Introduction
Myxosporeans (Cnidaria, Myxozoa) are highly-reduced, obligate cnidarian parasites that mainly infect aquatic animals (Lom and Dyková, Reference Lom and Dyková2006; Feng et al., Reference Feng, Xiong, Zhang, Yang, Yao, Zhou and Miao2014). Known life cycles typically involve a myxospore stage that develops in fish, and an actinospore stage that develops in annelid worms (see Eszterbauer et al., Reference Eszterbauer, Atkinson, Diamant, Morris, El-Matbouli, Hartikainen, Okamura, Gruhl and Bartholomew2015). Horizontal transmission between these hosts is achieved through the production of two distinct types of spores – myxospores and actinospores. Myxospores are produced in the fish, being shed into the aquatic environment via urine, feces, cyst rupture, or after death of the host. Following release, they spread in the water column to encounter a viable annelid host, and begin a second developmental stage that culminates with the production and release of actinospores (Kent et al., Reference Kent, Whitaker and Dawe1997, Reference Kent, Andree, Bartholomew, El-Matbouli, Desser, Devlin, Feist, Hedrick, Hoffmann, Khattra, Hallett, Lester, Longshaw, Palenzeula, Siddall and Xiao2001; Hallett and Bartholomew, Reference Hallett, Bartholomew, Woo and Buckmann2011; Fontes et al., Reference Fontes, Hallett, Mo, Okamura, Gruhl and Bartholomew2015). Upon contact with water, the valves of the actinospores inflate, so that they become buoyant and drift passively in the water column to encounter a susceptible fish host, thus giving continuity to the life cycle (Kent et al., Reference Kent, Andree, Bartholomew, El-Matbouli, Desser, Devlin, Feist, Hedrick, Hoffmann, Khattra, Hallett, Lester, Longshaw, Palenzeula, Siddall and Xiao2001; Lom and Dyková, Reference Lom and Dyková2006).
To date, only ca. 60 myxosporean life cycles have been clarified (Eszterbauer et al., Reference Eszterbauer, Atkinson, Diamant, Morris, El-Matbouli, Hartikainen, Okamura, Gruhl and Bartholomew2015). Overall, the information acquired so far demonstrates that there is no obvious correlation between myxospore and actinospore morphotypes. For example, species of the genus Myxobolus Bütschli, 1882 (Bivalvulida, Myxobolidae) have been associated with aurantiactinomyxon, triactinomyxon and raabeia [including former echinactinomyxon (Rocha et al., Reference Rocha, Alves, Fernandes, Antunes, Azevedo and Casal2019b)] counterparts (Eszterbauer et al., Reference Eszterbauer, Atkinson, Diamant, Morris, El-Matbouli, Hartikainen, Okamura, Gruhl and Bartholomew2015; Rocha et al., Reference Rocha, Rangel, Castro, Severino, Azevedo, Santos and Casal2019c). In fact, phylogenetic analyses show the clade of myxobolids comprising most of the actinospore morphotypes represented in the NCBI database, namely antonactinomyxon, aurantiactinomyxon, helioactinomyxon, hexactinomyxon, hungactinomyxon, neoactinomyxum, raabeia, sphaeractinomyxon, seisactinomyxon, synactinomyxon and triactinomyxon (Hallett et al., Reference Hallett, Erséus and Lester1999; Kent et al., Reference Kent, Andree, Bartholomew, El-Matbouli, Desser, Devlin, Feist, Hedrick, Hoffmann, Khattra, Hallett, Lester, Longshaw, Palenzeula, Siddall and Xiao2001; Holzer et al., Reference Holzer, Sommerville and Wootten2004; Eszterbauer et al., Reference Eszterbauer, Marton, Rácz, Letenyei and Molnár2006; Milanin et al., Reference Milanin, Atkinson, Silva, Alves, Maia and Adriano2017; Rangel et al., Reference Rangel, Rocha, Casal, Castro, Severino, Azevedo, Cavaleiro and Santos2017; Rocha et al., Reference Rocha, Rangel, Castro, Severino, Azevedo, Santos and Casal2019c). Types of helioactinomyxon, hexactinomyxon, hungactinomyxon, sphaeractinomyxon, seisactinomyxon and synactinomyxon, however, have never been linked directly to the life cycle of any given species. Recently, the members of the sphaeractinomyxon collective group were hypothesized to play a role in the life cycle of mugiliform-infecting myxobolids based on phylogenetic data (Rocha et al., Reference Rocha, Rangel, Castro, Severino, Azevedo, Santos and Casal2019c).
The majority of known life cycles involve freshwater species that use oligochaetes as invertebrate definitive hosts (see Eszterbauer et al., Reference Eszterbauer, Atkinson, Diamant, Morris, El-Matbouli, Hartikainen, Okamura, Gruhl and Bartholomew2015). In marine environments, polychaetes have been suggested to be the hosts of choice (Køie et al., Reference Køie, Whipps and Kent2004, Reference Køie, Karlsbakk and Nylund2007, Reference Køie, Karlsbakk and Nylund2008; Rangel et al., Reference Rangel, Santos, Cech and Székely2009, Reference Rangel, Cech, Székely and Santos2011, Reference Rangel, Castro, Rocha, Severino, Casal, Azevedo, Cavaleiro and Santos2016a; Karlsbakk and Køie, Reference Karlsbakk and Køie2012). Few exceptions are known to occur in estuarine waters: the triactinomyxon stages of Ortholinea auratae Rangel et al., Reference Rangel, Rocha, Castro, Severino, Casal, Azevedo, Cavaleiro and Santos2015 and Ortholinea labracis Rangel et al., Reference Rangel, Rocha, Casal, Castro, Severino, Azevedo, Cavaleiro and Santos2017, as well as the aurantiactinomyxon stage of Paramyxidium giardi (Cépède, 1906) Freeman and Kristmundsson, 2018, were reported to develop in marine oligochaetes (Rangel et al., Reference Rangel, Rocha, Castro, Severino, Casal, Azevedo, Cavaleiro and Santos2015, Reference Rangel, Rocha, Casal, Castro, Severino, Azevedo, Cavaleiro and Santos2017; Rocha et al., Reference Rocha, Alves, Antunes, Azevedo and Casal2019a); while the tetractinomyxon stages of Ceratonova shasta Noble, 1950 and Parvicapsula minibicornis Kent et al., Reference Kent, Whitaker and Dawe1997 were reported to use the freshwater polychaete Manayunkia occidentalis Atkinson et al., Reference Atkinson, Bartholomew and Rouse2020 as invertebrate host (Bartholomew et al., Reference Bartholomew, Whipple, Stevens and Fryer1997, Reference Bartholomew, Atkinson and Hallett2006; Atkinson et al., Reference Atkinson, Bartholomew and Rouse2020).
