Introduction
Kerguelen Islands are predominantly volcanic and the fossil references are thus rare. Paleontological outcrops in Kerguelen consist of continental deposits reported at the end of the nineteenth century and three marine ones described in the early twentieth century (Giret et al., Reference Giret, Leyrit, Lauriat-Rage, Grégoire, Carriol and Lozouet1994, Reference Giret, Weis, Grégoire, Mattielli, Moine, Michon, Scoates, Sylvie Tourpin, Delpech, Gerbe, Doucet, Ethien and Cottin2003). The continental deposits correspond to lignite and to a few silicified levels that yielded many plant remains, Miocene to Oligocene in age, including large pieces of trunks, displaying very exceptional states of preservation (see fossils from the Ronarc’h Peninsula; Seward and Conway, Reference Seward and Conway1934; Duchêne, Reference Duchêne1989; Philippe and Giret, Reference Philippe and Giret1998; Giret et al., Reference Giret, Weis, Grégoire, Mattielli, Moine, Michon, Scoates, Sylvie Tourpin, Delpech, Gerbe, Doucet, Ethien and Cottin2003). Besides these paleobotanical remains, only three localities are known to yield marine fossils. Located on both sides of the Morbihan Gulf (Fig. 1), these localities correspond to marine sediments between lava layers that originated with outbreaks of basalts and phonolites (Nougier, Reference Nougier1970). The first reported fossil locality is known as the “Cat Ears” (see Tate, Reference Tate1900; Leyrit, Reference Leyrit1992) and was described by Fletcher (Reference Fletcher1938) for its fossil content (bryozoans, bivalve and gastropod mollusks, barnacles, and imprints of spirorbid annelids). The second locality is the Mont Rond deposit, discovered in 1989, which yielded a rich fauna of endobiontic mollusks (bivalves and gastropods) and barnacles that are early to middle Miocene in age. The Mont Rond assemblage has been identified as a coastline community of temperate waters living on sandy to muddy substrates (Carriol et al., Reference Carriol, Leyrit and Giret1992; Giret et al., Reference Giret, Leyrit, Lauriat-Rage, Grégoire, Carriol and Lozouet1994; Lauriat-Rage et al., Reference Lauriat-Rage, Carriol, Lozouet, Giret and Leyrit2002). The third fossiliferous site corresponds to the Cap Milon fossil strata, located north of the Morbihan Gulf, on the Prince of Wales Foreland. This outcrop is the least accessible of the Kerguelen deposits because of its location on the cliffs. It was reported by Nougier (Reference Nougier1970) as containing fossiliferous sandstone nodules, which were never described. Some of these nodules were collected in 1974 and 1987 (by Richer de Forges and Lemaire, respectively) and deposited at the Muséum national d’Histoire naturelle, Paris. They contain mollusks and rare possible barnacles, but above all a majority of fossil brachyuran crabs (about 60%), the sole known Decapoda from Kerguelen. This is of special interest knowing that only one extant crustacean decapod is reported in Kerguelen: Halicarcinus planatus (Fabricius, Reference Fabricius1775), which is a brachyuran species displaying an uncommonly soft and uncalcified carapace (Duchêne, Reference Duchêne1989). The Cap Milon material corresponds to a unique species displaying exceptional preservation with cuticles in situ, fragile endoskeleton parts (internal pleurites), and even gills preserved in 3D. Here, we study these fossil crabs through two complementary aspects: systematics and preservation with petrographic and geochemical analyses.
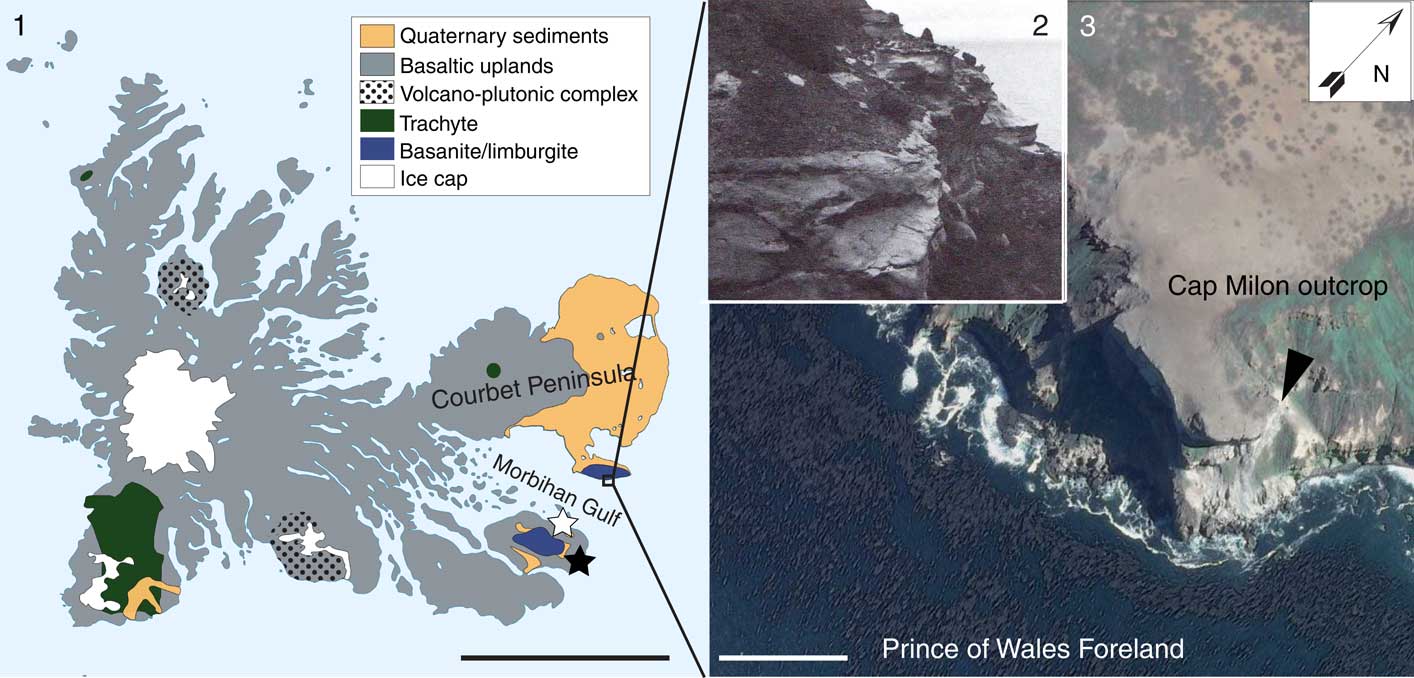
Figure 1 Fossil site location. (1) Simplified geological map of the Kerguelen Islands (modified after Nougier, Reference Nougier1970 and Lorand et al., Reference Lorand, Delpech, Grégoire, Moine, O’Reilly and Cottin2004); (2) Cap Milon tufas yielding the crab-bearing nodules (110 m above sea level; from Nougier, Reference Nougier1970); (3) satellite south-eastern view of Prince of Wales Foreland showing the Cap Milon and the Miocene outcrops (black arrow). Stars represent the two other fossiliferous deposits in Kerguelen (black=Mont Rond site; white=Cat Ears locality). Scale bars=50 km (1), 500 m (3).
