INTRODUCTION
As Sumberg (Reference Sumberg2012) has observed about the renewed interest in agriculture for development: what goes around comes around. After decades of absence, attention to the yield gap in agricultural production has returned in recent years in such initiatives as the Global Yield Gap and Water Productivity Atlas project (www.yieldgap.org) which aims to provide new data on yield gaps from around the world. The yield gap is hardly a new concern in agronomy, crop production ecology or agricultural economics. Indeed, early attention to the subject can be traced to Gomez’ work in 1977 and continues in fits and starts to the present day. What supports this revitalization of the yield gap and gives it renewed urgency is the ever present narrative of ‘how will we feed the world's 9 billion people’ in 2050 (Bruinsma, Reference Bruinsma2009).
To find out whether there are important omissions in studies and approaches to the yield gap, we reviewed a portion (62 papers) of the rather vast number of papers produced on the topic. We selected publications for review based on literature searches with the key words ‘yield gap’, ‘yield potential’ and by then consulting the references of papers that are widely cited on the topic and written my scientists known for the work on the topic. The resulting top publications (62) were then reduced by eliminating apparent duplicates (same paper presented various times) and excluding publications of a more general nature (e.g. introductory chapters). Papers that were widely cited were prioritized (such as those of Van Ittersum M. and Cassman, K.) as well as including special journal issues that focussed on the topic. The review discovered some of the complexities in yield gap studies and also exposed some significant lacunae. This paper highlights some of the findings from this review and outline areas of research we think are critical if yield gaps are to be better understood, but more importantly addressed in future.
Yield gaps are only important insofar as they relate to meeting important needs in food, fuel or other products. Yield gap research is part of a wider body of work looking at increasing agricultural productivity, the latest of which focuses on ‘sustainable intensification’ (Garnett and Godfray, Reference Garnett and Godfray2012). Historically, most research focussed on crop breeding, fertilizer application and other technical solutions. However, there is scope for addressing the issue of agricultural productivity with a much wider lens that will include both attention to the social, economic and political context, the possible ecological impacts of increasing productivity, as well as nutrient security and social equity. Whilst the narrative power of feeding 9 billion is compelling, all narratives should be examined with a critical eye as they spur us to frame certain problems in sometimes overly narrow ways that may benefit some more than others. As a recent paper on yield gaps points out, ‘[M]aking progress on food security requires more than a biological view’ because yields ‘are an object of choice for farmers’ (Beddow et al., Reference Beddow, Hurley, Pardey and Alston2015: 28). Understanding farmers’ choices and decisions is rarely tackled in yield gap research.
The yield gap concept is useful to government planners, implementers, crop breeders, agronomists and the private sector in particular because it informs estimates of future crop yields for any given region and type of crop. It thus supports targeting top-down interventions and the technologies and inputs they require. It also can lead to a narrow approach that fails to address the wider contextual factors that affect yields such as market access, consumer tastes, trade policies, etc.
DEFINING THE GAP
Gomez’ (Reference Gomez1977) early definition focussed on the difference observed between yields produced on experiment stations and actual farm yields. In subsequent literature, further refinements to this basic definition were developed to include attention to spatial and temporal scale (Lobell et al., Reference Lobell, Cassman and Field2009) and variation in management practices (Cassman, Reference Cassman1999; Foley et al., Reference Foley, Ramankutty, Brauman, Cassidy, Gerber, Johnston, Mueller, O'Connell, Ray, West, Balzer, Bennett, Carpenter, Hill, Monfreda, Polasky, Rockström, Sheehan, Siebert, Tilman and Zaks2011). Yield gap definitions by necessity actually focus on yield potential which Lobell et al. (Reference Lobell, Cassman and Field2009) argued can lead to inconsistencies in analyses and thus a plethora of yield gap ranges. Gomez (Reference Gomez1977) divided the yield gap into two types, the gap between experiment station and farmers’ fields and the gap between potential farm yield and actual farm yield. He singled out environmental differences between experiment station and farmers’ fields but also, in the second gap, pointed to biological and social-economic constraints. The FAO (2000) modified Gomez’ model to include a theoretical potential yield (Figure 1).
