Introduction
Our understanding of the morphology and systematics of Hurdia Walcott, Reference Walcott1912 has greatly expanded in recent years, and it is now recognized as a significant taxon within Radiodonta present in several of the well-known Cambrian soft-bodied biotas including the Burgess Shale in Canada and the nearby Stanley Glacier, Marble Canyon, Tulip Beds, and Mount Stephen sites (Daley et al., Reference Daley, Budd, Caron, Edgecombe and Collins2009, Reference Daley, Budd and Caron2013a); the Jince Formation in the Czech Republic (Chlupáč and Kordule, Reference Chlupáč and Kordule2002, fig. 7); Wheeler Formation (Robison and Richards, Reference Robison and Richards1981, pl. 4, fig. 1a, b) and the Spence Shale (Daley et al., Reference Daley, Budd and Caron2013a) in Utah, USA; the Shuinjingtuo Formation in China (Cui and Huo, Reference Cui and Huo1990); and the Fezouata Biota in Morocco (Van Roy and Briggs, Reference Van Roy and Briggs2011, figs. 1d–i, S4a–c, 1l, S3c,d, S4f). Notably, the soft-bodied biotas from the middle Cambrian (Series 3) of Utah have yielded a large number of specimens previously identified as radiodontans in general, and usually Anomalocaris Whiteaves, Reference Whiteaves1892 or Peytoia Walcott, Reference Walcott1911 (Daley and Bergström, Reference Daley and Bergström2012) (e.g., Conway Morris and Robison, Reference Conway Morris and Robison1982, Reference Conway Morris and Robison1988; Briggs and Robison, Reference Briggs and Robison1984; Robison, Reference Robison1991; Briggs et al., Reference Briggs, Lieberman, Hendricks, Halgedahl and Jarrard2008), but the systematic position of most of this material has not yet been reevaluated in light of the new discoveries on Hurdia. By analysis of appendages and mouthparts originally described by Conway Morris and Robison (Reference Conway Morris and Robison1988), Daley et al. (Reference Daley, Budd and Caron2013a) were able to conclude that Hurdia was in fact present in the middle Cambrian (Series 3) of Utah alongside Peytoia, and they described four new specimens from the Spence Shale. Herein, we reconsider the identifications of radiodontan specimens from Utah in detail and confirm that Hurdia is well represented there. Further, we identify H. victoria Walcott, Reference Walcott1912 in the Spence Shale for the first time. A 3D model of an idealized Hurdia appendage potentially allows characters used in previous phylogenetic analyses (e.g., Cong et al., Reference Cong, Ma, Hou, Edgecombe and Strausfeld2014; Vinther et al., Reference Vinther, Stein, Longrich and Harper2014; Van Roy et al., Reference Van Roy, Daley and Briggs2015) to be visualized and evaluated in the hopes of possibly inferring which characters might be influenced by taphonomic factors.
The middle Cambrian (Series 3) of Utah is well known for its soft-bodied deposits that preserve a diverse array of taxa in several different depositional settings (Robison, Reference Robison1991; Briggs et al., Reference Briggs, Lieberman, Hendricks, Halgedahl and Jarrard2008; Gaines et al., Reference Gaines, Briggs and Yuanlong2008, Reference Gaines, Hammarlund, Hou, Qi, Gabbott, Zhao, Peng and Canfield2012; Brett et al., Reference Brett, Allison, DeSantis, Liddell and Kramer2009; Halgedahl et al., Reference Halgedahl, Jarrard, Brett and Allison2009). The Gunther family of Utah, along with Richard Robison (Robison, Reference Robison1965; Gunther and Gunther, Reference Gunther and Gunther1981), played a pivotal role in helping this treasure trove of fossils come to light. Many significant finds have been made from these deposits over the years (Resser, Reference Resser1939; Brooks and Caster, Reference Brooks and Caster1956; Briggs and Robison, Reference Briggs and Robison1984; Babcock and Robison, Reference Babcock and Robison1988; Conway Morris and Robison, Reference Conway Morris and Robison1986, Reference Conway Morris and Robison1988; Robison and Wiley, Reference Robison and Wiley1995; Briggs et al., Reference Briggs, Lieberman, Halgedahl and Jarrard2005), and new discoveries continue to be made (Robison and Babcock, Reference Robison and Babcock2011; Stein et al., Reference Stein, Church and Robison2011; Conway Morris et al., Reference Conway Morris, Selden, Gunther, Jamison and Robison2015; LoDuca et al., Reference LoDuca, Caron, Schiffbauer, Xiao and Kramer2015; Robison et al., Reference Robison, Babcock and Gunther2015). Taxa from these deposits have also provided insights into higher-level arthropod relationships (Hendricks and Lieberman, Reference Hendricks and Lieberman2008) while forming a core source of data used to study paleobiogeographic and macroevolutionary patterns during the Cambrian radiation interval (Hendricks et al., Reference Hendricks, Lieberman and Stigall2008).
Non-hurdiid radiodontans reported from the Langston Formation (Spence Shale Member), Wheeler Formation, and Marjum Formation are limited to two body fossils of Anomalocaris: one from the Spence Shale and one from the Wheeler Formation, both described by Briggs et al. (Reference Briggs, Lieberman, Hendricks, Halgedahl and Jarrard2008, figs. 1, 3). Neither specimen has well-preserved large frontal appendages, and the two specimens seem to represent two different and new species. Isolated appendages of Anomalocaris aff. A. canadensis Whiteaves, Reference Whiteaves1892 and Anomalocaris? sp. from the younger (Guzhangian) Weeks Formation in Utah have been described by Lerosey-Aubril et al. (Reference Lerosey-Aubril, Hegna, Babcock, Bonino and Kier2014). No new Anomalocaris appendages or bodies were identified during the course of this study. We emphasize new findings relating to Hurdia and Peytoia.
