1. Introduction
Zircon is an important accessory mineral phase generally rich in sediments and sedimentary rocks. Due to its chemical and physical stability, zircon can survive sedimentary processes like erosion, transport and deposition with inherent information on its source rocks intact (Link et al. Reference Link, Fanning and Beranek2005; Yang et al. Reference Yang, Zhang and Wang2012; Liu et al. Reference Liu, Wu, Fisher, Hanchar, Beranek, Gao and Wang2017). Modern large rivers have extensive drainage areas and strong transport capacities, so detrital zircons from rivers can provide valuable information on crustal growth and evolution, which cannot be fully revealed by zircons from exposed rocks. In recent decades, integrated U–Pb and Lu–Hf isotope analyses of detrital zircons from worldwide fluvial sediments have been carried out with the aim of characterizing continental crustal growth and evolution and tracing sediment provenances in the catchments. The Mississippi, Indus, Amazon, Yellow, Mekong, Hantan and Yangtze rivers are among those studied (Rino et al. Reference Rino, Komiya, Windley, Katayama, Motoki and Hirata2004; Iizuka et al. Reference Iizuka, Hirata, Komiya, Rino, Katayama, Motoki and Maruyama2005; Wang et al. Reference Wang, Campbell, Allen, Williams and Eggins2009, Reference Wang, Campbell, Stepanov, Allen and Burtsev2011; Yang et al. Reference Yang, Gao, Chen, Tang, Yuan, Gong, Xie and Wang2009, Reference Yang, Zhang and Wang2012; Safonova et al. Reference Safonova, Maruyama, Hirata, Kon and Rino2010; Alizai et al. Reference Alizai, Carter, Clift, Vanlaningham, Williams and Kumar2011; Choi et al. Reference Choi, Yong and Orihashi2016). The available data indicate that crustal growth is characterized by several discrete peaks at ∼2700, ∼1900, ∼1000 and ∼600 Ma, in response to the supercontinent assembly and break-up. Coupled zircon U–Pb age and Nd–Hf isotope data further suggest that 50 % and >80 % ofexisting continental crust was formed in the latest Archaean and in the Precambrian, respectively, and that less than 20 % of existing continental crust has been formed during the last 600 Ma (Condie et al. Reference Condie, Belousova, Griffin and Sircombe2009; Belousova et al. Reference Belousova, Kostitsyn, Griffin, Begg, O’Reilly and Pearson2010; Condie & Aster, Reference Condie and Aster2010; Iizuka et al. Reference Iizuka, Komiya, Rino, Maruyama and Hirata2010; Condie, Reference Condie2014). The Phanerozoic zircons generally show null to negative initial Hf isotopic values, implying that the Phanerozoic continental crust is characterized by remelting/reworking of pre-existing ancient continental crust or by crust–mantle interaction (Griffin et al. Reference Griffin, Wang, Jackson, Pearson, O’Reilly, Xu and Zhou2002; Belousova et al. Reference Belousova, Kostitsyn, Griffin, Begg, O’Reilly and Pearson2010).
The South China region has a complex tectonic evolution history, and consists of two major continental blocks: the Cathaysia Block to the southeast and the Yangtze Block to the northwest (Fig. 1). Based on the fact that the basement is unconformably overlain by Devonian sediments, Grabau (Reference Grabau1924) first proposed the term ‘Cathaysia old continent’, the ‘Cathaysia Block’ in current terminology. However, there has been no consensus regarding the age and distribution of the basement rocks, due to their poor exposures (e.g. Xu et al. Reference Xu, O’Reilly, Griffin, Wang, Pearson and He2007, Reference Xu, Wang and Zhao2016; Zheng et al. Reference Zheng, Griffin, Tang, Zhang, Su and Liu2008; Wang et al. Reference Wang, Zhang, Fan, Zhang, Chen, Cawood and Zhang2010). Up to now, Lüliangian, Grenvillian, Jinningian, Caledonian, Indosinian and Yanshanian rocks with Archaean detrital/inherited zircons have been documented in the Cathaysia Block (Gilder et al. Reference Gilder, Gill, Coe, Zhao, Liu, Wang, Yuan, Liu, Kuang and Wu1996; Chen & Jahn, Reference Chen and Jahn1998; Zhou & Li, Reference Zhou and Li2000; Sun, Reference Sun2006; Xu et al. Reference Xu, O’Reilly, Griffin, Wang, Pearson and He2007, Reference Xu, Wang and Zhao2016; Zheng & Zhang, Reference Zheng and Zhang2007; Zhou, Reference Zhou2007; Z Li et al. Reference Li, Li, Wartho, Clark, Li, Zhang and Bao2010; Wang et al. Reference Wang, Zhang, Fan, Zhang, Chen, Cawood and Zhang2010; XH Li et al. Reference Li, Li and He2012; Yu et al. Reference Yu, O’Reilly, Zhou, Griffin and Wang2012a; Zhang et al. Reference Zhang, Wang, Fan, Zhang and Yang2012; Zhao & Cawood, Reference Zhao and Cawood2012). Thus, the Cathaysia Block is a complex continental block that experienced multi-stage magmatic and metamorphic events. The formation and evolution history of the Cathaysia Block has been a highly controversial topic. The Ganjiang River, one of eight major tributaries of the Yangtze River, located in the western part of the Cathaysia hinterland, has a length of 823 km and a drainage area of 82 809 km2, which provides a valuable opportunity to explore the crustal growth and evolution history of the Cathaysia Block. In the present study, 389 concordia U–Pb age and trace element analytical spots and 201 Lu–Hf isotopic analytical spots of detrital zircons from the Ganjiang River have been carried out in order to trace sediment provenances of the river and characterize the continental crustal growth and evolution history of the Cathaysia Block.

