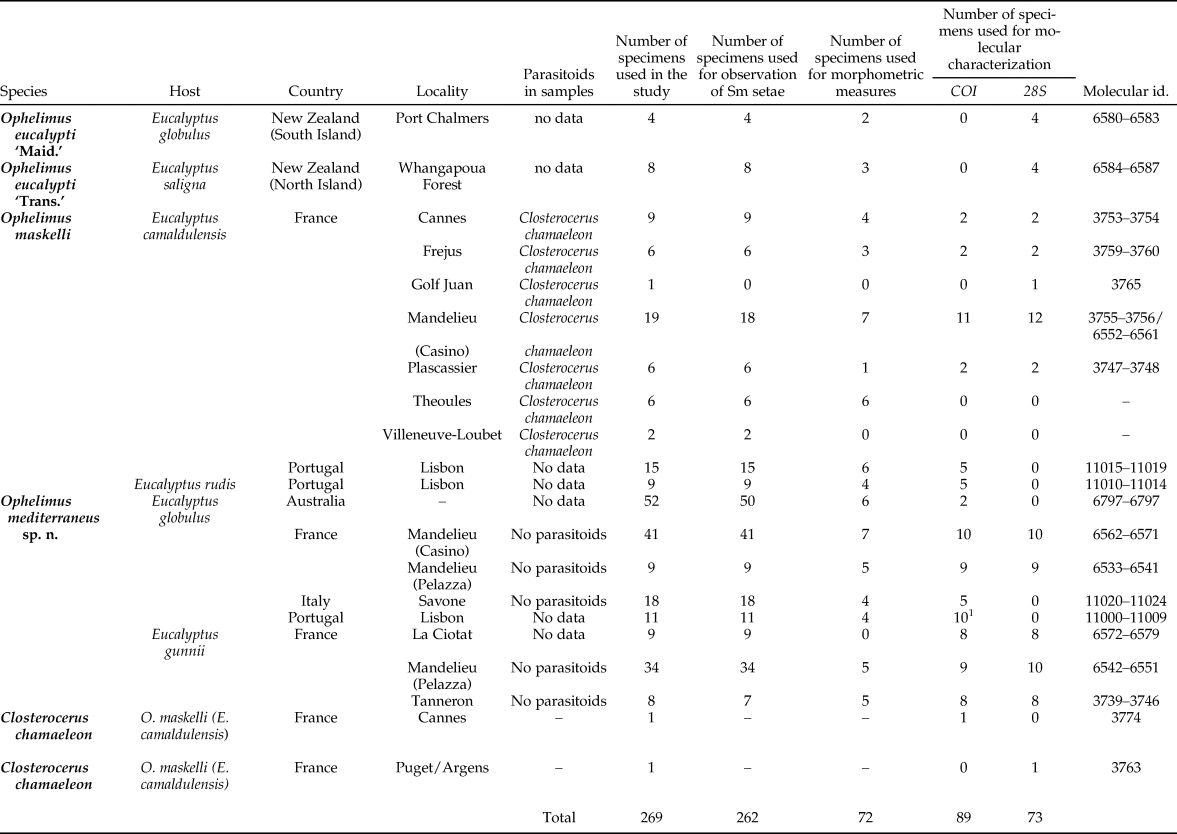
1 For two specimens, PCR failed; one was contaminated (blast with Penicillium).
Molecular characterization
Two molecular markers were used: the cytochrome oxidase subunit I (COI – mitochondrial coding marker) and the 28s rDNA region (28S – nuclear non-coding marker). Among all the Ophelimus specimens collected in the field, 99 were selected for molecular characterization in order to adequately cover geographical and host-plant ranges. Two specimens of C. chamaeleon collected in France on O. maskelli were also selected and used as outgroup (information about molecular methods used for each specimen is given in table S1).
DNA extraction
Genomic DNA was extracted from frozen (individuals previously kept in 95% ethanol at −20°C) tissues using a prepGEM® Insect kit (ZYGEM, PIN0500). In order to allow subsequent examination of morphology, specimens were placed individually in different extraction volumes (methods ‘zygem3 h_20 µL’, ‘zygem3 h_30 µL’, ‘zygem3 h_40 µL’; table S2) of mix without destruction of the specimens and incubated 3 h at 75°C. The DNA extracts were then stored at −20°C. For specimens collected in Australia that were kept dry for more than 10 years, we decided to use a destructive method to extract sufficient quantity of DNA (methods ‘Chitinase_QiaBT’ and ‘Chitinase_QiaAmp’; table S2).
Amplification by polymerase chain reaction (PCR)
The COI barcoding fragment was amplified using either the primers LCO1490 (5′-GTCAACAAATCATAAAGATATTGG-3′) and HCO2198 (5′-TAAACTTCAGGGTGACCAAAAAATCA-3′) (Folmer et al., Reference Folmer, Black, Hoeh, Lutz and Vrijenhoek1994) or LCO1490puc (5′-TTTCAACWAATCATAAAGATATTGG-3′) and HCO2198puc (5′-TAAACTTCWGGRTGWCCAAARAATCA-3′) (Cruaud et al., Reference Cruaud, Jabbour-Zahab, Genson, Cruaud, Couloux, Kjellberg, van Noort and Raspus2010).
The 28s gene was amplified using the primers D2-3551F (5′-CGTGTTGCTTGATAGTGCAGC-3′) and D2-4057R (5′-TCAAGACGGGTCCTGAAAGT-3′) (Gillespie et al., Reference Gillespie, Munro, Heraty, Yoder, Owen and Carmichael2005). Detailed PCR conditions are described in table S3. All the PCRs were performed on a GeneAmp 9700 thermocycler. PCR products were visualized using the QIAxcel Advanced System and QIAxcel DNA Fast Analysis Kit (Qiagen, Germany). They were sent to Genoscreen (Lille, France) or to Beckman Coulter Genomics (Stansted, UK) for sequencing in both directions.