As is the case for other radiodontans, Hurdia and Peytoia are found mostly as isolated elements (carapace elements, mouthparts, appendages, and body flaps) and rarely as whole bodies, which can at times make taxonomic identification challenging. In general, the morphology of Hurdia can be described as follows. The head region comprises a pair of frontal appendages either side of a circular oral cone. The oral cone is made up of four large plates, equally spaced, with seven small plates between each pair of large plates; these surround an opening with multiple inner rows of teeth. A large frontal carapace of three sclerotized elements (two lateral P-elements and one dorsal H-element) and stalked eyes complete the head region. The body is made up of seven to nine segments, with reduced swimming flaps and prominent setal structures (Daley et al., Reference Daley, Budd, Caron, Edgecombe and Collins2009, Reference Daley, Budd and Caron2013a). A morphometric analysis showed that there are two species of Hurdia, H. victoria and H. triangulata Walcott, Reference Walcott1912, which are differentiated by comparing the length and width of the carapace H-element (Daley et al., Reference Daley, Budd and Caron2013a). Hurdia and Peytoia have recently been recovered within Hurdiidae (e.g., Van Roy et al., Reference Van Roy, Daley and Briggs2015), but these genera differ in a number of ways. Peytoia and Hurdia have a similar overall frontal appendage morphology in that both have elongated ventral spines, but these differ in numerous details, including the number and length-width ratio of the podomeres and the shape, arrangement, and number of ventral spines (Daley et al., Reference Daley, Budd and Caron2013a). Hurdia has a complex frontal carapace composed of three sclerite elements, whereas Peytoia has no evidence for such a large frontal carapace, with only traces of possible carapace material immediately surrounding the head in ventrally preserved specimens (Daley et al., Reference Daley, Budd, Caron, Edgecombe and Collins2009). The oral cone has the same arrangement of outer plates in Hurdia and Peytoia, but the multiple inner rows of teeth present in Hurdia are absent in Peytoia. The body trunk in Hurdia consists of seven to nine segments that are more cylindrical than the dorsoventrally flattened body of Peytoia, which has 13 body segments. The swimming flaps of Hurdia are much smaller than the wide flaps of Peytoia, but setal blades are more prominent in Hurdia as compared to Peytoia (Whittington and Briggs, Reference Whittington and Briggs1985, fig. 101).
Materials and methods
One body specimen (USNM 374593) is held at the Smithsonian Museum of Natural History, Washington, D.C., USA. The remainder of the material studied is held at the Division of Invertebrate Paleontology, Biodiversity Institute, University of Kansas, Lawrence, USA (KUMIP). Detailed information for the fossil localities is available in Hendricks et al. (Reference Hendricks, Lieberman and Stigall2008, table 3). All specimen numbers, previous publications, and new identifications are provided in Table 1.
Table 1 Specimens examined in this study, including original and new taxonomic interpretations.

Photographs were taken with a Canon EOS 500D DSLR Camera with Canon EF-S 60 mm Macro Lens, controlled for remote shooting using the EOS Utility 2 program. Photographs were taken under cross-polarized light, under nonpolarized light, wet and dry, and under high- and low-angle lighting. Measurements for calculating RI values, and length:width ratios were taken from digital photographs using ImageJ 2.The 3D model was made using Blender 2.76b. A box model was created from a sketch of Hurdia adapted from Daley and Budd (Reference Daley and Budd2010). This was modified with a subdivision surface and rendered to a video. A phylogenetic analysis in TNT v. 1.5 (Goloboff and Catalano, Reference Goloboff and Catalano2016) was run using implicit enumeration under equal weighting on a data matrix modified from Van Roy et al. (Reference Van Roy, Daley and Briggs2015) consisting of 33 taxa and 61 characters. Modifications to the phylogenetic analysis data matrix were made in Mesquite v. 3.2 (Maddison and Maddison, Reference Maddison and Maddison2017).
Geologic setting
The Spence Shale Member of the Langston Formation, middle Cambrian Series 3, Stage 5, is a diverse soft-bodied biota (Gunther and Gunther, Reference Gunther and Gunther1981; Robison, Reference Robison1991; Liddell et al., Reference Liddell, Wright and Brett1997), and knowledge of the paleontology, sedimentology, geochemistry, and taphonomy of this deposit has increased substantially over the past few years (Briggs et al., Reference Briggs, Lieberman, Hendricks, Halgedahl and Jarrard2008; Gaines et al., Reference Gaines, Hammarlund, Hou, Qi, Gabbott, Zhao, Peng and Canfield2012; Garson et al., Reference Garson, Gaines, Droser, Liddell and Sappenfield2012; Olcott Marshall et al., Reference Olcott Marshall, Wehrbein, Lieberman and Marshall2012; Gaines, Reference Gaines2014; Kloss et al., Reference Kloss, Dornbos, Chen, McHenry and Marenco2015). The Spence Shale is primarily made up of shale, with some limestone, and it is developed in a series of parasequences (Liddell et al., Reference Liddell, Wright and Brett1997; Garson et al., Reference Garson, Gaines, Droser, Liddell and Sappenfield2012). Detailed discussions of the sedimentology, taphonomy, and geochemistry of the Spence Shale are provided by Liddell et al. (Reference Liddell, Wright and Brett1997), Garson et al. (Reference Garson, Gaines, Droser, Liddell and Sappenfield2012), and Kloss et al. (Reference Kloss, Dornbos, Chen, McHenry and Marenco2015), respectively. All the specimens from the Spence Shale discussed herein come from the Wellsville Mountains of northern Utah (Hendricks et al., Reference Hendricks, Lieberman and Stigall2008; Hendricks, Reference Hendricks2013).
The Wheeler Formation, Drumian, Cambrian Series 3, from the House Range of Utah is slightly younger than the Spence from the Wellsvile Mountains, and it too contains a diverse soft-bodied biota (Robison, Reference Robison1964, Reference Robison1991; Gunther and Gunther, Reference Gunther and Gunther1981; Briggs and Robison, Reference Briggs and Robison1984; Rogers, Reference Rogers1984; Rees, Reference Rees1986; Robison et al., Reference Robison, Babcock and Gunther2015). There have been a substantial number of relatively recent sedimentological, taphonomic, and geochemical studies of the soft-bodied biota from this formation and region (e.g., Gaines and Droser, Reference Gaines and Droser2003, Reference Gaines and Droser2005; Briggs et al., Reference Briggs, Lieberman, Hendricks, Halgedahl and Jarrard2008; Brett et al., Reference Brett, Allison, DeSantis, Liddell and Kramer2009; Halgedahl et al., Reference Halgedahl, Jarrard, Brett and Allison2009; Gaines, Reference Gaines2014). The unit consists of homogeneous mudstones and interbedded mudstones with thin-grained, fine-bedded limestones. The soft-bodied material occurs primarily within carbonaceous shales (Gaines and Droser, Reference Gaines and Droser2003, Reference Gaines and Droser2005).