Fig. 1. The main river system in the South China Block and the location of Ganjiang River (modified from Xu et al. Reference Xu, O’Reilly, Griffin, Wang, Pearson and He2007).
2. Geological setting and sample collection
The Ganjiang River is located in an area between the northern section of Nanling and the southern section of the middle reaches of the Yangtze River (Fig. 1). It is one of eight major tributaries of the Yangtze River, and is the largest river in Jiangxi Province, SE China, with a drainage area of 82 809 km2 and a length of 823 km (Cheng, Reference Cheng2003). It stems from Shiliaodong of Shicheng county, Ganzhou city, S Jiangxi, and ends at Wangjiangting of Yongxiu county, Jiujiang city, N Jiangxi, with an approximately south–north-trending extension. The Ganjiang River can be further divided into upper, middle and lower reaches with nodal points at Ganzhou city and Xin’gan county. The area from starting point to Ganzhou city belongs to the upper reach with a length of 312 km, and those from Ganzhou city to Xin’gan county and from Xin’gan county to the end point belong to the middle and lower reaches with lengths of 303 and 208 km, respectively. The Ganjiang River’s primary tributaries are the Xiangshui River, Lianshui River, Meijiang River, Taojiang River, Zhangshui River, Suichuanjiang River, Shushui River, Gujiang River, Heshui River, Wujiang River, Yuanshui River, Xiaojiang River and Jinjiang River (Fig. 2). Geologically, the Ganjiang flows through the Wuyi Mountains, Nanling Mountains, Ganjiang Fault, Shi-Hang belt and some important Meso-Cenozoic basins like Ganzhou Basin and Jitai Basin (Zhou & Li, Reference Zhou and Li2000; Sun, Reference Sun2006; Zhou, Reference Zhou2007). Thus, the above-mentioned geological bodies together with volcanic–sedimentary rocks, granites and metamorphic basement rocks whether exposed or not provide sediment provenances of the river.
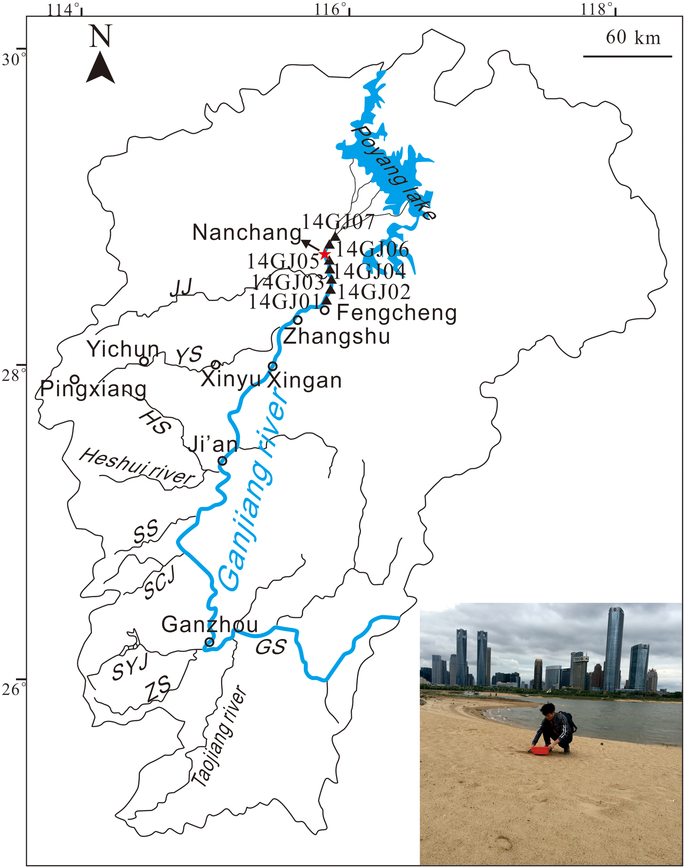
Fig. 2. Sketch map of the Ganjiang River and its tributaries. The abbreviations TJ, JJ, YS, HS, SS, SCJ, SYJ, ZS and GS represent Taojiang River, Jinjiang River, Yuanshui River, Hushui River, Shushui River, Suichuanjiang River, Shangyoujiang River, Zhangshui River and Gongshui River, respectively (modified from Cheng, Reference Cheng2003). The inset picture is a river sand sample collection site in Nanchang, the lower reach of the Ganjiang River.
