Introduction
Cystic ovarian disease (COD) is among the most relevant causes of subfertility in dairy cattle (Garverick, Reference Garverick1997). This disease generates important economic losses to the dairy industry because it increases the calving-to-conception and inter-calving intervals and because, if the condition persists, the animals should be eliminated from the herd (Peter, Reference Peter2004; Cattaneo et al., Reference Cattaneo, Signorini, Bertoli, Bartolomé, Gareis, Díaz, Bo and Ortega2014). The incidence of this disease in dairy cattle varies from 5 to 30% (Garverick, Reference Garverick1997; Silvia et al., Reference Silvia, Alter, Nugent and Laranja da Fonseca2002, Reference Silvia, McGinnis and Hatler2005; Peter, Reference Peter2004; Vanholder et al., Reference Vanholder, Opsomer and de Kruif2006; Probo et al., Reference Probo, Comin, Mollo, Cairoli, Stradaioli and Veronesi2011; Rizzo et al., Reference Rizzo, Campanile, Mutinati, Minoia, Spedicato and Sciorsci2011; Cattaneo et al., Reference Cattaneo, Signorini, Bertoli, Bartolomé, Gareis, Díaz, Bo and Ortega2014; Ortega et al., Reference Ortega, Díaz, Salvetti, Hein, Marelli, Rodríguez, Stassi and Rey2016). COD is the result of several alterations in ovulatory mechanisms and follicular development (Ortega et al., Reference Ortega, Díaz, Salvetti, Hein, Marelli, Rodríguez, Stassi and Rey2016). Despite the causes, when ovulation does not occur, the dominant follicle becomes an anovulatory cystic structure, interfering with normal ovarian function (Peter, Reference Peter2004). Ovulation is considered an inflammatory event mediated by cytokines (Bukulmez and Arici, Reference Bukulmez and Arici2000; Richards et al., Reference Richards, Liu and Shimada2008; Oakley et al., Reference Oakley, Kim, El-Amouri, Lin, Cho, Bani-Ahmad and Ko2010; Richards and Pangas, Reference Richards and Pangas2010). Cytokines have immunoregulatory effects and are recognized as growth factors involved in many cellular processes in ovarian cells such as apoptosis, proliferation, differentiation, folliculogenesis, oogenesis, luteogenesis and hormone release (Orsi, Reference Orsi2008; Orsi and Tribe, Reference Orsi and Tribe2008; Field et al., Reference Field, Dasgupta, Cummings and Orsi2014). Cytokines are also important factors affecting fertility and, in some cases, involved in the development of different ovarian disorders, including COD (Sirotkin, Reference Sirotkin2011; Smolikova et al., Reference Smolikova, Mlynarcikova and Scsukova2012; Baravalle et al., Reference Baravalle, Stassi, Velázquez, Belotti, Rodríguez, Ortega and Salvetti2015).
Cytokines include interleukins (IL) such as IL-1, IL-4 and IL-8. The IL-1 family acts as one of the most important mediators of inflammation (Mu et al., Reference Mu, Liu, Wang, Wen, Wang, Yan, Zhou, Ma and Cao2010). The first reported and most studied ligands of the IL-1 family are IL-1α and IL-1β (Dinarello, Reference Dinarello2013). This family also includes two receptors, IL-1 receptor I (IL-1RI) and IL-1 receptor II (IL-1RII) (Sims et al., Reference Sims, Giri and Dower1994), and an IL-1 receptor antagonist (IL-1RA). This last protein acts as a ligand that blocks the action of IL-1 by binding to the receptors and inhibiting the signal by lack of the domain that interacts with IL-1 receptor accessory protein, which regulates IL-1 biological activity (Garlanda et al., Reference Garlanda, Dinarello and Mantovani2013; Akdis et al., Reference Akdis, Aab, Altunbulakli, Azkur, Costa and Crameri2016). Both IL-1α and IL-1β bind to IL-1RI with similar effects, and can even bind to IL-1RII. However, IL1RII does not generate signals because it lacks the intracellular domain, acting as decoy receptor (Dinarello, Reference Dinarello2013). IL-1 has been found to be biologically active in the follicular fluid (FF) of swine, horses and humans (Hurwitz et al., Reference Hurwitz, Loukides, Ricciarelli, Botero, Katz, McAllister, Garcia, Rohan, Adashi and Hernandez1992; Terranova and Rice, Reference Terranova and Rice1997). In addition, IL-1 participates in several ovulation-related processes such as stimulation of progesterone production (Dang et al., Reference Dang, Zhu, He, Wang, Lu, Li, Qi, Wu and Sun2017), prostaglandin and nitric oxide production by granulosa cells (reviewed by Field et al., Reference Field, Dasgupta, Cummings and Orsi2014), induction of gelatinases, and synthesis of proteases (Ellman et al., Reference Ellman, Corbett, Misko, McDaniel and Beckerman1993; Hurwitz et al., Reference Hurwitz, Dushnik, Solomon, Ben-Chetrit, Finci-Yeheskel, Milwidsky, Mayer, Adashi and Yagel1993).
IL-4 is extensively recognized for its anti-inflammatory properties but, depending on the tissue and stimuli, this cytokine may also have pro-inflammatory effects (Woods and Judd, Reference Woods and Judd2008). In the ovary, IL-4 is able to affect ovulation by interrupting the inflammatory process by suppressing IL-1β and tumour necrosis factor-α (TNF-α) production (Bonder et al., Reference Bonder, Dickensheets, Finlay-Jones, Donnelly and Hart1998). IL-4 plays its roles through the interaction with its receptor (IL-4Rα).
IL-8 is involved in a large number of ovarian processes including folliculogenesis, steroidogenesis and atresia, and is able to promote luteinization (Garcia-Velasco and Arici, Reference Garcia-Velasco and Arici1998; Bornstein et al., Reference Bornstein, Rutkowski and Vrezas2004). This cytokine is crucial in ovarian physiology and in many processes like apoptosis and proliferation of granulosa cells and ovarian angiogenesis (Koch et al., Reference Koch, Polverini, Kunkel, Harlow, DiPietro, Elner, Elner and Strieter1992; Ujioka et al., Reference Ujioka, Matsukawa, Tanaka, Matsuura, Yoshinaga and Okamura1998; Belayet et al., Reference Belayet, Kanayama, Khatun, Asahina, Okada, Kitamura, Kobayashi and Terao2000; Li et al., Reference Li, Varney, Valasek, Godfrey, Dave and Singh2005; Jiemtaweeboon et al., Reference Jiemtaweeboon, Shirasuna, Nitta, Kobayashi, Schuberth, Shimizu and Miyamoto2011; Ou et al., Reference Ou, Sun, Peng and Wu2016).