The still slightly younger soft-bodied deposits from the Marjum Formation, Drumian, Cambrian Series 3, generally resemble lithologically, stratigraphically, and taphonomically those deposits from the Wheeler Formation where it is exposed in the House Range (Elrick and Snider, Reference Elrick and Snider2002; Brett et al., Reference Brett, Allison, DeSantis, Liddell and Kramer2009; Gaines and Droser, Reference Gaines and Droser2010) although they represent a shallower facies (Briggs and Robison, Reference Briggs and Robison1984; Brett et al., Reference Brett, Allison, DeSantis, Liddell and Kramer2009).
The relative global chronostratigraphic ages and polymerid trilobite biostratigraphy of Radiodonta-preserving units in Utah and British Columbia can be seen in Figure 1.

Figure 1 Stratigraphic column showing relative ages of Burgess Shale, Spence Shale, Wheeler Formation, Marjum Formation, and Weeks Formation, with reference to global chronostratigraphic units and polymerid trilobite biostratigraphy. Adapted from Robison et al. (Reference Robison, Babcock and Gunther2015).
Results
Taxonomic identifications of new and previously described material are summarized in Table 1. Hurdia victoria Walcott, Reference Walcott1912 is described for the first time from the Spence Shale. Hurdia also occurs in the Wheeler Formation. Peytoia occurs in the Wheeler and Marjum formations, but should no longer be reported as present in the Spence Shale.
Hurdia from the Spence Shale Member
Some of the material interpreted as Hurdia from the Spence Shale comprises appendages and mouthparts (Figs. 2, 3); these include both previously described specimens (Briggs et al., Reference Briggs, Lieberman, Hendricks, Halgedahl and Jarrard2008; Daley et al., Reference Daley, Budd and Caron2013a, fig. 24) and new material. New carapace material (Fig. 4.1–4.5), which allows identification to the species level, and a large, isolated flap (Fig. 4.6, 4.7) are also discussed here for the first time. In addition, appendages previously interpreted as Peytoia nathorsti Walcott, Reference Walcott1911 (Conway Morris and Robison, Reference Conway Morris and Robison1988) are here reinterpreted as belonging to a Sidneyia-like taxon.
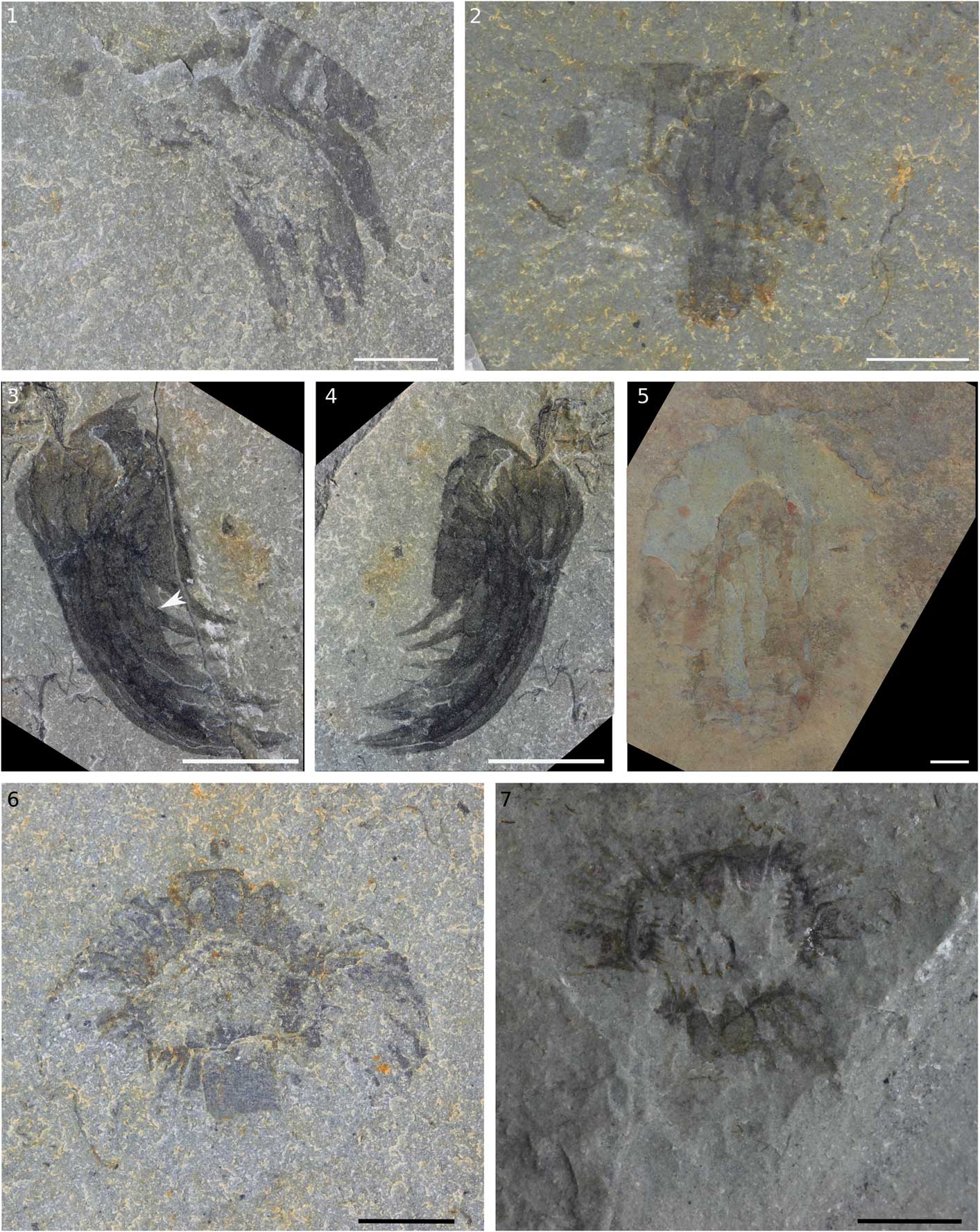
Figure 2 Hurdiid appendages and oral cones from the Spence Shale Member, Langston Formation, Wellsville Mountains, Utah, USA. (1) Appendage KUMIP 314145; (2) appendage KUMIP 314178; (3) appendage KUMIP 314040a with arrow indicating broken ventral spine; (4) KUMIP 314040b, counterpart to 3; (5) appendage KUMIP 314042; (6) oral cone KUMIP 314175a; (7) oral cone KUMIP 314265a. Scale bars=5 mm.