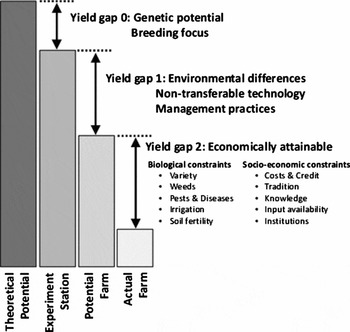
Figure 1. Yield gap as defined by FAO (2000).
Fischer et al. (Reference Fischer, Byerlee and Edmeades2009) added another dimension to yield potential, that of economically attainable yield. This category was further broken down to two types: one given current market conditions and institutions and the other assuming efficient markets and institutions, thus based on a theoretically optimum market and institutional environment.
Research has further devoted attention to yield-defining, yield-limiting and yield-reducing factors (Tittonell and Giller, Reference Tittonell and Giller2013) and differentiated between irrigated and rainfed systems (van Ittersum et al., Reference Van Ittersum, Cassman, Grassini, Wolf, Tittonell and Hochmand2013). It is important to note that much of the discussion on yield gaps indicates that reaching ideal yield potential is largely impossible given that models assume a near perfect set of enabling factors for both management practices and environmental conditions.
The concept of yield itself has generated a number of conceptual difficulties as different authors and disciplines use the terminology differently. Table 1 illustrates the breadth and variety of definitions across disciplines.
Table 1. Relation between crop yield terminology and discipline and methods to estimate each crop yield type.
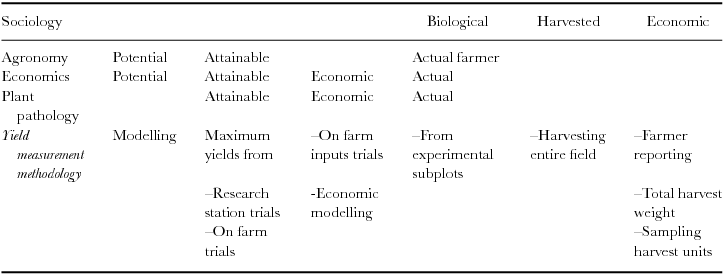
Adapted from Fermont and Benson (Reference Fermont and Benson2011).
MEASURING THE GAP
Efforts to understand what constrains yields have focussed primarily on abiotic (nutrients, water) and biotic factors (pests, weeds and disease). Some attention, if far less, has been focussed on socio-economic and infrastructural factors (Waddington et al., Reference Waddington, Li, Dixon, Hyman and Vicente2010). Emerging from the literature is also a recognition that in many parts of the world there is yield stagnation – affecting rice in Japan and China, maize in China, Italy and France and wheat in northern Europe and India (Cassman, Reference Cassman2010; Ray et al., Reference Ray, Ramankutty, Mueller, West and Foley2012; van Wart et al., Reference Van Wart, Kersebaum, Peng, Milner and Cassman2013).
Methods of measuring yield gaps differ according to scale. Site specific or local analyses employ primarily four different approaches: (i) crop model simulations; (ii) field experiments; (iii) yield contests and (iv) maximum farmer yields.
Crop modelling arose in the early 1960s and utilizes computer algorithms to represent crop growth, development and yield together with simulations of soil conditions, weather and management practices (Van Keulen and Wolf, Reference Van Keulen and Wolf1985). Modelling allows researchers to extrapolate patterns of crop growth and yield beyond a single site. Field experiments provide a useful complement to models to generate real data from on-the-ground experiments. Working more directly with farmers on their fields, yield contests use competition and incentives to boost production and thus, with strong controls and monitoring, provide good estimates for attainable yields. Understanding the maximum farm yield potentials can also be obtained through gathering historical data from farmers. All of these methods have their limitations. All share a restriction on scale, limited as they are to localized, site specific contexts.
Other methods have been used which attempt to overcome these limitations of scale. These techniques include remote sensing and GIS analysis. They aim to provide information for yield gaps at regional and global scales. These approaches, whilst furthering understanding at a much larger scale, also have their drawbacks. Van Wart et al. (Reference Van Wart, Kersebaum, Peng, Milner and Cassman2013) and van Ittersum et al. (Reference Van Ittersum, Cassman, Grassini, Wolf, Tittonell and Hochmand2013) have provided critiques of global scale analyses which draw upon these methods noting the following shortcomings: different definitions of yield potential are used in different papers; data are not disaggregated into irrigated and rainfed crops; interpolation of data causes errors; data are too coarse; single generic crop models or statistical procedures are used for the world masking differences in crop management practices; model calibration and evaluation is not always transparent; and finally there are too many assumptions in each model. Whilst deploying different methods is useful, it also means that it can be difficult to impossible to compare the results from different studies.