The Cathaysia Block, which experienced multi-stage magmatic and metamorphic events, has a complex tectonic evolution history. Lüliangian (Li, Reference Li1997; Yu et al. Reference Yu, O’Reilly, Zhou, Griffin and Wang2012a; Liu et al. Reference Liu, Yu, O’Reilly, Zhou, Griffin, Wang and Cui2014), Grenvillian to Jinningian (Zhang et al. Reference Zhang, Wang, Fan, Zhang and Yang2012; Zhao & Cawood, Reference Zhao and Cawood2012), Caledonian (Z Li et al. Reference Li, Li, Wartho, Clark, Li, Zhang and Bao2010; Wang et al. Reference Wang, Zhang, Fan, Zhang, Chen, Cawood and Zhang2010), Indosinian to Hercynian (Li & Li, Reference Li and Li2007; Gao et al. Reference Gao, Wang and Li2017) and Yanshanian (Zhou & Li, Reference Zhou and Li2000; Sun, Reference Sun2006; Li & Li, Reference Li and Li2007; Huang et al. Reference Huang, Li, Li and Li2015) magmatic and/or metamorphic events together with Archaean detrital/inherited zircons (Xu et al. Reference Xu, O’Reilly, Griffin, Wang, Pearson and He2007, Reference Xu, Wang and Zhao2016; Zheng & Zhang, Reference Zheng and Zhang2007; Zheng et al. Reference Zheng, Griffin, Tang, Zhang, Su and Liu2008; Li et al. Reference Li, Li and He2012; Yu et al. Reference Yu, O’Reilly, Zhou, Griffin and Wang2012a) have been documented in the Cathaysia Block. However, their tectonic natures and geological implications are controversial. For example, the Mesozoic granites have been interpreted as a reactivation of pre-existing fault/rift systems or a link with flat-slab delamination and slab roll-back (Zhou et al. Reference Zhou, Sun, Shen, Shu and Niu2006; Li & Li, Reference Li and Li2007). The Caledonian granites have been considered to be associated with arc–continent collision or intraplate orogenesis (Guo et al. Reference Guo, Shi, Lu, Ma, Dong and Yang1989; Z Li et al. Reference Li, Li, Wartho, Clark, Li, Zhang and Bao2010). The Cathaysia Block is thought to be composed of a single unified basement or different Proterozoic basement rocks (Xu et al. Reference Xu, O’Reilly, Griffin, Wang, Pearson and He2007, Reference Xu, Wang and Zhao2016). Due to the uniqueness of detrital zircons from sediments and sedimentary rocks, many authors have carried out combined U–Pb and Lu–Hf isotopic analyses of detrital zircons to explore the crustal growth and evolution history of the Cathaysia Block. For example, Xiang & Shu (Reference Xiang and Shu2010) have determined 312 concordia U–Pb age spots of detrital zircons from Devonian and Ordovician coarse sandstones from Ganzhou, S China, to characterize the pre-Devonian tectonic evolution of the Cathaysia Block. Yao et al. (Reference Yao, Shu and Santosh2011) have obtained similar results on the basis of more zircon U–Pb age spots and extra Lu–Hf isotope analyses from the adjoining area. Yu et al. (Reference Yu, O’Reilly, Wang, Griffin, Zhang, Wang, Jiang and Shu2008) have carried out coupled U–Pb and Lu–Hf isotope analyses of detrital zircons from late Neoproterozoic sediments of the Cathaysia Block to constrain the location of the South China Block in the Rodinia supercontinent. Xu et al. (Reference Xu, O’Reilly, Griffin, Wang, Pearson and He2007) have conducted coupled U–Pb and Lu–Hf isotope analyses of detrital zircons from Oujiang and North River from eastern and western parts of the Cathaysia Block, while Xu et al. (Reference Xu, Wang and Zhao2016) have carried out coupled U–Pb and Lu–Hf isotopic determinations of detrital zircons from Minjiang and Zhujiang rivers from the eastern Cathaysia Block. He et al. (Reference He, Zheng and Jia2013) have analysed more than 100 U–Pb and Lu–Hf isotopic spots of zircons from the lower reach of the Ganjiang River to trace its sediment provenance. In the present study, seven river sand samples 14GJ01–07 collected from the lower reach of Ganjiang River have been conducted for coupled zircon U–Pb and Lu–Hf isotope analyses with the aim of tracing its sediment provenance and also depicting the crustal growth and evolution history of the Cathaysia Block (Fig. 1). About 10 kg of river sands for each sample were collected.