In a previous study, we found that the expression of IL-1α, IL-6 and TNF-α in bovine cystic follicles is altered, suggesting that the pathogenesis of COD may be related to an altered immune response (Baravalle et al., Reference Baravalle, Stassi, Velázquez, Belotti, Rodríguez, Ortega and Salvetti2015). In subsequent studies, using an experimental model, we were able to detect changes in a greater number of components of the immune system (Stassi et al., Reference Stassi, Baravalle, Belotti, Rey, Gareis, Díaz, Rodríguez, Leiva, Ortega and Salvetti2017; Reference Stassi, Baravalle, Belotti, Amweg, Angeli, Velázquez, Rey, Salvetti and Ortega2018). However, the results observed in an experimental model should be corroborated in animals with spontaneously developed disease. This will allow the validation of the model previously used and will provide valuable information. In addition, considering the large number of components of the immune system at reproductive level, we consider it important to expand the range of cytokines evaluated to have an integrated view. Based on all these data, and to verify the hypothesis that cytokines involved in the ovulatory process can contribute to the anovulation observed in COD and the consequent development of this disease, the aims of this study were: (i) to evaluate the protein expression of other components of the IL-1 system, including IL-1β, IL-1RA, IL-1RI, IL-1RII, as well as IL-4 and IL-8, in ovarian follicular structures from control cows and cows with spontaneous COD; (ii) to analyse the protein expression of the same cytokines through folliculogenesis in control cows and cows with spontaneous COD; and (iii) to determine the concentrations of IL-1β, IL-4 and IL-8 in FF and serum of control cows and cows with spontaneous COD.
Materials and Methods
Animals
Control Group
The control group consisted of 10 healthy multiparous Holstein cows (66.2 ± 25.4 months old; 2.8 ± 1.3 lactations) from local dairy farms. To obtain samples at proestrus, the estrous cycle was synchronized starting with the procedure commonly referred to as G6G (Bello et al., Reference Bello, Steibel and Pursley2006), with modifications (Díaz et al., Reference Díaz, Stangaferro, Gareis, Silvia, Matiller, Salvetti, Rey, Barberis, Cattaneo and Ortega2015). The synchronization protocol consisted of two doses of prostaglandin (PG) F2α (150 µg D+Cloprostenol; Enzaprost DC, Biogénesis Bagó, Garín, Buenos Aires, Argentina) administered 12 h apart on day 0 to induce luteolysis (Hatler et al., Reference Hatler, Hayes, Ray, Reames and Silvia2008), followed by a dose of a gonadotropin-releasing hormone (GnRH) analogue (20 µg Buserelin acetate; Gonaxal, Biogénesis Bagó, Argentina), administered 2 days later (i.e. day 2) to stimulate the ovulation of the preovulatory follicles present. Six days after this first GnRH dose (i.e. on day 8), the cows received a second dose of GnRH, and 7 days later (i.e. on day 15), cows received the last two doses of PGF2α, 12 h apart, to ensure luteolysis. The expected time of ovulation was about 4 days after the administration of the dose of PGF2α administered on day 15 of the protocol, i.e. approximately on day 19. For this reason, the samples were taken on day 18 of the proestrus stage. The size, growth and regression of all follicles > 5 mm, corpora lutea and dominant follicles were monitored by rectal palpation and ultrasonographic examinations using a real-time B-mode scanner equipped with a 5 MHz linear-array, transrectal transducer (Honda HS101V, Japan) (Amweg et al., Reference Amweg, Salvetti, Stangaferro, Paredes, Lara, Rodríguez and Ortega2013).
Spontaneous COD Group
The COD group consisted of 10 multiparous Holstein cows with COD (64.8 ± 24.9 months old; 3.3 ± 1.5 lactations) from local dairy farms. Cysts were detected in the routine reproductive examination of the cows, and subsequently confirmed by rectal palpation and ultrasonography, discarding other diseases and abnormalities in the reproductive system. Spontaneous follicular cysts were defined as follicular structures with ≥ 20 mm in diameter, persisting in the ovary in the absence of a corpus luteum, interrupting the estrous cycle and without uterine tone (Bartolomé et al., Reference Bartolomé, Thatcher, Melendez, Risco and Archbald2005; Vanholder et al., Reference Vanholder, Opsomer and de Kruif2006).
Tissue sampling and processing
Ovaries from the cows of both groups were obtained by bilateral ovariectomy (Amweg et al., Reference Amweg, Salvetti, Stangaferro, Paredes, Lara, Rodríguez and Ortega2013; Marelli et al., Reference Marelli, Díaz, Salvetti, Rey and Ortega2014). To prevent the rupture of dominant follicles from the control group and follicular cysts from the COD group during surgery, the FF was aspirated before ovariectomy, using a digital ultrasound system 8300vet Chison equipped with a micro-convex transducer of 5.0 MHz mounted on a transvaginal probe for follicular aspiration (Watanabe Applied Technology Limited, Brazil). The FF was collected in Falcon tubes, refrigerated on ice, and centrifuged at 2000 g for 10 min to obtain pellet cells in a centrifuge refrigerated at 4°C. Pellet cells were immediately frozen at −80°C. After pellet cell extraction, the FF was stored at −80°C until analysis.
After surgery, the ovaries were dissected and follicular structures were measured. Small samples from ovarian tissues were obtained and immediately frozen at −80°C until use for other evaluations. Ovaries were sectioned for an appropriate histological processing, fixed in 4% buffered formaldehyde at 25°C for 8–10 h, washed in phosphate buffered saline (PBS), dehydrated in an ascending series of ethanol, cleared in xylene, and finally embedded in paraffin. Sections of 4 μm thickness were mounted on slides previously treated with 3-aminopropyltriethoxysilane (Sigma-Aldrich, St. Louis, MO, USA), and then labelled with haematoxylin and eosin (Sigma-Aldrich) for a preliminary observation of all ovarian structures.
Blood samples from cows of both groups were collected via coccygeal venepuncture at the time of ovariectomy, to determine the concentrations of IL-1β, IL-4 and IL-8. Blood samples were kept at room temperature (22–25°C) for 30 min, placed at 4–8°C for 1 h and finally centrifuged at 400 g for 10 min. Serum was separated and stored at −80°C until analysis.
Follicular classification
Follicles were classified by the criteria established by Braw-Tal and Yossefi (Reference Braw-Tal and Yossefi1997) into the following categories: primary, small preantral, large preantral, antral, and atretic follicles. Additionally, we evaluated the dominant follicle (oestrogen active, composed of a complete healthy granulosa cell layer and an active theca interna) and follicular cysts (composed of a layer of granulosa cells of variable thickness and granulosa and theca cells without signs of luteinization) (Amweg et al., Reference Amweg, Salvetti, Stangaferro, Paredes, Lara, Rodríguez and Ortega2013). Only cystic follicles with a complete granulosa cell layer were used.
