1. Introduction
Skarn orebodies are usually associated with and unevenly developed around magmatic bodies (e.g. Einaudi et al. Reference Einaudi, Meinert, Newberry and Skinner1981; Baker et al. Reference Baker, Van Achterberg, Ryan and Lang2004; Meinert, Reference Meinert, Hedenquist, Thompson, Goldfarb and Richards2005; Mao et al. Reference Mao, Xie, Duan, Pirajno, Ishiyama and Chen2011; Sial et al. Reference Sial, Bettencourt, De Campos and Ferreira2011; Xiong et al. Reference Xiong, Zhu, Zhang, Guo, Zheng and Jiang2019). It is broadly recognized that the magma composition, oxidation state and degree of fractionation, lithology and structure of country rock will affect the ore genesis process and type of ore deposit (e.g. Einaudi et al. Reference Einaudi, Meinert, Newberry and Skinner1981; Thompson et al. Reference Thompson, Sillitoe, Baker, Lang and Mortensen1999; Audétat et al. Reference Audétat, Günther and Heinrich2000; Richards, Reference Richards2003; Baker et al. Reference Baker, Van Achterberg, Ryan and Lang2004; Meinert, Reference Meinert, Hedenquist, Thompson, Goldfarb and Richards2005; Seedorf et al. Reference Seedorf, Dilles, Proffett, Einaudi, Zurcher, Stavast, Johnson, Barton, Hedenquist, Thompson, Goldfarb and Richards2005). However, the mechanism of localization of intrusion-related orebody is less investigated, which is important to improve understanding of the intrusion-related ore-forming system and further exploitation in the depth (e.g. Ferry & Dipple, Reference Ferry and Dipple1992; Gerdes et al. Reference Gerdes, Baumgartner and Person1998; Heinrich, Reference Heinrich2005; Eldursi et al. Reference Eldursi, Branquet, Guillou-Frottier and Marcoux2009; Liu et al. Reference Liu, Zhao and Sun2012, Reference Liu, Sun and Zhou2014).
Previous studies show that the variation of magma composition, temperature and pressure gradient around the magma chamber, permeability and fluid circulation in the contact zone may cause the ore deposition (Ray et al. Reference Ray, Webster and Ettlinger1995; Audétat et al. Reference Audétat, Günther and Heinrich2000; Boyce et al. Reference Boyce, Fulignati and Sbrana2003; Lu et al. Reference Lu, Liu, Wang, Xu and Li2003; Vallance et al. Reference Vallance, Cathelineau, Boiron, Fourcade, Shepherd and Naden2003; Matter et al. Reference Matter, Goldberg, Morin and Stute2005; Eldursi et al. Reference Eldursi, Branquet, Guillou-Frottier and Marcoux2009; Gerbault, Reference Gerbault, Healy, Butler, Shipton and Sibson2012; Luan et al. Reference Luan, Song, Chen, Zheng, Zhang, Yu, She, Tian and Ran2014; Gonnermann et al. Reference Gonnermann, Giachetti, Fliedner, Nguyen, Houghton, Crozier and Carey2017). Nevertheless, this variation is dominantly controlled by the magma emplacement mechanism (e.g. Eldursi et al. Reference Eldursi, Branquet, Guillou-Frottier and Marcoux2009; Chen & Nabelek, Reference Chen and Nabelek2017). For example, the gradually emplaced magma injections may accrete in vertical or lateral directions, bilateral or unilateral accretion, and internal filling, which produce different degrees of metamorphism, scale of deformation and fluid circulation patterns in the pluton and contact zone (Scaillet et al. Reference Scaillet, Holtz, Pichavant and Schmidt1996; Kratinová et al. Reference Kratinová, Závada, Hrouda and Schulmann2006; Menand, Reference Menand2008; Tibaldi et al. Reference Tibaldi, Rovida and Corazzato2007; Paterson et al. Reference Paterson, Okaya, Memeti, Economos and Miller2011; Liu et al. Reference Liu, Martelet, Wang, Erdmann, Chen, Faure, Huang, Scaillet, Le Breton, Shu and Wang2018 a, Reference Liu, Chen, Wang, Faure, Erdmann, Martelet, Scaillet and Huang2020). Furthermore, the intrusion-related skarn ore deposit is usually associated with small magmatic bodies (Dines, Reference Dines1956; Dilles & Proffett, Reference Dilles, Proffett, Pierce and Bolm1995; Tang, Reference Tang2002). This opinion is well supported by the magmatism–mineralization system in the Tongling district, eastern China (insert in Fig. 1a, b). The Tongling district is characterized by widely developed Lower Cretaceous dioritic stocks (145–135 Ma; Liu et al. Reference Liu, Shao, Wang and Liu2018 b) that intruded in a NE–SW-striking fold-fault belt in which several world-class skarn-type Cu–Au–Pb–Zn ore deposits have been discovered and mined (Fig. 1b; Chang et al. Reference Chang, Liu and Wu1991; Pan & Dong, Reference Pan and Dong1999; Wu et al. Reference Wu, Dong, Robinson, Frost, Gao, Lei, Chen and Qin2013). In contrast, the emplacement of small magmatic bodies, such as stocks, are usually considered as a diapirically emplaced body with instantaneously emplaced magma injection, for example, the La Bazana stock in Iberian Massif (Galadí-Enríquez et al. Reference Galadí-Enríquez, Galindo-Zaldívar, Simancas and Expósito2003) or the Oulmès stock in Morocco (Tahiri et al. Reference Tahiri, Simancas, Azor, Galindo-Zaldívar, González Lodeiro, El Hadi, Poyatos and Ruiz-Constán2007). The detailed study of emplacement process of magmatic stock, currently limited, appears to be of major significance in understanding the mechanism of orebody localization in skarn deposits.