THE INFLUENCE OF CLIMATE CHANGE
The increase in temperature, atmospheric CO2 concentrations and changes in rainfall patterns that occur as a result of climate change is particularly important in influencing crop yields. Timing and amount of rain will affect crop production globally but is of greatest possible threat to farmers relying solely on rainfed production. Worldwide, it is predicted that maize and wheat will suffer losses and the average change at a global level will be − 8% by 2050 for both Africa and Asia (Knox et al., Reference Knox, Hess, Daccache and Wheeler2012). Various models, however, produce different results, and many of the limitations and drawbacks outlined above (van Ittersum et al., Reference Van Ittersum, Cassman, Grassini, Wolf, Tittonell and Hochmand2013; Van Wart et al., Reference Van Wart, Kersebaum, Peng, Milner and Cassman2013) apply. Besides, often model estimates assume static conditions, meaning that the same crop varieties and management conditions that are used and practiced today, would also still prevail in 2050 (or beyond), even if conditions had changed considerably. These issues undermine the credibility of biophysical model-based results of the impact of climate change on yields and calculated yield gaps, unless these are corrected for attenuating impacts of adaptation to climate change, through changes in crop management (e.g. planting date or N-fertilizer rates; Valdivia et al., Reference Valdivia, Antle, Rosenzweig, Ruane, Vervoort, Ashfaq, Hathie, Homann-Kee Tui, Mulwa, Nhemachena, Ponnusamy, Rasnayaka, Singh, Rosenzweig and Hillel2015) or switching crops and/or farming systems (Rippke et al., 2016). Nevertheless, Wheeler and von Braun (Reference Wheeler and von Braun2013) argue that despite the variations, the evidence suggests that the impact of climate change on crop yields will be greatest in the tropics and that the effects will be exacerbated in areas which already experience food insecurity. A recent study by the UK–US task force on Extreme Weather and Global Food System Resilience (2015) presents evidence that the global food system is vulnerable to production shocks caused by extreme weather, and that this risk is growing.
THE SOCIAL, ECONOMIC AND POLITICAL CONTEXT
Whilst the yield gap literature frequently mentions the challenges presented by socio-economic dynamics, very few papers actually incorporate these factors into their models or analysis. This omission reflects the general absence of this perspective in the wider agronomy literature and research. When socio-economic factors are considered, they tend to be quite narrowly focussed on economic elements at a household or farm scale. Farm inefficiencies, market access, farm management practices, level of knowledge, labour availability, availability of capital, presence of farmer associations, infrastructure and institutional factors such as government support programmes, strength of extension services and access to credit are all elements explored in these papers (Baldos and Hertel, Reference Baldos and Hertel2012; de Koeijer et al., Reference De Koeijer, Wossink, van Ittersum, Struik and Renkema1999; Hoang, Reference Hoang2013; Neumann et al., Reference Neumann, Verburg, Stehfest and Müller2010; Schreinemachers, Reference Schreinemachers2005; van Dijk et al., Reference Van Dijk, Meijerink, Rau and Shutes2012). Areas left underexplored in this literature are other factors influencing farmers’ decisions such as risk aversion, opportunity costs, seasonal and permanent out-migration, land holding types, distance to plots and location on the landscape, food and crop preferences, health and presence and importance of other income-generating activities.
CLOSING THE GAP: ADDRESSING WEAKNESSES IN YIELD GAP RESEARCH
The considerable body of research on yield gaps has led to methodological advances for measuring the gap, determining where gaps at both local and global scales are most pronounced, and identifying key constraints to closing the gap. However, gaps remain in yield gap research and there are implications of using the yield gap to address food security that are not well explored in the literature. Any new approach that addresses yield gaps must first identify the underlying constraints to agricultural production which include a wide range of biophysical to social, economic and political factors. There are usually many constraints operating in any one given context, varying over space and time (Fischer et al., Reference Fischer, Byerlee and Edmeades2009; Harris and Orr, Reference Harris and Orr2014). For example, nitrogen deficiency may be found in combination with poor market access and lack of livestock. A technical fix of increasing fertilizer use will only help if market access is improved and products are reliable and affordable.