Geological setting
The Miocene layers of Cap Milon crop out on the southern slope of the Prince of Wales Foreland, at the southeastern extremity of the Courbet Peninsula (Kerguelen Islands). This fossil locality is of limited extent (some tens of m2) and of very difficult access, requiring coming through the western side of the foreland (Pointe Suzanne), which is much less steep than the sharp southern cliffs (Lauriat-Rage et al., Reference Lauriat-Rage, Carriol, Lozouet, Giret and Leyrit2002). The sedimentary layers are exposed below some substantial outpourings of basanite and limburgite, which cover the whole south of the foreland (Fig. 1.1). The outcrop, ~150 m above sea level, consists of basaltic conglomerates ~50 m thick, the aspect of which indicates a marine beach deposit (e.g., slope of the waves breaking). Within these conglomerates, an 8 m thick subhorizontal deposit of very fine-grained tufa that includes spherical fossiliferous sandstone nodules (Fig. 1.2, 1.3). These nodules contain remains of a monospecific assemblage of crabs, but also bivalve and gastropod mollusks, as well as bones and teeth of vertebrates (actinopterygians, chondrichtyans, and sirenians) (Duchêne, Reference Duchêne1989; Lemaire, personal observation). These fossils characterize a proximal shore facies that was further exhumed, along with the whole foreland, due to the activity of a fault located north of the Passe Royale (entrance of the Morbihan Gulf; Nougier, Reference Nougier1970; Duchêne, Reference Duchêne1989). The age of the layers could not be dated more precisely than Miocene.
Materials and methods
Studied specimens
We examined 122 specimens of fossil crabs preserved in nodules, and deposited at the MNHN (MNHN.F.A59107–A53219, A59618–A59626). All of them were successively collected in 1974 and 1987 by B. Richer de Forges and L. Lemaire, respectively, at the Cap Milon site, during winters in Kerguelen. Among these fossils, 35 display a measurable carapace, one of which (MNHN.F.A59117) displays fouling bryozoans. Three crabs display preserved gills; two are open endoskeletons (MNHN.F.A59110, MNHN.F.A59115) and one is an external mold of a partially broken carapace (MNHN.F.A59116). The geochemistry of the fossils and their surrounding matrix was characterized on specimen MNHN.F.A59114 in order to document the taphonomic conditions. Modern gills were sampled for comparative observations on a con-familial specimen of Cancer pagurus Linnaeus, Reference Linnaeus1758 from Finistère (France), which were equivalent in size to the studied fossil crabs (MNHN-IU-2014-12647).
SEM, EDS, and DRX analyses
For fossil material, the gills and the fouling bryozoans were observed in situ. The modern gills were sampled after immersion of the crab in successive ethanol baths for 20 minutes each to reach 100% ethanol equilibrium (dilutions at 80%, 90%, and 100%). The gills were dried at the critical point (EmiTechK850, carbon dioxide solvent) to allow their 3D preservation for SEM and then mounted on stubs and sputter-coated in carbon. Observations were made using a Tescan SEM (VEGA II LSU) linked to an X-ray detector SD3 (Bruker) (Direction des Collections, MNHN, Paris). The chemical composition of the fossil crab bodies and the non-fossiliferous matrix, as well as that of the modern gills, were semi-quantitatively determined by energy dispersive X-ray spectrometry (X-EDS). These analyses were performed on C-coated (1) fragments of the crabs, (2) polished thin sections of the nodule matrix, and (3) sampled modern gills. The mineralogy of the matrix was investigated by X-ray diffractometry (XRD) on tiny fragments of the nodule MNHN.F.A59114 (respectively, brown friable outermost layer and gray innermost rock). These fragments were crushed in an agate mortar; the resulting powder was dispersed in alcohol and the solution dried on a zero diffraction plate before measurement. XRD analyses were performed using a Bruker D2 PHASER operating at the MNHN (Paris, France) with a Lynxeye detector (opening: 5.821°2Theta) and a Ni-filtered Cu Kα radiation (30 kV, 10 mA) with a 0.02°2Theta step width.
