Introduction
Organisms that create, modify or maintain habitats (or microhabitats) are known as ecosystem engineers (Jones et al., Reference Jones, Lawton and Shachak1994, Reference Jones, Lawton and Shachak1997). Many organisms are known to modify the physical structure, complexity and heterogeneity of marine environments, and thus influence the characteristics of the associated communities (Jones et al., Reference Jones, Lawton and Shachak1994, Reference Jones, Lawton and Shachak1997; Crooks, Reference Crooks2002) including mussel beds, reef-building organisms and tube-building worms (Khaitov et al., Reference Khaitov, Fokin and Nicolaeva1999; Callaway, Reference Callaway2006; Commito et al., Reference Commito, Como, Grupe and Dowa2008). Tubiculous polychaetes can build their tubes from a number of different materials (e.g. mud, sand, shell, sandstone). These tubes play an especially important ecological role by providing structures that increase the physical complexity and biodiversity of habitats (Dauer et al., Reference Dauer, Tourtelotte and Ewing1982; Bailey-Brock, Reference Bailey-Brock1984; Dubois et al., Reference Dubois, Retière and Olivier2002; Thomsen et al., Reference Thomsen, Muth and McGlathery2011).
Polychaete tubes are known to influence near-bed hydrodynamics (Jumars & Nowell, Reference Jumars and Nowell1984), by either stabilizing (Bolam & Fernandes, Reference Bolam and Fernandes2003) or destabilizing sediments (Eckman et al., Reference Eckman, Nowell and Jumars1981; Carey, Reference Carey1983; Luckenbach, Reference Luckenbach1986). In general, tube structures have three principal effects, influencing the properties of the sediment, the hydrodynamic regime and the availability of surfaces for attachment. The intensity of the effect on hydrodynamics and sediments depends on the density of the tubes (Eckman, Reference Eckman1983).
The genus Diopatra includes ~50 tubiculous species (Budaeva & Fauchald, Reference Budaeva and Fauchald2008), which are common in the intertidal and shallow subtidal waters of all the major oceans, but are more diverse in warmer waters. These worms often reinforce the walls of their simple tubes of mucous-bound particles with fragments of shell, algae and other debris (Hartman, Reference Hartman1969). Diopatra cuprea (Bosc, 1802) occurs in coastal waters between Cape Cod and Brazil (Mangum et al., Reference Mangum, Santos and Rhodes1968). This species builds vertical tubes, which typically penetrate the substratum to a depth of 50–60 cm (Myers, Reference Myers1972), with a hook-shaped ‘tube cap’ emerging 2–5 cm above the sediment surface. Isolated D. cuprea tubes or aggregations of tubes are especially common in intertidal estuarine areas or other sheltered coastal environments.
The construction of Diopatra tubes on unconsolidated bottoms may influence the structure of the meiofauna (Bell & Coen, Reference Bell and Coen1982a, Reference Bell and Coen1982b; Bell & Woodin, Reference Bell and Woodin1984; Bell, Reference Bell1985; Guilherme, Reference Guilherme, Silva, El-Deir and Santos2011) and benthic macrofauna (Woodin, Reference Woodin1978; Bell & Devlin, Reference Bell and Devlin1983; Thomsen et al., Reference Thomsen, McGlathery, Schwarzschild and Silliman2009) communities, as well as the bacteria (Phillips and Lovell, Reference Phillips and Lovell1999; Matsui et al., Reference Matsui, Ringelberg and Lovell2004) and algae (Thomsen, Reference Thomsen2004, Reference Thomsen, McGlathery, Schwarzschild and Silliman2009). In general, there is a positive influence on the species richness and abundance of the fauna associated with these tubes.
While some studies have focused on the fauna associated with worm tubes, there are virtually no data from tropical regions. On the Brazilian Amazon coast, in particular, no information is available on even the occurrence or ecology of D. cuprea, despite the fact that this species is common on mudflats and protected sandy beaches. This coastal region has a number of unique features, including high volumes of river discharge, a macrotidal regime and intense dry and rainy seasons, which contribute to marked fluctuations in the salinity and hydrodynamics of the coastal environments (Dittmar and Lara, Reference Dittmar and Lara2001; Souza-Filho et al., Reference Souza-Filho, Lessa, Cohen, Costa and Lara2009; Pereira et al., Reference Pereira, Sozinho da Silva, da Costa, Asp, da Costa and Vila-Concejo2012). This provokes extreme temporal variations in the characteristics of Amazonian benthic communities (Rosa Filho et al., Reference Rosa Filho, Almeida and Aviz2009, Reference Rosa Filho, Gomes, Almeida and Silva2011; Silva et al., Reference Silva, Pereira, Gorayeb, Vila-Concejo, Sousa, Asp and Costa2011; Braga et al., Reference Braga, Silva, Rosa-Filho and Beasley2013).