In the case of sphaeractinomyxon, 32 types (including former tetraspora and endocapsa representatives) have been reported from either freshwater or marine oligochaetes (Marques, Reference Marques1984; Hallett et al., Reference Hallett, Erséus, Lester and Morton1997, Reference Hallett, O'Donoghue and Lester1998, Reference Hallett, Erséus and Lester1999, Reference Hallett, Erséus, O'Donoghue and Lester2001; Hallett and Lester, Reference Hallett and Lester1999; Rangel et al., Reference Rangel, Castro, Rocha, Cech, Casal, Azevedo, Székely, Cavaleiro and Santos2016b; Rocha et al., Reference Rocha, Rangel, Castro, Severino, Azevedo, Santos and Casal2019c, Reference Rocha, Alves, Antunes, Fernandes, Azevedo and Casal2020). In fact, more than half of these types were described from Portuguese estuaries, mainly from infections in the marine oligochaetes Limnodriloides agnes Hrabě, 1967 (Oligochaeta, Naididae), Tubificoides pseudogaster (Dahl, 1960) (Oligochaeta, Naididae) and Tubificoides insularis (Stephenson, 1923) (Rangel et al., Reference Rangel, Castro, Rocha, Cech, Casal, Azevedo, Székely, Cavaleiro and Santos2016b; Rocha et al., Reference Rocha, Rangel, Castro, Severino, Azevedo, Santos and Casal2019c, Reference Rocha, Alves, Antunes, Fernandes, Azevedo and Casal2020). Four types were reported to occur in freshwater, infecting Psammoryctides barbatus (Grube, 1861) (Oligochaeta, Naididae), and an unidentified species of the genus Potamothrix Vejdovský and Mrázek, 1903 (Oligochaeta, Naididae); this included one type that was also molecularly shown to occur in the marine oligochaete T. pseudogaster (Rocha et al., Reference Rocha, Alves, Antunes, Fernandes, Azevedo and Casal2020). The broader environmental range of this type was proposed to correlate with the biological features of the vertebrate host, namely its migratory patterns (Rocha et al., Reference Rocha, Alves, Antunes, Fernandes, Azevedo and Casal2020).
In this study, myxosporean surveys were performed on oligochaetes collected from another Portuguese estuary, more specifically from the lower estuary of the River Douro, near the city of Porto, northern Portugal. The results reinforce the already recognized rich biodiversity of the sphaeractinomyxon collective group in brackish waters, as four new types are morphologically and molecularly described from a marine oligochaete of the genus Baltidrilus Timm, 2013 (Oligochaeta, Naididae). Molecular analyses matched the SSU rDNA sequences of three of these types with those of mugiliform-infecting Myxobolus spp., thus confirming the involvement of the sphaeractinomyxon morphotype in the life cycle of this specific group of myxobolids. The features of the Myxobolus and sphaeractinomyxon morphotypes are suggested to have optimized transmission between mullets and deposit-feeding oligochaetes, thus having played a crucial role in the hyperdiversification of this myxosporean genus in mugiliform hosts.
Materials and methods
Sampling and morphological characterization
Between September 2014 and April 2015, six sporadic samplings of mud were performed at low tide from a single site (‘Cabedelo’) in the lower estuary of the River Douro (41°08′32″N, 08°39′41″W), northern Portugal. The mud was transported to the laboratory and analysed for the manual collection of oligochaetes, which were then kept separately in 48-well plates containing saltwater at −4°C, for ca. 2 weeks. During this time period, a stereo microscope was used to determine actinospore release into the well-plates. Finally, all oligochaete specimens were examined under a light microscope for the detection of actinospores, and other developmental stages in the coelomic cavity and tissues. As waterborne actinospores were never observed, the prevalence of infection was calculated from specimens in which actinospore development was microscopically detected and molecularly confirmed.
Actinospores were examined and photographed using a Zeiss Axiophot microscope (Grupo Taper, Sintra, Portugal), equipped with a Zeiss AxioCam Icc3 digital camera and the AxioVision 4.6.3 software (Grupo Taper) for image analysis. Morphology and morphometry were determined using fresh material, in accordance with the recommendations given by Lom et al. (Reference Lom, McGeorge, Feist, Morris and Adams1997). Measurements include the mean value ± standard deviation (s.d.), range of variation and number of measured actinospores (n).
Molecular characterization
Infected oligochaetes were kept separately in absolute ethanol until used for molecular analysis. Genomic DNA was extracted using the GenEluteTM Mammalian Genomic DNA Miniprep Kit (Sigma-Aldrich, St Louis, USA), following the manufacturer's instructions. The DNA was stored in 50 μL of TE buffer at −20°C until further use.
A nested PCR was performed in order to amplify the SSU rDNA gene of the actinospores. The first round of PCR used the universal eukaryotic primers ERIB1 and ERIB10 (Table 1) in 25 μL reactions comprised of 0.25 μL of 10 pmol of each primer, 0.2 mm of each dNTP, 2.5 mm of MgCl2, 2.5 μL of 10 × Taq polymerase buffer, 1.25 units Taq DNA polymerase (NZYTech, Lisbon, Portugal), 2 μL (approximately 100–150 ng) of genomic DNA and 18 μL of water. The reactions were run on a Bio-Rad MJ Mini Gradient Thermal Cycler, with initial denaturation at 95°C for 3 min, followed by 35 cycles of 95°C for 1 min, 55°C for 1 min and 72°C for 2 min. The final elongation step was performed at 72°C for 7 min. The second round of PCR was performed using as template 1 μL of the first round PCR and specific myxosporean primers (Table 1). The reaction mixtures were the same as for the first round PCR, except for the usage of 0.5 μL of 10 pmol of each primer. Amplification was achieved using an initial denaturation at 95°C for 3 min, followed by 35 cycles of 94°C for 45 s, 53°C for 45 s and 72°C for 90 s, and a final elongation step at 72°C for 7 min. In turn, the 16S mitochondrial DNA (mtDNA) of the oligochaete hosts was amplified using the universal primers 16sar-L and 16sbr-H (Table 1). PCRs were carried out in a single reaction using the conditions previously described for the second round PCR of the actinospores, with 2 μL of extracted genomic DNA as a template.
Table 1. Polymerase chain reaction primers used for actinospore and oligochaete DNA amplification and sequencing.