Improved crop breeds, conservation agriculture with minimal soil disturbance, increasing access to credit, integrated pest management, integrated soil fertility management and improving infrastructure (markets, roads, water) have all been proposed as possible solutions to closing the yield gap. Any possible intervention, however, must be tailored closely to the specific context in which it is being proposed. This tailoring, especially including farmer's views and priorities, is most often absent in efforts to close the gap. In addition, any yield gap will undoubtedly require a combination of interrelated interventions.
As mentioned previously, whilst socio-economic constraints are acknowledged, in 62 papers reviewed related to yield gaps, less than 13% papers had models which included socio-economic factors. The emphasis in the majority of these papers was on biophysical factors and most (48.4%) did not even mention socio-economic influences. In a special issue of Field Crops Research (Van Ittersum and Cassman, Reference Van Ittersum and Cassman2013) which focussed on yield gap analysis, only one paper (van den Berg and Singels, Reference Van den Berg and Singels2013) examines trends in the economics of the specific crop in their study; 50% of the papers do not even mention the phrase socio-economics. The Global Yield Gap Atlas (GYGA) project, which is meant to examine underlying causes of the gap, does not yet include socio-economic data (http://www.yieldgap.org/web/guest/methods-overview). However, Wageningen University in the Netherlands has begun a project specifically to evaluate economic and infrastructural constraints for agricultural productivity in sub-Saharan Africa. It is expected that these data will be linked eventually to the GYGA. Given that closing the yield gap is highly dependent on farmers’ decisions and actions, the lack of information on farmers’ perceptions, choices, constraints and decision-making is striking. Furthermore, the biggest yield gaps are in developing countries in locations where poverty, inequity and food insecurity are the greatest and arguably where socio-economic constraints are most criticalFootnote 1 .
Another notable absence in the literature is attention to the potential ecological impacts of closing the yield gap. The ‘business-as-usual’ approach to agriculture has resulted in serious environmental impacts. Whilst many authors raise concerns about damage to the environment (including Cassman, Reference Cassman1999; Cassman, Reference Cassman2010; Cassman and Wood, Reference Cassman, Wood and Hassan2005; FAO, 2014; Foley et al., Reference Foley, Ramankutty, Brauman, Cassidy, Gerber, Johnston, Mueller, O'Connell, Ray, West, Balzer, Bennett, Carpenter, Hill, Monfreda, Polasky, Rockström, Sheehan, Siebert, Tilman and Zaks2011; Godfray et al., Reference Godfray, Beddington, Crute, Haddad, Lawrence, Muir, Pretty, Robinson, Thomas and Toulmin2010; Hall and Richards, Reference Hall and Richards2013; Monfreda et al., Reference Monfreda, Ramankutty and Foley2008; Ramankutty et al., Reference Ramankutty, Evan, Monfreda and Foley2008), there appears to be little consensus on how best to address it, though a multitude of approaches have been proposed and tested in certain settings. These include organic farming, conservation agriculture, integrated pest management, water harvesting and various other approaches grouped under ‘sustainable agriculture’.
THE FARM AND LANDSCAPE GAP
Yields on farmers’ fields are part of a farm and wider landscape system that complicate both the measurement and understanding of yield gaps, but also the constraints that underlie them and shape farmers’ decisions and ability to increase productivity. This heterogeneity in the biophysical, social and economic landscapes is rarely a feature in the yield gap literature. If the point of closing the yield gap is to raise productivity and thus address food security, then surely it becomes critical to understand and address this wider landscape. Perhaps overall farm productivity, that meets both food and cash needs, can be achieved but may come at the expense of yield in a particular crop. If viewed too narrowly (for example, via commodity based research programmes), this crop yield may be viewed as a decline in production. But, if the farm is viewed as an entire system, the productivity might actually be increasing. This increase in farm productivity may then again in turn be a part of a larger increase across the landscape and may then have effects on the supporting natural resource base, as well as regional and global agricultural markets.