Figure 3 Assemblage of two Hurdia appendages with an oral cone. (1) KUMIP 312405a; (2) KUMIP 312405b, counterpart to 1; (3) interpretative drawing of 2. app. 1=appendage 1; app. 2=appendage 2; as=auxiliary spine; lp=large plate; p1=podomere 1; p6=podomere 6; tr=tooth row; ts=terminal spine; vs=ventral spine. Scale bars=10 mm.

Figure 4 Hurdia carapace elements and flap from the Spence Shale Member, Langston Formation, Wellsville Mountains, Utah, USA. (1) H-element KUMIP 314050; (2) H-element KUMIP 314039; (3) H-element 314058; (4) boxed region in 1; (5) Boxed region in 3; (6) flap KUMIP 314057b; (7) KUMIP 314057a, part to 6. (1–3, 6, 7) Scale bars=10 mm; (4, 5) scale bars=2.5 mm.
KUMIP 314145a/b (Fig. 2.1) is a small, single incomplete Hurdia appendage with seven visible podomeres with well-defined boundaries of around 1 mm in thickness. Podomeres at the proximal end of the appendage where the ventral spines attach are not preserved. KUMIP 314178 (Fig. 2.2) is a mostly complete small, single Hurdia appendage with 10 podomeres separated by clear podomere boundaries of around 1 mm thickness. KUMIP 314040a/b (Fig. 2.3, 2.4) is a small Hurdia appendage with nine podomeres. Five large ventral spines, attached to podomeres 2–6, are tightly packed and appear curved forward, beyond the distal end of the appendage. Auxiliary spines are only visible on the distal-most ventral spine. KUMIP 314042 (Fig. 2.5) is a larger Hurdia appendage with 10 podomeres with clear podomere boundaries of around 1 mm thickness. The five large, straight ventral spines have slightly curved distal ends.
Briggs et al. (Reference Briggs, Lieberman, Hendricks, Halgedahl and Jarrard2008) identified KUMIP 312405a/b (Fig. 3) as a pair of radiodontan appendages with mouthparts. The two appendages are preserved with one (‘app. 1’ in Fig. 3.3) on a higher level of rock than the other (‘app. 2’ in Fig. 3.3). App. 1 is well preserved and made up of 10 podomeres. Large ventral spines are present on podomeres 2–6, and a small ventral spine is visible on podomere 9 (‘vs’ in Fig. 3.3). A terminal spine is visible on podomere 10 (‘ts’ in Fig. 3.3). App. 2 is not as clearly visible. The distal-most podomeres are visible. Three large ventral spines are preserved together, with the distal one angled forward, similar to the overlying appendage. The mouthparts are made up of four large plates (‘lp’ in Fig. 3.3) arranged at 90° to each other around a rectangular opening. The total number of smaller plates is not clear, as the outer edge of the oral cone is not well preserved, but where it can be counted there are seven smaller plates between the large plates, which extrapolates to a total of 32 plates, four large and 28 small, characteristic of Hurdia and Peytoia. By contrast, Anomalocaris mouthparts have three large plates at 120° (Daley and Bergström, Reference Daley and Bergström2012). Peytoia mouthparts can be differentiated from Hurdia as Hurdia has numerous tooth rows in the central opening, whereas in Peytoia the central opening lacks tooth rows (Daley and Bergström, Reference Daley and Bergström2012). In the central opening of this specimen, additional tooth rows are visible (‘tr’ in Fig. 3.3), indicating this specimen is a Hurdia. The appendages associated with the mouthparts are consistent with this interpretation and are likely from the same animal. KUMIP 314175a/b (Fig. 2.6) is a small, oval oral cone of Hurdia. It is unusual in that it has small raised nodes (radius 1 mm) visible on one of the large plates and several small plates. KUMIP 314265a/b (Fig. 2.7) is another small Hurdia oral cone. The outer margins of the plates are not preserved, but multiple inner rows of teeth in an approximately rectangular central opening are clearly visible. Again, there are some possible small round nodes (radius 1 mm) visible on some plates.
The length:width ratio of H-elements from the carapace of Hurdia can be used to distinguish H. victoria from H. triangulata: H. victoria has H-elements with lengths greater than 1.5 times the width (but less than 2.0 times), and H. triangulata has H-elements with lengths less than 1.5 times the width (Daley et al., Reference Daley, Budd and Caron2013a). KUMIP 314039 (Fig. 4.2), KUMIP 314050 (Fig. 4.1, 4.4), and KUMIP 314056 (Fig. 4.3, 4.5), identified by height:width ratios, are the first H. victoria specimens identified from the Spence Shale; H. triangulata has not yet been identified. Reticulation polygons were observed on parts of the surface of some elements (Fig. 4.4). The specimen illustrated in Figure 4.3, 4.5 has 10 small brown patches (1–5 mm in radius) and a trilobite with inferred manganese dendrites radiating from it, obscuring parts of the fossil. Similar dendrites with elevated manganese content have been reported from the Pioche Shale (Moore and Lieberman, Reference Moore and Lieberman2009). Evidence for the two-layered H-element can be seen toward the strengthened tip (Fig. 4.5).