Morphological characterization
Terminology of morphological characters follows Gibson et al. (Reference Gibson, Huber and Woolley1997). Except for the study published by Girault (Reference Girault1913c) that included only eight species, no useful identification key is available for the genus Ophelimus. However, according to Protasov et al. (Reference Protasov, La Salle, Blumberg, Brand, Saphir, Assael, Fisher and Mendel2007b), O. maskelli can be discriminated from other Ophelimus species by the presence of only one seta on the submarginal vein of forewings. Using a Leica M205C microscope (×140 magnification), we thus investigated the number of submarginal setae on forewings of a total of 262 specimens of Ophelimus collected on various species of Eucalyptus (E. camaldulensis, E. globulus Labill., E. gunnii Hook., E. rudis Endl., E. saligna Sm.) and in different countries (Australia, France, Italy, New Zealand, Portugal) (table 1).
For 72 of these 262 specimens (all females), we also did measurements of five morphological characters. Since adults of Ophelimus tend to collapse, in particular after storage in alcohol, we only used characters from wings and from hind legs: length of hind tibia (T3L), length of forewing (FwL), length of forewing submarginal vein (SmvL), length of forewing marginal vein (MvL) and length of forewing stigma vein (StvL). The end of forewing post-marginal vein was difficult to locate unambiguously and since this character seems to present wide interspecific variation (Bouček, Reference Bouček1988), we did not measure this vein. To avoid variation due to asymmetry, we did all these measurements and observations on left hand side of the specimens. For this work, all left forewings and left hind tibias were removed for all specimens and embedded in Hoyer's medium on slides prior to measurements using a Leica M205C microscope coupled with image processing software Leica Application Suite version 4.0 (Leica Microsystems GmbH) (fig. 1). Images presented in fig. 9 were taken using a Keyence VHX-5000 digital microscope, available at INRA CBGP, Montpellier.

Fig. 1. O. mediterraneus: measurements of forewings (a) and hind tibia (b) for morphometrics analysis. T3L: hind tibia length; FwL: forewing length; SmvL: submarginal vein length; MvL: marginal vein length; StvL: stigmal vein length.
Bio-ecological investigations
We investigated the size of galls induced by both O. maskelli and O. mediterraneus sp. n. by collecting leaves with fully developed galls on E. camaldulensis and E. globulus respectively. Since the presence of galls on some species of Eucalyptus seems to be seasonal (Borowiec et al., Reference Borowiec, Brancaccio, Thaon, Warot, Branco, La Salle, Ris and Malausa2013), leaves with mature galls were collected in May 2011 in Mandelieu (France) and in the arboretum of Lisbon in April 2013 (Portugal). Diameter of galls was measured on an x–y basis. Measurements were performed:
(i) using a Leica M205C stereomicroscope (100× magnification) coupled with Leica Application Suite software (version 4.0) for galls collected in France: E. camaldulensis (100 galls); E. globulus (95 galls) and
(ii) using an Olympus SZX-ZB12 stereomicroscope (80× magnification) equipped with ocular micrometre for galls collected in Portugal: E. camaldulensis (100 galls); E. globulus (100 galls).
We also investigated the biological cycle of O. mediterraneus sp. n. on E. gunnii by carrying out a field survey in a Eucalyptus plantation in Mandelieu (Pelazza) between January 2011 and January 2012. Eight samples were collected in 2011 (January, March, April, May, June, August, October and December) and one sample was collected in January 2012. For each of these samples, 20–30 leaves were randomly collected on young trees of E. gunnii. Leaves were brought to the laboratory where galls were dissected using a Leica M205C microscope. Gall's content was categorized as follows:
• ‘larva’: Ophelimus larva was observed feeding within the gall;
• ‘nymph’: nymph of Ophelimus was observed within the gall;
• ‘adult’: adult of Ophelimus was observed;
• ‘exit holes’: the gall was empty and characterized by the presence of an adult's exit hole on the surface of the gall;
• ‘empty’: no O. mediterraneus sp. n. inside nor exit holes were visible but, often, some organic remains were observed that were attributed to O. mediterraneus corpse. We used this item to estimate the mortality of this species in natural conditions, even though we were not able to determine its causes (phytotoxicity, predation, viruses, etc.).
Abbreviations: The following abbreviations were used for depositions of voucher specimens (paratypes and holotypes):
• ANIC: Australian National Insect Collection, Canberra (Australia)
• BNHM: British Natural History Museum, London (UK)
• MNHN: Muséum National d'Histoire Naturelle, Paris (France)
• NMNH: National Museum of Natural History, Washington (USA)
• UCRC: University of California, Riverside Entomology Research Museum (USA)
Data analysis
Phylogenetic reconstruction
Sequences were aligned using MEGA 7 (Kumar et al., Reference Kumar, Stecher and Tamura2016). Alignments were corrected manually and, for COI, sequences were translated to amino acids to detect frame-shift mutations and premature stop-codons that may indicate the presence of pseudogenes. Sequences were analysed using the neighbour-joining method and the Kimura's two-parameters evolutionary model (Kimura, Reference Kimura1980). Bootstrap support was evaluated with 1000 replicates starting with a random tree.