Repositories and institutional abbreviations
Types, figured, and the other 109 specimens examined in this study are deposited in the following institutions: Collection de Paléontologie (MNHN.F) and Collection de Zoologie (MNHN.IU) of the Muséum national d’Histoire naturelle, Paris, France.
Systematic paleontology
Anatomical abbreviations
CL, carapace length (inclusive of rostrum); CW, carapace width (including lateral spines); FM, frontal margin width; OFM, orbitofrontal margin width; PM, posterior margin width.
Decapoda Latreille, Reference Latreille1802
Pleocyemata Burkenroad, Reference Burkenroad1963
Brachyura Latreille, Reference Latreille1802
Eubrachyura Saint Laurent, Reference Saint Laurent1980
Heterotremata Guinot, Reference Guinot1977
Cancroidea Latreille, Reference Latreille1802
Cancridae Latreille, Reference Latreille1802
Genus Romaleon Gistel, Reference Gistel1848
Type species
Corystes (Trichocera) gibbosula De Haan, Reference De Haan1833 by monotypy.
Other species
Romaleon antennarium (Stimpson, Reference Stimpson1856), as Cancer, Pliocene–Pleistocene, extant, west coast North America; R. branneri (Rathbun, Reference Rathbun1926) as Cancer, Pliocene–Pleistocene, extant, west coast North America; R. dereki Nations, Reference Nations1975, as Cancer (Romaleon), middle Miocene, California, USA; R. gibbosulum (De Haan, Reference De Haan1833,), as Corystes (Trichocera), extant, Japan; R. granti (Rathbun, Reference Rathbun1932), as Cancer, Pliocene–Pleistocene, west coast North America; R. jordani (Rathbun, Reference Rathbun1900), as Cancer, Pliocene–Pleistocene, extant, west coast North America; R. nadaensis (Sakai, Reference Sakai1969), as Cancer, extant, Taïwan to Japan; R. luzonensis (Sakai, Reference Sakai1983), as Cancer, extant, Philippines; R. nakagawaensis Kato and Hikida, Reference Kato and Hikida2002, as Romaleon, middle Miocene, Japan; R. parspinosus Casadío et al., Reference Casadío, Feldmann, Parras and Schweitzer2005, as Romaleon, lower upper Miocene, Eastern Argentina; R. setosum (Molina, Reference Molina1782), as Cancer, Pliocene–Pleistocene, west coast North America, extant, west coast South America; R. sakamotoi (Kato, Reference Kato1996), as Cancer, middle Miocene, Japan; R. sanbonsugii (Imaizumi, Reference Imaizumi1962), as Cancer, middle Miocene, Japan; R. urbanus (Rathbun, Reference Rathbun1917), as Cancer, Pliocene, California; R. yanceyi (Nations, Reference Nations1975), as Cancer, Pliocene–Pleistocene, west coast North America.
Diagnosis
Carapace moderately areolate, preserved cuticle with small granules located primarily but not exclusively on swollen regions. Front moderately advanced, projected beyond orbits, with five frontal teeth, median three clustered, pointed median tooth. Anterolateral margin with nine singular teeth, sharply triangular, curving forward, lined with granules; first tooth (outer orbital corner) pointed. Posterolateral margins weakly concave, with single tooth anteriorly. Chelipeds with sharp spines or granules on the outer and upper surfaces of the carpus and propodus.
Remarks
Emended diagnosis modified from Nations, Reference Nations1975 and from Schweitzer and Feldmann, Reference Schweitzer and Feldmann2000.
Romaleon franciscae Van Bakel, Robin, and Noël new species

Figure 2 Type material of Romaleon franciscae n. sp. from the Miocene of Cap Milon, Kerguelen Islands. (1) holotype MNHN.F.A59107, dorsal view; (2) paratype MNHN.F.A59108, dorsal view; (3) paratype MNHN.F.A59109, dorsal view; (4, 5) paratype MNHN.F.A59108, frontal (4) and left lateral (5) views; (6) paratype MNHN.F.A59110, ventral inner view revealing sections of the preserved gills; (7) paratype MNHN.F.A59111, dorsal view; (8) paratype MNHN.F.A59112, inner view of left chela; (9) paratype MNHN.F.A59113, carpus and right propodus; (10) paratype MNHN.F.A59114, left carpus and propodus. Scale bars=5 mm (1–7), 10 mm (8–10). Ammonium chloride coating (1–6, 8–10). Photographs, B. van Bakel, L. Cazes.