KUMIP 314057a/b (Fig. 4.6, 4.7) is a part and counterpart of an isolated radiodontan swim flap covered with regularly spaced, prominent transverse lines, also referred to as ‘strengthening rays’ (Whittington and Briggs, Reference Whittington and Briggs1985) or ‘veins’ (Chen et al., Reference Chen, Ramsköld and Zhou1994; Hou et al., Reference Hou, Bergström and Ahlberg1995), about 1 mm wide and 2 mm apart. The flap is relatively large compared to Hurdia flaps reported from the Burgess Shale (Daley et al., Reference Daley, Budd and Caron2013a), measuring approximately 65 mm in width and 45 mm in height. This specimen is tentatively identified as Hurdia because of the presence of transverse lines across the entire surface of the flap, which is not seen in Peytoia (where the transverse lines are confined to the anterior half of the flap) or Anomalocaris (which lacks transverse lines entirely).
Sidneyia? from the Spence Shale Member
Conway Morris and Robison (Reference Conway Morris and Robison1988, fig. 26.1a, 26.1b, 26.2) identified four specimens (KUMIP 204777–204780) as broken spines of Peytoia nathorsti appendages. These are reinterpreted as distal podomeres of endopods (walking appendages) of a Sidneyia-like taxon on the basis of the rounded curvature of the overall structure, the oblique angle of the spines, the characteristic arrangement of repetitive bundles of decreasing spine size, and the presence of podomere boundaries faintly visible on some specimens (compare KUMIP 204777–204780: Conway Morris and Robison, Reference Conway Morris and Robison1988, fig. 26.1a, 26.1b, 26.2 to Bruton, Reference Bruton1981, figs. 48, 53, 55, 58, 60, 88, 92 and Stein, Reference Stein2013, fig. 7B–D). This therefore indicates Peytoia should no longer be reported as present in the Spence Shale. Sidneyia was previously reported from the Spence Shale (Briggs et al., Reference Briggs, Lieberman, Hendricks, Halgedahl and Jarrard2008).
Hurdiids from the Wheeler Formation
Hurdia is known from the Wheeler Formation by a single P-element. Peytoia is known from one appendage and several mouthparts. KUMIP 153901a/b (Fig. 5.6, 5.7) was first described by Robison and Richards (Reference Robison and Richards1981, pl., 4, fig. 1a, b) as Proboscicaris agnosta, which at the time was thought to be a phyllocarid. Proboscicaris is now identified as the P-element of the Hurdia carapace (Daley et al., Reference Daley, Budd, Caron, Edgecombe and Collins2009). KUMIP 314086a/b (Fig. 5.1, 5.2) was first described by Briggs et al. (Reference Briggs, Lieberman, Hendricks, Halgedahl and Jarrard2008, fig. 2.2) as a radiodontan appendage. Owing to the relatively limited preservation, they did not classify it to genus. It is an appendage with 10 podomeres, with elongated ventral spines on podomeres 2–6. Six auxiliary spines are present perpendicular to the ventral spine of podomere 5. There are three small triangular terminal spines on podomere 10. The presence of three terminal spines, the orientation of ventral spines, and the curved distal end indicate it is a Peytoia appendage. Conway Morris and Robison (Reference Conway Morris and Robison1982, text-fig. 1, pl. 1, figs. 1–5) described two specimens, KUMIP 153093a/b (Fig. 5.10, 5.11) and KUMIP 153094 (Fig. 5.5), of radiodontan oral cones as Peytoia cf. P. nathorsti, and we support this interpretation because of the overall arrangements of plates and the lack of tooth rows inside the main opening. The genuine absence of additional rows of teeth can be confirmed by examining the central opening, which is well preserved. KUMIP 314078 (Fig. 5.8, 5.9), first described by Briggs et al. (Reference Briggs, Lieberman, Hendricks, Halgedahl and Jarrard2008, fig. 2.2), is an oral cone with four large plates and seven smaller plates between each pair of larger plates. Part of the mouth apparatus is not preserved, but it can be inferred that it had 32 plates (four large, 28 small) radially arranged. The central opening of the incomplete mouth apparatus does not have additional tooth rows, so it can be identified as Peytoia.

Figure 5 Hurdiid appendages, oral cones, and carapace element from the Wheeler Formation, House Range, Utah, USA. (1) Appendage KUMIP 314086b; (2) KUMIP 314086a, part to 1; (3) appendage KUMIP 204781a; (4) KUMIP 204781b, counterpart to 3; (5) oral cone KUMIP 314094; (6) Hurdia P-element 153901a; (7) KUMIP 153901b, counterpart to 6; (8) oral cone KUMIP 314078b; (9) KUMIP 314078a, part to 8; (10) oral cone KUMIP 153093b; (11) KUMIP 153093a, part to 10. Scale bars=10 mm.
Conway Morris and Robison (Reference Conway Morris and Robison1988, fig. 26.3) identified KUMIP 204781a/b (Fig. 5.3, 5.4) from the Wheeler Formation as a P. nathorsti appendage. A previous taxonomic analysis (Daley et al., Reference Daley, Budd and Caron2013a) suggested that this was potentially a Hurdia appendage. As the distal end of the appendage is not preserved and the morphology of the ventral spines is not conclusive, it is identified here as a hurdiid, but no identification to the genus level is made.