This study describes the structure of the macrobenthic fauna associated with D. cuprea tubes on a sandy beach of the Amazon coast. Two hypotheses were tested: (i) the presence of tubes contributes to the establishment of a macrobenthic assemblage distinct from that found on sediments with no tubes, and (ii) the structure of the benthic macrofauna associated with the tubes varies significantly between climatic seasons.
Materials and methods
Study area
The island of Algodoal-Maiandeua is located on the northern coast of Brazil (0°34′45″–0°37′30″S 47°32′05″–47°34′12″W), and is surrounded on three sides by rivers and estuarine channels (Figure 1A). The region is dominated by semidiurnal macrotides, with amplitude of between 4 and 7 m (Silva et al., Reference Silva, Pereira, Gorayeb, Vila-Concejo, Sousa, Asp and Costa2011). The local climate is humid tropical with a mean annual temperature of 27.7 ± 1.1°C (Martorano et al., Reference Martorano, Pereira, César and Pereira1993) and mean annual precipitation (30-year records) of 2300–2800 mm (Moraes et al., Reference Moraes, Costa, Costa and Costa2005). Precipitation varies considerably over the course of the year, however, with a well-defined rainy season from January to July (total rainfall ~1657 mm), and a dry season from August to December, with total rainfall of 490 mm (Moraes et al., Reference Moraes, Costa, Costa and Costa2005).

Fig. 1. Map (UTM: 23S zone) of Algodoal-Maiandeua Island (A); Farol Beach (B); the exposed portion of a Diopatra cuprea tube (C).
Algodoal-Maiandeua Island has 35 km of sandy beaches, which vary considerably in their slope, extension and width, and their exposure to wave action, and are covered in substrates ranging from mud to sand (Mendes, Reference Mendes and Fernandes2005). The study beach, Farol, is located on the western margin of the island, which is bathed by the Marapanim River and is a low-tide terrace beach with a wide intertidal zone (200–400 m) composed mainly of fine sediments (Rosa Filho et al., Reference Rosa Filho, Gomes, Almeida and Silva2011). Diopatra cuprea tubes are common in the intertidal zone of this beach (Figure 1B).
Sampling and sample processing
Samples were collected in June (rainy season) and September (dry season) 2012 from two different areas of the intertidal zone, each with an area of ~250 m2, at the same distance from the tideline. One of these areas was populated with D. cuprea tubes (area 1), the other had no tube worms (area 2). During each survey, 20 replicate samples were collected haphazardly in each area using cylindrical cores (0.0079 m2, 20 cm deep). In area 1, a D. cuprea tube (occupied by a single worm) was positioned at the centre of each cylindrical core (Figure 1C). The samples were filtered through a 0.3 mm mesh screen, and all the macrofauna was retrieved and fixed in 4% formalin saline. To estimate the density of the D. cuprea within area 1, the number of tubes were counted in eight randomly distributed square subplots (25 m2), in both study months.
Simultaneously to the biological sampling, a sediment sample was collected from each area for granulometric analyses using the same core sampler. The temperature of the sediment was determined from three random replicates taken with a soil thermometer in each sampling area. The salinity of the seawater was also determined from a sample of the surface water of the infralittoral, taken with a manual refractometer. Data on air temperatures and precipitation levels were obtained from the meteorological station in Salinópolis (~30 km from the study site), and were provided by the Brazilian National Meteorological Institute (INMET).
In the laboratory, the biological samples were examined under a stereoscopic microscope, and the organisms observed were counted and identified to the lowest possible taxonomic level. The granulometric analysis was conducted by sieving out the coarse sediments and pipetting the fine sediments, as proposed by Suguio (Reference Suguio1973). The textural parameters (mean grain size, sorting, sand and percentage gravel) were calculated using the equations of Folk & Ward (Reference Folk and Ward1957). Grain sizes were determined by sieving the sediment in an automatic shaker and classifying the grains according to the Wentworth scale (Buchanan, Reference Buchanan, Holme and McIntyre1984).