Types
Holotype: MNHN.F.A59107 (complete carapace; L=16 mm, W=38 mm), paratypes: MNHN.F.A59108 (complete carapace; CL=14 mm, CW=21 mm), MNHN.F.A59109 (nearly complete carapace, front teeth and orbits; CL=11 mm, CW= 14 mm), MNHN.F.A59110 (preserved endoskeleton; CL=10 mm, CW=13.5 mm), MNHN.F.A59111 (complete carapace; CL=11 mm, CW=15 mm), MNHN.F.A59112 (cheliped: dactylus+propodus and imprint), MNHN.F.A59113 (cheliped: carpus+right propodus with tubercles on top), MNHN.F.A59114 (smallest cheliped: left carpus+propodus).
Type locality
Miocene of the Kerguelen Islands, Prince of Wales foreland, Cap Milon, (49°26'57''S, 70°23'04''E).
Diagnosis
Carapace wider than long (CL/CW ratio 0.66–0.85), gently convex in longitudinal and transverse cross-sections; front tridentate, median tooth smaller; orbit with two distinct notches, anterolateral margin with nine triangular teeth with teeth 4–9 grouped in clusters of two; a tenth reduced tooth on the posterolateral margin; chelipeds massive, tubercular on upper margin of propodus, palm with four equidistant blunt crests.
Description
Crab of moderate size, carapace width 13–48 mm (n=35 measurable carapaces). Holotype ratios: OFM 47% of the CW; CL 73% of the CW; FM (inner orbital corners) 22% of the CW; PM 38% of the CW. Carapace oval, wider than long, ratio width to length 1.2–1.5. Carapace gently convex in longitudinal and transverse cross-sections, weakly defined grooves delineating gastric, cardiac, and branchial regions; regions moderately marked, more inflated at protogastric, epibranchial, and cardiac regions; hepatic, intestinal, and branchial areas less inflated. Orbitofrontal margin conspicuously wide for the genus and family, occupying 47% of maximum carapace width. Front (excluding inner orbital corners) narrow, horizontal, weakly produced, tridentate, the base of the interdental space practically arriving at the orbital line of the two inner orbital corners; axial tooth considerably smaller, slightly more projected, and more depressed than lateral teeth; all teeth with slightly rounded tips. Orbits very large for the genus, rounded, with two well-defined supraorbital notches; inner notch parallel to carapace axis; outer notch directed towards median line. Inner orbital corners pronounced, raised; outer orbital corners more subtle and depressed. Anterolateral margin convex, slightly raised, with nine teeth (includes outer orbital tooth); a tenth weakly developed and low tooth on the posterolateral margin; all teeth sharp, triangular, lined with small granules; teeth 4–5, 6–7, and 8–9 clustered, more depressed than preceding teeth; these groups of two teeth limited by a deeper notch; teeth 4–5 slightly forwardly directed, teeth 6–7 and 8–9 perpendicular to lateral carapace margin. Posterolateral margin gently concave, lined by a distinct, finely granular rim, fading into rounded posterior corner. Posterior margin gently rounded, weakly concave medially, narrower than orbitofrontal margin. Protogastric regions large, U-shaped; mesogastric region sharply triangular, with rounded posterior margin, containing well-visible widely separated gastric pits; cardiac region large, well defined, hexagonal. Chelipeds massive; merus triangular in cross-section, preserved cuticle with granular lower margin and a distinct tubercle at the distal joint. Outer surface of carpus smooth or slightly granular, without keel; a line of granules highlights the angular margins. Propodus with flattened and hollowed inner surface; preserved cuticle of external face of palm characterized by four blunt equidistant granular crests; upper margin tubercular with a tooth near the articulation with the carpus. Dactylus flattened, high, with external granular rim, and inner margin with a dozen small and sharp teeth. Fixed finger with small, sharp teeth. Walking legs rather short, laterally compressed, with narrow, straight, styliform dactyls.
Dorsal carapace with smooth surface microstructure in small individuals, preserved cuticle finely granular or areolate in larger specimens in particular in the central regions.
Etymology
The specific epithet honors the late Mrs. Françoise Noël, physician specializing in endocrinology.
Materials
114 nodules (MNHN.F.A59115–A59219, A59618–A59626) containing more or less complete carapaces, some with partially preserved endoskeletons and chelipeds.
Remarks
The new species fits well in the diagnosis of Romaleon because of its relative carapace ratios, a weakly concave posterolateral margin, anterolateral margin with nine sharply triangular teeth that are separated to their base and curved forwards, and the front, which is projected beyond the orbital level with the medial tooth at a lower level. Romaleon is a genus with Miocene species from Japan, California, and Patagonia, Argentina (Imaizumi, Reference Imaizumi1962; Kato, Reference Kato1996; Kato and Hikida, Reference Kato and Hikida2002; Casadío et al., Reference Casadío, Feldmann, Parras and Schweitzer2005); Pliocene–Pleistocene species from the west coast of North America (Molina, Reference Molina1782; Stimpson, Reference Stimpson1856; Rathbun, Reference Rathbun1900, Reference Rathbun1926), and extant species from Japan and the west coasts of North and South America (Molina, Reference Molina1782; De Haan, Reference De Haan1833; Rathbun, Reference Rathbun1900; Sakai, Reference Sakai1969, Reference Sakai1983). In Romaleon, the clustered anterolateral teeth 4–5, 6–7, and 8–9 of R. franciscae n. sp. is only seen in the lower upper Miocene species from Patagonia, R. parspinosus. Romaleon parspinosus is morphologically closest to R. franciscae n. sp., however the orbits are clearly smaller, the orbitofrontal width (47%, vs 37% in R. parspinosus) and posterior margin width (38% vs 20% in R. parspinosus) are much smaller, and the notches clustering the anterolateral teeth arecmore distinct in R. parspinosus.