Morphological characterization
To analyse morphometric measures, we used multivariate ratio analysis (MRA; Baur & Leuenberger, Reference Baur and Leuenberger2011) that allows the interpretation of results from principal component analysis (PCA) and linear discriminant analysis (LDA) in terms of body ratios. These tools have been recently used to disentangle cryptic species complex in several groups of hymenoptera (Laszlo et al., Reference Laszlo, Baur and Tothmeresz2013; Baur et al., Reference Baur, Kranz-Baltensperger, Cruaud, Rasplus, Timokhov and Gokhman2014; Fusu, Reference Fusu2017; Gebiola et al., Reference Gebiola, Monti, Johnson, Wooley, Hunter, Giorgini and Pedata2017). According to Baur & Leuenberger (Reference Baur and Leuenberger2011), we first defined an isometric size axis (isosize), calculated as the geometric mean of all variables, and then performed a shape PCA (i.e. a PCA in the space of all ratios) to evaluate how well the morphometric pattern fitted groups obtained with molecular analysis. The most important shape components are then plotted against isosize axis to visualize the correlation between size and shape and evaluate data allometry. We also performed a PCA ratio spectrum – a graphical tool that aims to explain shape PCA components in term of body ratios – and an allometry ratio spectrum that allows checking allometry behaviour of characters used for calculation of ratios. Finally we used the LDA ratio extractor to find ratios that best discriminate groups previously identified by shape PCA.
We performed statistical analyses in R version 3.3.3 (R Core Team, 2017) using R-scripts provided by Baur & Leuenberger (Reference Baur and Leuenberger2011). Because of the very low number of individuals (five), specimens of O. eucalypti were not included in the analyses.
Gall size
Area of galls induced by both O. maskelli and O. mediterraneus sp. n. was estimated using both radii measured on an x–y basis with the formula of an ellipse area (Area = π × r1 × r2, with r1 the longest radius and r2 the smallest radius). After an exploratory step, we decided to fit linear model (function lm) using the galls’ area as the dependent variable and the species of Ophelimus and the country as possible explanatory variables. A transformation (1/sqrt(x)) was applied to the dependent variable to reach homogeneity of variances. We then applied a two-way analysis of variance to the model.
Results
Molecular characterization
Among the 99 specimens of Ophelimus, 61 were used for both COI and 28S, 27 were used only for COI and 11 were used only for 28S. For 28S, the amplification was successful for all the specimens, the 72 sequences of Ophelimus ranging from 550 to 609 bp. For COI, three sequences were not amplified so that we obtained 85 sequences of Ophelimus ranging from 540 to 583 bp. For both COI and 28S, the minimum interspecific distance exceeds the maximum intraspecific distance (table 2).
Table 2. Estimates of evolutionary divergence between sequences (COI and 28S) expressed as mean number of base substitutions per site between sequences × 100 (min–max) using Kimura two-parameters model. The analysis involved 87 nucleotide sequences for COI and 74 nucleotide sequences for 28S.

Both phylogenetic analyses confirmed the presence of two distinct clusters of Ophelimus in Europe (figs 2 and 3). The first one encompasses individuals collected in several localities of South-eastern France, in Italy and in Portugal as well as previously identified O. maskelli collected in Italy by Burks et al. (Reference Burks, Heraty, Gebiola and Hansson2011) (accession number: HM365046 for COI and HM364944 for 28S). In our samples, O. maskelli was recovered on two different species of Eucalyptus belonging to Exertaria section (E. camaldulensis and E. rudis). The intra-cluster distance in O. maskelli is 0.0% for 28S, and ranged from 0.0 to 1.7% for COI (table 2).

Fig. 2. Neighbour-joining tree figuring relationships between Ophelimus species based on the nuclear gene 28S. C. chamaeleon is used as outgroup. Kimura two-parameters analysis with 1000 bootstrap replicates. Each line represents a sequenced individual with information in the following order: molecular code, molecular marker, species (host species), country and (locality).

Fig. 3. Neighbour-joining tree figuring relationships between Ophelimus species based on the mitochondrial gene COI. C. chamaeleon is used as outgroup. Kimura two-parameters analysis with 1000 bootstrap replicates (supports >70% are indicated at nodes). Each line represents a sequenced individual with information in the following order: molecular code, molecular marker, species (host species), country and (locality).
The second cluster of Ophelimus encompasses individuals collected on E. globulus and E. gunnii (section Maidenaria) in South-Eastern France, in Italy and in Portugal as well as two specimens collected on E. globulus in Western Australia (Victoria state) in 2003 (molecular codes: 6796 and 6797) (figs 2 and 3). This cluster is clearly genetically differentiated from O. maskelli both with COI and 28S. The inter-cluster distance between this species and O. maskelli ranged from 8.1 to 9.6% for COI and from 0.4 to 0.8% for 28S.
Finally, on the 28S tree, we have some other specimens that are differentiated from both previously discussed Ophelimus species (fig. 2). These were collected in New Zealand on E. globulus and E. saligna and were identified as two ‘biotypes’ according to their host-plants: O. eucalypti ‘Maid.’ collected on E. globulus and O. eucalypti ‘Trans.’ collected on E. saligna. For the first one, the interspecific distance is 1% with O. maskelli, and ranged from 1 to 1.4% with O. mediterraneus sp. n. For O. eucalypti ‘Trans.’, interspecific distance ranged from 2.2 to 2.4%, and from 2.2 to 2.8% with O. maskelli and O. mediterraneus sp. n. respectively. Interestingly, the distance between each of these two ‘biotypes’ (ranging from 1.6 to 1.8%) is higher than the interspecific distance found for O. maskelli and O. mediterraneus sp. n., suggesting that these two ‘biotypes’ may be two distinct species.