Statistical analysis
We calculated the total taxon richness and density (ind. m−2) for each biological sample, and the variation in these parameters was analysed using a two-way (area and season) analysis of variance (ANOVA) after verifying the normality and homogeneity of variances assumptions, using the Kolmogorov–Smirnov and Levene tests, respectively. When necessary, the data were transformed (fourth root or Log(x + 1)). When the ANOVA detected a significant difference, Tukey's a posteriori test was applied to identify significant pairwise differences. The data on the abundance of D. cuprea were analysed separately from those of the associated fauna, and were tested between seasons using a one-way ANOVA.
To assess the effects of the D. cuprea tubes on the macrofauna and validate our a priori grouping (area 1 and 2; dry and rainy seasons), a Principal Coordinates Analysis (PCO) was run on a Bray–Curtis similarity matrix of the fourth root-transformed species abundance data. To identify the species that characterized each area and season, species that correlated (Spearman's coefficient) more than 60% with one of the first two axes were plotted in each PCO. Simultaneously, the same density matrices used for the PCO were analysed using a two-way permutational ANOVA (PERMANOVA) designed using the same layout as the ANOVA. The contribution of each taxon to the similarity and dissimilarity found among the groups was assessed using the SIMPER (similarity percentage) routine. A 5% significance level was considered in all analyses.
Results
Environmental parameters
Precipitation was much higher during the rainy season month (June), whereas the air and sediment temperatures and the salinity were higher during the dry season (Table 1). Overall, temperatures were higher in area 2 (control) in comparison with area 1 (with D. cuprea tubes). The sediment in area 1 was classified as fine sand, with some finer sediments (silt and clay) in both months, whereas in area 2, the sediment was classified as fine to medium sand in June (rainy season) and medium sand in the dry season (Table 1).
Table 1. Environmental characteristics of the study area

VFS, very fine sand; FS, fine sand; MS, Medium sand.
Macrofauna