3. Analytical methods
Seven medium- to coarse-grained river sand samples were collected from the lower reach of the Ganjiang River. Detrital zircon grains were abstracted from each sample through standard procedures by crushing and sieving, followed by magnetic and heavy liquid separation and then hand-picking under a binocular microscope. More than 200 zircon grains of each sample were mounted in an epoxy disc. The mount was then cleaned and polished until all zircon grains were approximately cut in half. After that, all zircon grains were examined by transmitted and reflected light micrographs and cathodoluminescent (CL) imaging with the purpose of better selecting potential target locations for further mass spectrometry analyses. The CL imaging was undertaken at State Key Laboratory of Nuclear Resources and Environments, East China University of Technology (ECUT). CL image observations and mineral inclusion analyses by Raman spectra show that zircon grains from all seven river sand samples exhibit the same features. Thus we chose four samples, 14GJ01, 14GJ02, 14GJ04 and 14GJ07, for further zircon U–Pb and Lu–Hf isotope analyses. For ages younger and older than 1000 Ma, 206Pb/238U and 207Pb/206Pb ages were used respectively. Only ages with 95–105 % concordance were used for geological interpretation.
Zircon U–Pb dating was carried out at the School of Resources and Environmental Engineering, Hefei University of Technology, using laser ablation inductively coupled plasma mass spectrometry (LA-ICP-MS) technology. The LA-ICP-MS system consists of an Agilent 7500a ICP-MS coupled with a ComPex102 ArF-Excimer laser source with a wavelength of 193 nm. The details of LA-ICP-MS analytical procedure are described in Liu et al. (Reference Liu, Gao, Hu, Gao, Zong and Wang2010a, b). U–Pb dating and trace element analyses were determined synchronously by this technique. The obtained zircon U–Pb age and trace element data were reduced following Liu et al. (Reference Liu, Gao, Hu, Gao, Zong and Wang2010a, b) with the software ICPMSDataCal. Concordia diagrams and weighted mean calculations were made using Isoplot/Ex_brt3.00 (Ludwig, Reference Ludwig2003), and individual analyses in the data tables and concordia diagrams were presented with 1σ error, while the mean and intercepted ages were given with 2σ error (95 % confidence level). The zircon U–Pb isotope data and rare earth element (REE) contents are listed in Table S1 and Table S2, respectively, in the online Supplementary Material available at https://doi.org/10.1017/S001675681900116X.
Lu and Hf isotope analyses were conducted by a Neptune Plus multi-collector ICP-MS equipped with a Resolution M-50 laser-ablation system at the Laboratory of Geoanalysis and Geochronology, Tianjin Geological Survey Center, China Geological Survey (CGS). The Lu–Hf isotopic measurements were made on the same locations that were previously determined for U–Pb age spots. The beam diameter of the Lu–Hf isotopic spot is 45 μm, which is larger than the 32 μm beam diameter of the U–Pb isotopic spot. 173Yb and 175Lu were used to correct the isobaric interference of 176Yb and 176Lu on 176Hf. The Penglai zircon sample was used as the reference standard (XH Li et al. Reference Li, Long, Li, Liu, Zheng, Yang, Chamberlain, Wan, Guo, Wang and Tao2010). The detailed analytical procedures, instrumental parameters and data acquisition are described by Wu et al. (Reference Wu, Yang, Xie, Yang and Xu2006) and Yuan et al. (Reference Yuan, Gao, Dai, Zong, Günther, Fontaine, Liu and Diwu2008). During the analyses, the 176Hf/177Hf ratios of referenced Penglai zircon were 0.282907 ± 0.00016, which are in good agreement with the recommended 176Hf/177Hf values (0.282907 ± 0.00016) (XH Li et al. Reference Li, Long, Li, Liu, Zheng, Yang, Chamberlain, Wan, Guo, Wang and Tao2010). The main parameters used in the ε Hf(t) (t = crystallization time of zircon) and model age calculations are listed as follows: (176Lu/177Hf)CHUR = 0.0332 and (176Hf/177Hf)CHUR,0 = 0.282772 for chondritic uniform reservoir (Blichert-Toft et al. Reference Blichert-Toft, Gleason, Télouk and Albarède1997), (176Lu/177Hf)DM = 0.0384 and (176Hf/177Hf)DM = 0.28325 for depleted mantle reservoir (Griffin et al. Reference Griffin, Pearson, Belousova, Jackson, van Achterbergh, O’Reilly and Shee2000). 176Lu decay constant λ = 1.867 × 10−5 Ma−1 was used for calculations (Söderlund et al. Reference Söderlund, Patchett, Vervoort and Isachsen2004). The zircon Lu–Hf isotope data are listed in Table S3 in the online Supplementary Material available at https://doi.org/10.1017/S001675681900116X.
4. Results
4.a. Structure features and rare earth element contents of zircon
The representative zircon CL images are shown in Figure 3, and the zircon REE contents and chondrite-normalized REE distribution patterns are shown in supplementary Table S2 (available online at https://doi.org/10.1017/S001675681900116X) and Figure 4. The zircon grains are mostly dark grey, with minor ones being light white, and in shape they are mostly long columnar. The lengths of most zircon grains have a range between 100 and 250 μm, with length and width ratios varying from 2 to 4. According to the CL images, most zircon grains exhibit well-developed oscillatory zoning, suggesting that they are of igneous origin (Corfu et al. Reference Corfu, Hanchar, Hoskin and Kinny2003; Wu & Zheng, Reference Wu and Zheng2004) (Fig. 3). The poor psephicity of most zircon grains suggests that they have a relatively short transporting distance. In addition, minor zircon grains show core–rim structure, suggesting that they experienced multi-phase overgrowths, consistent with multi-stage tectonothermal events in the study area.