Fig. 1. Tectonic framework of the Tongling district, modified from the 1:50 000 geological map (Geological Team of Anhui Bureau of Geology and Mineral Resources, 1989). K – Cretaceous strata; NCC – North China Craton; SCB – South China Block; S – Silurian strata, T1+2 – lower and middle Triassic strata; TC – Tarim Craton.
The Tongguanshan stock (TGS in Fig. 2; surface area c. 1 km2) is a representative Lower Cretaceous dioritic stock associated with typical skarn and stratabound skarn Cu–Fe–Au deposits developed in the intensively deformed western margin of the Tongling district (Fig. 1b; Pan & Dong, Reference Pan and Dong1999; Mao et al. Reference Mao, Xie, Duan, Pirajno, Ishiyama and Chen2011; Li et al. Reference Li, Li and Yang2019). Due to the intensive ore exploration in the Tongguanshan area, detailed geometry of the geological bodies in the depth is well established and therefore provides an excellent base from which to understand the stock emplacement processes and evaluation of the relationship between the stock emplacement and skarn mineralization. The Tongguanshan stock was therefore chosen as our target to reveal how the stock emplacement controls the localization of associated skarn-type ore deposits. We conducted detailed macro- and microscopic structure and fabric investigations, anisotropy of magnetic susceptibility (AMS) and three-dimensional (3D) geometric modelling on the Tongguanshan stock and its related orebodies, acquiring new constraints on the stock emplacement process and the associated orebody.

Fig. 2. Geological map of the Tongguanshan ore field (according to the 1:50 000 geological map (Geological Team of Anhui Bureau of Geology and Mineral Resources, 1989).
2. Geological background
The Tongling district, located at the eastern margin of China mainland, is characterized by widely developed Lower Cretaceous dioritic stocks with surface area ranging over c. 0.5–20 km2 (Fig. 1). The Early Cretaceous magmatism in the Tongling district can be classified into two main series, namely the high-K, calc-alkaline series and the shoshonitic series, related to the typical skarn-type Cu-Fe and Au deposits, respectively (Fig. 1; Chang et al. Reference Chang, Liu and Wu1991; Li et al. Reference Li, Pei, Zhang, Mei, Zang, Meng, Zeng, Li and Di2007; Xie et al. Reference Xie, Yang, Du and Sun2008; Liu et al. Reference Liu, Zhao and Zhao2010, Reference Liu, Sun and Zhou2014; Deng et al. Reference Deng, Wang, Xiao, Yang, Liu, Gong and Zhang2011; Wu et al. Reference Wu, Dong, Robinson, Frost, Gao, Lei, Chen and Qin2013). The Tongling district was highly deformed by pre-Cretaceous tectonic events since Neoproterozoic time, including the Neoproterozoic collision between the Yangtze and Cathaysia blocks, the late Neoproterozoic South China block rifting, the Palaeozoic and early Mesozoic intracontinental events, and the Early Cretaceous compression and extension events, producing the current major series of NE–SW-striking S-type fold system (Fig. 1; e.g. Wang & Li, Reference Wang and Li2003; Hacker et al. Reference Hacker, Ratschbacher and Liou2004; Xu et al. Reference Xu, Zeng and Liu2006; Faure et al. Reference Faure, Shu, Wang, Charvet, Choulet and Monie2009; Deng et al. Reference Deng, Wang, Xiao, Yang, Liu, Gong and Zhang2011; Shu et al. Reference Shu, Faure, Yu and Jahn2011). Due to the rigidity difference and differential deformation developed at the brittle crust level, there are some saddle-like weak zones existing in the core of the anticline. The E–W-trending basement faults were also revealed by the geophysical studies (Lü et al. Reference Lü, Hou and Zhao2003), the activation of which during the early Mesozoic epoch produced the NW-trending faults (Fig. 1; Deng et al. Reference Deng, Wang, Huang, Wan, Yang and Gao2006). The intersection of these NE-, NW- and EW-trending faults facilitate the magma ascent and emplacement.
The Tongguanshan stock is an Early Cretaceous quartz–monzodioritic stock (141.8 ± 1.0 Ma; Wu et al. Reference Wu, Gao, Guo, Guo, Liu, Gao, Lei, Qin and Chen2010), intruded in the western limb and hinge of the NE–SW-striking Tongguanshan anticline (Figs 1, 2), and displays a roughly oval-shaped exposure of c. 1 km2 with its long axis parallel to the hinge of the Tongguanshan anticline (Fig. 2; Wang et al. Reference Wang, Zeng, Yang, Meng and Tian2004; Xu et al. Reference Xu, Fun, O’Reilly, Jiang, Griffin, Wang and Qiu2004; Du et al. Reference Du, Li, Cao, Qin and Lou2007; Xie et al. Reference Xie, Yang, Du and Sun2008; Wu et al. Reference Wu, Gao, Guo, Guo, Liu, Gao, Lei, Qin and Chen2010). Macroscopically, the Tongguanshan stock is an isotropic monzodioritic body mainly composed of medium- to fine-grained quartz–monzodiorite without any obvious lithological or textural zonation (Fig. 3a). Dioritic enclaves are irregularly shaped and weakly oriented, with long axes parallel to the regional structures or the stock–country-rock boundary. Previous geobarometric studies on the Tongguanshan stock and the surrounding contemporaneous Tiane’baodan stock (TEBD in Fig. 2) have similar emplacement depths at c. 3–5 km (Du et al. Reference Du, Qin and Tian2004; Cao et al. Reference Cao, Du, Pang, Li, Zhang and Zhang2009; Lei et al. Reference Lei, Wu, Gao, Guo, Liu, Guo, Gao, Chen and Qin2010). Numerous NE–SW-striking garnet-bearing veins, dipping to the SE and parallel to the regional structures, are developed in the eastern margin of the Tongguanshan stock (Fig. 3b, c).