Immunohistochemistry(IHC)
Histological sections of ovaries from cows of both groups were used for IHC to locate and quantify the protein expression of IL-1β, IL-1RA, IL-1RI, IL-1RII, IL-4 and IL-8 in the follicular wall. The streptavidin–biotin immunoperoxidase method used has been previously described in Stassi et al. (Reference Stassi, Baravalle, Belotti, Rey, Gareis, Díaz, Rodríguez, Leiva, Ortega and Salvetti2017) and the antibodies used previously validated by western blot in bovine ovarian samples (Stassi et al., Reference Stassi, Baravalle, Belotti, Amweg, Angeli, Velázquez, Rey, Salvetti and Ortega2018). Homology between the target peptide of the antibody and the corresponding bovine protein was tested with Basic Local Alignment Search Tool (BLAST software; http://www.ncbi.nlm.nih.gov/BLAST) to determine the location of the peptide and to confirm antigen specificity. Homology was higher than 90% for all the antibodies.
Briefly, after deparaffinization and hydration, antigen retrieval was performed as detailed in Table 1. Endogen peroxidase activity was inhibited with 3% (vol/vol) H2O2 in methanol, and nonspecific binding was blocked with 10% (vol/vol) normal goat serum for all the antibodies except for IL-1RA and IL-4, which were blocked with normal donkey serum. All sections were incubated with the primary antibodies and detection was performed with the secondary biotin-conjugated antibodies (Table 1), which were incubated at 25°C for 30 min. The conditions of the IHC and the suppliers of the antibodies used are reported in Table 1. Antigens were visualized using the CytoScan™ HRP Detection System (Cell Marque, Rocklin, CA, USA), and 3,3-diaminobenzidine (Liquid DAB-Plus Substrate Kit; Zymed, San Francisco, CA, USA) was used as the chromogen. Finally, the slides were washed in distilled water, counterlabelled with haematoxylin, dehydrated, and mounted. To validate endogenous peroxidase activity blockade, some sections were incubated with DAB alone. Specificity was determined by replacing the primary antibodies with goat, rabbit and mouse non-immune sera. The specificity of the secondary antibodies was tested by incubation with the primary antibodies with negative reaction to bovine antigens: anti-human CD45 (clone: PD7/26; Dako, Carpinteria, CA, USA) and anti-human oestrogen receptor α (polyclonal; Cell Marque, Rocklin, CA, USA).
Table 1. Antigen retrieval and primary antibodies incubation conditions used for immunohistochemistry analysis

IL, interleukin; O.N., overnight; R, receptor; RA, receptor antagonist.
Image analysis
Microscopic images were digitized with a colour video camera Nikon DS-Fi2 mounted on a conventional light microscope Nikon Eclipse Ci-L Ni (Tokyo, Japan), with an objective magnification of ×40, and analysed with Image Pro-Plus 3.0.1 (Media Cybernetics, Silver Spring, MD, USA). The microscope was prepared for Koehler illumination. This was achieved by recording a reference image of an empty field for the correction of unequal illumination (shading correction) and calibrating the measurement system with a reference slide to determine background threshold values. The reference negative slides contained a series of tissue sections labelled in the absence of a primary antibody. The percentage of immunopositive area was calculated from the percentage of total area evaluated through colour segmentation analysis, which extracts objects by locating all objects of a specific colour (brown label). The positive controls were used as inter-assay controls to maximize the levels of accuracy and robustness of the method (Ranefall et al., Reference Ranefall, Wester, Andersson, Busch and Bengtsson1998; Ortega et al., Reference Ortega, Rey, Velázquez and Padmanabhan2010). The methodological details of image analysis as a valid method to quantify protein expression have been described previously (Salvetti et al., Reference Salvetti, Müller, Acosta, Gimeno and Ortega2007; Wang et al., Reference Wang, Zhou, Holmqvist, Zhang, Li, Adell and Sun2009; Ortega et al., Reference Ortega, Rey, Velázquez and Padmanabhan2010).
The percentage of the immunopositive-labelled area was calculated separately in each follicular wall layer (granulosa and theca interna) of the primary (only granulosa), small preantral (only granulosa), large preantral, antral, atretic, dominant and cystic follicles defined previously by the criteria established by Braw-Tal and Yossefi (Reference Braw-Tal and Yossefi1997) and Amweg et al. (Reference Amweg, Salvetti, Stangaferro, Paredes, Lara, Rodríguez and Ortega2013).
IL-1β, IL-4 and IL-8 determination in serum and follicular fluid by ELISA
IL-1β, IL-4 and IL-8 concentrations in serum and FF in control cows and cows with COD were quantified using commercial ELISA kits (bovine IL-1β and bovine IL-4 ELISA reagent kits from Thermo Scientific Inc., Rockford, USA, and bovine IL-8 ELISA kit from LifeSpan BioSciences, Inc., Washington, USA), following the manufacturer’s instructions. The microplates were sensitized overnight with coating antibody and blocked with a solution of 4% serine bovine albumin, 5% sucrose in D-PBS. All FF and serum samples were used undiluted. FF samples, serum, and standards were placed in duplicate in the wells of the plate and incubated at room temperature for 1 h for IL-1β or for 1.5 h for IL-4. The IL-8-coated plate was provided by the manufacturer. Standards and samples were incubated at 37°C for 2 h. Samples were washed and detection antibody (biotin-conjugated antibody) was added and incubated for 1 h. After appropriate washings, streptavidin conjugated to horseradish peroxidase was added and incubation was performed for 30 min. The reaction was evidenced by the addition of 3,3′,5,5′-tetramethylbenzidine (TMB) as chromogen solution (TMB substrate), which was incubated for 20 min for IL-1β and IL-4 or for 30 min for IL-8. The reaction was stopped with H2SO4 and read at 450 nm in an ELISA reader (Microplate Reader, SPECTROstarNano, BGM/LABTECH, Ortemberg, Germany). The detection limit was 7.8 pg/ml for IL-1β and IL-8 and 15.6 pg/ml for IL-4.
Statistical analysis
The statistical software package SPSS 15.0 for Windows (SPSS Inc., Chicago, IL, USA) was used in this study. The adequate number of images per follicle and the number of follicles per category were confirmed from a sample size calculation that evaluated the number of samples necessary to produce an estimate of the immunoexpression that would fall within 0.4 units of the real value. The appropriate number of images per follicle, depending on the category, was found to be: 15 representative images for cystic and dominant follicles and 10 representative images for antral follicles and all primary follicles (preantral small and large follicles), whereas the number of follicular structures per category was found to be: 1 cystic/dominant follicle per animal and 5–7 follicles of other categories per animal; in the latter, the average of the total number of follicles per animal was determined. Normal distribution was evaluated using the Kolmogorov–Smirnov test.
The data were evaluated to determine whether there were changes in the expression of the molecules evaluated between groups for each category and whether there were changes within each group throughout follicular development. This led us to perform the following statistical analysis: the percentages of immunopositive area in the control group and the animals with COD were compared in each follicular category separately by Student’s t-test to determine the differences between groups. The percentage of immunopositive area in different follicular categories along folliculogenesis was compared by analysis of variance (ANOVA) followed by Duncan multiple range tests separately in control and COD animals. In all cases, the data were analysed in each follicular wall layer (granulosa and theca interna). The results of the ELISA were analysed to determine differences between groups and between the levels in serum and FF within each group, using Student’s t-test. Differences were considered as significant when P-values were < 0.05. Results are expressed as mean ± standard deviation (SD).