Morphological investigations
Observations of the submarginal vein of the forewings of 262 specimens of Ophelimus collected on five different Eucalyptus species and in five countries showed results that are congruent with those obtained with molecular tools (fig. 4). Indeed, we confirm that O. maskelli is the only species that presents no variability of this character, having always only one submarginal seta on the forewings, be it developing on E. camaldulensis or E. rudis. On the other hand, O. mediterraneus sp. n. and the two ‘biotypes’ of O. eucalypti present at least two submarginal setae. O. mediterraneus sp. n., collected on E. globulus and E. gunnii in Australia, France, Italy and Portugal present from two to four submarginal setae on the forewings but mostly three (60% of the total number of specimens observed). Interestingly, differences in the number of submarginal setae were found between the two ‘biotypes’ of O. eucalypti: two to four submarginal setae for O. eucalypti ‘Maid.’ and five to six submarginal setae for O. eucalypti ‘Trans.’.

Fig. 4. Number of submarginal setae observed in different species of Ophelimus collected on different species of Eucalyptus (Ecam: E. camaldulensis; Eglob: E. globulus; Egun: E. gunnii, Erud: E. rudis; Esal: E. saligna) and in different countries (Aus: Australia; Ita: Italy; Fra: France; NZ: New-Zealand; Por: Portugal). Numbers in square bracket (top) represent the number of specimens observed. White: one submarginal seta; light grey: two submarginal setae; medium grey: three submarginal setae; dark grey: four submarginal setae; black: >4 submarginal setae.
For the shape PCA, we used only the two first components that accounted for 81% of the total variance (43 and 38% for the first and second component respectively). Specimens were assigned to species based on qualitative characters (number of submarginal setae). The shape PCA showed that O. maskelli and O. mediterraneus sp. n. cannot be separated using the quantitative characters used for this study, with a strong overlap between individuals of these two species. Along the second component, the overlap is also almost total with only two specimens of O. maskelli that are separated from O. mediterraneus sp. n. (not shown). The first component is a bit more discriminant with the separation of five (among 37) O. mediterraneus sp. n. and five (among 30) O. maskelli (fig. 5a). Scatterplot of isosize against first (fig. 5b) and second (not shown) shape PC showed that size ranges of these two species are largely overlapping but O. mediterraneus sp. n. seems on average slightly larger than O. maskelli. Size variation for both species is high.

Fig. 5. Results of MRA for females of O. maskelli and O. mediterraneus sp. n. (a, b) Scatterplot of PCA in shape space: scatterplot of first against second shape PC (a), scatterplot of isosize against first shape PC (b). Symbols: black dots, O. maskelli; grey dots, O. mediterraneus sp. n. (c, d) Ratio spectra: PCA ratio spectrum (c), allometry ratio spectrum (d). Horizontal bars in the ratio spectra represent 68% bootstrap confidence intervals based on 500 replicates.
PCA ratio spectrum (fig. 5c) of the first component of the shape PCA showed that most of the variation along this axis is explained by characters that are lying to the opposite ends of the spectrum (such as ‘SmvL:StvL’) whereas ratios composed of characters lying close to each other in the spectrum (such as ‘FwL:T3L’) contributed very little to this component. The PCA ratio spectrum ranged from −0.41 to 0.45 for the variable coefficients. In the same way, the allometry ratio spectrum (fig. 5d) showed that variables lying close to each other are those showing the lowest amount of allometry (such as ‘MvL:StvL’) whereas the most distant variable are those showing the greatest amount of allometry (such as such as ‘T3L:StvL’).
Finally the LDA ratio extractor gives us the two ratios that best discriminate groups previously identified: ‘FwL:MvL’ and ‘FwL:SmvL’ (table 3). For these two ratios, the theta value is 0.36 and 0.40 respectively, indicating that discrimination between these two species of Ophelimus is mainly due to shape rather than size. However, ranges of the best two ratios are mostly overlapped indicating that they cannot be used to discriminate these two species. This is confirmed by the value of standard distance of these two ratios that are 1.86 and 1.60 respectively. Interestingly, the ratio ‘T3L:FwL’, that is not useful to discriminate between O. maskelli and O. mediterraneus sp. n., may be used to distinguish O. eucalypti ‘Maid.’ from O. eucalypti ‘Trans.’ as the range of this ratio is not overlapping (table 3).
Table 3. Summarized data of body measurements (in μm) for each species of Ophelimus and best ratios.

Bio-ecological investigations
Gall area
Results of gall size measurements showed that both in France and in Portugal, there is a significant effect of the species of Ophelimus (F 1,391 = 781, p < 2e-16) and of the country (F 1,391 = 10.59, p = 0.0012) on galls’ area. Indeed, in both countries, galls induced by O. maskelli (on E. camaldulensis) are larger than those induced by O. mediterraneus sp. n. (on E. globulus) (fig. 6). There is no significant effect of the interaction between ‘species of Ophelimus’ and ‘country’ (F 1,391 = 1.02, p = 0.31).

Fig. 6. Boxplots of gall's area (mm2) induced by O. maskelli (white boxes) and O. mediterraneus sp. n. (grey boxes) in France and Portugal. Boxes represent the 25–75th percentile range and the horizontal line within each box represents the median value. The range lines correspond to the highest and lowest values, with outliers marked with circles.