Fig. 3. (a) Field observations of the Tongguanshan stock, (b) quartz–monzodiorite of the stock and (c) garnet-bearing veins developed in the NE margin of the stock.
The country rocks of the Tongguanshan stock mainly consist of Permian limestone and siltstone in its western side, and Carboniferous limestone–dolomite strata and Devonian sandstone in its eastern side (Fig. 2). The Devonian strata are dominated by the Wutong group, which possess the highest rigidity among these country rocks (Deng et al. Reference Deng, Wang, Huang, Wan, Yang and Gao2006). In the northern part of the stock (site TL01 in Fig. 2), the bedding of Permian limestone strikes NE–SW and dips to the NW at c. 50° with weak recrystallization of the limestone (Fig. 4a). Moreover, according to the field survey in the Tongguanshan orefield, the lower Permian strata in its NE margin are folded (Fig. 5). In the SE part of the Tongguanshan stock, the hornfels-facies Devonian sandstone is well preserved and exposed in the thermal aureole (i.e. sites TL05, TL07, TL08 and TL09 in Fig. 2). The bedding of the Devonian sandstone presents consistent NE–SW strikes with dip angles varying over 54–66° (Fig. 4b–f). Moreover, the folds of the Devonian strata developed along the contact zone show SE vergence (Site TL07 in Fig. 4c). Similar structures are also recorded in the sites far away from the stock, for example, the crenulation of shale (Fig. 4d) and thrust (Fig. 4e) can be observed at sites TL08 and TL09, respectively. These consistent kinematic indicators were revealed in and far away from the stock–country-rock contact zone, suggesting that the country rocks had suffered a top-to-SE thrusting before the magma emplacement. However, due to the urbanization on the western side of the stock, no available country rocks and stock exposures can be observed on the surface.

Fig. 4. Field observations of the country rocks of the Tongguanshan stock: (a) bedding of the Permian limestone in the northern stock margin; (b, c) Devonian sandstone country rocks along the contact; and (d–f) Devonian sandstone of the country rock with certain distance to the stock, equal-area projection of the bedding of the strata inserted.

Fig. 5. Representative cross-sections along the Tongguanshan stock, according to the drilling data.
The Tongguanshan stock is also characterized by voluminous skarn-type copper deposits, which are dominantly located in the northeastern, eastern and southeastern parts of the stock, and the orebodies are mainly developed in the contact zones with strikes parallel to the contact surface, as well as the regional structures (Fig. 5). According to Meng et al. (Reference Meng, Yang, Zeng, Xu and Wang2004) the age of mineralization is 135.48 ± 0.45 Ma in the Tongguanshan stock, that is, subsequent to the stock emplacement (c. 140 Ma; Wu et al. Reference Wu, Gao, Guo, Guo, Liu, Gao, Lei, Qin and Chen2010). Six representative profiles around the Tongguanshan stock revealed by the drilling data are presented in Figure 5, showing that the inward dip angles of the contact surface gradually decrease from c. 75° in the south (profile E in Fig. 5) to c. 40° in the north (profile A in Fig. 5).
3. Methodology and sampling
3.a. Petrofabric observation
As mentioned above, our field survey shows that no obvious emplacement-related fabrics can be observed in the Tongguanshan stock; we therefore conducted detailed microscopic petrofabric studies by thin-section observation and anisotropy of magnetic susceptibility (AMS) analysis to acquire a better understanding of its emplacement process. We collected five samples (TL05-D, TL07, TL08, TL09A and TL09B; Table 1 and Fig. 2), oriented by both magnetic and solar compasses, from the country rocks and prepared them for microscopic fabric and kinematic investigations. The samples were cut along the XZ plane, that is, perpendicular to the foliation and parallel to the lineation. One massive quartz–monzodiorite sample was also collected at site TL06 for thin-section investigation (Fig. 2).
Table 1. Country rock samples collected from the Tongguanshan area

3.b. Anisotropy of magnetic susceptibility
Being an efficient method for fabric investigation, AMS has been widely applied on magma emplacement studies in South China (Feng et al. Reference Feng, Wang, Zhang and Liang2012; Wei et al. Reference Wei, Martelet, Le Breton, Shi, Faure, Chen, Hou, Lin and Wang2014, Reference Wei, Chen, Faure, Martelet, Lin, Wang, Yan and Hou2016; Ji et al. Reference Ji, Chen, Chen, Wei, Faure and Lin2018; Liu et al. Reference Liu, Martelet, Wang, Erdmann, Chen, Faure, Huang, Scaillet, Le Breton, Shu and Wang2018 a). In the Tongling district, the AMS was also applied on the contemporaneous Fenghuangshan pluton to reveal its emplacement process (Zhang et al. Reference Zhang, Liu, Wu, Li, Di, Zang and Huang2008). As the western part of the Tongguanshan stock is inaccessible due to extensive urbanization, only four fresh outcrops in the eastern side of the stock were collected, that is, samples TL02, TL03, TL04 and TL05 (Fig. 2 and Table 2). At least seven oriented core samples were collected at each site using a portable gasoline drill. The raw core samples were cut into standard cylindrical specimens of length 2.2 cm and diameter 2.5 cm, respectively. Finally, 64 specimens were prepared for the AMS measurement. Before the measurement, the orientation of the cores without solar compass measurement was corrected by –4.2°, that is, the average of azimuth differences between magnetic and solar azimuths.