Results
Follicular characterization
The health status of the dominant follicles, which was determined by assessing the 17β-estradiol/testosterone ratio in FF (Rodgers and Irving-Rodgers, Reference Rodgers and Irving-Rodgers2010), was >1 (Díaz et al., Reference Díaz, Stangaferro, Gareis, Silvia, Matiller, Salvetti, Rey, Barberis, Cattaneo and Ortega2015). In addition, hormonal concentration of spontaneous follicular cysts in FF was evaluated and has been published previously (Amweg et al., Reference Amweg, Salvetti, Stangaferro, Paredes, Lara, Rodríguez and Ortega2013; Baravalle et al., Reference Baravalle, Stassi, Velázquez, Belotti, Rodríguez, Ortega and Salvetti2015).
Expression and immunolocalization of cytokines
IHC allowed the detection and localization of expression of IL-1β, IL-1RA, IL-1RI, IL-1RII, IL-4 and IL-8 in different follicular structures (Figs 1, 2, 3, 4, 5 and 6 respectively). All negative controls showed negative reactions.
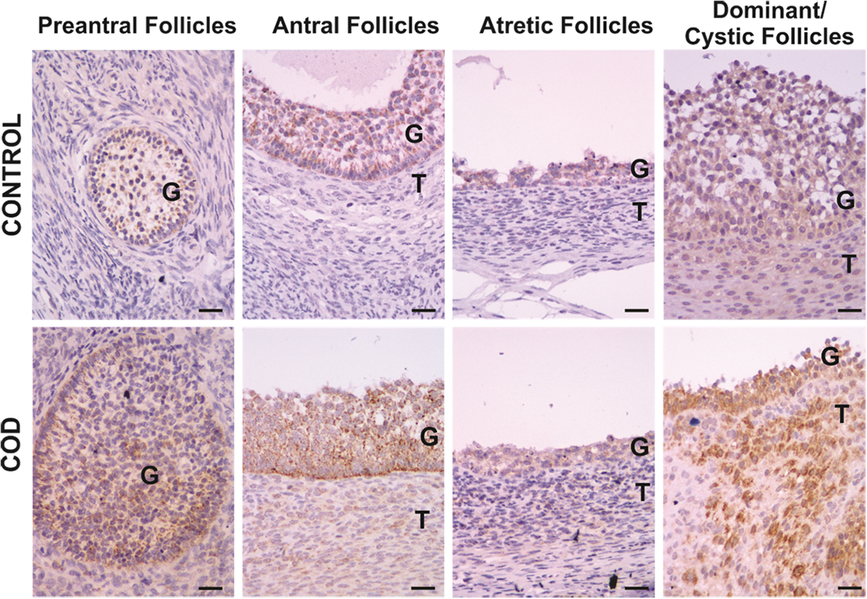
Figure 1. Representative images of IL-1β immunolabelling in primary, preantral, antral, atretic, dominant and cystic follicles from control ovaries and ovaries from cows with cystic ovarian disease (COD). T: theca cells, G: granulosa cells. Scale bar, 25 μm.
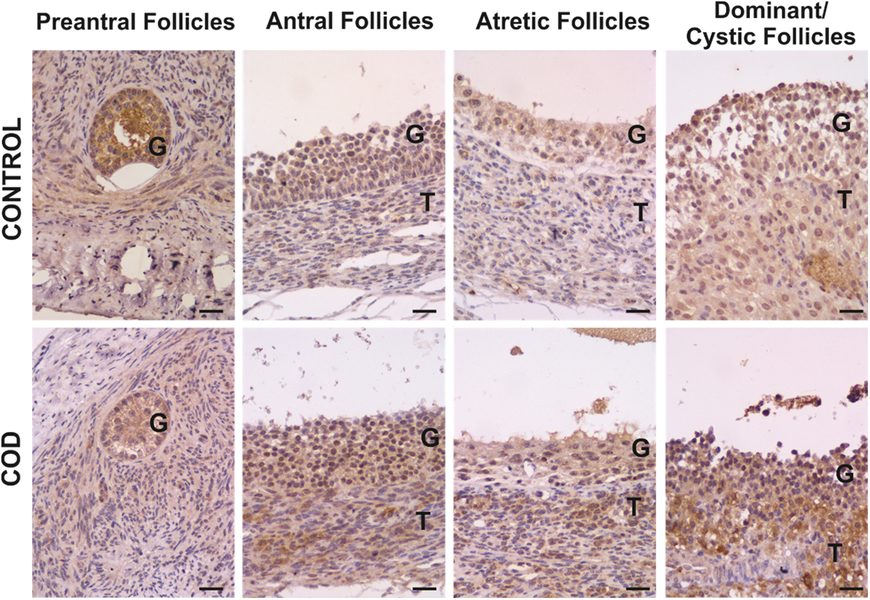
Figure 2. Representative images of IL-1RA immunolabelling in primary, preantral, antral, atretic, dominant and cystic follicles from control ovaries and ovaries from cows with cystic ovarian disease (COD). T: theca cells, G: granulosa cells. Scale bar, 25 μm.

Figure 3. Representative images of IL-1RI immunolabelling in primary, preantral, antral, atretic, dominant and cystic follicles from control ovaries and ovaries from cows with cystic ovarian disease (COD). T: theca cells, G: granulosa cells. Scale bar, 25 μm.

Figure 4. Representative images of IL-1RII immunolabelling in primary, preantral, antral, atretic, dominant and cystic follicles from control ovaries and ovaries from cows with cystic ovarian disease (COD). T: theca cells, G: granulosa cells. Scale bar, 25 μm.

Figure 5. Representative images of IL-4 immunolabelling in primary, preantral, antral, atretic, dominant and cystic follicles from control ovaries and ovaries from cows with cystic ovarian disease (COD). T: theca cells, G: granulosa cells. Scale bar, 25 μm.