Aliquots (5 μL) of the PCR products were electrophoresed through a 1% agarose 1 × tris-acetate-EDTA buffer (TAE) gel stained with GreenSafe Premium (NZYTech). PCR products were purified using Puramag™ magnetic beads coated with carboxylic acid groups (MCLAB, San Francisco, California, USA). Sequencing reactions were performed with the same primers used for amplification (Table 1), using a BigDye Terminator v3.1 Cycle Sequencing Kit from AppliedBiosystems (Thermo Fisher Scientific, Waltham, Massachusetts, USA), and were run on an ABI3700 DNA analyser from AppliedBiosystems (Thermo Fisher Scientific).
Sequence assembly and distance estimation analysis
The partial SSU rDNA sequences obtained for each infected oligochaete were aligned separately in MEGA7 (Kumar et al., Reference Kumar, Stecher and Tamura2016), allowing the construction of assembled sequences that were then compared amongst each other, in order to assertively calculate the prevalence of infection of each actinospore type. A dataset of close relatives was retrieved from GenBank according to the highest similarity scores obtained using BLAST search; it included 61 sequences representing all mugiliform-infecting myxobolids and sphaeractinomyxon types sequenced to date. Sequence alignments were performed using MAFFT version 7 available online, and distance estimation was calculated in MEGA7, using the p-distance model with all ambiguous positions removed for each sequence pair. Cut-off for intraspecific variability was set at 0.2%, in accordance with the range that is generally accepted for myxosporeans: 0‒3.6%, usually lower than 1% (see Atkinson et al., Reference Atkinson, Bartošová-Sojková, Whipps, Bartholomew, Okamura, Gruhl and Bartholomew2015 and references therein).
Results
Myxozoan survey and host recognition
During this study, 720 oligochaete specimens were collected from the lower estuary of the River Douro, northern Portugal. Three morphologically distinct oligochaete species were represented in the mud samples analysed, but myxozoan infection was only detected in an unidentified species of the genus Baltidrilus Timm, 2013 (Oligochaeta, Naididae) that composed ca. 70% of the total oligochaetes examined. Host identification was performed based on the combined analysis of morphological traits and molecular data of the 16S mtDNA gene. All infected specimens shared the same morphological features, which were generally congruent with those described for Baltidrilus costatus (Claparède, 1863), i.e. presence of dorsal palmate chaetae between segments III and XIII, with bifid chaetae in the remaining segments, and absence of hair chaetae and cuticular papillation (Brinkhurst, Reference Brinkhurst1971; Timm, Reference Timm2013). Comparative analysis of the 16S mtDNA sequences obtained from each case isolate revealed them to be identical amongst each other, further sharing 99.1% of similarity to the sequences of B. costatus currently available in GenBank (AY340460 and AY885609). The latter sequence (AY885609) was misidentified as T. pseudogaster but, according to Kvist et al. (Reference Kvist, Sarkar and Erséus2010), actually belongs to B. costatus. Despite the considerable morphological and molecular similarity to B. costatus, identification was performed only at the genus-level. As non-oligochaete taxonomists, we felt unsure in determining whether the genetic distance found between the sequences obtained here and those available in GenBank for B. costatus results from intra- or interspecific variability and, therefore, conservatively identified all infected specimens as belonging to an unidentified Baltidrilus sp. One 16S mtDNA sequence with 529 bp was deposited in GenBank with the accession no. MT624321, and is representative of the identical sequences that were separately obtained for each oligochaete host specimen.
Ten specimens of Baltidrilus sp. displayed myxozoan infection by members of the sphaeractinomyxon collective group, corresponding to an overall prevalence of infection of 1.4%. Infection by any other actinospore collective groups was not found in the oligochaete samples analysed. Specimens displaying sphaeractinomyxon infection were detected throughout the entire sampling period, but waterborne actinospores were never observed in the well-plates. Mature actinospores and other developmental stages were present in the coelomic cavity, having asynchronous development. In turn, the actinospore development within the pansporocysts was synchronous.
Four new types of sphaeractinomyxon could be distinguished based on molecular analysis of the SSU rDNA gene. Morphological comparisons were only performed in relation to the 12 types of sphaeractinomyxon that lack molecular data, i.e. S. stolci, S. gigas, S. danicae, S. ilyodrili, S. amanieui, S. rotundum, S. leptocapsula, Sphaeractinomyxon types 1 and 2 of Hallett et al. (Reference Hallett, Erséus, Lester and Morton1997), Sphaeractinomyxon type B of Hallett and Lester (Reference Hallett and Lester1999), Sphaeractinomyxon type 2 of Hallett et al. (Reference Hallett, Erséus and Lester1999) and Sphaeractinomyxon type of Hallett et al. (Reference Hallett, Erséus, O'Donoghue and Lester2001). Among these, S. amanieui was also originally described from an oligochaete host of the genus Baltidrilus, specifically B. costatus. Notwithstanding, none of the types described here bared resemblance to the actinospores of S. amanieui, which are reported to measure 50–60 μm in diameter (see Puytorac, Reference Puytorac1963). Furthermore, the identification of the host species of S. amanieui as B. costatus is dubious, given that this parasite was reported from freshwater [near Clermont-Ferrand, France] and, while B. costatus is euryhaline, it is commonly associated with brackish and marine coastal waters (Sweeney, Reference Sweeney2016; Knight-Jones et al., Reference Knight-Jones, Knight-Jones, Mortimer-Jones, Nelson-Smith, Schmelz, Timm, Hayward and Ryland2017; Marszewska et al., Reference Marszewska, Dumnicka and Normant-Saremba2017).
Characterization of four novel Sphaeractinomyxon types (Cnidaria, Myxozoa)
Sphaeractinomyxon type 1 (Figs 1A–C and 2A)
Description: Mature actinospores rounded or slightly angular in apical view and rounded to slightly oblong in lateral view, measuring 17.6 ± 0.7 (16.0–18.9) μm in length (n = 12), 18.9 ± 0.8 (17.8–19.9) μm in width (n = 12) and 19.3 ± 0.6 (18.4–20.5) μm in diameter (n = 13). Three polar capsules positioned centrally, pyriform and symmetric, 4.4 ± 0.3 (3.8–4.9) μm long (n = 14) and 3.5 ± 0.2 (3.1–3.8) μm wide (n = 14), each comprising a polar tubule displaying 2–3 longitudinal coils. Sporoplasm containing many secondary cells. Pansporocysts with synchronous development, each containing eight actinospores in equivalent stages.