The technical focus on closing the yield gap presupposes that farmers will adopt new technologies to increase productivity if they are available and proven to increase crop yields. As Schreinemachers (2006) points out, this perspective, which is rooted in a western bias and experience, does not take into account or understand farmers’ objectives and challenges, particularly in the developing world. Land tenure, availability of labour, opportunity costs (intensifying production rather than selling one's labour for immediate cash needs), food crop preferences entrenched in taste and texture, avoidance of risk that would lead to total crop failure, strategies of diversification to ensure food and cash products are just a few of the factors that have a bearing on yield gaps in the developing world. Yield gap models tend to be based on single cropping and do not reflect the realities of intercropping and mixed crop livestock systems on farmers’ lands. If an increase in one crop is sought, it will often come at the expense of another crop or another activity. Schreinemachers (2006) noted that an increase in production of an additional ton of rice will result in a reduction of 390 kg of other cereals and 400 kg of groundnut in the Gambia. In Niger, farmers prioritized risk reduction due to erratic weather patterns and thus rejected packages designed to increase their productivity because they feared these interventions would put them at greater risk to variable rainfall.
Farmers encounter numerous obstacles to increasing yields, as well as many disincentives to intensifying production. In Tanzania, farmers in the Kilombero Valley adopted the system of rice intensification in recent years and have had great success in improving yields and this increase has led, largely, to improved livelihoods. However, in 2014, the production of smallholders and large estates was complemented by cheap imports from outside Tanzania and the market was flooded with rice. This market saturation meant very low prices for farmers and considerable crop wastage as well. Private sector Kilombero Plantations Limited claims to have suffered a four billion Tanzanian Shilling (= ~2 million US$) loss as a result of cheap imports of rice (see http://agritrade.cta.int/Agriculture/Commodities/Rice/Tanzanian-rice-sector-stakeholders-call-for-consistent-application-of-rice-import-tariffs and author interviews) in addition to uncalculated losses faced by smallholder farmers who struggled to sell rice on regional markets. Thus, trade policies and other market factors-such as poor roads that limit transport during critical market times-can demotivate farmers from increasing production of particular crops.
In Laos, the government has placed considerable emphasis on increasing rice production to meet food security. Due to a variety of factors, including market prices and preferences for how to allocate labour, farmers in one area of Laos chose to farm high return vegetable crops or rear livestock rather than invest in more rice production (Suhardiman et al., Reference Suhardiman, Giordano, Leebouapao and Keovilignavong2016). This research illustrated the diversity of socio-economic factors affecting farmer decisions, the diversity of farmers and their actions within a single community, as well as the diversity of objectives from government to farm levels.
In neighbouring Vietnam, government efforts to increase maize production through promoting hybrid varieties and chemical fertilizers took an interesting twist in the highlands in the north. There, Hmong farmers only partially adopted hybrid maize, preferring instead to carry on simultaneously with local varieties that were better suited to the hilly terrain areas in their landscape and which produced maize preferred for its taste. They planted hybrid maize in flat areas where it was better suited and they used the harvest to feed to livestock and brew beer. Whilst doing so increased their incomes, the government of Vietnam was promoting hybrids for food security, not livestock feed and beer. In addition, farmers were able to intercrop pumpkin and beans with local maize varieties and thus get diversified food from one plot. Intercropping is not promoted with hybrid varieties. Hybrids were also found more susceptible to post-harvest spoilage and pest attack than local varieties. So, focussing only on hybrid production would increase farmers’ risks (Kyeyune and Turner, Reference Kyeyune and Turner2016). This example points to some of the social complexities around closing the yield gap and addressing food security. Farmers often have very different objectives (taste preferences, income needs) and operate under constraints not well-understood when the focus is on technical solutions.
In many parts of Africa, off-farm income opportunities often are pursued instead of or in addition to farming due to the need for a more reliable and consistent source of cash, particularly during times of need (such as for paying school fees or medical costs). These off-farm labour opportunities, whilst rarely lifting people out of poverty, still can provide a more guaranteed income. Harris and Orr (Reference Harris and Orr2014) have pointed out, for smallholders around the world, increasing agricultural productivity does not necessarily raise them out of poverty. Off-farm income opportunities, as a supplement to agriculture, may provide better returns. Bryceson (Reference Bryceson2002) has observed that very few smallholder farmers in Africa exist on agriculture alone. This de-agrarianization reflects the reality that investing in agriculture is a risky and often not profitable path. Without crop insurance, support from state institutions such as extension services, quality inputs that are available and affordable, and reliable markets with reasonable prices, there is often very little incentive for individual farmers to close the yield gap, a gap they may not recognize.