Fig. 3. Representative CL images of detrital zircons from the Ganjiang River. Solid small circles are U–Pb dating spots, and dotted large circles are Lu–Hf isotope analytical spots. Numbers in zircon grains represent the analytical spot numbers. The numbers below zircon grains represent ε Hf(t) values and zircon U–Pb ages.

Fig. 4. Chondrite-normalized REE distribution patterns of detrital zircons from the Ganjiang River. The normalization values of chondrite are from Sun & McDonough (Reference Sun, McDonough, Saunders and Norry1989).
Zircons of magmatic origin can be further constrained by the following data. Firstly, more than 90 % of zircon grains exhibit Th/U ratios larger than 0.4 (supplementary Table S1, available online at https://doi.org/10.1017/S001675681900116X). Previous results suggest that magmatic and metamorphic zircons generally have Th/U >0.4 and <0.1, respectively (Wu & Zheng, Reference Wu and Zheng2004). Secondly, as shown in Figure 4, most zircon grains display steep HREE (heavy-REE) patterns (mean HREE/LREE (light-REE) ratio = 103) and marked positive Ce and negative Eu anomalies (mean Ce* = 3.4, mean Eu* = 0.69) in the chondrite-normalized REE distribution patterns, indicating that they are magmatic zircons in origin (Wu & Zheng, Reference Wu and Zheng2004). All the above data indicate that most detrital zircons from Ganjiang River sands are of magmatic origin, with no marked later alteration, thus they can represent inherent information on their host rocks.
4.b. Zircon U–Pb dating results
Zircon U–Pb dating results of samples 14GJ01, 14GJ02, 14GJ04 and 14GJ07 are shown in supplementary Table S1 (available online at https://doi.org/10.1017/S001675681900116X) and Figures 5 and 6. Four hundred and twenty zircon U–Pb analytical spots, comprising 105 spots from sample 14GJ01, 105 spots from sample 14GJ02, 105 spots from sample 14GJ04, and 105 spots from sample 14GJ07, have been determined. Only 389 analytical spots with concordance of 95–105 % are listed in Table S1. There is no remarkable statistical difference in U–Pb ages between samples 14GJ01, 14GJ02, 14GJ04 and 14GJ07 (Fig. 5). Therefore, all 389 concordia zircon analytical spots are shown as a whole in Figure 6. They have a wide age range from 130 Ma to 3491 Ma, and can be further divided into seven age groups: (1) 130–185 Ma with a peak at 153 Ma, 7 %; (2) 217–379 Ma with a peak at 224 Ma, 16 %; (3) 390–494 Ma with a peak at 424 Ma, 37 %; (4) 500–698 Ma, 5 %; (5) 716–897 Ma with a peak at 812 Ma, 10 %; (6) 902–1191 Ma with a peak at 976 Ma, 13 %; (7) 2232–2614 Ma, 5 % (Fig. 5). Thus, detrital zircons from the Ganjiang River are dominated by Yanshanian, Indosinian to Hercynian, Caledonian, Pan-African, Jinningian, Grenvillian and Palaeoproterozoic–Archaean ages.

Fig. 5. The U–Pb concordia diagrams and age distribution histogram diagrams of detrital zircons from the Ganjiang River.

Fig. 6. All 389 concordia U–Pb age statistical histograms of detrital zircons from the Ganjiang River.
4.c. Zircon Lu–Hf isotope analyses
Zircon Lu–Hf isotope analytical results are listed in supplementary Table S3 (available online at https://doi.org/10.1017/S001675681900116X) and shown in Figure 7. Two hundred and one spots, comprising 50 spots from sample 14GJ01, 50 spots from sample 14GJ02, 50 spots from sample 14GJ04 and 51 spots from sample 14GJ07, have been determined. All determined zircons have 176Lu/177Hf ratios of 0.000003–0.002536 with a weighted value of 0.000824, most of which are smaller than 0.002 with the exception of spots 14GJ01-67, 14GJ02-46, 14GJ04-38 and 14GJ07-101, and display 176Hf/177Hf ratios of 0.280617–0.0282808 with a weighted value of 0.282140, suggesting that most zircon grains have relatively lower radiogenic Hf isotopic compositions. They have a wide ε Hf(t) range from −30.77 to +12.79, with most values being negative (26 spots with positive ε Hf(t) values and 175 spots with null to negative ε Hf(t) values), and TDM2 ages of 797 to 4016 Ma with a wide peak at 1500–2100 Ma and a keen peak at 1824 Ma (Fig. 7).

Fig. 7. (a) Hf-isotope evolution diagram and (b) Hf-isotope model age histogram diagram of detrital zircons from the Ganjiang River.