Biological cycle
A total of 651 galls of O. mediterraneus sp. n. collected on E. gunnii were dissected between January 2011 and January 2012. As shown in fig. 7, the emergence period of O. mediterraneus sp. n. is in late spring–early summer. After, no sign of gall development was visible on leaves collected between August and October. Using a transmitted light source, it was nevertheless possible to observe oviposition marks. Attempts to observe eggs by dissection were however not conclusive. Following this 3–4 months period without sign of gall development, reddish flat marks appeared on leaves in early winter (December) (fig. 8a) with young larval instars of O. mediterraneus sp. n. present inside. This period of early stage of gall development was followed by the development of first galls in January. These galls are brown coloured, ellipsoid shaped, with a crackled surface and are visible only on the upper surface of the leaf (fig. 8b). After (January–March), these fully developed galls were occupied by larval instars but in March around 12% of the total number of the galls were empty indicating mortality of the larvae. In spring, the presence of larval instars became scarce (from 0 to 1.6% of the dissected galls) whereas nymphal instars became predominant (from 88 to 94% of the dissected galls). During this stage, few adults were observed within these galls and the mortality ranged from 3 to 9%. In June, 60% of the total number of dissected galls was characterized by the presence of exit holes of emerged O. mediterraneus sp. n. whereas around 7% of the galls was at the nymphal stage and 7% of the galls contained adults not yet emerged. It is during this emergence period that the mortality rate is the most important with 26% of the total number of the galls being empty.

Fig. 7. Seasonal evolution of the relative frequency (%) of the different ‘life stages’ within dissected galls induced by O. mediterraneus sp. n. on E. gunnii in France. White: ‘larvae’; light grey: ‘nymphs’; dark grey: ‘adults’; black: ‘empty’; hatched: ‘exit holes’.

Fig. 8. Galls of O. mediterraneus sp. n. on E. globulus: (a) newly formed galls and (b) fully developed galls.
Parasitism
C. chamaeleon emerged from the galls induced by O. maskelli on E. camaldulensis in all the samples we collected in France and Portugal indicating that this parasitoid is well established in these countries. On the contrary, no parasitoid emerged from the galls induced by O. mediterraneus sp. n., in France, Italy and Portugal despite suitable conditions and an important sampling (more than 2000 O. mediterraneus emerged).
Species description
Ophelimus Haliday
Ophelimus Haliday, Reference Haliday1844: 300. Type species Eulophus ursidius Walker, designated by Ashmead, 1904: 353.
Chrysoatomus Ashmead, 1904: 342. Type species Chrysoatomus zealandicus Ashmead
Rhicnopeltella Girault, Reference Girault1913a: 109. Type species Rhicnopeltella immaculatipennis Girault
Cirrospilomyia Girault, Reference Girault1913b: 76. Type species Cirrospilomyia magniventris Girault
Brachychrysocharella Girault, Reference Girault1913c: 168. Type species Brachychrysocharella dubia Girault
Omphalomorphella Girault and Dodd, in Girault, Reference Girault1913c: 178. Type species Omphalomorphella auripes Girault
Elachertetrastichus Girault, Reference Girault1913c: 264. Type species Elachertetrastichus purpureus Girault
Omphalomorphoides Dodd, in Girault, Reference Girault1915: 214. Type species Omphalomorphoides violescens Dodd
Deciana Girault, Reference Girault1925: 92. Type species Deciana aenoviridis Girault
Ophelimus mediterraneus Borowiec & Burks, n. sp.
Material examined (all females)
Holotype. France: Bouches-du-Rhône: La Ciotat, jardin, 24.v.2010, H. Dumas [MNHN: UCRCENT00485453], deposited in MNHN (GenBank accession numbers: COI, JX096442; 28S, MH651684).
Paratypes. 34 paratypes with depositions indicated by coden: France: Alpes-Maritimes: Mandelieu, casino; alt: 7 m; 43°31′56″N, 6°55′53″E; 3.v.2011; N. Borowiec [MNHN: UCRCENT00485484, GenBank accession numbers: COI, MH651533; 28S, MH651673 – NMNH: UCRCENT00485485, GenBank accession numbers: COI, JX096435; 28S, MH651675]. Mandelieu, Pelazza; alt: 217 m; 43°33′05″N, 6°54′51″; 3.v.2011; N. Borowiec [MNHN: UCRCENT00485482, GenBank accession numbers: COI, MH651528; 28S, MH651653 – UCRC: UCRCENT00485483, GenBank accession numbers: COI, JX096426; 28S, MH651652]. Bouches-du-Rhône: La Ciotat, jardin; 24.v.2010; H. Dumas [MNHN: UCRCENT00485454–55, GenBank accession numbers: COI, JX096440 and JX096441; 28S, MH651682 and MH651683]. Var: Tanneron, Courrin; 43°35′00″N, 6°50′06″E; 27.iv.2010; N. Borowiec [MNHN: UCRCENT00485456; MNHN: UCRCENT00485464; MNHN: UCRCENT00485466–73; MNHN: UCRCENT00485473–81; BMNH: UCRCENT00485474]. Italy: Savona Prov.: Loano; alt: 15 m; 44°08′34″N, 8°16′05″E; 11.iv.2012 ; M. Thaon [ANIC: UCRCENT00485495–96, GenBank accession numbers: COI, MH651520 and MH651521 – MNHN: UCRCENT00485497, GenBank accession number: COI, MH651522].
Other material examined (not paratypes because locality is uncertain): Australia: Victoria?; 8.x.2003; Z. Mendel [MNHN: UCRCENT00499638; MNHN: UCRCENT00499641].
Etymology. The names mediterraneus refers to the large geographical distribution of this species in the Mediterranean region.