The mean density of D. cuprea was 69.6 ± 14.4 ind. m−2 in the rainy season and 38.0 ± 13.3 ind. m−2 in the dry season, although there was no significant difference between seasons (ANOVA, F (1.38) = 2.59, P = 0.11). Fifty-three macrobenthic taxa (excluding D. cuprea) were recorded during the present study, of which 26 were found exclusively in area 1 (with D. cuprea tubes), and 12 exclusively in area 2 (control) (Supplementary Material 1). The Annelida was the phylum represented by the largest number of taxa (23), and was the most abundant group in both areas in both seasons (Figure 2), with Orbinia sp. (20% of total abundance) being most abundant.
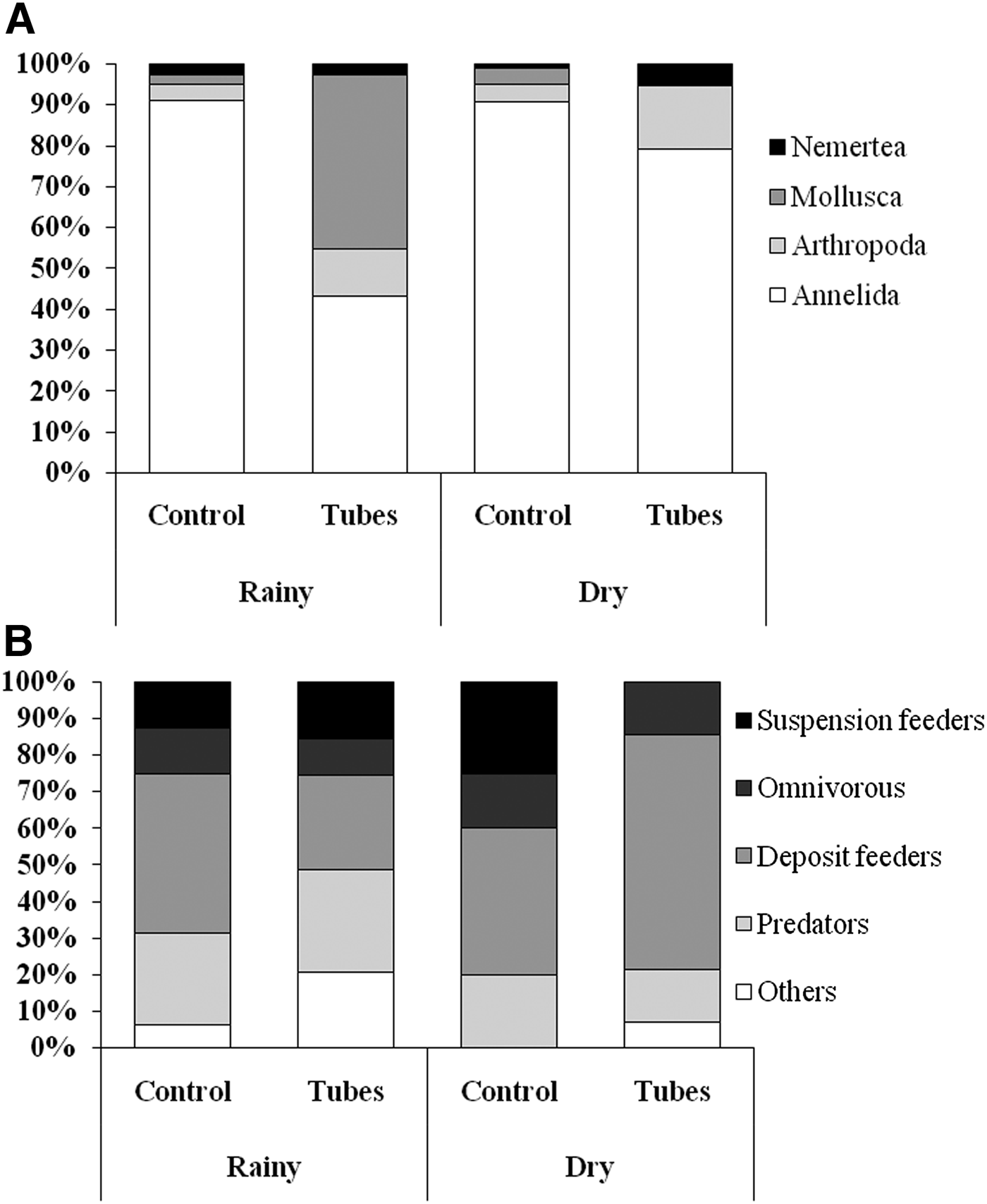
Fig. 2. Relative abundance (%) of taxonomic (A) and feeding groups (B) of the macrobenthic fauna of the two sampling plots in the two study months. (N = 20 samples by area/season).
Molluscs and arthropods were recorded at higher densities in area 1, in particular during the rainy season. In the dry season, molluscs were less abundant in area 1 and more abundant in area 2 (Figure 2A). With regard to the contribution of the feeding guilds to total abundance, deposit-feeders dominated the trophic web in both areas, in particular during the dry season (Figure 2B). A greater diversity of functional groups was recorded in area 1 during the rainy season, in comparison with both the dry season in area 1, and both months in area 2. An increase in deposit-feeders and a marked decrease in suspension feeders were observed in area 1 in the dry season (Figure 2B).
The mean macroinvertebrate densities and total number of taxa varied significantly among areas and seasons. The ANOVA indicated a significant interaction between site and month for both taxon richness (F (1.76) = 46.23; P = 0.00) and density (F (1.76) = 18.72; P = 0.00). In the rainy season (Figure 3), the taxon richness and density were higher in area 1 (1525.32 ± 343.29 ind. m−2; 38 taxa) than in area 2 (506.33 ± 126.58 ind. m−2; 17 taxa). The opposite pattern was observed during the dry season, however, when diversity and density were both higher in area 2 (1436.71 ± 170.88 ind. m−2; 22 taxa), in comparison with area 1 (607.59 ± 122.03 ind. m−2; 16 taxa). The results of Tukey's test indicated that significant differences between areas in these parameters occurred only during the rainy season, and that significant differences between seasons were recorded in both areas (Figure 3).

Fig. 3. Mean density (ind. m−2 ± standard error) and taxon richness (B) of the macrobenthic fauna of the two sampling plots in the two study months. (N = 20 samples by area/season).