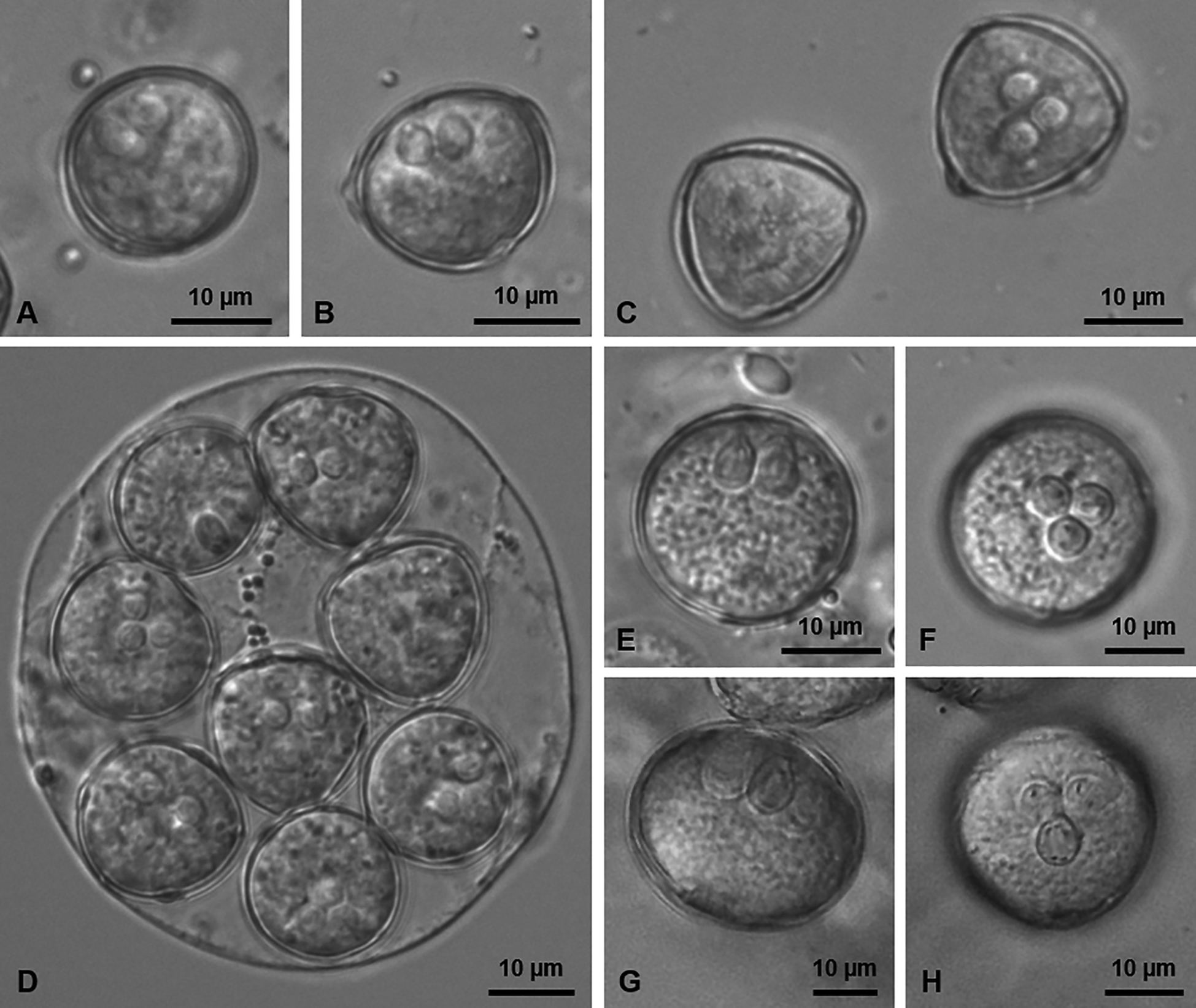
Fig. 1. Light micrographs showing mature actinospores of the new types of sphaeractinomyxon found infecting the coelomic cavity of a Baltidrilus sp. in the lower estuary of the River Douro.
(A‒C) Sphaeractinomyxon type 1 in lateral (A, B) and apical (C) view. (D) Pansporocyst of the Sphaeractinomyxon type 2 showing eight actinospores in different perspectives. (E, F) Sphaeractinomyxon type 3 in lateral (E) and apical (F) view. (G, H) Sphaeractinomyxon type 4 in lateral (G) and apical (H) view.

Fig. 2. Schematic drawings depicting mature actinospores of the new types of sphaeractinomyxon found infecting the coelomic cavity of a Baltidrilus sp. in the lower estuary of the River Douro, as observed in lateral (left) and apical (right) view. (A) Sphaeractinomyxon type 1. (B) Sphaeractinomyxon type 2. (C) Sphaeractinomyxon type 3. (D) Sphaeractinomyxon type 4.
Type host: Species of the genus Baltidrilus Timm, 2013 (Oligochaeta, Naididae).
Type locality: ‘Cabedelo’ (41°08′32″N, 08°39′41″W), lower estuary of the River Douro, northern Portugal.
Site of infection: Throughout the coelomic cavity.
Prevalence: 0.3% (2 infected in 720 oligochaetes examined).
Type material: Series of phototypes of the hapantotype deposited together with a representative DNA sample in the Type Material Collection of the Laboratory of Animal Pathology, Interdisciplinary Centre of Marine and Environmental Research, Porto, Portugal, reference CIIMAR 2019.52.
Molecular data: One SSU rDNA sequence with 2014 bp, deposited in GenBank with the accession no. MN037506. The latter is representative of two identical sequences that were separately obtained from the actinospore developmental stages in the coelomic cavities of two infected oligochaetes.
Remarks: Morphometry was determined from mature actinospores observed in both infected hosts, among which morphological variation was not registered. Morphological comparison showed no gross similarity between the parasite in study and the 12 types of sphaeractinomyxon that lack molecular data. In turn, distance estimation analysis revealed 100% of similarity to the SSU rDNA sequences of Myxobolus mugiliensis Rocha et al., 2019 parasitizing the gills of flathead grey mullet Mugil cephalus Linnaeus, 1758 (Mugiliformes, Mugilidae) in the River Minho estuary (northern Portugal) (MK203082), and in the Spanish Mediterranean coast (MF118767) (see Sharon et al., Reference Sharon, Ucko, Tamir and Diamant2019; Rocha et al., Reference Rocha, Casal, Alves, Antunes, Rodrigues and Azevedo2019d). A similarity value of 99.8% was further determined in relation to a third SSU rDNA sequence of M. mugiliensis that is available from infections in M. cephalus from the Samsun coasts of the Black Sea in Turkey (MH392320), where Baltidrilus spp. are also present in the annelid community (Arslan, Reference Arslan2006). All other SSU rDNA sequences included in the analysis retrieved similarity values lower than 98.0%. Sphaeractinomyxon types genetically more similar were the Sphaeractinomyxon type 2 of Rangel et al. (Reference Rangel, Castro, Rocha, Severino, Casal, Azevedo, Cavaleiro and Santos2016a) (KU569311) (97.5%) and the Sphaeractinomyxon type 2 reported in this study (MN037503) (96.6%).