Table 2. Sampling information and site-mean anisotropy of magnetic susceptibility measurement results for the Tongguanshan stock. Lithology: quartz–monzodiorite

K m = (K 1 + K 2 + K 3)/3; P J = exp({2[(n 1 – n)2 + (n 2 – n)2 + (n 3 – n)2]}2), T = (2n2 – n 1 – n 3)/(n 1 – n 3), where n 1, n 2 and n 3 are the natural-logarithm-normalized susceptibilities K 1, K 2 and K 3, respectively.
Our AMS measurements and magnetic mineralogy experiments were conducted in the Paleomagnetism Laboratory of Nanjing University. The core samples and rock powders were analysed by the KLY-3S Kappabridge coupled with the CS3 furnace of AGICO Company. Protective argon gas was added to reduce the oxidation during the heating process. The AMS data were calculated with the open access software ANISOFT of AGICO Company. Site-mean directions are calculated from the three principal axes of the magnetic ellipsoid (K 1 ≥ K 2 ≥ K 3) with 95% confidence level, where K 1 and K 3 correspond to the magnetic lineation and pole of the magnetic foliation, respectively. Detailed explanations of the magnetic parameters are provided in Table 2. Ten cylindrical quartz–monzodiorite specimens (TL02-7B, TL02-9, TL03-2, TL03-7, TL04-2A, TL04-7, TL05-1, TL05-5, TL05-10 and TL05-13) were chosen for the oriented thin-section study, and were cut along the XZ plane, that is, perpendicular to the magnetic foliation and parallel to the magnetic lineation.
3.c. Geometric modelling of the Tongguanshan stock and its related orebodies
The recognition of the geometry of the Tongguanshan stock and its related ore bodies is pivotal to the understanding of its emplacement process, as well as the relationship between the magma emplacement and the related mineralization. However, considering its small size and complexity, as well as the surrounding intensive human activities, traditional geophysical investigation methods in this area are less efficient in determining the stock geometry. To determine the 3D geometry of the geological bodies, we therefore acquired a high-resolution digital elevation model (DEM), drilling data and regional resistivity surveying data from the Tongling Nonferrous Metals Group Holdings Company, and combined these with our structure and petrofabric investigation results in the Tongguanshan area (Table 3). We integrated and processed these data using Micromine 2014 (https://www.micromine.com/) to reconstruct the 3D surface of the geological bodies. First, the contacts of the geological bodies in the depth were digitalized from the borehole profiles. Second, outlines of adjacent contacts between similar geological units were connected using a triangulated irregular network (TIN) to obtain the contact surface. Third, the contact surface was smoothed using a discrete smooth interpolation (DSI) to approximate the real geological interface. The method for the reconstruction of the 3D geometry of the geological bodies is reported in detail by Liu et al. (Reference Liu, Zhao and Sun2012).
Table 3. Data compilation for 3D geometric modelling

4. Results
4.a. Petrofabric investigation of the Tongguanshan stock and its country rocks
According to our thin-section observation, the quartz–monzodiorite of the Tongguanshan stock is composed of plagioclase, K-feldspar, quartz, hornblende, sphene, biotite, apatite, pyrrhotite and magnetite (Fig. 6). Most of the samples present a similar magmatic texture and euhedral mineral with grain sizes ranging from 0.5 to 1.5 mm, but weak orientation in the mineral long axis direction can be observed (Fig. 6a–c). Nevertheless, sample TL05, located in the contact zone and characterized by fine-grained and irregular grain boundary of the quartz and feldspar (Fig. 6b), shows a grain boundary migration recrystallization, probably a result of the interaction with the country rocks during the magma emplacement. No consistent sub-solidus deformation fabrics were identified in the quartz–monzodiorite.

Fig. 6. (a–c) Thin-section observations of the quartz–monzodiorite of the TGS stock and (d–f) thin-section observations of the country rocks with indicated kinematic information. Ap – apatite; Bt – biotite; Hbl – hornblend; Kfs – K-feldspar; Pls – plagioclase; Po – pyrrhotite; Spn – sphene.
Country-rock samples TL05D and TL07 collected in the contact zone are characterized by homogeneous clastic texture and strong thermal alteration; these samples are not suitable for kinematic study because of their fine-grained mineral size. The other three samples of sandstone (TL08, TL09A and TL09B; Fig. 2), collected from south of the Tongguanshan stock and on the western limb of the Tongguanshan anticline, have similar clastic texture with clast grain sizes of c. 100 μm (Fig. 6d–f), and the foliation is well defined by the oriented biotite and muscovite grains. We observed ‘sigmoidal’ plagioclase and quartz grains (Fig. 6d–f) and ‘bookshelf’ mica grains (Fig. 6e), suggesting a top-to-SE movement. These phenomena further confirm that the western limb of the Tongguanshan anticline experienced a top-to-SE movement before the stock emplacement. No high-grade metamorphic minerals were identified in the country rocks.
4.b. Magnetic fabrics
4.b.1. Magnetic mineralogy analysis
A total of eight specimens were milled for the thermal magnetic mineral analysis and present similar curves of magnetic susceptibility (k) versus temperature (T); we therefore only depict two representative k–T curves in Figure 7a and b. The heating curves display a slight increasing of magnetic susceptibility during the heating process, and a rapid drop to zero at 580 °C, suggesting that the magnetite is the major magnetic susceptibility carrier in the Tongguanshan quartz–monzodiorite. The lack of Hopkinson peak in these curves suggests that the magnetite is dominated by samples of a multidomain nature (Dunlop, Reference Dunlop1974). This interpretation is also supported by the relatively high site-mean bulk magnetic susceptibility of the quartz–monzodiorite, ranging from c. 31 to 53 × 10–3 (Table 2). Accordingly, the magnetite is the dominant contributor to the magnetic fabric of the quartz–monzodiorite in the Tongguanshan stock. We calculated K 1 and K 3 of the magnetic ellipsoid for the magnetic lineation and pole of magnetic foliation, that is, the AMS result corresponding to a normal petrofabric.