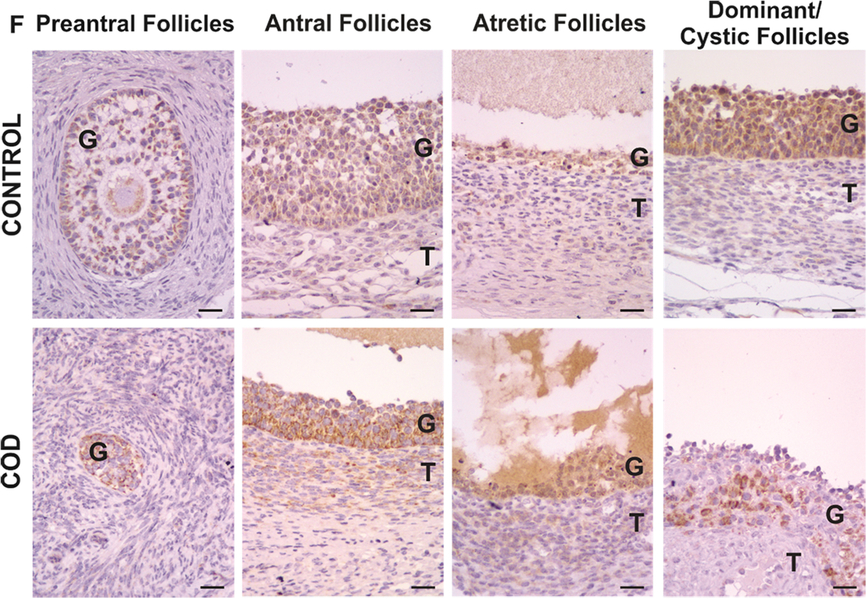
Figure 6. Representative images of IL-8 immunolabelling in primary, preantral, antral, atretic, dominant and cystic follicles from control ovaries and ovaries from cows with cystic ovarian disease (COD). T: theca cells, G: granulosa cells. Scale bar, 25 μm.
IL-1β
IL-1β expression was localized in the cytoplasm of granulosa and theca interna cells of all follicles analysed (Fig. 1). Along folliculogenesis, IL-1β expression in granulosa and theca cells was similar in control and COD groups (P > 0.05; Fig. 7). When each specific follicular structure was compared between groups, IL-1β expression in granulosa cells of small preantral follicles from control cows was lower than that in those from COD cows (P < 0.05; Fig. 8), and no significant differences were detected for the other follicular categories evaluated (P > 0.05; Fig. 8).

Figure 7. Relative protein expression (measured as percentage of immunopositive area) of IL-1β, IL-1RA, IL-1RI, IL-1RII, IL-4 and IL-8 in granulosa (open bars) and theca interna (black bars) cells of different follicular categories (control group) and cystic follicles (COD group) within each group. Values represent the mean ± standard deviation (SD). Bars with different letters (granulosa) or numbers (theca) are different (P < 0.05).

Figure 8. Relative protein expression (measured as percentage of immunopositive area) of IL-1β, IL-1RA, IL-1RI, IL-1RII, IL-4 and IL-8 in primary, small preantral, large preantral, antral, atretic and dominant follicle (DF) from control ovaries (open bars) and ovaries from cows with COD (black bars), both in granulosa and theca cells. Values represent the mean ± standard deviation (SD). *Values were significantly different at P < 0.05 as compared with the corresponding follicular category.
IL-1RA
IL-1RA expression was detected in the cytoplasm of granulosa and theca interna cells of all the follicles analysed (Fig. 2). Along folliculogenesis, in granulosa cells of the control group, IL-1RA expression was higher in large preantral follicles than in those of antral and atretic follicles, as well as higher in small preantral follicles than in atretic follicles (P < 0.04; Fig. 7). In theca cells, no differences were found between the different follicular categories evaluated (P > 0.05; Fig. 7). In the COD group, no differences were observed in granulosa cells but, in theca cells, IL-1RA expression was higher in cysts than in large preantral and atretic follicles (P < 0.01; Fig. 7).
Comparison of each specific follicular structure between groups showed that IL-1RA expression in granulosa cells was higher in cysts than in dominant follicles of the control group, as well as higher in the atretic follicles of the COD group than in those of the control group (P < 0.05; Fig. 8). In theca cells, IL-1RA expression was higher in cysts than in dominant follicles of the control group and lower in the antral follicles of the control group than in those of COD cows (P < 0.05; Fig. 8).
IL-1RI
IL-1RI expression was detected in the cytoplasm of granulosa and theca interna cells of all follicles analysed (Fig. 3). In the control animals, no differences were observed between the different follicular categories analysed during folliculogenesis in both granulosa and theca cells (P > 0.05; Fig. 7). In the COD group, IL-1RI expression in granulosa cells was lower in the primary and large preantral follicles than in cysts (P < 0.03; Fig. 7). In theca cells of the COD group, the expression was similar between the different follicular categories (P > 0.05; Fig. 7).
Instead, comparison between groups for equivalent follicular structures showed that IL-1RI expression was significantly higher in cysts than in dominant follicles of the control group, both in granulosa and theca interna cells (P < 0.05; Fig. 8).
IL-1RII
IL-1RII expression was detected in the cytoplasm of granulosa and theca interna cells of all the follicles analysed (Fig. 4). Along folliculogenesis, in granulosa cells of the control group, IL-1RII expression was higher in atretic follicles than in the rest of the follicular categories analysed and lower in antral follicles than in small preantral follicles (P < 0.01; Fig. 7). In the COD group, IL-1RII expression in granulosa cells was higher in cysts and atretic follicles than in preantral and antral follicles (P < 0.01; Fig. 7). In theca cells, no differences were found between the different follicular structures analysed in both groups (P > 0.05; Fig. 7).
When each specific follicular structure was compared between groups, IL-1RII expression was significantly higher in cysts than in dominant follicles of the control group, both in granulosa and theca interna cells (P < 0.01; Fig. 8).
IL-4
IL-4 expression was detected in the cytoplasm of granulosa and theca interna cells of all the follicles analysed (Fig. 5). Along folliculogenesis, in granulosa cells of the control group, IL-4 expression was higher in atretic follicles than in the rest of the follicular categories analysed (P < 0.03; Fig. 7). In the COD group, IL-4 expression in granulosa cells was higher in cysts and atretic follicles than in primary, preantral and antral follicles (P < 0.01; Fig. 7). In theca cells, no differences were detected between the different follicular categories evaluated in both groups (P > 0.05; Fig. 7).
Conversely, comparison between groups showed that IL-4 expression was significantly higher in cysts than in dominant follicles of the control group, both in granulosa and theca interna cells (P < 0.05; Fig. 8).
IL-8
IL-8 expression was detected in the cytoplasm of granulosa and theca interna cells of all the follicles analysed (Fig. 6). In the control group, no differences were observed between the different follicular categories during folliculogenesis, both at the level of granulosa and theca cells (P > 0.05; Fig. 7). Instead, in the COD group, IL-8 expression in granulosa cells was higher in antral and atretic follicles than in cysts and lower in primary follicles than in antral follicles (P < 0.05; Fig. 7). No significant differences were detected in theca cells between the different follicular categories analysed (P > 0.05; Fig. 7).
Comparison of each specific follicular structure between groups showed that IL-8 expression levels of dominant follicles from the control group and cystic follicles were similar (P > 0.05; Fig. 8). In theca cells of antral follicles, IL-8 expression was lower in the control group than in COD cows (P < 0.05; Fig. 8).