Casadío et al. (Reference Casadío, Feldmann, Parras and Schweitzer2005, p. 162) noted that Anisospinos Schweitzer and Feldmann, Reference Schweitzer and Feldmann2000 (late Eocene, Washington, USA; Miocene, Washington, USA and Japan) also exhibits paired anterolateral teeth. However, Anisospinos has eight anterolateral teeth instead of nine, has no posterolateral spine instead of one, and has five frontal teeth that are evenly spaced, instead of the three median teeth clustered. Anisospinos is one of the oldest cancrid crabs and may be ancestral to Romaleon (see also Schweitzer and Feldmann, Reference Schweitzer and Feldmann2000, fig. 1).
Within extant Romaleon, R. franciscae n. sp. resembles the extant Japanese type species R. gibbosulum, but is distinguished by having its middle frontal tooth shorter than in the type species, by the two distinct notches of the orbital margin, the teeth of the anterolateral margin grouped in clusters of two and less anteriorly directed, and cheliped significantly less spinose. Romaleon luzonensis has a more areolated carapace, and distinct teeth on the posterolateral margin. Romaleon franciscae n. sp. is also morphologically close to R. branneri (Pliocene–Pleistocene, North America), whose frontal teeth are very similar; however, the anterolateral teeth of R. branneri end in a spine, which is not the case in R. franciscae n. sp. Another related species, R. jordani (Pliocene–Pleistocene to extant, North American), has a narrower PM of the carapace (20% vs 38% in R. franciscae n. sp.) and a cheliped with the propodus upper margin less tubercular. Romaleon franciscae n. sp. also resembles R. dereki (Miocene, California), but is distinguished by the presence of a tooth at the posterolateral corner, and by the teeth of the anterolateral margin less spiniform and assembled in pairs. Other species of Romaleon seem more distinctly different: R. antennarium (Pliocene to extant, North American Pacific) exhibits more spiniform anterolateral teeth, which are more anteriorly directed; R. nadaensis, an extant Japanese species, has the anterolateral teeth regularly spaced and equal in size; R. urbanus (Pliocene, California) shows deep grooves and depressions on the carapace, especially in the metagastric region; R. granti (Pliocene, California) lacks a tooth at the posterolateral margin and rows of large tubercles on the cheliped. Romaleon yanceyi (Pliocene or Pleistocene, California) is known only by two propodi of chelipeds that lack the spiny ornamentation of R. franciscae n. sp. Romaleon nakagawaensis, from the Miocene of Japan, has smaller, more forwardly directed anterolateral teeth, and a weaker groove system on the dorsal carapace; R. sakamotoi has deeper, more acute carapace grooves, and more slender, pointed anterolateral teeth; and R. sanbonsugii has two small distinct posterolateral teeth or tubercles which distinguish it from R. franciscae n. sp.
Results
Bryozoan post-mortem fouling
Two distinct bryozoan colonies are found on the internal mold of one of the largest crabs (Fig. 3.1, CW=42 mm). They correspond to hundreds of zooecia of cheilostomes preserved as basal imprints (Fig. 3.2). Diagnostic features are not preserved, so the colonies are not further identifiable. Some of the zooecia shapes may in some aspect evoke Calloporidae Norman, Reference Norman1903 (K. Zagorsek, personal communication, 2017; Fig. 3.3, 3.4), but this cannot be confirmed. The location of the colonies indicates a post-mortem fouling of the carapace, which had to be dislocated to allow their internal colonization by bryozoans (Jakobsen and Feldmann, Reference Jakobsen and Feldmann2004). The large size of the colonies implies a quite long exposure of the broken carapace lying on the seabed before its burial, and thus a certain resistance of the carapace dorsal side (Amui-Vedel et al., Reference Amui-Vedel, Hayward and Porter2007; Robin et al., Reference Robin, Van Bakel, d’Hondt and Charbonnier2015).

Figure 3 Encrusting cheilostome bryozoans (MNHN.F.A59882) on MNHN.F.A59117. (1) Internal mold of the carapace; (2) SEM view of the colonies; (3) SEM close-up on the basal imprints of the zooecia; (4) SEM view of the zooecia imprints evoking Calloporidae. Scale bars=1 cm (1), 4 mm (2), 1 mm (3), 100 µm (4). Photographs, N. Robin, S. Pont.