Sphaeractinomyxon type 2 (Figs 1D and 2B)
Description: Mature actinospores subspherical in apical and lateral view, measuring 18.1 ± 0.7 (17.0–19.6) μm in length (n = 19), 19.1 ± 0.5 (17.7–19.8) μm in width (n = 19) and 19.6 ± 0.7 (18.0–20.9) μm in diameter (n = 17). Three polar capsules positioned centrally, pyriform and symmetric, 4.7 ± 0.5 (4.1–5.4) μm long (n = 12) and 3.3 ± 0.2 (2.9–3.7) μm wide (n = 12), each comprising a polar tubule displaying 2–3 longitudinal coils. Sporoplasm containing many secondary cells. Pansporocysts with synchronous development, each containing eight actinospores in equivalent stages.
Type host: Species of the genus Baltidrilus Timm, 2013 (Oligochaeta, Naididae).
Type locality: ‘Cabedelo’ (41°08′32″N, 08°39′41″W), lower estuary of the River Douro, northern Portugal.
Site of infection: Throughout the coelomic cavity.
Prevalence: 0.3% (2 infected in 720 oligochaetes examined).
Type material: Series of phototypes of the hapantotype deposited together with a representative DNA sample in the Type Material Collection of the Laboratory of Animal Pathology, Interdisciplinary Centre of Marine and Environmental Research, Porto, Portugal, reference CIIMAR 2019.53.
Molecular data: One SSU rDNA sequence with 2020 bp, deposited in GenBank with the accession no. MN037503. The latter is representative of two identical sequences that were separately obtained from the actinospore developmental stages in the coelomic cavities of two infected oligochaetes.
Remarks: Morphometry was determined from mature actinospores observed in both infected hosts, among which morphological variation was not registered. Morphological comparison showed no gross similarity to the 12 types of sphaeractinomyxon that lack molecular data. In turn, distance estimation revealed 99.8 and 99.6% of similarity to the SSU rDNA sequences of a Myxobolus sp. reported from the muscle and kidney of M. cephalus in the Spanish Mediterranean coast (MF118773 and MF118771, respectively) (see Sharon et al., Reference Sharon, Ucko, Tamir and Diamant2019). All other SSU rDNA sequences included in the analysis retrieved similarity values lower than 98.0%. Sphaeractinomyxon types genetically more similar were the Sphaeractinomyxon type 2 reported in this study (MN037506) (96.6%) and the Sphaeractinomyxon type 1 of Rangel et al. (Reference Rangel, Castro, Rocha, Severino, Casal, Azevedo, Cavaleiro and Santos2016a) (KU569311) (95.8%).
Sphaeractinomyxon type 3 (Figs 1E–F and 2C)
Description: Mature actinospores spherical in apical and lateral view, measuring 21.6 ± 1.2 (19.1–23.7) μm in length (n = 30), 22.6 ± 0.9 (20.7–25.2) μm in width (n = 30) and 23.0 ± 0.8 (21.7–24.9) μm in diameter (n = 19). Three polar capsules positioned centrally, pyriform and symmetric, 5.8 ± 0.4 (4.9–7.1) μm long (n = 34) and 4.4 ± 0.3 (3.7–5.0) μm wide (n = 34), each comprising a polar tubule displaying 2–3 longitudinal coils. Sporoplasm containing many secondary cells. Pansporocysts with synchronous development, each containing eight actinospores in equivalent stages.
Type host: Species of the genus Baltidrilus Timm, 2013 (Oligochaeta, Naididae).
Type locality: ‘Cabedelo’ (41°08′32″N, 08°39′41″W), lower estuary of the River Douro, northern Portugal.
Site of infection: Throughout the coelomic cavity.
Prevalence: 0.3% (2 infected in 720 oligochaetes examined).
Type material: Series of phototypes of the hapantotype deposited together with a representative DNA sample in the Type Material Collection of the Laboratory of Animal Pathology, Interdisciplinary Centre of Marine and Environmental Research, Porto, Portugal, reference CIIMAR 2019.54.
Molecular data: One SSU rDNA sequence with 2023 bp, deposited in GenBank with the accession no. MN037504. The latter is representative of two identical sequences that were separately obtained from the actinospore developmental stages in the coelomic cavities of two infected oligochaetes.
Remarks: Morphometry was determined from mature actinospores observed in both infected hosts, among which morphological variation was not registered. Morphological comparison to the 12 types of sphaeractinomyxon that lack molecular data revealed some similarity to S. leptocapsula and S. rotundum. The actinospores of S. leptocapsula, however, are overall smaller than those in the study (19 μm in length × 21 μm in width × 22 μm in diameter), further differing by being triangular in apical view and ellipsoidal to broad pyriform in lateral view (see Hallett et al., Reference Hallett, Erséus and Lester1999). In turn, despite the actinospores of S. rotundum also being spherical, they differ in being shorter (17 μm long) and having bigger polar capsules (7 μm long × 5 μm wide) (see Marques, Reference Marques1984). The sequence obtained for the parasite did not match any of the SSU rDNA sequences currently available for myxozoans. Distance estimation showed closest similarity to all other sphaeractinomyxon types and Myxobolus spp. reported from mullets, namely Sphaeractinomyxon type 2 of Rocha et al. (Reference Rocha, Rangel, Castro, Severino, Azevedo, Santos and Casal2019c) (MH017877) (97.2%), Sphaeractinomyxon type 9 of Rangel et al. (Reference Rangel, Castro, Rocha, Severino, Casal, Azevedo, Cavaleiro and Santos2016a) (KU569318) (96.5%) and a Myxobolus sp. reported from the gills, intestine and tail of M. cephalus from the Mediterranean Sea off Northern Israel (MF118765) (96.0%). All other SSU rDNA sequences included in the analysis retrieved similarity values lower than 96.0%.
Sphaeractinomyxon type 4 (Figs 1G–H and 2D)
Description: Mature actinospores spherical in apical view and ellipsoidal in lateral view, measuring 24.5 ± 1.8 (23.6–27.2) μm in length (n = 4), 26.5 ± 1.2 (25.3–28.1) μm in width (n = 5) and 26.2 ± 0.9 (24.6–27.5) μm in diameter (n = 8). Three polar capsules positioned centrally, pyriform and symmetric, 7.2 ± 0.6 (6.2–7.9) μm long (n = 8) and 5.5 ± 0.2 (5.3–5.9) μm wide (n = 8), each comprising a polar tubule displaying 2–3 longitudinal coils. Sporoplasm containing many secondary cells. Pansporocysts with synchronous development, each containing eight actinospores in equivalent stages.