The PCO plots distinguished the macrofauna samples between the two study areas and seasons (Figure 4A). In the rainy season (Figure 4B), axis 1 explained 24.3% of the variation in the data and was responsible for separating the two areas. The species most correlated with this axis were more abundant in area 1, including the hermit crab, Clibanarius symmetricus (Randall, 1840), the molluscs Leukoma pectorina (Lamarck, 1818), Mytella guyanensis (Lamarck, 1819), Phrontis vibex (Say, 1822) and Littoraria angulifera (Lamarck, 1822), as well as the polychaetes Nephtys simoni (Perkins, 1980) and Laeonereis culveri (Webster, 1879). In the dry season (Figure 4C), axes 1 and 2 explained 39.2% and 14.6% of the variation in the data, respectively, with the samples of the two areas grouping in opposite directions along each axis. The species most associated with area 1 were the isopod Excirolana armata (Dana, 1853), the worms Sigambra sp. and Mediomastus sp. and the Nemertea. By contrast, the polychaetes Armandia sp., Nephtys simoni and Orbinia sp. were most closely associated with area 2. The PERMANOVA confirmed the spatial configuration of the samples, showing significant differences between seasons and areas (Table 2).
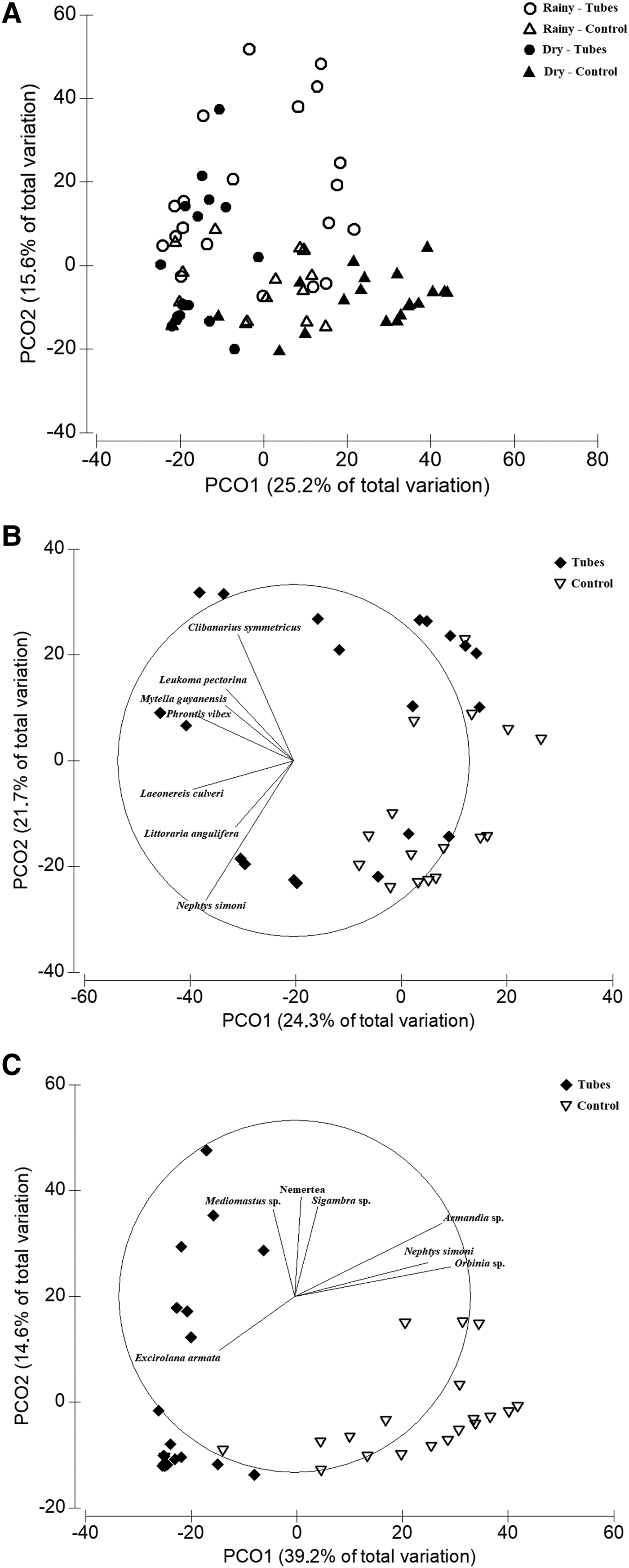
Fig. 4. Plots of the Principal Coordinates Analysis (PCO) of the samples of the macrobenthic fauna collected from the two plots in the different study months. The vectors represent species correlating more than 50% (based on Spearman correlation coefficients) with one of the first two PCO axes. (A) All samples (N = 80); (B) Dry season samples (N = 20 samples per area); (C) Rainy season samples (N = 20 samples per area).
Table 2. Results of the PERMANOVA and pairwise tests for the structure of the benthic macrofauna between plots (with and without tubes) and months (dry and rainy season)

*Significant differences (P < 0.05).
df, degrees of freedom; MS, mean squares.