Diagnosis. Mostly with three (from two to four) setae on the submarginal vein dorsally (fig. 1a). Ventral half of face with long setae. Mesoscutum at most only slightly longer than mesoscutellum (mesoscutum 0.93–1.13× mesoscutellum length; Fig. 9c). Otherwise similar to O. maskelli. The mesoscutum in O. maskelli is noticeably longer: about 1.3× the mesoscutellum length, and O. maskelli has only one dorsal submarginal vein seta. O. eucalypti, the other well-known invasive species of the genus, differs in having more than three submarginal vein setae, in having far more callar setae on the propodeum (over five, instead of only two), and in having a body about twice as long (nearly 2 mm, vs. about 1 mm or less).
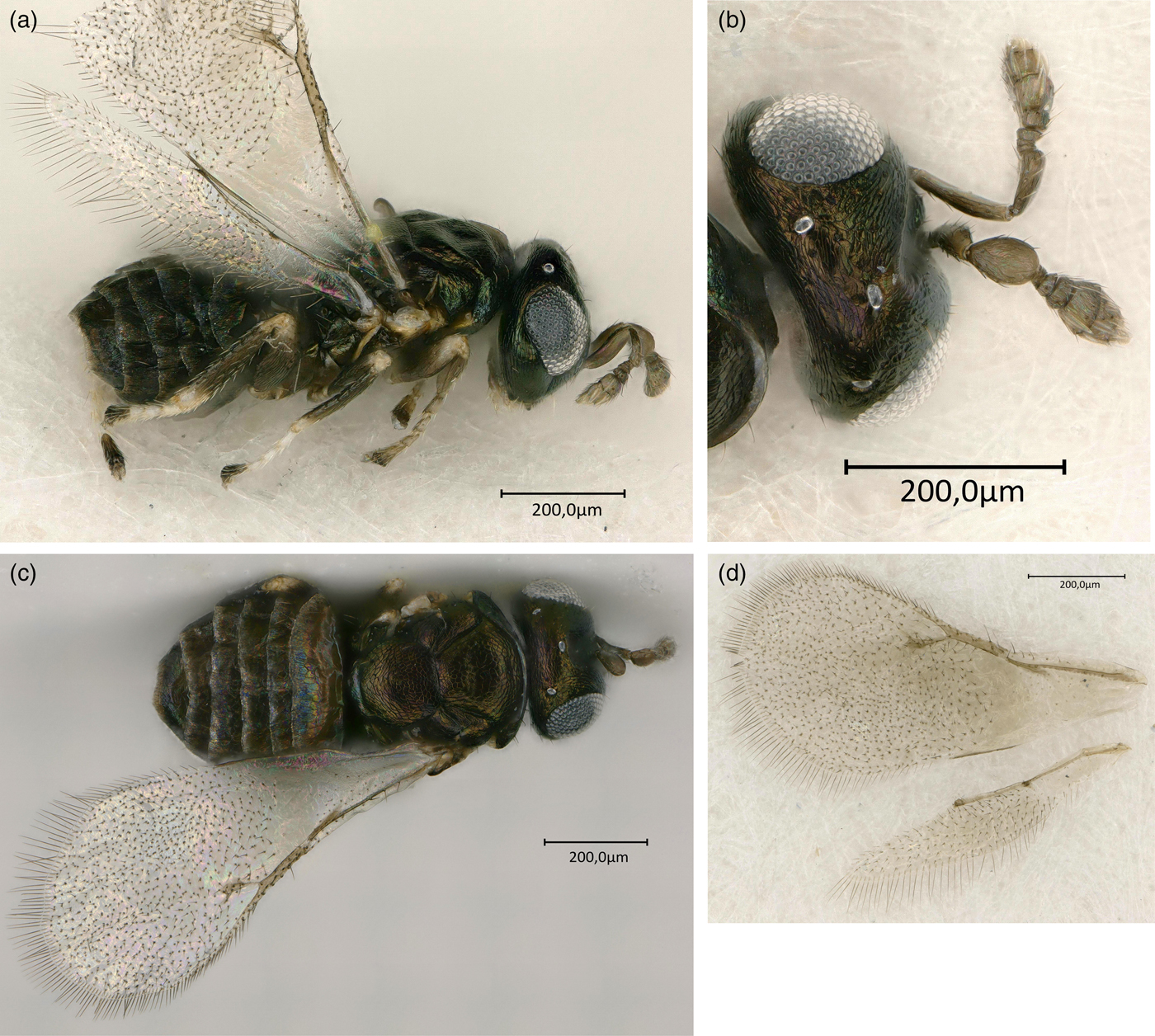
Fig. 9. O. mediterraneus sp. n. female: (a) lateral view; (b) head and antennae in dorso-lateral view; (c) dorsal view and (d) wings.
Description: Female.
Body length: 0.8–1.0 mm.
Colour: Head and body brown with variable metallic luster (fig. 9a, c). Eyes and ocelli grey (fig. 9b). Antenna light brown (fig. 9b). Legs brown, but tips of femora and tibiae and first three tarsomeres pale brown (fig. 9a).
Head (fig. 9b): Dorsally imbricate and ventrally becoming reticulate; interantennal elevation distinctively reticulates, scrobal depressions broad and smooth. With antennal scrobe hardly visible in some specimens, but united immediately below centre of face, reaching oblique grooves at ocellar triangle. Interantennal elevation, parascrobal area, and most of ventral half of face with long setae, but scrobal area bare. Eyes with setae that are visible at high magnifications. Toruli at lower eye margin. Malar sulcus distinct. Clypeal margin transverse and hardly distinguished from adjacent parts of mouth margin.