Fig. 7. (a, b) Representative magnetic mineralogy investigation and (c, d) magnetic parameters of the Tongguanshan stock quartz–monzodiorite.
4.b.2. Features of the magnetic parameters
Most of the measured specimens show a degree of anisotropy (P J) greater than 1.1 (Fig. 7c; Table 2), and these high values may be related to the high concentration of magnetite as opposed to the post-emplacement deformation (Rochette et al. Reference Rochette, Jackson and Aubourg1992). The preferred orientation of magnetic minerals, which is another cause of degree of anisotropy, may be due to the magma flow or interaction between magma and country rocks. Values of P J are higher in the sites located closest to the stock–country-rock contact (Fig. 7c), implying that high values of P J may be due to the result of the interaction between magma and country rocks. The shape parameter (T), representing the shape of the magnetic susceptibility ellipsoid, is divided into two groups: oblate (0 < T < 1) and prolate (–1 < T < 0). The majority of the specimens are located in the oblate domain (Fig. 7d; Table 2), implying that the planar fabric is better developed than the linear fabric.
4.b.3. AMS of the Tongguanshan stock
We present the AMS results by equal-area projections (lower hemisphere) with the three principal axes, site-mean value and 95% confidence level (Fig. 8; Table 2). The sites near the margin of the stock (i.e. TL02, TL04 and TL05) are characterized by well grouped inwards-dipping and sub-vertical to vertical (45–80°) magnetic foliations with strikes parallel to the stock boundary (Fig. 8). The magnetic lineations of these sites, which may record the trend of magma flow, are horizontal to sub-horizontal (Fig. 8; Table 2). However, site TL03 (c. 200 m to the stock boundary) presents vertical NE–SW-striking magnetic foliations parallel to the regional structures; however, the magnetic lineations show variable plunges (Fig. 8).
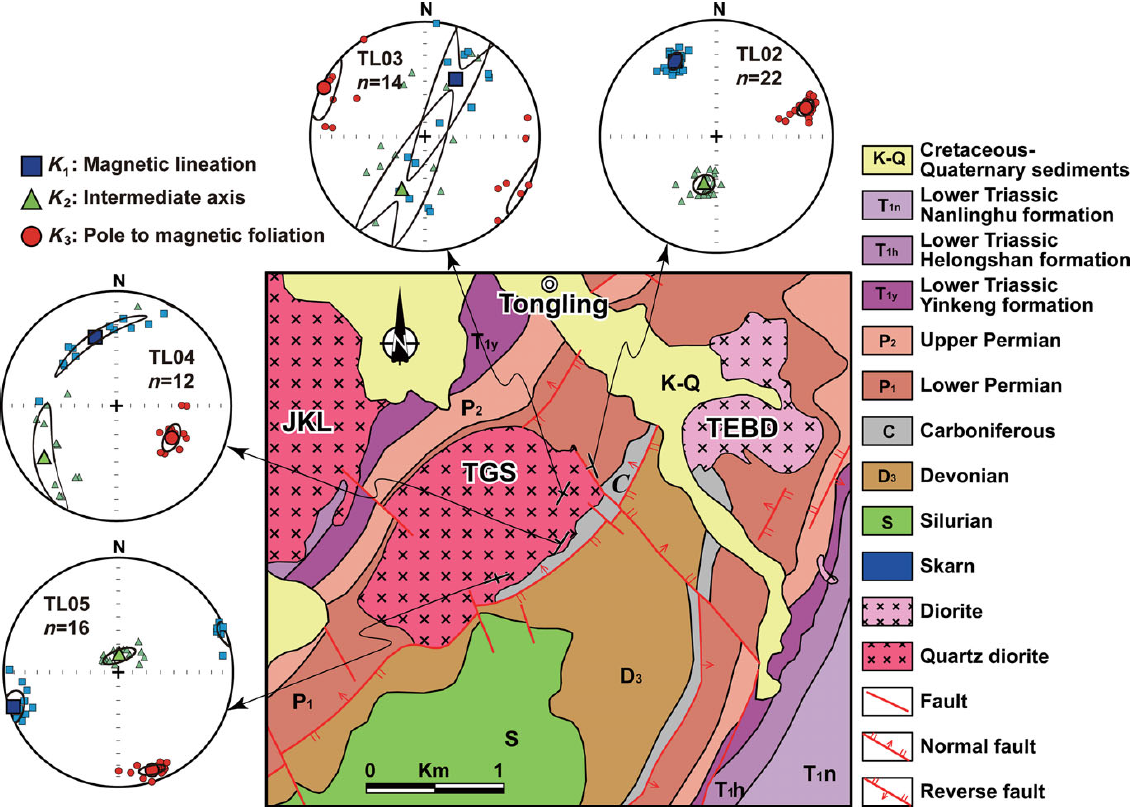
Fig. 8. AMS Tongguanshan stock results.