Peytoia from the Marjum Formation
Hurdia is not known from the Marjum Formation. Briggs and Robison (Reference Briggs and Robison1984) identified USNM 374593 (Figs. 6, 7) from the Marjum Formation as a partial body (lacking frontal appendages) of Peytoia nathorsti because of the presence of transverse lines on the flaps. These had only been observed in P. nathorsti and not Anomalocaris canadensis, which at the time was the only other radiodontan body type known. We support placement in Peytoia because of the presence of large posterior-tapering swim flaps (in contrast to the small flaps of Hurdia) with transverse lines (which are absent in Anomalocaris) and the absence of a tail fan (present in Hurdia and Anomalocaris). The specimen consists of the 11 most posterior segments and tail of the animal, with flaps and central body structures preserved together. There is slight overlap of the anterior and posterior edges of the flaps and the presence of some high-relief mineralized structures (Fig. 7). A dark brown-grey linear structure (‘ba’ in Fig. 6.5) runs down the median axis of the animal, 6–7 mm wide near the anterior, tapering to a point and disappearing as it reaches the pair of body flaps. This region has a very thin (1 mm wide) feature at its midline running along the length of the body, particularly visible in the counterpart (‘g’ in Fig. 6.5). This is interpreted to be the gut running through the body cavity. It is flanked on both sides by a series of bilaterally symmetrical dark grey features (‘s1–s11’ in Fig. 6.5). They are larger anteriorly (3×25 mm) than posteriorly (1.5×10 mm) and are interpreted as setal blade blocks because of their preservation, position, and co-occurrence with body flaps. Lateral to the setal blade structures, and partly overlapping them, there is a series of dark reflective structures with high relief, present in the region where the base of the flaps meets the axial region (‘m1–m6’ in Fig. 6.5). These structures are interpreted as musclulature because of similarities between them and musculature in Anomalocaris canadensis (Daley and Edgecombe, Reference Daley and Edgecombe2014, figs. 15, 17). Both have a fibrous texture (Fig. 7.3–7.5), are similar in size and shape (Fig. 7), and are at the base of body flaps (Fig. 7.1, 7.2). In A. canadensis, these structures are preserved as an orange material or as a high-relief dark grey to black reflective material. In Peytoia (USNM 374593), they are similarly preserved as high-relief dark reflective material, although the fibrous details are less well preserved than in A. canadensis (compare Fig. 7.3, 7.5 to Fig. 7.4). They are not interpreted as gut diverticulae, which are often preserved as high-relief dark reflective material, as they do not intersect the gut and are instead associated with the intersection of the body flaps with the cuticularized body, far from the body axis. However, it must be noted that euarthropod gut diverticulae are preserved in a variety of ways (Lerosey-Aubril et al., Reference Lerosey-Aubril, Hegna, Kier, Bonino, Habersetzer and Carré2012), and the preservation of this musculature is different from musculature reported from some other Burgess-Shale type localities: Pambdelurion from Sirius Passet (Budd, Reference Budd1998) and Myoscolex from the Emu Bay Shale (Briggs and Nedin, Reference Briggs and Nedin1997).

Figure 6 Peytoia partial body and partial oral cone from the Marjum Formation, House Range, Utah, USA, USNM 374593 (1) Counterpart; (2) part; (3) box from 1, showing flap and strengthening rays; (4) box from 2; arrow indicates high-relief linear structures; (5) interpretive sketch of 1. ba=body axis; s1–11=setal blade blocks, labeled anterior to posterior; df=dorsal flap; g=gut; hr?=head region?; m1–6=muscle blocks, labeled anterior to posterior; st=staples; t=tail; vf=ventral flap. (6) Partial oral cone KUMIP 314095b; (7) part to 6; Scale bars=10 mm.

Figure 7 Comparison of musculature in Peytoia partial body from the Marjum Formation, House Range, Utah, USA, and Anomalocaris from the Burgess Shale, British Columbia, Canada. (1) USNM 374593, box 7.1 from Figure 6.1 , showing position of musculature at the base of flaps; (2) ROM 62547, showing position of musculature at the base of flaps; (3) box from 1, showing faint linear features in musculature; (4) box from 2, showing clear linear features in musculature; (5) box 7.5 from Figure 6.1 , showing linear features in matrix where musculature has been removed. (1, 2) Scale bars=10 mm; (3, 4, 5) scale bars=1 mm.
The second most anterior flap on the right side of the counterpart preserves a set of high-relief linear structures near its base, located between the musculature of this flap and the flap in front of it (Fig. 6.4, ‘st’ in Fig. 6.5). The six parallel, evenly spaced structures are mineralized, and although they are closely packed, they do not touch one another. The longest one, closest to the body axis, is just under 1 mm in length, and the structures become shorter away from the body axis, with the shortest one just under 0.5 mm in length. Two millimeters below the linear structures, there are a number of circular mineralized structures, around 0.25 to 0.5 mm in diameter. Small spheres 0.5 mm in diameter are present on other phosphatized blocks. Similar structures, which were identified as clusters of pyrite framboids, have been reported from the middle Cambrian (Series 3) Pioche Shale by Moore and Lieberman (Reference Moore and Lieberman2009). Transverse lines only cover the anterior portion of the flap (Fig. 6.3), and no internal structure of the flaps is preserved, similar to P. nathorsti from the Burgess Shale (Whittington and Briggs, Reference Whittington and Briggs1985). Ten large ventral flaps (‘vf1–vf10’ in Fig. 6.5) are preserved on the side that most clearly shows a dorsal flap (‘df1’ in Fig. 6.5), and six large ventral flaps are preserved on the other side (‘vf1–vf6’ in Fig. 6.5), with one dorsal flap preserved there also (‘df1’ in Fig. 6.5). The front pair of flaps is the largest, and they reduce in size sequentially. The flaps associated with body segments 7–11 are overlapping due to the orientation of preservation. There are no flaps associated with the tail (‘t’ in Fig. 6.5). On the part, two dorsal flaps are also preserved at the front of the animal, in addition to the larger ventral flaps (‘df1’ in Fig. 6.5).
A partial mouthpart, KUMIP 314095 (Fig. 6.6, 6.7) is identified as Peytoia because of the visible plate morphology and lack of internal tooth rows. One large plate with large triangular inner spines is preserved, with five smaller plates on one side and seven on the other side of the large plate. These smaller plates are a regular size and overlap each other, with the plate closer to the large plate overlapping the one next closest. The partially preserved central opening shows no evidence of additional rows of teeth. The large plate has 10 small triangular spines pointing inward, the widest of which, at a central point of the plate, is around 2 mm. The others are smaller, at around 1 mm wide. Some of the smaller plates have a single projection also pointing inward, around 1 mm wide. Unusually for Peytoia, this mouthpart has small (diameter approximately 0.3 mm) nodes on the surface of the large plate and some adjacent plates (visible on both part and counterpart; Fig. 6.6, 6.7).