Antennae (fig. 9b): Scape reaching only slightly above mid eye height. Pedicel longer than funicle. First four flagellomeres anelliform, the last also transverse but larger and bearing multiporous plate sensilla. Clava longer than the other flagellomeres together, with three distinct clavomeres, the apical one bearing a long terminal seta.
Mesosoma (fig. 9c): Pronotum medially vertical, but laterally with collar. Mesoscutum 0.93–1.13× mesoscutellum length along midline. Mesoscutal midlobe anteriorly imbricate, posteriorly becoming reticulate to coriaceous, with two pairs of setae; mesoscutal sidelobe reticulate. Axilla coriaceous, advanced for about half its length. Mesoscutellum coriaceous, 1.07–1.33× as broad as long, with two pairs of setae, the anterior pair in anterior half near scutoscutellar sulcus, the posterior pair near posterior rim of mesoscutellum; mesoscutellar rim not carinate, but overhanging metascutellum slightly; axillular groove present but not visible from dorsal view. Metascutellum very shallowly reticulate, slightly overhanging propodeum. Propodeum medially not longer than metascutellum, posterior margin deeply excavated medially; in some specimens there is a broad and shallow median carina that splits to form a nuchal triangle.
Wings (figs 1a and 9d): Forewing about as long as body, about twice as long as broad. Submarginal vein about 1/3 forewing length, with three dorsal setae (these often broken off, but their sockets are easily seen). Parastigma with slight extension at basal fold, with distinct parastigmal break at base of marginal vein; two parastigmal sensilla present. Costal cell and basal cell with a few extremely short dorsal setae. Speculum present. Marginal vein slightly longer than stigmal vein, but shorter than postmarginal vein; three distinctively long setae present along anterior edge of marginal vein. Stigmal vein with dorsal setae only on expanded stigmal area; uncus present. Apex of postmarginal vein indistinct. Wing disc densely setose; admarginal setae only present near along stigmal vein and apical half of marginal vein. Longest fringe setae as long or slightly longer than marginal vein. Hindwing with dark spot near base of the single parastigmal seta.
Legs (figs 1b and 9a): Coxa shallowly reticulate. Femora and tibiae imbricate. Mesotibial spur about as long as the first two mid tarsomeres together. Hind tibia densely setose, with one apical spur.
Metasoma: About as long as mesosoma. Petiole transverse and not easily visible without removing metasoma. Terga easily collapsing and in some areas with faint reticulation. First gastral tergum the longest. Very small, semi-translucent apical tergum present that may be an epipygium. Ovipositor short, stylets slightly down-turned apically. Hypopygium reaching about 0.8× gaster length.
Male. Unknown
Distribution: France, Italy, Portugal, Australia. Probably present in more countries, in particular in the Mediterranean region, since the presence of O. maskelli may have dissimulated its presence.
Hosts: Exclusively associated with Eucalyptus species and to date reported only on Eucalyptus species from the Maidenaria Section. Mainly found on E. globulus in Italy, France and Portugal. In a context of outbreaks in France, it was also found on E. cinerea, E. gunnii and E. parvula, as well as on E. cypellocarpa in Portugal (Garcia et al., Reference Garcia, Goncalves, Borowiec, Franco and Brancosubmitted).
Comments: Univoltine, the adults’ flying period is around late spring. Fully developed galls are visible from January (see above for details). In native (Australia) or invaded (Italy, France, Portugal) areas, only females of O. mediterraneus were collected, this species being supposed to reproduce by thelytoky.
Discriminating between Ophelimus species using previous work is quite a hard task since Girault's descriptions (42 descriptions among a total of 51 known species of Ophelimus; Noyes, Reference Noyes2018) are always poor and the types are in very bad conditions. The genus Ophelimus would definitively need a taxonomic revision to evaluate the status of all species and provide an identification key.
Identification key for some Ophelimus species of agricultural interest
1. Small species, <1.2 mm long (2)
1′. Larger species, >1.5 mm long (3)
2(1). Only one submarginal seta on forewings; induces large green round-shaped galls, visible on both sides of the leaves, that become reddish with maturation. Mainly found on Eucalyptus species belonging to Transversaria section (e.g. E. camaldulensis and E. rudis). At least two generations per year maskelli
2′. Two—four (mostly three) submarginal setae on forewings. Induces small ellipsoid green galls, only visible on upper side of leaves, that become brownish with maturation. On Eucalyptus species belonging to the Maidenaria section (mainly E. globulus). Univoltine mediterraneus sp. n.
3(1′). Two—four submarginal setae on forewings. Develops on Eucalyptus from section Maidenaria eucalypti ‘Maid.’
3′. More than four submarginal setae on forewings. Develops on Eucalyptus from section Transversaria eucalypti ‘Trans.’
Discussion
Using an integrative taxonomy approach (Al Khatib et al., Reference Al Khatib, Fusu, Cruaud, Gibson, Borowiec, Rasplus, Ris and Delvare2014; Baur et al., Reference Baur, Kranz-Baltensperger, Cruaud, Rasplus, Timokhov and Gokhman2014; Fusu, Reference Fusu2017; Gebiola et al., Reference Gebiola, Monti, Johnson, Wooley, Hunter, Giorgini and Pedata2017), our study unambiguously evidences the presence in the Mediterranean region (France, Italy, Portugal) of a third species of Ophelimus that threatened Eucalyptus plantations. This new species was also found in its supposed native area (Australia) where it was not until now described. A full description of this new species, finally named O. mediterraneus, is provided here.