IL-1β, IL-8 and IL-4 concentrations in serum and follicular fluid
Comparison between groups
Analysis of serum and FF concentrations of IL-1β and IL-4 showed no significant differences between control and COD cows (P > 0.05; Fig. 9). The FF of the control group did not show values higher than the limit of detection of 7.8 pg/ml of IL-8, while the FF of the spontaneous COD group showed mean values of 14.6 pg/ml (Fig. 9). Therefore, the corresponding statistical analysis could not be performed for IL-8. Analysis of IL-8 serum concentrations showed no significant differences between control and COD cows.

Figure 9. IL-1β, IL-4 and IL-8 concentrations in serum and FF of dominant follicles from the estrous-synchronized control group and in cystic follicles from cows with COD. Values represent the mean ± standard deviation (SD). #IL-8 in FF of control animals was not detected (< to 7.8 pg/ml). *Values were significantly different at P < 0.05 for comparison of FF and serum within the same group.
Comparison within the same group
Comparison of IL-1β between FF and serum concentrations within the same group showed no significant differences between control and COD cows. In contrast, IL-4 levels were higher in FF than in serum in control and COD cows (P < 0.05; Fig. 9). The comparison of IL-8 between FF and serum concentrations showed no significant differences in COD cows.
Discussion
The cytokines evaluated in this study have been previously shown to be relevant in ovarian physiology. These cytokines are fundamental to reproductive events such as ovulation and follicular maturation (Brännström et al., Reference Brännström, Wang and Norman1993; Terranova and Rice, Reference Terranova and Rice1997; Bukulmez and Arici, Reference Bukulmez and Arici2000). In humans, a vicious cycle between pro- and anti-inflammatory cytokines is thought to play a role in the pathogenesis of polycystic ovary syndrome (PCOS) (Ebejer and Calleja-Agius, Reference Ebejer and Calleja-Agius2013; Rashid et al., Reference Rashid, Nigam, Saxena, Jain and Wajid2017).
It is well known that IL-1β plays a role in ovulation. In this study, IL-1β expression was similar in all follicular categories analysed, except in the small preantral follicles, in which it was lower in the granulosa of the control group than in that of the COD group. In a previous study in which we used an experimental model of induction of persistent follicles in cows, we determined similar differences: in granulosa cells, IL-1β expression was lower in the small preantral follicles of the control group than in those of groups for 0 and 15 days of follicular persistence (Stassi et al., Reference Stassi, Baravalle, Belotti, Amweg, Angeli, Velázquez, Rey, Salvetti and Ortega2018). These results indicated that both in COD and in the experimental model, IL-1β expression is slightly modified, and its role in ovulation could be due to the action of other proteins in this system, such as antagonists or receptors. Other studies have shown that, during follicular development, IL-1 regulates the transition of the granulosa and theca cells from a proliferative to a differentiated stage by favouring proliferation and preventing premature differentiation (Brännström, Reference Brännström, Leung and Adashi2004). An alternative way in which IL-1 acts on follicular development is by facilitating apoptosis, the primordial component of follicular atresia (Chun et al., Reference Chun, Eisenhauer, Kubo and Hsueh1995; Brännström, Reference Brännström, Leung and Adashi2004). This result suggests that IL-1 is involved in the determination of whether follicles progress to ovulation or atresia (Field et al., Reference Field, Dasgupta, Cummings and Orsi2014). These data highlight the relevance of the IL-1 system in the early stages of folliculogenesis. In the present study, the concentrations of IL-1β were low in both groups. This can be explained by the absence of any apparent disease in the animals included in this study. Also, no differences were found between the follicular cysts and the control group in FF or serum, a result that agrees with those found by the IHC technique and with the results previously shown in the experimental model (Stassi et al., Reference Stassi, Baravalle, Belotti, Amweg, Angeli, Velázquez, Rey, Salvetti and Ortega2018). The results here obtained are in agreement with those of Niu et al. (Reference Niu, Ye, Xia, Fengm and Zhang2017), who found similar concentrations of IL-1β and IL-4 in FF from women with PCOS compared with control patients.
An important component of the intraovarian IL-1 system is naturally occurring IL-1RA, which is responsible for attenuating the IL-1 ligand effects. Passos et al. (Reference Passos, Costa, da Cunha, Silva, Ribeiro, de Souza, Barroso, Dau, Saraiva, Gonçalves, van den Hurk and Silva2016) demonstrated the presence of this cytokine in all the structures of the bovine ovary, with low expression in theca cells. These results coincide with IL-1RA expression here observed throughout folliculogenesis in the control group but not with those observed in the COD group, in which IL-1RA expression throughout folliculogenesis was relatively constant. By intrafollicular injection of this cytokine in mares, Caillaud et al. (Reference Caillaud, Duchamp and Gérard2005) demonstrated in vivo that IL-1RA exerts actions in ovulation, inducing a time-dependent inhibition of hCG-induced ovulation. Therefore, taking into account that the ovulatory process is a cascade of inflammatory reactions and that the organism tries to prevent an exacerbated reaction, it may be suggested that IL-1RA acts in an autocrine/paracrine manner in the immediate vicinity of cells to minimize the inflammatory response generated by IL-1β (Rashid et al., Reference Rashid, Nigam, Saxena, Jain and Wajid2017). In addition, it has been shown that IL-1RA concentrations in peri-ovulatory human FF are relatively high (Büscher et al., Reference Büscher, Chen, Kentenich and Schmiady1999), and that the balance between the levels of IL-1RA and IL-1 in local tissues influences the relative physiological or pathophysiological effects of IL-1 (Arend, Reference Arend2002). Therefore, it is very likely that circulating IL-1RA diffuses into tissues and influences the local relationship between IL-1RA and IL-1. Our group has previously demonstrated an overexpression of IL-1α, the other agonist of the IL-1 system, in the granulosa of cysts and persistent follicles (Baravalle et al., Reference Baravalle, Stassi, Velázquez, Belotti, Rodríguez, Ortega and Salvetti2015; Stassi et al., Reference Stassi, Baravalle, Belotti, Rey, Gareis, Díaz, Rodríguez, Leiva, Ortega and Salvetti2017). This finding could explain the high expression of IL-1RA, to counteract IL-1, observed in follicular cysts both in the present work and in follicles of 15 days of persistence. This was studied in our previous work in relation to dominant follicles in the control group (Stassi et al., Reference Stassi, Baravalle, Belotti, Amweg, Angeli, Velázquez, Rey, Salvetti and Ortega2018), a situation that could interfere with the ovulatory process.
IL-1RI is the main effector of IL-1 and leads to unleashing of actions of this interleukin. In the present study, we found that IL-1RI immunoexpression was significantly higher in follicular cysts than in the dominant follicles of the control group, in both granulosa and theca interna cells. These results do not reflect those obtained in the experimental model of follicular persistence, in which expression of this receptor showed no differences (Stassi et al., Reference Stassi, Baravalle, Belotti, Amweg, Angeli, Velázquez, Rey, Salvetti and Ortega2018). Conversely, expression of IL-1RI was significantly lower in granulosa of follicles at the first stages of development than in cysts from the COD group, and was constant along folliculogenesis in the control group. Previous studies in rats have described IL-1RI as the main receptor, with high and constant expression during folliculogenesis, and low expression of IL-1RII (Scherzer et al., Reference Scherzer, Ruutiainen-Altman, Putowski, Kol, Adashi and Rohan1996; Brännström, Reference Brännström, Leung and Adashi2004).