POST-HARVEST WASTE
In addition, post-harvest factors are also very significant. Gustavsson et al. (Reference Gustavsson, Cederberg, Sonesson, van Otterdijk and Meybeck2011) found that approximately 1/3 of the food produced for food for humans – 1.3 billion tons year−1 – is ‘lost or wasted’. In low-income countries, loss of food from the stage of harvest to processing reached 40%, whilst in middle and high-income countries, 40% of the food was wasted i.e. thrown away, even if it was still edible (Gustavsson et al., Reference Gustavsson, Cederberg, Sonesson, van Otterdijk and Meybeck2011). Also, distribution of harvested food is poor (Stockholm International Water Institute, 2008). Addressing post-harvest waste and distribution, not production and yield gaps, may be the bottleneck to address the predicted food crisis of 2050 (Figure 2).

Figure 2. Per capita food losses for cereal by stage of loss and waste.
FOOD VERSUS ENERGY
The competition between food and energy is an enormous risk that is currently facing the agricultural sector. Between 2000 and 2008, the use of crops for the production of biofuels (maize in the US, sugarcane in Brazil and vegetable oil seeds and cereals in Europe) increased by three times the 2000 value –110 million tonnes, about 10% of global production – driven by policies and incentives that promote growth of this sector (FAO, 2009). The FAO study estimates that under a major increase in biofuel production, by 2050, there would be 3 million and 1.7 million more malnourished children in sub-Saharan Africa and South Asia, respectively (FAO, 2009).
ECOLOGICAL IMPACTS
The potential impact on the environment of closing the yield gap has received remarkably little attention in the literature even though the terminology of ‘sustainable intensification’ aims to increase yield whilst also benefiting the environment and the economy. As Loos et al. (Reference Loos, Abson, Chappell, Hanspach, Mikulcak, Tichit and Fischer2014) note, a framework to analyse and address the long-term impacts of intensification practices is currently lacking. Research on ecosystem services has begun to focus on agricultural productivity, often under the narratives of ‘sustainable intensification’. Tittonell (Reference Tittonell2014) argues, as do others, that the current model for agricultural intensification, of which the yield gap focus can be seen to be a part, is not sustainable socially or ecologically: ‘it is neither ecological nor eco-efficient, it is ineffective at feeding the world, it is harmful for the environment and contributes to biodiversity loss’.
There is a growing number of researchers calling for a reorientation to how we approach agricultural research, moving from a more narrowly focussed search for technological solutions to more holistic ones that consider the wider ecological landscape. Robertson and Swinton (Reference Robertson and Swinton2005) call for managing agricultural landscapes for multiple ecosystem services. This approach would require more integrative research on both the ecology and social-economic context of these systems than is currently common. They argue that indeed raising productivity is an ecological challenge as it relies on how ‘organisms interact among themselves and with their abiotic environments” and how these interactions, then in turn, affect the rate at which nutrients, pesticides and other by-products of agricultural intensification leave the ecosystem and affect the ecology of other areas (2005). Their work is part of a larger long-term cropping experiment in southwestern Michigan that examined the ecological interactions that underpin the performance of different crop management systems, including conventional management, to no-till, reduced input and biological-based approaches (Robertson et al., Reference Robertson, Gross, Hamilton, Landis, Schmidt, Snapp and Swinton2014). They adopted a landscape approach and included long-term observations and experiments. Their findings suggest that ecosystem services can be maintained with intensification if management systems are designed carefully to fit the specific landscapes. To design these management systems, however, the research paradigm needs to shift from an approach that is too narrowly focussed on specific field crops, and to develop a richer understanding of the multiple parts of complex landscapes, including cropped and non-cropped habitats (Robertson et al., Reference Robertson, Gross, Hamilton, Landis, Schmidt, Snapp and Swinton2014).