Type host: Species of the genus Baltidrilus Timm, 2013 (Oligochaeta, Naididae).
Type locality: ‘Cabedelo’ (41°08′32″N, 08°39′41″W), lower estuary of the River Douro, northern Portugal.
Site of infection: Throughout the coelomic cavity.
Prevalence: 0.6% (4 infected in 720 oligochaetes examined).
Type material: Series of phototypes of the hapantotype deposited together with a representative DNA sample in the Type Material Collection of the Laboratory of Animal Pathology, Interdisciplinary Centre of Marine and Environmental Research, Porto, Portugal, reference CIIMAR 2019.55.
Molecular data: One SSU rDNA sequence with 1954 bp, deposited in GenBank with the accession no. MN037505. The latter is representative of four identical sequences that were separately obtained from the actinospore developmental stages in the coelomic cavities of four infected oligochaetes.
Remarks: Morphometry was determined from one of the four infected hosts, in which fully matured actinospores could be measured. Morphological comparison to the 12 types of sphaeractinomyxon that lack molecular data revealed some similarity to the Sphaeractinomyxon type B of Hallett and Lester (Reference Hallett and Lester1999). Nonetheless, the latter can be distinguished from the type in study based on original host, type locality and morphology of its polar capsules, which are spherical, smaller (~4 μm in diameter) and have a higher number of polar tubule coils (3 minimum) (Hallett and Lester, Reference Hallett and Lester1999). Distance estimation analysis revealed the parasite sharing 99.8% of similarity with the sequence of Myxobolus labrosus Rocha et al., 2019 from the urinary bladder of thicklip grey mullet Chelon labrosus (Risso, 1827) (Mugiliformes, Mugilidae) in another Portuguese northern River (MK203081) (see Rocha et al., Reference Rocha, Casal, Alves, Antunes, Rodrigues and Azevedo2019d). All other SSU rDNA sequences included in the analysis retrieved similarity values lower than 95.0%. Sphaeractinomyxon types genetically more similar were the Sphaeractinomyxon type 1 of Rocha et al. (Reference Rocha, Alves, Antunes, Fernandes, Azevedo and Casal2020) (MK418446) (94.2%) and the Sphaeractinomyxon type 1 of Rocha et al. (Reference Rocha, Rangel, Castro, Severino, Azevedo, Santos and Casal2019c) (MH017876) (94.0%).
Discussion
During this study, four new types of sphaeractinomyxon were found infecting a marine oligochaete species of the genus Baltidrilus in the lower estuary of the River Douro, Northern Portugal. Their development in the coelomic cavity of infected specimens followed the pattern that has been commonly reported for members of the sphaeractinomyxon collective group (e.g. Caullery and Mesnil, Reference Caullery and Mesnil1905; Hallett et al., Reference Hallett, O'Donoghue and Lester1998; Rangel et al., Reference Rangel, Castro, Rocha, Cech, Casal, Azevedo, Székely, Cavaleiro and Santos2016b; Rocha et al., Reference Rocha, Rangel, Castro, Severino, Azevedo, Santos and Casal2019c), i.e. generalized asynchrony of the overall parasitic development, and synchrony of the sporogonic phase within the pansporocysts. The low individual values of prevalence of infection determined for these types were also congruent with those previously reported for other types of sphaeractinomyxon in Portuguese estuaries, and other geographic locations (e.g. Hallett et al., Reference Hallett, Erséus and Lester1999; Rangel et al., Reference Rangel, Castro, Rocha, Cech, Casal, Azevedo, Székely, Cavaleiro and Santos2016b; Rocha et al., Reference Rocha, Rangel, Castro, Severino, Azevedo, Santos and Casal2019c, Reference Rocha, Alves, Antunes, Fernandes, Azevedo and Casal2020). In turn, the value obtained for the overall prevalence of infection of the collective group differed significantly from the values previously reported for the same type of myxozoan survey in the Aveiro (18.7%), Alvor (6.1%) and Minho estuaries (0.2%) (see Rangel et al., Reference Rangel, Castro, Rocha, Cech, Casal, Azevedo, Székely, Cavaleiro and Santos2016b; Rocha et al., Reference Rocha, Rangel, Castro, Severino, Azevedo, Santos and Casal2019c, Reference Rocha, Alves, Antunes, Fernandes, Azevedo and Casal2020). Although little is known about myxosporean–annelid interactions, these value differences most likely reflect the complexity and variability of the biotic and abiotic factors that shape the ecosystem and naturally influence the encounter rates between myxospores and permissive annelid hosts. These factors may include the composition, distribution and permissiveness of oligochaete communities, pressure exerted by predator foraging, nature of the substratum, water temperature, depth and flow, among others (Alexander et al., Reference Alexander, Kerans, El-Matbouli, Hallett, Stevens, Okamura, Gruhl and Bartholomew2015).