Discussion
Morphological interpretations on Hurdia appendages can be influenced by specimen orientation
Hurdia appendages are preserved in a variety of orientations (see Daley et al., Reference Daley, Budd and Caron2013a). Ventral spines of Hurdia are often preserved curved, both anteriorly (e.g., Fig. 2.3, 2.4) and posteriorly (e.g., Fig. 2.1, 2.2), and straight (e.g., Figs. 2.5, 3), sometimes in the same specimen (e.g., Daley et al., Reference Daley, Budd, Caron, Edgecombe and Collins2009, fig. 2C). The appendages have some element of plasticity, and during preservation they can become deformed. In some specimens, the curvature of ventral spines appears to change along the length of the appendage due to the appendage being preserved at an angle (e.g., Daley et al., Reference Daley, Budd and Caron2013a, figs. 12C, E, 24A, where the distal-most ventral spines appear more curved as the appendage is rotated one way, and Daley et al., Reference Daley, Budd and Caron2013a, fig. 12G, where the proximal-most ventral spines appear more curved as the appendage is rotated the other way). Appendages not preserved at such angles tend to have the distal-most podomeres more clearly preserved, not overlapping more proximal podomeres (compare the position of the distal-most podomeres in Fig. 3 and Daley et al., Reference Daley, Budd and Caron2013a, fig. 12A, to those described as rotated).
The effect that these preservational factors might have on morphological reconstructions and inferred evolutionary affinities can be observed by considering phylogenetic analyses of Radiodonta. Recent phylogenies (Cong et al., Reference Cong, Ma, Hou, Edgecombe and Strausfeld2014; Van Roy et al., Reference Van Roy, Daley and Briggs2015) based on the data matrix and analysis of Vinther et al. (Reference Vinther, Stein, Longrich and Harper2014) consider four distinct representatives of Hurdia: H. victoria, H. cf. victoria Utah, H. sp. B Spence Shale, and H. sp. B Burgess Shale (the latter two were coded identically except for missing character states). Other than missing character states, H. victoria and H. cf. victoria Utah only differ in the condition of Character 29: Vinther et al. (Reference Vinther, Stein, Longrich and Harper2014) coded H. victoria as having distally projecting dorsal spines on the terminal segments; these were coded as absent in Hurdia cf. victoria Utah. Vinther et al. (Reference Vinther, Stein, Longrich and Harper2014) coded Hurdia victoria (including Hurdia cf. victoria Utah) and H. sp. B as differing in three characters. In Character 34, the ventral spines were coded as broader distally than proximally in Hurdia victoria and subequal or narrower distally in Hurdia sp. B. In character 39, the distal tips of the ventral spines are hooked forward in Hurdia victoria but strongly hooked forward and forming a 90° angle with the spine base in Hurdia sp. B. The phylogenetic significance of Characters 29, 34, and 39 may be called into question by the aforementioned preservational variation. Similarly, Character 46 (curvature of ventral spines) may reflect preservational rather than taxonomic variation. Hurdia sp. B was coded as having proximal ventral spines that curve posteriorly, whereas H. victoria was coded as having ventral spines all straight or anteriorly curved. However, H. victoria specimens with straight proximal ventral spines and anteriorly curving distal ends are common (e.g., Daley et al., Reference Daley, Budd and Caron2013a, fig. 12A, C, E, G), and this reflects taphonomic variation.
To visualize how the angle of preservation influences morphological interpretations of Hurdia appendages, a 3D model was created in Blender based on the morphology of the Hurdia appendage presented by Daley and Budd (Reference Daley and Budd2010, text-fig. 1D). This 3D model (Fig. 8) suggests that the apparent broadness of ventral spines on distal podomeres will be influenced by how a specimen is oriented when it is preserved, and so the broadness of ventral spines (Vinther et al., Reference Vinther, Stein, Longrich and Harper2014, Character 34) is likely not a good character for distinguishing Hurdia species. A small difference in orientation affecting apparent thickness of ventral spines can be seen by comparing KUMIP 314086 (Fig. 5.1, 5.2, with ventral spines of equal thickness) and KUMIP 314042 (Fig. 2.5, where the distal-most ventral spine appears thicker because of its orientation). This is visualized by the 3D model, where Figure 8.1 (no rotation) shows ventral spines of equal thickness, and Figure 8.2 (small rotation) shows an apparently thicker distal-most ventral spine. A more extreme example of the variation in the orientation of appendage preservation can be seen in the two appendages of KUMIP 312405 (Fig. 3). These appendages are presumably from the same animal but preserved at very different orientations.

Figure 8 3D model of Hurdia appendage, with ventral spines reconstructed as being of equal thickness. (1) Lateral view, showing ventral spines appearing equally thick; (2) oblique view, showing distal ventral spines appearing thicker than proximal ones and differences in ‘hooked’ appearance at distal tip of ventral spines.
In summary, Vinther et al.’s (Reference Vinther, Stein, Longrich and Harper2014) characters 29, 34, 39, and 46, which comprise the evidence to distinguish four different representatives of Hurdia, may be influenced by preservational factors. A phylogenetic analysis of the data matrix from Van Roy et al. (Reference Van Roy, Daley and Briggs2015), which is based on the original data matrix of Vinther et al. (Reference Vinther, Stein, Longrich and Harper2014), was run in TNT v. 1.5 using implicit enumeration under equal weighting. The data matrix was modified in the following ways: In Character 29, H. cf. victoria Spence is coded as dorsal spines present, and both H. sp. B taxa are coded as unknown; Character 34 was deleted as it has been shown to reflect preservation and not true morphological difference; Character 39 (now Character 38) was changed to being unordered, and both H. sp. B taxa and Stanleycaris were coded as having hooked forward ventral spines; and in Character 46 (now Character 45), both Hurdia sp. B taxa are coded as having straight or curved anterior ventral spines. An analysis under equal weighting recovers 70 most parsimonious trees of 106 steps, and in strict consensus (CI=0.66, RI=0.85), all four Hurdia taxa and Stanleycaris are recovered in an unresolved polytomy. This is in contrast to the resolved relationships depicted in Vinther et al. (Reference Vinther, Stein, Longrich and Harper2014) and Van Roy et al. (Reference Van Roy, Daley and Briggs2015), where the two H. sp. B specimens form a clade that is sister to Stanleycaris, rather than to H. victoria. From current evidence, Hurdia cannot be identified to the species level by its frontal appendages alone, and appendages from the Spence Shale and the Burgess Shale cannot be distinguished as KUMIP 314040 and 314178, described herein, show that Hurdia appendages from Utah do possess dorsal spines (Fig. 2.2–2.4). Hurdia can still only be separated into two distinct species by the shape of its H-element (Daley et al., Reference Daley, Budd and Caron2013a).