This work on agricultural landscapes also incorporated research on farmer practices and factors influencing their decisions about land use. Put simply, they found that farmers recognize and value a host of ecosystem services in their agricultural landscapes, but ranked profitability as a key factor in the adoption of any practice. Thus, for many costly (in labour, delayed benefits) changes in management practices, their research found that it is clear ‘that the provision of ecosystem services in agriculture will require incentives’ (Robertson et al., Reference Robertson, Gross, Hamilton, Landis, Schmidt, Snapp and Swinton2014). This conclusion echoes findings in other fields and sectors (forestry, conservation, REDD+) that argue incentives like payments for ecosystem services are necessary and a promising mechanism for changing management practices. Providing incentives requires someone or some institution willing to pay. A survey of approximately 2400 households in Michigan revealed respondents’ willingness to pay farmers to adopt practices to address, in particular, lake eutrophication caused by agricultural run-off.
Tittonell (Reference Tittonell2014) echoes this ecosystem approach to agricultural landscapes in what he sees as the difference between ‘sustainable intensification’ and what he calls ‘ecological intensification’. Ecological intensification includes a variety of paradigms (permaculture, agroecology) that have a very different approach to examining the impact of the wider natural environment on agriculture and vice versa. Tittonell (Reference Tittonell2014) points out that whilst agroecology and organic agriculture have been around for a long time, much of the funding and support for research has been directed at conventional agriculture. This research, founded in the Green Revolution, involves a focus on a few technologies that aim to address ‘single problems at a time. . . .technologies are developed elsewhere and farmers have to “adopt” them’ (Tittonell, Reference Tittonell2014). This paradigm has been central to national and international research institutions. Whilst they are beginning to adopt the narrative of ‘sustainable’ intensification, a radical shift in actual implementation that not only addresses the complexities of the problem at hand, but also moves away from business-as-usual is urgently needed.
Cunningham et al. (Reference Cunningham, Attwood, Bawa, Benton, Broadhurst, Raphael, Didham, McIntyre, Perfectoh, Samways, Tscharntke, Vandermeerh, Villard, Younge and Lindenmayer2013) point to the dangers of closing the yield gap on biodiversity. Historically, advances in technology have led to expansion of land under agriculture as greater profits can be realized. This expansion threatens the biodiversity on which in part successful agriculture depends and thus ultimately may undermine increased food production. They provide one example around crop pollination which can be compromised by the loss of biodiversity. The authors also point out the necessity of understanding differences across landscapes to better grapple with the potential trade-offs around production/conservation.
In order to move away from business-as-usual and address the weaknesses in yield gap research, it needs to integrate elements of agronomy, climate change, crop science, ecology, social science and economics (Figure 3). Additionally, as the works above illustrate, there is considerable value in approaching the yield gap question from a landscape approach so that issues of environmental sustainability and trade-offs can be better understood and addressed.
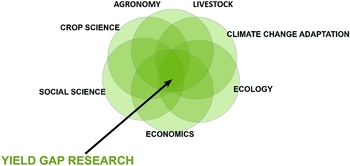
Figure 3. Re-positioning the yield gap (adapted from Meffe et al., Reference Meffe, Ronald, Meffe and Carroll1997).
CONCLUSION
Whilst the narratives around the necessity of feeding nine billion help ‘focus the mind’ (Sumberg, Reference Sumberg2012), they also contribute to a drive towards what is termed the ‘new productivism’ (Tomlinson, Reference Tomlinson2013). The yield gap narrative maintains the focus on a select number of grain and commodity crops, and excludes attention to fruits, vegetables or livestock as important pieces in the food security puzzle. Indeed this narrative diverts attention away from bigger questions about the entire food system, which as mentioned earlier in reference to post-harvest loss, includes the distribution and use of food after it is produced.
The aim of closing the yield gap is to meet the food needs of the earth's population now and in the future. Focussing narrowly on increasing yields does not account for off-site and negative impacts of intensification. What instead is necessary, or becomes, the ‘science question’ as Cunningham et al. point out, is ‘which practices lead to the best outcomes in different landscapes’ (2013:23). To answer this question, integrative research which brings in ecologists, a variety of possible social scientists (rural sociology, anthropology, geography, economics, political science) together with agronomists, soil scientists and crop physiologists must be developed. In this way, a better understanding of a wide variety of social, economic and biophysical trade-offs beyond the farm can be incorporated. This integration is no small task, and indeed willingness to collaborate is often met with institutional and funding barriers against doing so. This research may require more time and more financial resources, but if innovative breakthroughs in how to address yield gaps whilst also ensuring social equity and ecosystem benefits is to be realized, such investments are clearly critical.