In general, it is not uncommon for multiple actinospore types to use the same oligochaete species. For instance, El-Mansy et al. (Reference El-Mansy, Székely and Molnár1998) reported more than a dozen types of aurantiactinomyxon and neoactinomyxum parasitizing the freshwater oligochaete Branchiura sowerbyi Beddard, 1892 (Oligochaeta, Naididae) in a Hungarian fish farm. Xiao and Desser (Reference Xiao and Desser1998a, Reference Xiao and Desser1998b) described types of aurantiactinomyxon, neoactinomyxum, raabeia and triactinomyxon infecting the freshwater species Limnodrilus hoffmeisteri Claparède, 1862 (Oligochaeta, Naididae) in natural waters of the Algonquin Park, Ontario; and Rosser et al. (Reference Rosser, Griffin, Quiniou, Greenway, Khoo, Wise and Pote2014) reported types of the aurantiactinomyxon, helioactinomyxon, raabeia and triactinomyxon collective groups parasitizing specimens of the freshwater oligochaete Dero digitata (Müller, 1774) (Oligochaeta, Naididae) in a commercial catfish pond in the Mississippi Delta. Similarly, several types of sphaeractinomyxon have been reported to occur in the same host species: eight types were reported from T. pseudogaster, four types from T. insularis and three types from L. agnes (Rangel et al., Reference Rangel, Castro, Rocha, Cech, Casal, Azevedo, Székely, Cavaleiro and Santos2016b; Rocha et al., Reference Rocha, Rangel, Castro, Severino, Azevedo, Santos and Casal2019c, Reference Rocha, Alves, Antunes, Fernandes, Azevedo and Casal2020). In this study, four new types are described from a single Baltidrilus sp., suggesting this oligochaete as another highly susceptible naidid for the acquisition and development of myxozoan infection by members of the sphaeractinomyxon collective group. This supports the broadly documented usage of naidids [including ‘tubificoid naidids’ (Erséus et al., Reference Erséus, Wetzel and Gustavsson2008)] as annelid hosts for myxozoans (Alexander et al., Reference Alexander, Kerans, El-Matbouli, Hallett, Stevens, Okamura, Gruhl and Bartholomew2015; Rocha et al., Reference Rocha, Alves, Antunes, Azevedo and Casal2019a, Reference Rocha, Alves, Fernandes, Antunes, Azevedo and Casal2019b, Reference Rocha, Alves, Antunes, Fernandes, Azevedo and Casal2020). Moreover, it increases significantly the number of types of sphaeractinomyxon, and other collective groups in general, which use marine oligochaetes as definitive hosts in estuarine/marine habitats (see Rangel et al., Reference Rangel, Rocha, Castro, Severino, Casal, Azevedo, Cavaleiro and Santos2015, Reference Rangel, Castro, Rocha, Cech, Casal, Azevedo, Székely, Cavaleiro and Santos2016b, Reference Rangel, Rocha, Casal, Castro, Severino, Azevedo, Cavaleiro and Santos2017; Rocha et al., Reference Rocha, Alves, Antunes, Azevedo and Casal2019a, Reference Rocha, Rangel, Castro, Severino, Azevedo, Santos and Casal2019c, Reference Rocha, Alves, Antunes, Fernandes, Azevedo and Casal2020). The absence of mixed infections in the Baltidrilus specimens analysed is in accordance with what has been reported for other highly permissive oligochaete species in the above-mentioned studies (El-Mansy et al., Reference El-Mansy, Székely and Molnár1998; Xi et al., Reference Xi, Zhang, Xie, Pan, Xu and Ge2013, Reference Xi, Zhou, Xie, Pan, Yang and Ge2015; Rosser et al., Reference Rosser, Griffin, Quiniou, Greenway, Khoo, Wise and Pote2014; Rangel et al., Reference Rangel, Castro, Rocha, Cech, Casal, Azevedo, Székely, Cavaleiro and Santos2016b; Rocha et al., Reference Rocha, Rangel, Castro, Severino, Azevedo, Santos and Casal2019c, Reference Rocha, Alves, Antunes, Fernandes, Azevedo and Casal2020), and may also be suggested to be the outcome of specific host-, parasite- and environmental-related factors regulating myxosporean–annelid interactions (see Alexander et al., Reference Alexander, Kerans, El-Matbouli, Hallett, Stevens, Okamura, Gruhl and Bartholomew2015 and references therein). Considering the potential relevance of these factors in the successful dissemination, establishment and evolution of myxosporeans, future research should aim to provide insight into their real diversity and influence.
Acknowledging the widely reported artificiality of using morphological criteria for distinguishing between members of sphaeractinomyxon (Rangel et al., Reference Rangel, Castro, Rocha, Cech, Casal, Azevedo, Székely, Cavaleiro and Santos2016b; Rocha et al., Reference Rocha, Rangel, Castro, Severino, Azevedo, Santos and Casal2019c, Reference Rocha, Alves, Antunes, Fernandes, Azevedo and Casal2020), and other actinospore collective groups in general (e.g. Hallett et al., Reference Hallett, Atkinson and El-Matbouli2002, Reference Hallett, Atkinson, Erséus and El-Matbouli2004; Eszterbauer et al., Reference Eszterbauer, Marton, Rácz, Letenyei and Molnár2006; Caffara et al., Reference Caffara, Raimondi, Florio, Marcer, Quaglio and Fioravanti2009; Xi et al., Reference Xi, Zhou, Xie, Pan, Yang and Ge2015), differentiation between the four new types was mainly based on molecular data of the SSU rDNA gene. Indeed, types 1 and 2 could only be distinguished through comparison of their respective SSU rDNA sequences, given that their measurements considerably overlapped amongst each other. This reinforces the inaccuracy of using solely morphology-based criteria for distinguishing between types of the same collective group, especially when developing in the same oligochaete species.
Molecular comparison of the novel SSU rDNA sequences to others available in GenBank further revealed three of the four new types as life cycle counterparts to the mugiliform-infecting species M. mugiliensis, M. labrosus and an unnamed Myxobolus sp. from the muscle and kidney of M. cephalus in the Spanish Mediterranean coast (Sharon et al., Reference Sharon, Ucko, Tamir and Diamant2019). These life cycle inferences are the first to be recognized among mugiliform-infecting Myxobolus spp., and confirm the previously hypothesized involvement of sphaeractinomyxon as actinosporean counterparts for this specific group of myxosporeans (see Rocha et al., Reference Rocha, Rangel, Castro, Severino, Azevedo, Santos and Casal2019c).
To date, 51 species of Myxobolus have been reported from mugiliform hosts worldwide (see Marcotegui and Martorelli, Reference Marcotegui and Martorelli2017; Cardim et al., Reference Cardim, Silva, Hamoy, Matos and Abrunhosa2018; Rocha et al., Reference Rocha, Casal, Alves, Antunes, Rodrigues and Azevedo2019d, Reference Rocha, Azevedo, Oliveira, Alves, Antunes, Rodrigues and Casal2019e). Of these, 20 are known to occur in the Iberian Peninsula, parasitizing mullets that were either captured from the estuary of the River Minho in northern Portugal (Rocha et al., Reference Rocha, Casal, Alves, Antunes, Rodrigues and Azevedo2019d, Reference Rocha, Azevedo, Oliveira, Alves, Antunes, Rodrigues and Casal2019e), or from the Spanish Mediterranean coast (Yurakhno and Ovcharenko, Reference Yurakhno and Ovcharenko2014; Sharon et al., Reference Sharon, Ucko, Tamir and Diamant2019). This includes M. mugiliensis, M. labrosus and the Myxobolus sp., which life cycles are clarified in this study. Among this group, only Myxobolus adeli (Isjumova, 1964) Yurakhno and Ovcharenko, Reference Yurakhno and Ovcharenko2014, Myxobolus nile Negm-Eldin et al., 1999, and Myxobolus rohdei Lom and Dyková et al., 1994 are without molecular data and, therefore, cannot be molecularly compared to sphaeractinomyxon sequences. Considering that this study elevates to 21, the number of sphaeractinomyxon types molecularly described from Portuguese estuaries, and that each one of these types is presumably counterpart to a mugiliform-infecting Myxobolus sp., it can be affirmed that the biodiversity of this myxosporean genus in mullets remains underrated.