The SIMPER analysis indicated a mean dissimilarity of more than 90% between areas in both months. The dissimilarity between seasons was also high (>80%) in both areas (Table 3). Most of the species highlighted by the SIMPER were those most closely correlated with the PCO axes. Comparing sites, most of the species indicated by SIMPER were more abundant in area 1 during the rainy season, in particular polychaetes (N. simoni and Nereis sp.), molluscs – Olivella minuta (Link, 1807), P. vibex and M. guyanensis – and C. symmetricus. In the dry season, polychaetes (Orbinia sp., Armandia sp. and N. simoni) contributed more than 40% of the dissimilarity, and were more abundant in area 2 (Table 3). Most of the predominant species found in area 1 (e.g. Nereis sp., C. symmetricus, N. simoni, Anachis obese (Adams, 1845) and P. vibex) were more frequent and abundant in this area during the rainy season. In the control area, by contrast, four species (Orbinia sp., Armandia sp., N. simoni and Macoma sp.) were responsible for more than 55% of the dissimilarity between months, due to their increased frequency and density in the dry season (Table 3).
Table 3. The results of the SIMPER analysis, showing the means abundance (ind. m−2 ± standard error) and similarity of the species that most contributed the samples from the two plots (in the different study months)

Discussion
The dynamics of coastal benthic communities are mediated by a combination of physical, chemical and biological processes. Benthic species are known to be active agents in the interaction between these different components (Berke et al., Reference Berke, Mahon, Lima, Halanych, Wethey and Woodin2010; Breitburg et al., Reference Breitburg, Crump, Dabiri and Gallegos2010; Callaway et al., Reference Callaway, Desroy, Dubois, Fournier, Frost, Godet, Hendrick and Rabaut2010; Woodin et al., Reference Woodin, Wethey and Volkenborn2010). On Algodoal-Maiandeua Island, the presence of D. cuprea tubes is associated with fine sediments. The presence of polychaete tubes is known to influence hydrodynamics (Eckman et al., Reference Eckman, Nowell and Jumars1981; Callaway, Reference Callaway2006) by reducing the velocity of the near-bottom flow (Friederichs et al., Reference Friederichs, Graf and Springer2000). This may result in an increase in the deposition of fine sediments and the availability of organic matter (Bolan & Fernandes, Reference Bolam and Fernandes2003).
In Amazonian coastal environments, the major seasonal fluctuations in hydrodynamic conditions and fluvial discharge determine shifts in the sediment load and the dispositional patterns of nearshore environments (Jaeger & Nittrouer, Reference Jaeger and Nittrouer1995; Souza-Filho et al., Reference Souza-Filho, Lessa, Cohen, Costa and Lara2009). While the sampling of sediment in the present study was limited in scope, some variation was observed between months on Farol beach, in particular in area 2, where particles of fine sand were recorded only during the dry season. The granulometry varied much less in area 1, however, which was dominated by fine sediments (silt and clay). Tube-building polychaetes are known to promote greater sediment stability due to near-bed hydrodynamic effects (Luckenbach, Reference Luckenbach1986; Bolam & Fernandes, Reference Bolam and Fernandes2003).
Dense aggregations of Diopatra tubes are commonly found in protected intertidal areas, where organic debris is deposited (Bailey-Brock, Reference Bailey-Brock1984; Dagli et al., Reference Dagli, Ergen and Çinar2005; Thomsen & McGlathery, Reference Thomsen and McGlathery2005). In general, the density of D. cuprea recorded in the present study (means of 69.6 ± 14.4 and 38.0 ± 13.3 ind. m−2 in the rainy and dry seasons, respectively) was relatively low in comparison with the values recorded at many other sandy, intertidal flats. In North Carolina, in the USA, for example, Peckol & Baxter (Reference Peckol and Baxter1986) recorded mean densities of D. cuprea of between 76.7 ± 7.3 and 178.3 ± 6.0 ind. m−2, while Mangum et al. (Reference Mangum, Santos and Rhodes1968) recorded a density of 101.3 ± 19.1 ind. m−2. The density of D. cuprea recorded in the present study is also lower than the densities recorded for other species of the genus, such as Diopatra leuckarti Kinberg, 1865, which reached 21,800 ind.m−2 in a narrow sediment band adjacent to a sandy beach in the Niu Valley in Hawaii (Bailey-Brock, Reference Bailey-Brock1984), and Diopatra marocensis Paxton, Fadlaoui & Lechapt, 1995, with a density of 90 ind. m–2 being recorded by Çinar et al. (Reference Çinar, Fauchald and Dagli2014) in Mersin Bay in Turkey, at depths of 4 m (river mouth) and 25 m, in a lagoon. However, Thomsen et al. (Reference Thomsen, Muth and McGlathery2011) recorded a density of only 2.7 ind. m−2 for Diopatra spp. at two sandy beaches in Mozambique.