Presence of nodes on mouthparts
Nodes are present on the plates of Hurdia mouthparts from the Spence Shale (KUMIP 314175a/b and 314265a/b, Fig. 2.6, 2.7) and partial Peytoia mouthparts from the Marjum Formation (KUMIP 314095, Fig. 6.6, 6.7). Nodes are not often seen in Burgess Shale specimens. The nodes are similar to what is seen in Anomalocaris (e.g., Daley and Bergström, Reference Daley and Bergström2012, fig. 2a–d; Daley and Edgecombe, Reference Daley and Edgecombe2014, fig. 7.5). However, the plates of these mouthparts lack the subdivisions and furrowing on the outer margins that is often seen in Anomalocaris (e.g., Daley and Bergström, Reference Daley and Bergström2012, fig. 2g–j). The presence of nodes in the Utah specimens could be due to interspecific variation; however, a more likely cause is preservational differences, which allow more 3D structure to be preserved in Utah than in Burgess Shale specimens. Similar preservational differences are seen in the oral cones of A. canadensis, where nodes are preserved in varying degrees of relief in oral cones from the Burgess Shale and the Emu Bay Shale (Daley et al., Reference Daley, Paterson, Edgecombe, García‐Bellido and Jago2013b; Daley and Bergström, Reference Daley and Bergström2012).
Geographical and temporal distribution of hurdiids
Hurdia and Peytoia are distributed over a large temporal and geographic range (Table 2). Both are reported from China, the United States, and Canada. Hurdia is known additionally from the Czech Republic (Chlupáč and Kordule, Reference Chlupáč and Kordule2002), and Peytoia from Poland (Daley and Legg, Reference Daley and Legg2015). This study shows that Peytoia is not known from the Spence Shale. This does not have any implications for the first or last appearance of Peytoia as its oldest occurrence is from Holy Cross Mountains (Daley and Legg, Reference Daley and Legg2015) and it is reported from the younger Marjum Formation (Briggs and Robison, Reference Briggs and Robison1984; this study); however, it does change the earliest known occurrence of P. nathorsti to the Burgess Shale. Hurdia is not yet known from the Marjum Formation; however, it is reported from the younger Fezouata Lagerstätten (Van Roy and Briggs, Reference Van Roy and Briggs2011). As Hurdia and Peytoia do not co-occur in the Spence Shale or Marjum Formations, a potential hypothesis is that the similarities of their frontal appendages, and hence similar predation methods, prevented the two genera from co-existing. Indeed, a recent morphospace analysis of the first appendages of 36 euarthropod taxa (Aria and Caron, Reference Aria and Caron2015) supports functional similarities in the feeding appendages of Peytoia and Hurdia, which plotted close together. However, Hurdia and Peytoia do co-occur in the Wheeler Formation, Tulip Beds, and Burgess Shale (Table 2), suggesting that they were capable of co-existing in the right environment, and the collection of more hurdiids from the Spence Shale and Marjum Formation may in fact show that Peytoia and Hurdia are present where currently they are not known.
Table 2 Locations from which hurdiid specimens are known. HCM=Holy Cross Mountains, Poland; Shuj.=Shuijingtuo Formation, China; Balang=Balang Formation, China; Jince=Jince Formation, Czech Republic; Spence=Langston Formation (Spence Shale Member), Utah, USA; Tulip=Tulip Beds, Mount Stephen, Yoho National Park, Canada; Burg.=Fossil Ridge, Burgess Shale, Yoho National Park, Canada; Stan.=Stanley Glacier, Kootenay National Park, Canada; Wheel.=Wheeler Formation, Utah, USA; Marj.=Marjum Formation, Utah, USA; Fez.=Fezouata Formation, Morocco. Publications: 1=Daley and Legg (Reference Daley and Legg2015); 2=Cui and Huo (Reference Cui and Huo1990); 3=Liu (Reference Liu2013); 4=Chlupáč and Kordule (Reference Chlupáč and Kordule2002); 5=Conway Morris and Robison (Reference Conway Morris and Robison1988); 6=Briggs et al. (Reference Briggs, Lieberman, Hendricks, Halgedahl and Jarrard2008); 7=Daley and Budd (Reference Daley and Budd2010); 8=Caron et al. (Reference Caron, Gaines, Mángano, Streng and Daley2010); 9=Robison and Richards (Reference Robison and Richards1981); 10=Briggs and Robison (Reference Briggs and Robison1984); 11=Van Roy and Briggs (Reference Van Roy and Briggs2011).

Acknowledgments
M. Florence provided access to the specimen at the USNM. We thank the editor, J. Jin; the associate editor, Z. Zhang; an anonymous reviewer; and J. Ortega-Hernández for their valuable comments; and P. Selden (University of Kansas) for use of photographic equipment. The program TNT was made available with the sponsorship of the Willi Hennig Society. Funding was provided by a Palaeontological Association Sylvester-Bradley Award (PA-SB201503) and Oxford-St Catherine's Brade-Natural Motion Scholarship to SP, the OUMNH to ACD, and NSF-EAR-0518976 to BSL.
Accessibility of supplemental data
Data available from the Dryad Digital Repository: http://doi.org/10.5061/dryad.c5g25.