Holzer et al. (Reference Holzer, Bartošová-Sojková, Born-Torrijos, Lövy, Hartigan and Fiala2018) showed that the acquisition of fish as second hosts enabled myxozoans with alternative transmission and dispersion strategies that were crucial in the conquest of new habitats. Acknowledging this, Rocha et al. (Reference Rocha, Alves, Antunes, Fernandes, Azevedo and Casal2020) correlated the broader host range and wider geographic distribution of the Sphaeractinomyxon type 10 of Rangel et al. (Reference Rangel, Castro, Rocha, Cech, Casal, Azevedo, Székely, Cavaleiro and Santos2016b), and of the Sphaeractinomyxon type 4 of Rocha et al. (Reference Rocha, Alves, Antunes, Fernandes, Azevedo and Casal2020), with the ecological plasticity of the fish hosts sheltering their myxospore counterparts. The present study supports this contention, given the highly adaptable ecological nature of mullets (Cardona, Reference Cardona2001, Reference Cardona2006; Almeida, Reference Almeida2003; Crosetti and Blaber, Reference Crosetti and Blaber2015), and the distinct geographic locations from which the SSU rDNA sequences of the myxospores and actinospores of M. mugiliensis, M. labrosus and Myxobolus sp. were obtained. Thus, it is clear that the acquisition of mullets as second hosts allowed Myxobolus spp. to cross environmental barriers and conquer new geographic habitats, following adaptation of their sphaeractinomyxon counterparts to available invertebrate communities and environmental-related factors.
Longshaw and Feist (Reference Longshaw and Feist2005) proposed that actinospore morphology is a function of the environment, and that each actinospore type will not necessarily have a specific corresponding myxospore stage. Myxosporean phylogenetic analyses widely agree with this assumption, mostly showing actinospore morphotypes spanning over several different myxosporean taxa (e.g. Eszterbauer et al., Reference Eszterbauer, Marton, Rácz, Letenyei and Molnár2006; Fiala et al., Reference Fiala, Bartošová-Sojková, Okamura, Hartikainen, Okamura, Gruhl and Bartholomew2015; Xi et al., Reference Xi, Zhou, Xie, Pan, Yang and Ge2015; Rangel et al., Reference Rangel, Rocha, Casal, Castro, Severino, Azevedo, Cavaleiro and Santos2017; Rocha et al., Reference Rocha, Rangel, Castro, Severino, Azevedo, Santos and Casal2019c). Sphaeractinomyxon have been reported to constitute the only exception to this trend, given their consistent clustering within the monophyletic clade of mugiliform-infecting Myxobolus spp. (see Rocha et al., Reference Rocha, Rangel, Castro, Severino, Azevedo, Santos and Casal2019c). The life cycle inferences performed in this study confirm the evolutionary link between these specific actinospore and myxospore morphotypes, allowing us to begin understanding the functional role of the sphaeractinomyxon morphotype in myxosporean life cycles.
Despite sharing main morphological features, myxospores and actinospores exhibit a wide range of distinctive morphotypes (Lom and Dyková, Reference Lom and Dyková2006). While myxospores are resistant, and mostly adapted for settling, actinospores are short-lived and generally have inflatable valvular processes that provide floatability in order to increase encounter rates with permissive fish hosts (Yokoyama et al., Reference Yokoyama, Ogawa and Wakabayashi1993; Xiao and Desser, Reference Xiao and Desser1998a; Lom and Dyková, Reference Lom and Dyková2006). The species richness of the genus Myxobolus proves the evolutionary success of its morphotype for achieving transmission and infection in permissive oligochaete hosts. Besides being negatively buoyant due to their spherical shape and reduced contact surface, the myxospores of Myxobolus have been reported to be adapted for deposition with small particles, thus increasing the likelihood of consumption by permissive deposit-feeding annelids (Lemmon and Kerans, Reference Lemmon and Kerans2001; Kerans and Zale, Reference Kerans and Zale2002), such as Tubificoides, Baltidrilus and other naidids in general. In the case of sphaeractinomyxon, however, the previous lack of information regarding their role in myxosporean life cycles precluded us from acknowledging the functionality of its morphotype. Members of sphaeractinomyxon differ from other actinospore morphotypes by their lack of valvular processes (Caullery and Mesnil, Reference Caullery and Mesnil1904, Reference Caullery and Mesnil1905; Lom and Dyková, Reference Lom and Dyková2006), which suggests a limited capability for floatation. Taking into consideration the biological context provided by our study, this limited floatability may be suggested to reflect the evolution of functional features optimizing transmission to mullet hosts. As benthic feeders, mullets already have increased proximity to potentially infected annelids (Rocha et al., Reference Rocha, Azevedo, Oliveira, Alves, Antunes, Rodrigues and Casal2019e) and, therefore, are prone to contact with non-buoyant sphaeractinomyxon actinospores. Moreover, the consumption of infected annelids should not be disregarded as a potential viable route for these fish to acquire myxosporean infection, given the variety of living organisms that compose their diet (Cardona, Reference Cardona2001; Laffaille et al., Reference Laffaille, Feunteun, Lefebvre, Radureau, Sagan and Lefeuvre2002; Almeida, Reference Almeida2003). Although the gills, skin and buccal cavity have been recognized to constitute the main portals of entry for myxosporeans in fish hosts (e.g. Yokoyama and Urawa, Reference Yokoyama and Urawa1997; Antonio et al., Reference Antonio, El-Matbouli and Hedrick1999; Holzer et al., Reference Holzer, Sommerville and Wootten2003), infection via the intestine has also been demonstrated (Belém and Pote, Reference Belem and Pote2001).
Overall, it appears that the sphaeractinomyxon morphotype is highly adapted to the ecology of bottom-dwelling fish (namely mullets), allowing for an effective transmission, and for an evolutionary successful myxosporean–host relationship that most likely contributed significantly for the hyperdiversification of Myxobolus in mullets. As more life cycles are resolved, and additional myxospore–actinospore morphotype correlations are acknowledged, future research might aim to study morphotype evolution in the context of their interactions with susceptible hosts and abiotic-related factors.
Acknowledgments
The authors thank Thais Oliveira and Ricardo Castro for the mud collection and sampling of oligochaetes, and Miguel Pereira for his assistance in figure preparation.
Financial support
This work was supported by the Foundation for Science and Technology (Lisbon, Portugal) within the scope of the Ph.D. fellowship grant attributed to S. Rocha (SFRH/BD/92661/2013) through the programme QREN-POPH/FSE; the Eng° António de Almeida Foundation (Porto, Portugal); CIIMAR strategic Project, from FCT, with reference: UID/Multi/04423/2019.
Conflict of interest
None.
Ethical standards
None.