On Algodoal-Maiandeua Island, the tubes were typically scattered widely in the intertidal zone, which is probably due to the intense hydrodynamics of the local macrotidal beaches, which may prevent the development of denser aggregations. This is supported by the fact that a lower density of D. cuprea was recorded during the dry season (September), when hydrodynamics are more intense, due to the stronger easterly trade winds (Pereira et al., Reference Pereira, Mendes, Monteiro and Asp2009) and the strong tidal currents (Pereira et al., Reference Pereira, Sozinho da Silva, da Costa, Asp, da Costa and Vila-Concejo2012) typically found on Amazonian beaches during this season. Mangum et al. (Reference Mangum, Santos and Rhodes1968) found that the population density of D. cuprea is related only weakly to the particle size of the substrate, but is correlated strongly with current velocity. While higher current speeds may benefit the feeding mode of Diopatra (Mangum et al., Reference Mangum, Santos and Rhodes1968), it is possible that very fast currents will have a negative impact on the physical structure of the tubes, as discussed below.
While many studies have compared bare sediments with high-density tube aggregations, the results of the present study indicated that more scattered Diopatra tubes may also influence invertebrate communities. In this study on Algodoal-Maiandeua Island, the area with the D. cuprea tubes generally had a more diverse and abundant fauna. Similar findings have been obtained for the fauna associated with dense aggregations of D. cuprea and Lanice conchilega (Pallas, 1766) in the North Atlantic (e.g. Woodin, Reference Woodin1978; Bell & Coen, Reference Bell and Coen1982a, Reference Bell and Coen1982b; Callaway, Reference Callaway2003; Van Hoey et al., Reference Van Hoey, Guilini, Rabaut, Vincx and Degraer2008; Thomsen et al., Reference Thomsen, Wernberg, Altieri, Tuya, Gulbransen, McGlathery, Holmer and Silliman2010), as well as for D. cuprea in Brazil (Guilherme et al., Reference Guilherme, Silva, El-Deir and Santos2011). Previous studies have shown that even solitary tubes in low-density landscapes may have strong effects on the invertebrate community (Callaway, Reference Callaway2006; Thomsen et al., Reference Thomsen, Muth and McGlathery2011), although on a much smaller spatial scale. In addition to their influence on hydrodynamics and the texture of the substrate, solitary tubes may increase the complexity and heterogeneity of habitats, facilitating the establishment of a more diverse and abundant macrobenthic community (Rabaut et al., Reference Rabaut, Guilini, Van Hoey, Magda and Degraer2007; Toupoint et al., Reference Toupoint, Godet, Fournier, Retiere and Olivier2008).
While there are obvious limitations to the comparison of regions and/or habitats, the composition of the macrofauna associated with D. cuprea on Algodoal-Maiandeua Island was similar to that found in association with D. cuprea and L. conchilega in temperate habitats (e.g. Callaway, Reference Callaway2006; Van Hoey et al., Reference Van Hoey, Guilini, Rabaut, Vincx and Degraer2008; Callaway et al., Reference Callaway, Desroy, Dubois, Fournier, Frost, Godet, Hendrick and Rabaut2010; Thomsen et al., Reference Thomsen, Wernberg, Altieri, Tuya, Gulbransen, McGlathery, Holmer and Silliman2010). The fauna was composed primarily of estuarine and marine taxa, such as crustaceans, polychaetes and molluscs, in addition to taxa typically found on sandy beaches (Rosa Filho et al., Reference Rosa Filho, Almeida and Aviz2009, Reference Rosa Filho, Gomes, Almeida and Silva2011), in muddy environments (Rosa Filho et al., Reference Rosa Filho, Busman, Viana, Gregório and Oliveira2006; Beasley et al., Reference Beasley, Fernandes, Figueira, Sampaio, Melo, Barros, Saint-Paul and Schineider2010; Braga et al., Reference Braga, Monteiro, Rosa-Filho and Beasley2011, Reference Braga, Silva, Rosa-Filho and Beasley2013), and on consolidated substrate (Morais & Lee, Reference Morais and Lee2013) of the Amazon coast. For example, the molluscs, which were more diverse in the area with D. cuprea tubes, were represented primarily by species not normally found on sandy beaches, but in usually on hard substrates, such as rocks, mangrove roots and other biogenic materials. These taxa include Mytella spp., Littoraria angulifera (Lamarck, 1822), Littoraria flava (King, 1832), Hiatella sp., Sphenia sp. and Thaisella coronata (Lamarck, 1816) (Beasley et al., Reference Beasley, Fernandes, Figueira, Sampaio, Melo, Barros, Saint-Paul and Schineider2010).