Preserved gills
Different views of the preserved gills exist in the studied material (Fig. 4.1–4.6). The outer view of the broken carapace reveals the dorsal side of the gill lamellae (Fig. 4.2, 4.5). If it is possible to spot the limits of these different gills in dorsal view (Fig. 4.5), it is impossible to identify them. On the other hand, on the endoskeletons, the nature of the different gills structures can be suggested. Extant Cancer (Cancridae) displays nine gills at each side, plus three mastigobranchiae (Pearson, Reference Pearson1908; Calman, Reference Calman1909). These nine elements are located on thoracic somite 2 (g1, g2), somite 3 (g3, g4, g5), somite 4 (g6, g7), somite 5 (g8), and somite 6 (g9). On its left side, specimen MNHN.F.A59110 exhibits nine series of branchial lamellae in transversal view. These series may be identified as the paired lamellae (anterior and posterior), which compose g2–g7, with g8 and g9 missing and the anterior lamellae of g2 likely not visible. The g1 (a podobranch) is clearly recognizable. It lies with its outer face in contact with the posterior side of the branchial groove, and its inner face closely applied to the basal portions of gills 2–6. Its apex points backward and outward (Pearson, Reference Pearson1908). The gill segment, which lies outer of g5, could correspond to the g3, which is known on extant Cancer to be short and wedged in between g1 and g5 (Fig. 4.4.). On its right side, specimen MNHN.F.A59115 displays four lamellae series that could correspond to g4 and g5 (Fig. 4.5). The afferent (dorsal) and efferent (ventral) vessels, which enable the dioxygen exchanges in extant Cancer pagurus (Fig. 4.7–4.9), are not even partially preserved on the fossil material. The similarity between the fossil and extant lamellae shapes is difficult to appreciate due to the stiffening of fossil structures through diagenesis. However, we note that the fossil lamellae exhibit a general cup-shape very similar to that observed on the extant representatives (Fig. 4.8).

Figure 4 SEM views of the gills of Romaleon franciscae sp. nov. (Miocene, Kerguelen Islands) (1–6). (1–3) Endoskeletons (1, 3) and broken dorsal side of a carapace (2) displaying gills (MNHN.F.A59110, A59116, and A59115, respectively); (4) close-up of the branchial lamellae of MNHN.F.A59110 in transversal view; (5) close-up of the gills of MNHN.F.A59116 appearing in dorsal view (white arrow=gill width); (6) close-up of the branchial lamellae of MNHN.F.A59115 in transversal view. SEM views of the gills of Cancer pagurus Linnaeus, Reference Linnaeus1758 (extant, Finistère, France) (7–9). Modern gills of MNHN-IU-2014-12647: dorsal view of g7 showing the afferent vessel (7); latero-transversal view of the gill lamellae shape (8); ventral view of g9 showing the efferent vessel (9). Abbreviations: af=afferent vessel, ef=efferent vessel, g1–g7=gills 1 to 7. Scale bars=2 mm (1–3), 500 µm (4–6), 250 µm (7–9). Photographs, N. Robin, S. Pont.
Matrix and gills mineralization
The combination of XRD analyses on crushed matrix (Fig. 5) and local X-EDS analyses on grains and crab fragments allowed analysis of the mineral composition of the nodule. The nodule matrix displays for its most internal part a sandstone microfacies with volcanic remains (e.g., neat basaltic lithoclasts) and minerals stemming from the alteration of theses basalts, such as granulated feldspars (anorthoclase and anorthite), clinopyroxenes (likely augite and diopside), quartz, angular and opaque iron oxides identified as titanomagnetite and ilmenite, as well as a few micas (likely phlogopite) (Fig. 5.2, 5.3). Carbonate-fluoroapatite (CARFAP) constitutes an important cryptocrystalline phase (Fig. 5.1, 5.3–5.6) corresponding to: (1) the continuous layer that borders the edges of most of the clasts (Fig. 5.3–5.6), (2) dark angular to round phosphoclasts (Fig. 5.3), and (3) micritic bioturbation remains (fecal pellets and/or burrowing traces; Fig. 5.4). This 10 µm phosphate edging indicates diagenetic submarine phosphatization. More generally, this cryptocrystalline clay-phosphate-ferrous cement seems to encompass most of the grains. The crabs display a global CARFAP composition of the exoskeleton, endoskeleton, and of the gills. This CARFAP is partially mixed with clayey elements (Fig. 5.7). But a striking element on these structures is their whole covering by a more clay-rich fleecy film (enriched in Si, Al, and Mg; cf. montmorillonite?). This fleecy film shows tubular surface structures that may reflect the influence of bacteria in the development of the clayey covering (Fig. 5.7). The modern sampled gills display an organic X-EDS spectrum (O, Ca, Al, N, Si, S, P, Mg) that is rather homogeneous between the different gill components. However, we note that afferent and efferent structures are slightly poorer in phosphorous than the gill lamellae.

Figure 5 Petrography and chemical composition of nodule MNHN.F.A59114 (matrix and crab). (1–4) Microscopic view of the matrix cross-section showing minerals of detrital origin: (1) cross-polarized light; opaque minerals and part of a micritic phosphate area; (2) plane light; part of the phosphate bioturbation structures (pellets?); (3) plane light; a continuous 10 µm phosphate at the edge of the clasts; (4) plane light; (5) SEM close-up of the phosphate edging of clasts (C-coated cross-section of the matrix); (6) SEM top view of some of the crab soft tissues (gills) and of an endoskeleton thin element seen in cross-section (C-coated crab fragment); most of the crab structures display a thin clay-phosphate-ferrous cover. (7) XRD patterns of the indurated gray fraction of the nodule showing the feldspars and phosphate main signal. Abbreviations: Ba=basaltic lithoclast; CARFAP=Carbonate-fluoroapatite; Cl=clast; Cpx=clinopyroxene; F=feldspars; Iox=Iron oxide; Pe=pellets (or bioturbation); P=phosphoclast; Si-Al=clay elements; Si-Al-P-Fe=clay phosphate ferrous film. Arrows=CARFAP edgings (e); neat set of tubular surface structures that may correspond to bacterial structures (6). Scale bars=20 µm (1–4), 200 µm (5), 50 µm (6). Photographs, N. Robin, S. Pont; DRX spectrum, M.-M. Blanc-Valleron.