From a methodological point of view, both molecular markers (COI and 28S) showed unambiguous differentiation between the four species of Ophelimus sampled: O. eucalypti ‘Maid.’, O. eucalypti ‘Trans.’, O. maskelli and O. mediterraneus sp. n. The capacity of discrimination of the morphological characters used depends upon the characters and species considered. We hence confirm here that, to date, O. maskelli is the only species that present only one submarginal seta on its forewings. Even if few individuals were examined, O. eucalypti ‘Trans.’ seems to be the only one with at least five submarginal setae. This character was however not discriminant for the two last species that nevertheless differ in their overall size, O. eucalypti ‘Maid.’ being clearly bigger than O. mediterraneus sp. n. Finally, ecological criteria proved to be also relevant in distinguishing O. maskelli and O. mediterraneus sp. n. Indeed, the galls induced by both species are easily distinguishable, O. maskelli producing round and smooth, green reddish galls visible on both sides of the leaves while O. mediterraneus sp. n. produces ellipsoidal, conical shaped galls, brown coloured with rough and cracked surface on just the upper side of the leaves. Moreover, unlike O. maskelli that have several generations per year, O. mediterraneus sp. n. appears to be univoltine.
As O. maskelli was misidentified as O. eucalypti when it was firstly discovered in Europe (Protasov et al., Reference Protasov, La Salle, Blumberg, Brand, Saphir, Assael, Fisher and Mendel2007b), O. mediterraneus sp. n. may have been confused with O. maskelli in their sympatric introduced areas. Now, the discovery of O. mediterraneus sp. n. rather speaks for an ecological specialization in the different Ophelimus species, at least in Europe. Indeed, O. mediterraneus sp. n. may be strictly associated with Eucalyptus species from the section Maidenaria while O. maskelli, although generalist (Protasov et al., Reference Protasov, La Salle, Blumberg, Brand, Saphir, Assael, Fisher and Mendel2007b; Branco et al., Reference Branco, Dhahri, Santos and Ben Jamaa2014), develops mostly on E. camaldulensis and related species.
By the way, our study also evidences a structuration within O. eucalypti. Based on molecular, morphological (number of submarginal setae) as well as ecological (host range) features, we indeed confirm that the two biotypes, O. eucalypti ‘Trans.’ and O. eucalypti ‘Maid.’, may be two distinct species that are closely related morphologically. Because some Eucalyptus species have a restricted distribution in Australia and more than 50 species of Ophelimus are described, intra- or inter-specific variations in Eucalyptus–Ophelimus interactions are not so surprising (Withers et al., Reference Withers, Raman and Berry2000). However, the consequences of such eco-evolutionary processes (ecological specialization and speciation) on the management of Eucalyptus pests may have been under-estimated.
In the frame of biological control, the accurate identification of the pest species targeted and its biological control agents is the first indispensable pre-requisite for the implementation of a biological control programme (Rosen & DeBach, Reference Rosen and DeBach1973). If this is not the case, this could lead to the failure of the programme and could have consequences such as the non-establishment of the biological control agent released as well as unintentional effects such as non-target impacts on native communities (Kenis et al., Reference Kenis, Auger-Rozenberg, Roques, Timms, Péré, Cock, Settele, Augustin and Lopez-Vaamonde2009; Kenis & Branco, Reference Kenis and Branco2010). About the control of Ophelimus species, C. chamaeleon was proved to be successful against O. maskelli in several countries (this study and Branco et al., Reference Branco, Boavida, Durand, Franco and Mendel2009; Caleca et al., Reference Caleca, Lo Verde, Rizzo and Rizzo2011; Burks et al., Reference Burks, Mottern, Pownall, Waterworth and Paine2015b; Mendel et al., Reference Mendel, Protasov, La Salle, Blumberg, Brand and Branco2017). However, neither C. chamaeleon nor any other species emerged from O. mediterraneus sp. n. even in case of very close proximity between E. camaldulensis infested by O. maskelli and E. globulus infested by O. mediterraneus. This may be the consequence of an asynchronous life cycle between O. mediterraneus sp. n. and C. chamaeleon (Garcia et al., submitted). E. globulus covers about 3 million hectares worldwide with more than 50% of the total cultivated area localized in the Mediterranean region (Potts et al., Reference Potts, Vaillancourt, Jordan, Dutkowski, Da Costa e Silva, McKinnon, Steane, Volker, Lopez, Apiolaza, Li, Marques, Borralho, Borralho, Pereira, Marques, Coutinho, Madeira and Tomé2004). One can fear a rapid expansion and outbreaks of O. mediterraneus sp. n. Specific investigations on parasitoids of E. mediterraneus sp. n. in the native area should thus be welcomed to evaluate the feasibility of a classical biological control.
Supplementary material
The supplementary material for this article can be found at https://doi.org/10.1017/S0007485318001037.
Acknowledgements
The authors acknowledge the support of Hélène Dumas (La Ciotat, France), Rémi Pécout (Chambre d'Agriculture du Var, France) and Aurélie Tourlourat (Chambre d'Agriculture des Alpes-Maritimes, France) for their support with the field work, and to Jean Vescovi (Syndicat des exploitants agricoles du Tanneron, France) for the help in selecting study sites. We are also grateful to Toni Withers (Scion Research Institute, New Zealand) for sending us specimens of O. eucalypti as well as to Simon Lawson (CSIRO, Australia) for the fruitful discussions about Eucalyptus’ pests. Funding for this study was contributed by the ‘Chambre d'Agriculture des Alpes-Maritimes’ and the ‘Syndicat des exploitants agricoles du Tanneron’. Finally, we dedicate this paper to John La Salle, who tragically passed away on 27th May 2018.