4.c. Stock geometry and the occurrence of the orebodies
By detecting the contact surface from the drilling profiles and 3D modelling, we can build the geometry of the Tongguanshan stock in the depth (Fig. 9). Several features are highlighted in our 3D geometric model. First, the shape of the contact boundary in the eastern side is much more complicated than in the western side (Fig. 9a). Second, the Tongguanshan stock has a tongue-like geometry with a sharp wedge out at –2 km (dashed line in Fig. 9b). We therefore estimate the volume of the Tongguanshan stock as c. 1.25 km3. Third, the stock–country-rock contact surface generally dips to the NW in both east and west sides with dip angle steepening when moving to the depth, and is steeper in the west side than in the east side (Fig. 9c). Fourth, the orebodies are mainly located in the east side of the stock and the highest reservation is revealed in the NE margin. The extent of the orebodies is roughly parallel to the stock–country-rock contact surface.

Fig. 9. 3D geometry of the TGS stock (pink) and its related ore bodies (yellow) reconstructed by the drilling and regional resistivity surveying data, view from the (a) top, (b) east and (c) south. Dashed line part represents the modelled geometry in the depth.
5. Discussion
5.a. Emplacement mechanism of the Tongguanshan stock
5.a.1. Implications of the petrofabrics for the magma emplacement
Our field and microscopic observations revealed that the Tongguanshan stock has a macroscopically isotropic appearance. Although the values of degree of anisotropy are mainly > 1.1, probably because of the high concentration of magnetite (Fig. 7d), no sub-solidus deformation has been identified in the stock and its country rocks. We therefore propose that the Tongguanshan stock has not experienced a strong post-emplacement deformation related to a regional tectonic event. As a result, the magnetic fabrics developed in the stock are primary and reflect the magma emplacement process and the interaction between the magma and its country rocks, and the magnetic lineation may indicate the magma flow direction (Rochette et al. Reference Rochette, Jackson and Aubourg1992).
The Tongguanshan intrusion was considered as a stock and defined as a diapirically emplaced body in previous studies (Xu et al. Reference Xu, Fun, O’Reilly, Jiang, Griffin, Wang and Qiu2004; Lei et al. Reference Lei, Wu, Gao, Guo, Liu, Guo, Gao, Chen and Qin2010; Wu et al. Reference Wu, Gao, Guo, Guo, Liu, Gao, Lei, Qin and Chen2010; Yang et al. Reference Yang, Du, Li, Li and Cao2015). Previous studies on the diapiric emplaced intrusions show that such a body is usually characterized by a spherical shape, compositional zonation, circular pattern of steep foliation and lineation, and the foliation and lineation parallel to the planar and linear fabrics developed in its aureole (e.g. Dixon, Reference Dixon1975; Cruden, Reference Cruden1990; He et al. Reference He, Xu and Paterson2009; del Potro et al. Reference del Potro, Díez, Blundy, Camacho and Gottsmann2013). However, our AMS results reveal that the sites in the stock margin show a dominantly inwards-dipping magnetic foliation with the strike parallel to the stock boundary (i.e. sites TL02, TL04 and TL05 in Fig. 8). The most pronounced feature of these sites is that they are characterized by a sub-horizontal magnetic lineation (dip angle < 45°; Fig. 8; Table 2), which is contrary to the usually steep magnetic lineation pattern of the diapiric pluton revealed by previous studies (e.g. Cruden, Reference Cruden1990; He et al. Reference He, Xu and Paterson2009). We therefore consider that the one-time magma tank diapiric emplacement model seems incompatible with the Tongguanshan stock.
The remarkable feature of the Tongguanshan stock AMS result is the parallelism of the magnetic foliation and the NE–SW-trending fold–thrust belt of the country rock (Fig. 8), indicating that the emplacement of the Tongguanshan stock was either controlled by the NE–SW extended pre-emplacement structures of the country rocks, or the stock was constructed by NE–SW-striking dykes.
5.a.2. Space for magma emplacement
Previous geochemistry and geochronology studies of the Tongguanshan stock suggest that the stock has similar composition at the margin and in the centre. Zircon SHRIMP U–Pb dating of the Tongguanshan quartz–monzodiorite yields similar crystallization ages within the error bars – 139 ± 3, 139.5 ± 2.9 and 141.8 ± 1.0 Ma – suggesting that the duration of construction of the stock was relatively short (Du et al. Reference Du, Qin and Tian2004; Wang et al. Reference Wang, Zeng, Yang, Meng and Tian2004; Wu et al. Reference Wu, Gao, Guo, Guo, Liu, Gao, Lei, Qin and Chen2010). The short duration is also supported by the small stock volume (c. 1.25 km3) revealed by the 3D geometric modelling; as proposed by de Saint Blanquat et al. (Reference de Saint Blanquat, Horsman, Habert, Morgan, Vanderhaeghe, Law and Tikoff2011), the emplacement duration has a linear relationship with the volume of intrusion. Considering that the regional tectonic strain rate is usually much lower than the magma supply rate (Price, Reference Price1975), the contribution of syn-magmatism regional tectonics to the creation of the emplacement space can be neglected. The NE–SW-striking and SE-dipping garnet- and magnetite-bearing veins developed in the eastern margin of the Tongguanshan stock, which correspond to the Early Cretaceous regional extension in the NW–SE direction (Fig. 3b, c; Wang et al. Reference Wang, Wyman, Xu, Zhao, Jian and Zi2007; Li et al. Reference Li, Zhao, Zhou, Ma, Sergio de Souza and Vasconcelos2009; Deng et al. Reference Deng, Wang, Xiao, Yang, Liu, Gong and Zhang2011; Xie et al. Reference Xie, Yang, Sun and Du2012), cross-cut the NW-dipping magnetic foliation developed in the stock, suggesting that the regional extensional event occurred after the magma emplacement. As mentioned above, our field and thin-section observations also show that the stock is characterized by magmatic texture and fabrics developed in the country rocks before the magma emplacement (Figs 4, 6). We therefore believe that the Tongguanshan stock emplacement was possibly not influenced by the regional tectonic regime. Alternatively, the magma pressure from the accreted magma chamber may be considered as the principal space-creating force for magma emplacement.