5. Discussion
5.a. Sediment provenance constraints from zircon U–Pb ages
Detrital zircon U–Pb geochronology from modern river sediments has been considered to be a vital method to decipher the material sources of fluvial sediments (e.g. Condie et al. Reference Condie, Belousova, Griffin and Sircombe2009; Belousova et al. Reference Belousova, Kostitsyn, Griffin, Begg, O’Reilly and Pearson2010; Yang et al. Reference Yang, Zhang and Wang2012). For the Ganjiang River, almost all zircon U–Pb age groups can be found in exposed rocks. In particular, Yanshanian 130–185 Ma plutons are widely distributed in the northern Guangdong – southern Jiangxi – western Fujian and central Jiangxi regions: for example, 172 Ma Zhaibei pluton, 165 Ma Quannan pluton and late Jurassic – early Cretaceous plutons of Wugongshan Dome compound granites (Zhou & Li, Reference Zhou and Li2000; Li et al. Reference Li, Chen, Liu and Li2003a; Lou et al. Reference Lou, Shen, Wang, Shu, Wu, Zhang and Yu2005; Sun, Reference Sun2006; Li & Li, Reference Li and Li2007; Zhou, Reference Zhou2007). Although Hercynian to Indosinian 217–379 Ma plutons are not distributed as widely as Yanshanian plutons in the Cathaysia Block, more and more plutons of this stage have been reported. For instance, Guo et al. (Reference Guo, Chen, Lin, Lou and Zeng2011) and Yu et al. (Reference Yu, Chen, Chen, Hou, Zhao, Xu, Zhang and Zeng2012b) have reported 252 Ma Keshuling granites and 227–229 Ma Qingxi plutons in southern Jiangxi, respectively. Luo et al. (Reference Luo, Wang, Zhang, Zhang and Zhang2010) and Zhou et al. (Reference Zhou, Xie, Sun, Guo, Zhou, Liu and Liu2015) have documented 222 Ma Jintan granites and 230–235 Ma Xiankou granites in central Jiangxi, respectively. Tao et al. (Reference Tao, Li, Wyman, Wang and Xu2018) have recently reported c.220 Ma Jintan pluton in central Jiangxi. The Hercynian to Indosinian zircons account for a relatively higher proportion of 16 %, which can be attributed to the fact that these plutons are close to the Ganjiang River. Caledonian 390–494 Ma rock bodies are widely distributed in the central Jiangxi and southern Jiangxi – northern Guangdong regions, which are considered to be related to early Palaeozoic Wuyi–Yunkai intracontinental orogen (Z Li et al. Reference Li, Li, Wartho, Clark, Li, Zhang and Bao2010). For example, 434 Ma Ninggang granite (Shen et al. Reference Shen, Zhang, Shu, Wang and Xiang2008), 428–473 Ma Wugongshan complex (Lou et al. Reference Lou, Shen, Wang, Shu, Wu, Zhang and Yu2005; Zhong et al. Reference Zhong, Ma, Liu, Zhao, Zheng, Nong and Zhang2014), 434–444 Ma Fufang granite (FF Zhang et al. Reference Zhang, Wang, Fan, Zhang and Zhang2010) and 428–440 Ma Zhangjiafang granite (Lou et al. Reference Lou, Shen, Wang, Shu, Wu, Zhang and Yu2005; FR Zhang et al. Reference Zhang, Shu, Wang, Shen, Yu and Xie2010) belong to this stage. Devonian to Ordovician sandstones from Chongyi, Suichuan, Jianggangshan and Taihe regions within southern and central Jiangxi could be another potential source of Caledonian detrital zircons (Xiang & Shu, Reference Xiang and Shu2010; Yao et al. Reference Yao, Shu and Santosh2011). It is noted here that Caledonian detrital zircons take up the highest proportion of 37 %. Pan-African 500–698 Ma rocks have not been found within the Cathaysia Block, but have been documented in the Ordovician sandstones from southern Jiangxi (Xiang & Shu, Reference Xiang and Shu2010; Yao et al. Reference Yao, Shu and Santosh2011), which is further confirmed by the data from crustal xenoliths from other areas (Li et al. Reference Li, Zheng, Xiong, Zhou and Xiang2018). This event implies that South China likely preserves the record of a major