Discussion
Previous papers discussed the migration routes along which decapod crustaceans proceeded during the Cretaceous–Tertiary periods in the Atlantic-Pacific regions (Schweitzer, Reference Schweitzer2001; Rumsey et al., Reference Rumsey, Klompmaker and Portell2016), some of which described general patterns suggested in the Southern Hemisphere (Feldmann and Schweiter, Reference Feldmann and Schweitzer2006). The geographic position of Romaleon franciscae n. sp. during the Eocene is unexpected; indeed, it is very remote from any known occurrence of fossil or extant cancrid crabs. Also, it is not along any suspected migratory route of cancrid crabs. In fact, the opposite is true—a migratory route along the coast of Antarctica from Chile to New Zealand was proposed by Nations (Reference Nations1975; Fig. 6). This is not the most likely route, in regard of the Antarctic Circumpolar Current, which runs from South America eastward to New Zealand. It is most probably by this current that Romaleon reached the area of Kerguelen, taking into account the Mio-Pliocene occurrence of Chile and Patagonian (Fig. 6). No Cancrida is known from Antarctica (Feldmann and Wilson, Reference Feldmann and Wilson1988). The extant (and fossil) species that is the nearest geographically is Metacarcinus novaezealandiae (Hombron and Jacquinot, Reference Hombron and Jacquinot1846) from Tasmania and New Zealand, but it is very different morphologically. At the west coast of South America there is M. edwardsii (Bell, Reference Bell1835). The sole cancrid crab known today from New Zealand and Tasmania, M. novazealandiae, is also known from the middle or late Miocene of South Island, New Zealand (Feldmann et al., Reference Feldmann, Schweitzer and McLauchlan2006). It is unclear if Metacarcinus traveled a similar route as Romaleon, from Chile along the Antarctic coast westward to New Zealand. An alternative migratory route for Metacarcinus may be from Japan southwards. In our opinion, such a route seems extremely unlikely for Romaleon to have followed. An alternative route from South America westward is far more assumable and would better suit the geographical dissemination of the genus through the Cenozoic. In any case, it has to be noticed that this genus is no longer present nowadays in Kerguelen, where a single species of decapod crustacean from a remote brachyuran group is known to live (Halicarcinus planatus, Majoidea). This species displays an uncommonly soft and uncalcified carapace whose protective role remains unobvious (Richer de Forges, Reference Richer1977; Duchêne, Reference Duchêne1989). This pattern could be related to the calcareous poverty of the Kerguelen waters, even if this parameter could not entirely explain this carapace feature (Duchêne, Reference Duchêne1989). In comparison, knowing the fragility of crab carcasses and regarding (1) the preservation state (in connection) of the studied specimens and (2) the long exposure that some specimens underwent to display the bryozoan foulings, it seems very unlikely that R. franciscae n. sp. exhibited such a softness of the dorsal carapace. These preservational aspects imply that R. franciscae n. sp. displayed a likely higher carapace calcification than Halicarcinus planatus. Multiple factors could explain this variation in calcification of the crab carapaces, among which a substantial difference in Kerguelen water composition between nowadays and the Miocene.

Figure 6 Hypothesized migration route of Romaleon based on previously described occurrences from Late Cretaceous to Holocene.
The composition of the nodule matrix indicates that the crabs likely evolved in an environment with a dark sandy beach, stemming from of the alteration of the Kerguelen prevalent volcanic basements (basalts). The early mineralization of soft tissues is a bacterially induced phenomenon that depends on local chemical conditions of the medium enclosing the body remains (Briggs and Kear, Reference Briggs and Kear1993). These conditions change according to the initial chemistry of the medium, the decay progression, the system permeability, and the release of microbial metabolism products. The precipitation of CaCO3 or Ca3(PO4)2 in marine environments has been compared to a ‘switch’ with the default set for the precipitation of calcium carbonate. Indeed, in a permeable aqueous system, the precipitation of calcium phosphate is inhibited by high ambient concentration of microbially generated hydrogencarbonate ions (HCO3 -). This explains why calcium carbonate normally precipitates preferentially during diagenesis (Allison, Reference Allison1988; Briggs and Kear, Reference Briggs and Kear1993, Reference Briggs and Kear1994; Briggs and Wilby, Reference Briggs and Wilby1996). When the system closes, the accumulation of the acidic bioproducts, stemming from microbial metabolism (CO2, H2S), triggers a pH decrease, which allows calcium phosphate precipitation. Thus, the phosphatization of the crab skeletons and soft tissues, associated with that of the matrix clasts, must have occurred early in diagenesis, likely on still unbound grains from an impervious sedimentary system that enclosed the crabs alive or soon after death. The formation of the clay-phosphate-ferrous film and its role in the preservation remain unclear, but it seems to have occurred secondarily in the diagenesis. The likely microbially induced formation of this covering could result of secondary changes in the permeability of the nodule system and/or of the activity of bacteria inducing silica precipitation (Birnbaum et al., Reference Birnbaum, Wireman and Borowski1989); but these issues would need to be further documented. The origin of dissolved phosphate in the system may both be intrinsic and extrinsic to crab composition. Indeed, Briggs and Kear (Reference Briggs and Kear1993) showed in their experiments that phosphatization of decapod soft tissues occurred even where the sole source of phosphate is the crustacean itself. Since that, Bosselmann et al. (Reference Bosselmann, Romano, Fabritius, Raabe and Epple2007) quantitatively determined the proportion of phosphates in the exoskeleton of some decapod models, including Cancer pagurus, for the first time. They showed that PO4 3– represents up to 3.5% of the exoskeleton of the species adult form, with a higher relative concentration in the carapace than in chelipeds (equivalent or slightly higher value than species studied by Briggs and Kear). Moreover, other structures have been documented as phosphates, such as mandibles, whose phosphatization is more widely applied among crustaceans than previously thought (see Bentov et al., Reference Bentov, Aflalo, Tynyakov, Glazer and Sagi2016, but brachyurans are less involved than other decapods). A source could also be found in the phosphate granules produced in the digestive gland of many crabs (including C. pagurus), which plays a role in metal detoxication processes (Guary and Négrel, Reference Guary and Négrel1981; Luquet, Reference Luquet2012). In our case, the modern afferent/efferent structures, which display a slightly lower phosphorous composition than the lamellae, are not preserved as fossils. Aware of the multiple gaps in our understanding of local phosphatization in a fossil system, we wonder if this variation in composition could have affected the preservation.