The emplacement depth of the Tongguanshan stock is estimated at c. 3–5 km, that is, the brittle upper crust level (Du et al. Reference Du, Qin and Tian2004; Cao et al. Reference Cao, Du, Pang, Li, Zhang and Zhang2009; Lei et al. Reference Lei, Wu, Gao, Guo, Liu, Guo, Gao, Chen and Qin2010); the mechanical discontinuity interfaces in the country rocks are therefore preferable for the magma emplacement, and the re-arrangement of the country rocks also partly accommodated the intrusions (e.g. Gudmundsson, Reference Gudmundsson2011; Menand, Reference Menand2011; Schofield et al. Reference Schofield, Brown, Magee and Stevenson2012). For example, the shouldering effect observed at the western margin of the Tongguanshan stock suggests the lateral movement behaviour of the country rock (Fig. 2). The stock–country-rock contact profiles in the east side revealed by the drilling data reveal that the dip angle of the contact surface gradually decreases from c. 65° in the south to c. 45° in the north (Fig. 5), indicating that the space for magma emplacement was partly accommodated by the downwards displacement of the country rocks.
Accordingly, we propose that the space for the emplacement of the Tongguanshan stock was mainly created by magma pressure from the magma chamber along the inherited regional structures in the country rocks, and partially associated with the lateral and downwards country rock displacement.
5.a.3. Emplacement mechanism of the Tongguanshan stock
The reconstructed 3D geometry of the Tongguanshan stock shows that the west contact surface is much steeper than the east contact surface and the root of stock is in the SW part of the stock (Fig. 9b, c), indicating that the magma emplacement was possibly initiated at the western stock margin. The crystal grain size in the stock shows a gradually fining trend from the centre to the east stock margin (Figs 2, 6). In addition, the strike of the magnetic foliation at sites TL02 and TL04 is parallel to the NW–SE-trending stock boundary, where the lower Permian strata are folded (Figs 5, 8), suggesting that the magma was accumulated at the pluton northern part. It therefore seems reasonable to assume that the magma emplacement was initiated at the southwestern part of the stock.
Site TL03 has the furthest distance to the stock margin and lowest degree of anisotropy value compared with other sites (Fig. 8), indicating that the magmatic fabric of site TL03 is mainly produced by magma emplacement. The understanding of the magnetic fabrics at site TL03 is therefore important to reconstruct the accretion process of the stock. Site TL03 is characterized by vertical NE–SW-striking magnetic foliations with variable plunges of magnetic lineation (Fig. 8). This is different from the inwards-dipping magnetic foliation and horizontal to sub-horizontal magnetic lineation in the stock margin, suggesting that site TL03 may be derived from different magma pulses with respect to other sites in the stock margin, instead of just a single magma intrusion. The relatively lower degree of anisotropy of site TL03 compared with sites TL02, TL04 and TL05 supports this idea (Fig. 7b). However, the accretion process is still not clear due to the lack of exposure in the western part of the Tongguanshan stock. We consider that there are two possibilities of forming the magnetic fabric of site TL03, and therefore two corresponding emplacement models were proposed. One is that site TL03 represents one of the magma pulses intruded along the NE–SW-extended pre-emplacement structures in the country rock with unilateral E-wards accretion trend (Model 1 in Fig. 10a). The other is that site TL03 belongs to the youngest magma pulse squeezed into the early emplaced magma, that is, bilateral magma accretion process (Model 2 in Fig. 10b). The ambient stress from earlier emplaced magma and country rocks therefore resulted in the NE–SW-extended magnetic foliation.

Fig. 10. Cross-sections through the conceptual model of the TGS stock in the E–W direction (not to scale). I, II and III represent the gradually emplaced magma pulses.
In summary, we conclude that the Tongguanshan stock was constructed by multiple magma pulses emplaced along the NE–SW-trending pre-emplacement structures in the country rock. The magma emplacement was initiated in the western part of the stock with either unilateral or bilateral magma accretion trend.
5.b. Influence of magma emplacement on the localization of related skarn type orebodies
The influence of magma emplacement on the related orebody localization mainly included the modification of the thermal fluid composition and pressure condition (e.g. Ray et al. Reference Ray, Webster and Ettlinger1995; Audétat et al. Reference Audétat, Günther and Heinrich2000; Vallance et al. Reference Vallance, Cathelineau, Boiron, Fourcade, Shepherd and Naden2003; Gerbault, Reference Gerbault, Healy, Butler, Shipton and Sibson2012), the change of the circulation and accumulation of the hydrothermal fluid flow in the aureole (e.g. Boyce et al. Reference Boyce, Fulignati and Sbrana2003; Lu et al. Reference Lu, Liu, Wang, Xu and Li2003; Eldursi et al. Reference Eldursi, Branquet, Guillou-Frottier and Marcoux2009; Luan et al. Reference Luan, Song, Chen, Zheng, Zhang, Yu, She, Tian and Ran2014), and enhancement of the permeability and temperature gradients of the country rock (e.g. Nehlig, Reference Nehlig1994; Matter et al. Reference Matter, Goldberg, Morin and Stute2005; Gonnermann & Manga, Reference HM, M, Fagents, Gregg and Lopes2009). Previous geochemical studies in the Tongguanshan orefield suggested that the origin of the hydrothermal fluid is mainly from the Tongguanshan stock (Yuan, Reference Yuan2002; Tian et al. Reference Tian, Ding, Hou, Yang, Xie, Wang and Wang2005).