late Neoproterozoic – Cambrian orogeny and probably part of the Gondwana assembly. Jinningian 716–897 Ma with 10 %, which is related to the break-up of Rodinia supercontinent, is likely sourced from the Neoproterozoic Zhoutan Group in central Jiangxi (Li et al. Reference Li, Sun, Wang, Xing, Zhao, He, He and Zhang2011; Wang et al. Reference Wang, Yu, Shu, Tang and Xing2013), the Jiuling granite body in northern Jiangxi (Li et al. Reference Li, Li, Ge, Zhou, Li, Liu and Michanl2003b), and the Shuangqiaoshan Group in northern Jiangxi (XL Wang et al. Reference Wang, Yu, O’Reilly, Griffin, Sun, Wei, Jiang and Shu2008). Grenville-age meta-granitic, meta-volcanic and meta-sedimentary rocks in the northern Guangdong – southern Jiangxi regions including 972 Ma Jingnan meta-rhyolitic rocks, 0.9–1.0 Ga Hezi metamorphic basement rocks and 963–982 Ma Dawei and Longtang granitic gneisses can supply 902–1191 Ma detrital zircons, which indicates that South China is an essential part of the Rodinia supercontinent (Shu et al. Reference Shu, Deng, Yu, Wang and Jiang2008; LJ Wang et al. Reference Wang, Yu, O’Reilly, Griffin, Sun, Wei, Jiang and Shu2008; Yu et al. Reference Yu, O’Reilly, Wang, Griffin, Zhang, Wang, Jiang and Shu2008; Zhao & Cawood, Reference Zhao and Cawood2012; Cawood et al. Reference Cawood, Wang, Xu and Zhao2013; YJ Wang, Reference Wang, Zhang, Fan, Geng and Zou2014). Palaeoproterozoic–Archaean inherited and detrital zircons from meta-igneous and meta-sedimentary rocks from the northern Guangdong – southern Jiangxi – western Fujian region could supply detrital zircons of 2232–2614 Ma stage (Xu et al. Reference Xu, O’Reilly, Griffin, Deng and Pearson2005, Reference Xu, O’Reilly, Griffin, Wang, Pearson and He2007, Reference Xu, Wang and Zhao2016; Yu et al. Reference Yu, O’Reilly, Wang, Griffin, Jiang, Wang and Xu2007; Zheng & Zhang, Reference Zheng and Zhang2007; Yao et al. Reference Yao, Shu and Santosh2011).
The pre-Devonian zircon age distribution feature of our zircon age data is in good agreement with that of Xiang & Shu (Reference Xiang and Shu2010), who have determined detrital zircons from Devonian to Ordovician sandstones from southern and central Jiangxi. However, Yao et al. (Reference Yao, Shu and Santosh2011) have obtained an additional age peak of 1930–1520 Ma with a peak of 1700 Ma from Ordovician sandstones within southern Jiangxi, which does not show up in Xiang & Shu (Reference Xiang and Shu2010) and our study. Xu et al. (Reference Xu, O’Reilly, Griffin, Wang, Pearson and He2007) have only obtained Palaeoproterozoic detrital zircons from Oujiang River but not from North River. Xu et al. (Reference Xu, Wang and Zhao2016) have also obtained a 1.6–2.0 Ga age population of detrital zircons from Minjiang and Zhujiang rivers from the eastern Cathaysia Block. Although Palaeoproterozoic magmatic and metamorphic rocks/zircons have been reported in the NW Fujian – SW Zhejiang region (Li, Reference Li1997; Yu et al. Reference Yu, O’Reilly, Zhou, Griffin and Wang2012a; Liu et al. Reference Liu, Yu, O’Reilly, Zhou, Griffin, Wang and Cui2014), hardly any are captured in detrital zircons from the Ganjiang River and North River. A possible interpretation is that rocks of this stage are blocked by the Wuyi Mountains and cannot enter the Ganjiang River and North River. Considering that the 82 809 km2 Ganjiang River and 46 710 km2 North River have almost no Palaeoproterozoic detrital zircons, it is reasonable to assume that the Cathaysia Block could have different crustal growth and evolution histories and metamorphic basement rocks prior to the Mesozoic time, as suggested by Xu et al. (Reference Xu, O’Reilly, Griffin, Wang, Pearson and He2007).