The Diopatra tubes provide a wider range of infaunal niches, including mud, detritus, algae, and various types of infaunal prey (Lana et al., Reference Lana, Guiss and Disaró1991; Attolini et al., Reference Attolini, Flynn and Tararam1997; Flynn et al., Reference Flynn, Wakabara and Tararam1998). The aggregation of organisms with different life strategies results in an increase in both the taxonomic and functional richness of the benthic assembly. However, while other studies typically have a broader spatial comparative perspective – i.e. multiple environments with colonies of Diopatra vs mud flats with no tubes – the design of the present study, which only compared a single area with and without tubes on the same beach, is limited to a more local scale.
A significant increase in abundance and taxon richness of the samples associated with D. cuprea was recorded only in the rainy season, when tube density was higher. The temporal variation of the macrofauna in the two areas also followed a different pattern. Whereas the density and diversity of the macrofauna were higher in the rainy season in the area colonized by D. cuprea, the values in the control area were higher in the dry season. As in other Amazonian coastal environments, this may be related to an increase in salinity, since the significant decrease in salinity in the rainy season causes physiological stress to marine organisms (Beasley et al., Reference Beasley, Fernandes, Gomes, Brito, Santos and Tagliaro2005; Rosa Filho et al., Reference Rosa Filho, Gomes, Almeida and Silva2011; Silva et al., Reference Silva, Pereira, Gorayeb, Vila-Concejo, Sousa, Asp and Costa2011; Braga et al., Reference Braga, Silva, Rosa-Filho and Beasley2013). In addition, the more intense hydrodynamic conditions and the resuspension of sediments during the dry season may have a negative effect on the D. cuprea population and its associated fauna.
The D. cuprea tubes display two types of construction, those constructed only of mucous and sand particles, and those reinforced with debris, primarily in the external section, that rises above the sediment surface (Myers, Reference Myers1972; Berke & Woodin, Reference Berke and Woodin2008). The incorporation of items reflects the diversity of the available substrates and surface debris (Bell & Coen, Reference Bell and Coen1982a), and the tubes of this species may be ornamented with shells, algae or assorted debris (Mangum et al., Reference Mangum, Santos and Rhodes1968). On Algodoal-Maiandeua Island, however, the tubes are relatively simple, being decorated primarily with small fragments of plants. The complexity of the tube is further reduced during the dry season, as a result of the increased physical disruption, and perhaps also the greater difficulty of obtaining and incorporating materials under the stronger hydrodynamic conditions typical of this period. In the dry season, in fact, the tubes may often be buried (personal observation), and the restructuring process may have a negative effect on the presence of other organisms, in particular those that inhabit the reinforced portion of the tube, such as epifaunal molluscs and polychaetes, which are practically absent during this season.
As the samples were taken from different areas of the same beach, certain components of the natural variation in the characteristics of the community may not have been evaluated comprehensively, and this should be taken into account in future studies of the role of D. cuprea tubes in the ecology of the region. The exact influence of environmental variables on the populations of this bioconstructor and the characteristics of its tubes, and its effects on the associated fauna, require further investigation. In general, however, the results of the present study are consistent with those of previous research, which found changes in the composition of the benthic macrofauna, that is, higher species richness and densities of fauna in areas inhabited by these tube-building polychaetes in comparison with areas of bare sand, even though D. cuprea was sparsely distributed on the study beach.
Supplementary material
The supplementary material for this article can be found at https://doi.org/10.1017/S0025315418000711
Acknowledgements
We are grateful to Thomas Banha for his assistance in the field, and to Micaela Valentin for her help processing the samples. We thank Dr Stephen Ferrari for his valuable comments and revision of the English text of the manuscript.