Phosphatization is known for offering a better morphological fidelity than calcification in vertebrate soft tissues (Martill, Reference Martill1988, Reference Martill1990; Schultze, Reference Schultze1989; Wilby, Reference Wilby1993), which also holds true for fossil crustaceans. Indeed, with the exception of very specific chemistries and environments, fossil decapod soft tissues are almost always found in phosphate concretions or as phosphate preservation in shales. These tissues are mostly muscles (Wilby and Martill, Reference Wilby and Martill1992; Feldmann and Schweitzer, Reference Feldmann and Schweitzer2010; Haug et al., Reference Haug, Nyborg and Vega2013; Gueriau et al., Reference Gueriau, Mocuta, Dutheil, Cohen, Thiaudière, Charbonnier, Clément and Bertrand2014; Jauvion et al., Reference Jauvion, Audo, Charbonnier and Vannier2016), but also include thin sensory organs such as orbital bulla reticularis (Bishop, Reference Bishop1984) or eyes (Tanaka et al., Reference Tanaka, Smith, Siveter and Parker2009; Audo et al., Reference Audo, Schweigert, Saint Martin and Charbonnier2014; Vega et al., Reference Vega, Jackson and Ossó2014; Luque, Reference Luque2015). Gill preservation among fossil Malacostraca and other arthropod relatives corresponds to very limited occurrences reported for ostracodes (Siveter et al., Reference Siveter, Sutton, Briggs and Siveter2003, Reference Siveter, Briggs, Siveter and Sutton2010), cycloid crustaceans (Dzik, Reference Dzik2008), thylacocephalans (Vannier et al., Reference Vannier, Schoenemann, Gillot, Charbonnier and Clarkson2016; Charbonnier et al., Reference Charbonnier, Teruzzi, Audo, Lasseron, Haug and Haug2017), and astacidean and brachyuran crustaceans (Cushman, Reference Cushman1905; Moore, Reference Moore1969; Franţescu et al., Reference Franţescu, Feldmann and Schweitzer2016). Most of the time, they consist of 2D preservation of the lamellae. The present fossilization, which allows recognition of different gill anatomies, is thus rather exceptional. Providing highly resolved imaging of such preserved soft structures is especially worthy because it may of course document the evolution of their anatomy, but also the ecophysiological strategies in a taxon, which are most of the time exclusively inferred from studies on modern representatives.
Conclusions
The Miocene fossil crabs of Cap Milon correspond to a new species of Romaleon, which suggests by its geographical location a new possible migration route for this genus during the Cenozoic. This exceptionally preserved material reveals almost all of the animal gills three-dimensionally preserved (g8 and g9 missing). This uncommon preservation is linked to very early phosphatization of the systems during diagenesis, as the nodule matrix directly shows. This preservation also seems related to deposition of a thin clayey cover, possibly of bacterial origin, on most of the soft tissues before or after their phosphatization. It remains very unclear if the phosphorous intrinsic to the crab tissues, and especially to the gills, determined the phosphatization or loss of these fragile tissues. Finally, numerous nodules from Cap Milon yielded additional fauna (mollusks and remains of actinopterygians, chondrichtyans, and sirenians). This material would deserve the same approach for instance to document some questionable routes taken by taxa between the different continents of the Southern Hemisphere and affinities to extant representatives.
Acknowledgments
We want to thank A.A. Klompmaker and an anonymous reviewer for their valuable advice during the improvement of the manuscript. We warmly thank K. Zagorsek (Technical University of Liberec, Czech Republic) for advising us on the possible identification of unfortunately too damaged fouling bryozoans. We are grateful to G. Toutirais (UMR7205, MNHN, France) and V. Rommevaux (UMR7207) for assisting us with the gills drying at the critical point and the provision of the matrix thin sections, respectively. We thank L. Corbari (UMR7205) for helping us obtain gills of extant Cancer and L. Caze (UMR7207) for photographing fossil specimens. We warmly thank S. Pont (UMR7590) for his assistance with the SEM and EDS analyses, and thank to C. Jauvion (UMR7590) for interesting discussion on phosphatization processes. Finally, we thank T.F. Templeton for having reviewed the English wording of this work.