5.b. Crustal growth and evolution of the Cathaysia Block revealed by Lu–Hf isotope
Zircon Lu–Hf isotope compositions, in combination with zircon U–Pb age spectra, can further offer information on the source characteristics (reworked vs juvenile crust). As shown in supplementary Table S3 (available online at https://doi.org/10.1017/S001675681900116X) and Figure 7, all the determined 201 zircon grains have a wide ε Hf(t) range from −30.8 to +12.8, of which 26 spots display positive ε Hf(t) values, and have TDM2ages of 797 to 4016 Ma with a wide peak at 1500–2100 Ma and a keen peak at 1824 Ma (Fig. 7). This implies that most zircon grains, especially Phanerozoic zircons, are sourced from the reworked ancient crustal materials or crust–mantle mixing (Griffin et al. Reference Griffin, Wang, Jackson, Pearson, O’Reilly, Xu and Zhou2002; Guo et al. Reference Guo, Fan, Li, Zhao, Li and Yang2012), which is further confirmed by the observation that positive zircon ε Hf(t) spots generally have zircon U–Pb age larger than 800 Ma. Our zircon Hf model ages are in agreement with whole-rock Nd model ages of the studied area (Chen & Jahn, Reference Chen and Jahn1998). In order to better distinguish reworked crust from juvenile crust, Zheng et al. (Reference Zheng, Wu, Zhao, Zhang, Xu and Wu2005) proposed that zircon Hf model ages can represent the periods of crustal growth only when the minimum zircon Hf model ages approach zircon U–Pb ages and the maximum zircon ε Hf(t) values are close to those of coeval depleted mantle. Considering the errors of instrument analyses, model calculations and decay constants, Belousova et al. (Reference Belousova, Kostitsyn, Griffin, Begg, O’Reilly and Pearson2010) defined that ‘zircons possessing ε Hf ≥ 0.75 times the ε Hf of the Depleted Mantle curve, which is equivalent to 75 % of the MORB [mid-ocean ridge basalt] range’, can be deciphered as ‘juvenile crust’. According to this view, only Grenvillian zircons can represent juvenile crust (Fig. 6). Analytical spot 14GJ01-31 has a zircon U–Pb age and an Hf model age of 1072 Ma and 1089 Ma with ε Hf(t) value of +12.79. Some researchers also have confirmed that Grenvillian ocean subduction and arc–continent collision took place in the Cathaysia Block, which could result in crustal growth (LJ Wang et al. Reference Wang, Yu, O’Reilly, Griffin, Sun, Wei, Jiang and Shu2008; Zhang et al. Reference Zhang, Wang, Fan, Zhang and Yang2012; Zhao & Cawood, Reference Zhao and Cawood2012; YJ Wang, Reference Wang, Zhang, Fan, Geng and Zou2014; Zhao, Reference Zhao2015). It is noted that the oldest 3491 Ma zircon has a ε Hf(t) value and Hf model age of +0.9 and 3628 Ma, indicating some juvenile crustal growth in the Palaeo-Archaean. Phanerozoic zircons are notable for negative ε Hf(t) values as well as a wide range of 10 ε Hf units, indicating that they stemmed from reworked ancient crustal materials with some degree of crust–mantle mixing. It is interesting that the present study exhibits strong Palaeo-Mesoproterozoic Hf model age peaks but few contemporaneous zircon U–Pb ages, implying that 1500–2100 Ma is an important crustal growth period of the Cathaysia Block, but rocks or zircons of this stage are mostly reworked during later geological events. Alternatively, the Palaeo-Mesoproterozoic Hf model ages represent a mixing between magmas derived from a juvenile source and from remelting of an ancient crustal source.
In order to better characterize the crustal growth as revealed by detrital zircons from the Ganjiang River, a crustal growth curve diagram is presented (Fig. 8). The results suggest that there is a rapid increase of zircon age cumulative probability from 15 % to 75 % during 400–1000 Ma, and that there is a rapid crustal growth cumulative probability from 20 % to 90 % during 1500–2100 Ma. The zircon U–Pb age curve is roughly parallel to the zircon Hf model age curve (Fig. 8), implying that there is a relatively long period of zircon recycling. The results also show that c. 90 % of the crust of the Cathaysia Block was formed before 1.5 Ga, as revealed by detrital zircons from the Ganjiang River. In summary, the current study confirms that combined detrital zircon U–Pb chronology and Lu–Hf isotope data can provide important insights into sediment provenance and crustal growth, which cannot be fully revealed by the study of exposed rocks.

Fig. 8. Cumulative probability curves of U–Pb ages and Hf model ages of detrital zircons from the Ganjiang River.
6. Conclusions
The following conclusions can be addressed:
(1) Four hundred and twenty concordia U–Pb ages of detrital zircons from the Ganjiang River can be divided into seven groups: 130–185 Ma with a peak at 153 Ma; 217–379 Ma with a peak at 224 Ma; 390–494 Ma with a peak at 424 Ma; 500–698 Ma with a peak at 624 Ma; 716–897 Ma with a peak at 812 Ma; 902–1191 Ma with a peak at 976 Ma; and 2232–2614 Ma with a peak at 2471 Ma.
(2) Seven zircon age groups can be sourced from exposed rocks. Yanshanian, Hercynian to Indosinian, Pan-African, Grenvillian and Archaean zircons could be mainly sourced from the northern Guangdong – southern Jiangxi – western Fujian region; Caledonian zircons mainly come from southern and central Jiangxi, and Jinningian zircons are mainly from central and northern Jiangxi.
(3) Most determined zircon grains display negative ε Hf(t) values and TDM2 ages of 797 to 4016 Ma with a wide peak at 1500–2100 Ma and a keen peak at 1824 Ma, suggesting that most zircons sourced from the reworked ancient crustal materials or crust–mantle mixing.
(4) About 90 % of the crust of the Cathaysia Block was formed before 1.5 Ga as revealed by combined U–Pb and Lu–Hf isotope analyses of detrital zircons from the Ganjiang River.
Supplementary material
To view supplementary material for this article, please visit https://doi.org/10.1017/S001675681900116X
Acknowledgements
This study was supported by the National Natural Science Foundation of China (41763005, 41303041), The National Key Research and Development Program of China (2018YFA0404104), fund from the Education Department of Jiangxi Province (GJJ14476) and funds from the State Key Laboratory Breeding Base of Nuclear Resources and Environments (Z1606, Z1608, NRE1401 and RGET1606).