In the present study, IL-1RII expression was significantly higher in cysts than in dominant follicles from the control group, in both granulosa and theca interna cells. IL-1RII, which as mentioned above acts as a decoy receptor, is secreted in a soluble form (Kuhn et al., Reference Kuhn, Marjaux, Imhof, De Strooper, Haass and Lichtenthaler2007; Lorenzen et al., Reference Lorenzen, Lokau, Düsterhöft, Trad, Garbers, Scheller, Rose-John and Grötzinger2012; Akdis et al., Reference Akdis, Aab, Altunbulakli, Azkur, Costa and Crameri2016). IL-1RII modulates the biological activity of IL-1 by several mechanisms by binding 100 times less efficiently to IL-1RA than agonists in the system. Conversely, IL-1RII acts to select ligands, therefore becoming a molecular trap for IL-1α and IL-1β (Colotta et al., Reference Colotta, Re, Muzio, Bertini, Polentarutti, Sironi, Giri, Dower, Sims and Mantovani1993; Re et al., Reference Re, Sironi, Muzio, Matteucci, Introna, Orlando, Penton-Rol, Dower, Sims, Colotta and Mantovani1996; Smith et al., Reference Smith, Hanna, Della Friend Moore, Chen, Farese, MacVittie, Virca and Sims2003; Garlanda et al., Reference Garlanda, Dinarello and Mantovani2013). The expression of IL-1RII is promoted by anti-inflammatory signals, including cytokines (IL-4, IL-13, IL-10 and IL-27), prostaglandins, glucocorticoid hormones and aspirin (Spriggs et al., Reference Spriggs, Nevens, Grabstein, Dower, Cosman, Armitage, McMahan and Sims1992; Colotta et al., Reference Colotta, Re, Muzio, Bertini, Polentarutti, Sironi, Giri, Dower, Sims and Mantovani1993, Reference Colotta, Re, Muzio, Polentarutti, Minty, Caput, Ferrara and Mantovani1994, Reference Colotta, Saccani, Giri, Dower, Sims, Introna and Mantovani1996; Re et al., Reference Re, Muzio, De Rossi, Polentarutti, Giri, Mantovani and Colotta1994; Dickensheets and Donnelly, Reference Dickensheets and Donnelly1997; Kalliolias et al., Reference Kalliolias, Gordon and Ivashkiv2010; Daun et al., Reference Daun, Ball, Burger and Cannon1999), indicating that IL-1RII expression is involved in the anti-inflammatory action of these regulators (Garlanda et al., Reference Garlanda, Dinarello and Mantovani2013; Bonecchi et al., Reference Bonecchi, Garlanda, Mantovani and Riva2016). In this study, IL-1RII expression in cystic follicles was increased, and could reflect the activation of endogenous negative regulation pathways of inflammation. In addition, the higher expression of IL-1RII detected in cysts is consistent with that observed in persistent follicles (Stassi et al., Reference Stassi, Baravalle, Belotti, Amweg, Angeli, Velázquez, Rey, Salvetti and Ortega2018). These results support even more the utility of this model induced by prolonged treatment with progesterone in the study of cystogenesis. Through folliculogenesis, expression of IL-1RII in the granulosa cells of the control group was higher in atretic follicles than in other categories, and behaved in the same way in the COD group, showing that expression in cysts is similar to that in atretic follicles. These results could indicate that IL-1RII is responsible for blocking the action of IL-1 in the proliferation of granulosa cells.
These results showed that the complex regulation of the IL-1 system could be unbalanced by an alteration in any of its components. Overexpression of IL-1RI, IL-1RII and IL-1RA in spontaneous follicular cysts is clear evidence that the IL-1 system is unbalanced. The ligand IL-1β showed no differences, but the receptor responsible for its action could be responsible for an exacerbated action of this cytokine, which as known is involved in proliferation, differentiation and apoptosis (Chun et al., Reference Chun, Eisenhauer, Kubo and Hsueh1995; Brännström, Reference Brännström, Leung and Adashi2004). It has been previously confirmed that cystic follicles of animals with spontaneous COD present an altered proliferation/apoptosis balance (Salvetti et al., Reference Salvetti, Stangaferro, Palomar, Alfaro, Rey, Gimeno and Ortega2010). Such unusual circumstances favour the persistence of these structures, which continue to secrete altered levels of cytokines, growth factors and hormones, and therefore contribute to COD pathogenesis.
Regarding IL-4, its expression in granulosa and theca interna cells of the dominant follicles from the control group was lower than in cystic follicles. These results coincide with those observed from the follicles of a progesterone-induced persistence model (Stassi et al., Reference Stassi, Baravalle, Belotti, Amweg, Angeli, Velázquez, Rey, Salvetti and Ortega2018). Through studies in rats, Leo et al. (Reference Leo, Pisarska and Hsueh2001) showed that expression of IL-4 and its receptor in preovulatory follicles is increased. In the present study, throughout folliculogenesis, IL-4 expression in the granulosa cells of the control group was higher in atretic follicles than in the other follicular categories. This result could indicate a clear participation of IL-4 in regulation of proliferation of granulosa cells. In the COD group, IL-4 behaved in the same way, as its expression in cysts was similar to that in atretic follicles. In addition, it has been demonstrated that IL-4 stimulates the expression and secretion of IL-1RII, which, as already mentioned, is a decoy receptor that captures IL-1 (Colotta et al., Reference Colotta, Re, Muzio, Bertini, Polentarutti, Sironi, Giri, Dower, Sims and Mantovani1993). Because the expression of both IL-1RII and IL-4 was higher in atretic follicles and cysts, we could suggest a co-stimulation in the secretion of these cytokines. These results confirm those of Dembic (Reference Dembic2015), who demonstrated that IL-4 has a direct anti-inflammatory effect in vitro by suppressing the secretion of IL-1β, IL-12, IL-10 and TNF-α by monocytes as well as that of IL -1β and IL-12 by macrophages. In studies performed in vivo, it has been shown that, when IL-4 is administered locally or systemically, this cytokine has a strong anti-angiogenic activity (Volpert et al., Reference Volpert, Fong, Koch, Peterson, Waltenbaugh, Tepper and Bouck1998), a process found altered in cystic follicles (Isobe et al., Reference Isobe, Kitabayashi and Yoshimura2005, Reference Isobe, Kitabayashi and Yoshimura2008).