Our stock emplacement model suggests that the Tongguanshan stock was constructed by successively emplaced magma pulses along the NE–SW-striking fractures in the Tongguanshan anticline from west to east (Figs 11, 12). Consequently, the country rocks in the eastern side were heated and deformed by the successively emplaced magma arrivals. The variable inclination of the contact surface in the eastern side, the highly irregular stock–country-rock boundary and grain boundary migration recrystallization of the quartz–monzodiorite in the eastern margin (Fig. 6) also suggest that the country rocks on the eastern side experienced a stronger deformation than that on the western side (Fig. 11). The fracture of the country rocks may therefore be more developed in the eastern side, which may enhance the hydrothermal fluid and larger skarnization in the country rocks.

Fig. 11. Equal-area lower-hemisphere spherical projections of the stock–country-rock contact surface and its related skarn deposit. The pink and yellow spherical projections represent the stock-country rock surface and its related orebodies, respectively. The red and blue points in the image are the nodes of the Triangular Irregular Network (TIN) of analysed zone.

Fig. 12. Schematic magma emplacement and hydrothermal fluid flow pattern of the Tongguanshan stock (not to scale).
Recent studies show that the stock geometry, especially the curvature of the intrusion–country-rock contact surface, strongly controls the localization of orebodies (Cao et al. Reference Cao, Liu, Liu and Lai2020); the curvature of the contact surface affects the distribution of the dilation zone around the pluton, where the fluid pressure drops and ore depositing occurs (Liu et al. Reference Liu, Zhao and Sun2012; Sun & Liu, Reference Sun and Liu2014; Cao et al. Reference Cao, Liu, Liu and Lai2020). To better understand the constraints of the stock geometry variance on its associated orebodies, we therefore chose five zones (Z1, Z2 and Z3, associated with copper skarn deposit, and Z4 and Z5, devoid of ore deposit) around the Tongguanshan stock contact with width and depth of c. 200 m (Fig. 11). From the comparative analysis of the variation of stock contact surface and extension of the orebodies along the contact surface, we can possibly correlate the stock geometry and ore deposits.
The equal-area projections of the contact surface of the stock in zones Z1, Z2 and Z3 show that the extension of the orebodies (yellow stereograms in Fig. 11) is parallel to the stock–country-rock contact surface, and the dip of the stock contact surface ranges over c. 45–65° (purple stereograms in Fig. 11). However, in zones Z4 and Z5, the dip of the contact surface is between 56° and 83°, which is statistically steeper than those in zones Z1, Z2 and Z3 (Fig. 11), but the strike of the contact boundary is oblique to the regional structures and no industrial mineral deposit was revealed in these zones. Furthermore, strong sericitization was observed in the quartz–monzodiorite along the cleavage, which is parallel to the strike of the magnetic foliation (Fig. 3c) and the long axis of crystal grain in the country rocks (Fig. 6d–f). The sericitization was related to the hydrothermal fluid flow during magma emplacement, suggesting that the hydrothermal fluid flow was also along the strike of the regional structures. Consequently, the genesis of the skarn-type ore deposit was mainly dependent upon the interaction between the stock-derived thermal fluid and the country rock. We therefore suggest that the parallelism among the strike of the contact surface, regional structures and direction of fluid flow facilitated the ore development.
Generally, the lower the value of maximum density of stock contact surface, the more complex the curvature of the contact surface. Our study reveals that the value of maximum density of contact surface increased from Z1 to Z3 (5.3% to 17.5%), and almost doubled at Z4 and Z5 (28.8% to 30.7%, Fig. 11). However, the ore reservation decreased from Z1 to Z5, indicating that the curvature of the contact surface is positively correlated with the ore reservation. From a comparative analysis of these five zones, we consider that the curvature of the contact surface is equivalent to the distribution of the dilation zone, important ore-controlling factors in an intrusion-related ore deposit.
We therefore argue that the emplacement process, that is, the stock–country-rock contact surface geometry, emplacement depth and magma accretion process, may be an essential ore-controlling factor for the ore deposit localization. This idea can be used to guide further prospecting for large-scaled intrusion-related deposits, which are usually constructed from numerous magma batches. However, to obtain a more comprehensive understanding of the magma emplacement and its effect on the related mineralization process, detailed 3D emplacement process studies on a developed mining area are needed.
6. Conclusions
From our field and thin-section observations, AMS, and 3D geometry studies of the Tongguanshan stock and its related orebodies, we make the following conclusions.
-
(1) The Tongguanshan stock is composed of macroscopically isotropic quartz–monzodiorite with a ‘tongue-like’ geometry and a sharp wedge of c. 2 km depth in the western side of the Tongguanshan stock.
-
(2) The magma emplacement was initiated in the western side of the Tongguanshan stock, and the magma ascended along inherited NE–SW-striking fractures developed in the Tongguanshan anticline. The successive magma arrivals were either unilaterally accreted to the east side of the stock or bilaterally accreted by a succession of NE–SW striking magma pulses.
-
(3) The strike, dip angle and curvature situation of the stock–country-rock contact surface, which may affect the water–rock reaction process and distribution of the dilation zone, are important ore-controlling factors.
Acknowledgements
We thank Xiao Fuquan, Wu Shuo, Li Min and Chen Bin for their help with sample collection, and Hu Xuzhi (the Paleomagnetism Laboratory of the Nanjing University) and Tao Shuai (Northwest University) for their help with AMS measurements. We also thank Carl Stevenson and one anonymous reviewer for their constructive comments and suggestions. The provision of the drilling data from the Tongling Nonferrous Metals Group Holdings Company was greatly appreciated. Our study received financial support from the National Natural Science Foundation of China (grant nos 41372338 and 41772351).
Conflict of interest
None.