IL-4 concentrations in FF and serum showed no differences between the COD and control groups, but were higher in FF than in serum in both groups. IL-4 concentration was also higher in FF of persistent follicles, similar to that observed in spontaneous COD (Stassi et al., Reference Stassi, Baravalle, Belotti, Amweg, Angeli, Velázquez, Rey, Salvetti and Ortega2018). These results highlight the fact that this cytokine is regulated locally at the follicular level. Because cytokines carry out the autocrine and paracrine regulation in cells, it is evident that the higher concentrations of these molecules in FF than in serum demonstrate their relationship with ovarian physiology. Therefore, determining the intrafollicular concentrations of cytokines is of special interest in the study of their role in ovarian functions. Our results coincide with those of Gaafar et al. (Reference Gaafar, Hanna, Hammady, Amr, Osman, Nasef and Osman2014), who evaluated cytokine concentrations in FF from women with PCOS and concluded that these concentrations showed no association with PCOS.
Finally, IL-8 expression showed few alterations. IL-8 expression was higher in the theca interna of antral follicles of the COD group than in those of the control group. Considering our previous results in a progesterone-induced experimental model (Stassi et al., Reference Stassi, Baravalle, Belotti, Rey, Gareis, Díaz, Rodríguez, Leiva, Ortega and Salvetti2017), we may suppose that IL-8 participates in the pathogenesis of follicular persistence by contributing to the arrest of follicular development, interfering with folliculogenesis. However, in this study, we did not observe that difference in spontaneous COD. Beyond this, IL-8 is thought to be a potent mediator of the ovulatory process through the stimulation of neutrophil chemotaxis and is associated with luteinization of granulosa and theca cells before ovulation in cattle (Shimizu et al. Reference Shimizu, Kaji, Murayama, Magata, Shirasuna, Wakamiya, Okuda and Miyamoto2012, Reference Shimizu, Imamura, Magata, Murayama and Miyamoto2013). In the present study, the expression of this cytokine in the control group was constant throughout folliculogenesis, whereas that in the COD group was higher in the granulosa cells of antral follicles than in primary and cystic follicles. These results suggested that IL-8 could play a role in the regulation of angiogenesis and cell proliferation, as previously reported by other authors (Koch et al., Reference Koch, Polverini, Kunkel, Harlow, DiPietro, Elner, Elner and Strieter1992; Schadendorf et al., Reference Schadendorf, Moller, Algermissen, Worm, Sticherling and Czarnetzki1993). An excess of IL-8 may lead to a persistent invasion of neutrophils, and this may in turn impair folliculogenesis and the ovarian reserve (Kitaya and Yamada, Reference Kitaya and Yamada2011). In the present study, in FF from the control group, IL-8 concentration was too low to be measured, whereas, in FF from spontaneous cysts, IL-8 concentration was measurable in all samples. The increased expression of IL-8 in antral follicles of COD cows and the concentration found in FF of cysts could be responsible for pronounced vasculature development, previously described in follicular cysts (Isobe et al., Reference Isobe, Kitabayashi and Yoshimura2005, Reference Isobe, Kitabayashi and Yoshimura2008).
In summary, cytokines are differentially expressed during folliculogenesis, suggesting the possible influence of cytokines on follicular maturation (De et al., Reference De, Sanford and Wood1992, Deshpande et al., Reference Deshpande, Chang, Chapman and Michael2000). All cytokines studied were found to be expressed in granulosa and theca cells of follicles at all the stages of development evaluated, in both groups. These findings suggest that variations observed occur from the first stages of follicular development, and could be involved in the difficulty of resuming normal cycles that could be related to the recurrence of COD (Silvia et al., Reference Silvia, Alter, Nugent and Laranja da Fonseca2002; Vanholder et al., Reference Vanholder, Opsomer and de Kruif2006). Our results also showed an altered expression of IL-1RI, IL-1RII, IL-1RA and IL-4, particularly high in follicular cysts of cows with spontaneous COD, demonstrating that the regulation of this intercellular communication is altered. In this sense, the increased expression of IL-1RA and IL-1RII could interfere in the participation of IL-1 in ovulation. Conversely, IL-1RI overexpression might favour the action of this cytokine. Previous results also showed that IL-1α expression is significantly higher in the granulosa of cysts than in the granulosa of antral follicles of control cows (Baravalle et al., Reference Baravalle, Stassi, Velázquez, Belotti, Rodríguez, Ortega and Salvetti2015). Taking into account that both ligands bind to the same receptors, we can hypothesise that, in ovaries from animals with spontaneous COD, the IL-1 system is exacerbated. Conversely, the activity of IL-4 added to other anti-inflammatory cytokines, such as TGF-β1 and IL-6 whose levels were increased in cows with COD, could contribute to blocking ovulation (Matiller et al., Reference Matiller, Stangaferro, Díaz, Ortega, Rey, Huber and Salvetti2014; Baravalle et al., Reference Baravalle, Stassi, Velázquez, Belotti, Rodríguez, Ortega and Salvetti2015). In addition, this study supports our previous results (Stassi et al., Reference Stassi, Baravalle, Belotti, Rey, Gareis, Díaz, Rodríguez, Leiva, Ortega and Salvetti2017; Reference Stassi, Baravalle, Belotti, Amweg, Angeli, Velázquez, Rey, Salvetti and Ortega2018) and provides evidence that cytokine expression in spontaneous COD and follicular persistence induced by prolonged treatment with progesterone in dairy cows is concurrent. These results suggested that this model represents a scenario compatible with spontaneous disease, with the advantage that early stages of disease development can be evaluated. The results allowed us to assume that the cytokines here studied are involved in anovulation and follicular persistence processes associated with COD pathogenesis. However, further studies should be performed to expose the complexities of COD in cattle and clarify the action of cytokines in this disease, and therefore help to develop new preventive or therapeutic strategies.
Acknowledgements
We are grateful to the staff of the Large Animal Health Hospital of the Facultad de Ciencias Veterinarias de la Universidad Nacional del Litoral (Santa Fe, Argentina) for animal care and help with the generation of experimental animals and collection of samples. We also thank DVM Jose Bertoli and DVM Fabian Barberis for assistance with animal care, the staff members of the Laboratorio de Biología Celular y Molecular Aplicada (ICIVET-Litoral UNL CONICET) for their technical support during processing of slides and Biogénesis Bagó for provision of drugs. VM, FR, HHO, NRS and MEB are Research Career Members and AFS, NCG, BEM, CJML are fellows of the National Scientific Research Council (CONICET, Argentina).
Financial support
This study was supported by a grant from the Argentine National Agency for the Promotion of Science and Technology (ANPCyT) (PICT 2011–1274).
Conflict of interest
The authors declare that there is no conflict of interest that could be perceived as prejudicing the impartiality of the research reported.
Ethical standards
All procedures were evaluated and approved by the Institutional Ethics and Security Committee of the Facultad de Ciencias Veterinarias – Universidad Nacional del Litoral, Santa Fe, Argentina (protocol number: 84/11) and are consistent with the Guide for the Care and Use of Agricultural Animals in Research and Teaching, 3rd edition (Federation of Animal Science Societies, 2010).